ABSTRACT
This study aimed to evaluate the antitumor effect of chlorogenic acid (CGA) alone and in combination with doxorubicin (DOX) in solid Ehrlich carcinoma (SEC) model in mice. In addition, we investigated the possible cardioprotective effect of CGA against DOX-induced cardiotoxicity. CGA, DOX and their combination were given for 21 days in mice bearing SEC. Tumor volume and weight were measured, and serum CRP was determined by latex immunoassay. Moreover, mRNA expressions of TRAIL, TRAILR2, FasL, Fas, caspase-3 and Bcl-2 were measured using RT-PCR. Histopathological examination of tumor and cardiac tissues was carried out using hematoxylin and eosin. Active caspase-3 was detected by immunohistochemistry. CGA and/or DOX treatment showed a remarkable decrease in solid tumor volume and weight. CGA and/or DOX groups revealed upregulation in gene expressions of TRAIL/TRAILR2, FasL/Fas and caspase-3 genes and downregulation of Bcl-2 gene expression, as well as a marked increase in active caspase-3 expression. Moreover, CGA and DOX combination significantly decreased MDA and increased GSH levels in tumor tissues and also reduced the level of serum CRP, CK-MB and LDH as compared to DOX group. In conclusion, CGA has the ability not only to enhance the anti-tumor activity of DOX but also protects against DOX-induced cardiotoxicity.
Introduction
Cancer is the main public health problem in the world and the second leading cause of death after heart disease [Citation1]. Chemotherapeutic drugs are associated with serious side effects as they are harmful to both normal and tumor cells [Citation2]. In addition, generation of resistance against chemotherapy limits the efficacy of anti-cancer drugs [Citation3].
Doxorubicin (DOX), an anthracycline anticancer drug, is widely used as the main component in the therapy of many types of cancer such as breast, ovary, lung, liver cancers and leukemia [Citation4]. The antitumor mechanism involves the production of reactive oxygen species (ROS), which affect DNA and cell membranes, intercalation between DNA bases and inhibition of topoisomerase II activity resulting in impairing DNA synthesis [Citation5,Citation6]. Cardiotoxicity is the life-threatening side effect of DOX, which is mainly mediated by ROS generation [Citation7]. Therefore, there is a demanding need for the development of safe and effective strategies that allow decreasing the dose and protection of noncancerous tissues.
Chlorogenic acid (CGA) is a dietary polyphenol compound that is found in coffee and is an important ingredient of green coffee beans [Citation8]. It is also present in numerous types of fruits and vegetables such as cherries, apples, plums, berries, apricots, tomatoes and potatoes [Citation9].
CGA has been reported to display a wide spectrum of biological activities such as anticancer [Citation10], antioxidant [Citation11], anti-inflammatory [Citation12] and cardioprotective activities [Citation13]. In addition, CGA acts as an effective chemosensitizing agent leading to suppression of cancer growth depending on its ability to activate and inhibit some important pathways in cancer metabolism [Citation14]. However, the antitumor molecular mechanisms of CGA are not well understood.
Apoptosis is an essential programmed cell death pathway which is regulated by many factors including the death receptors and Bcl-2 family members. Death receptors are members of tumor necrosis factor (TNF) receptor superfamily including Fas and TNF-related apoptosis-inducing ligand receptor (TRAIL-R) 1 and TRAIL-2. Activation of these receptors by their corresponding ligands results in the formation of death-inducing signaling complex that induces caspase activation and induction of apoptosis cascade [Citation15–Citation17].
On the other hand, the overexpression of antiapoptotic proteins B cell lymphoma-2 (Bcl-2) family like Bcl-2 and its relative Bcl-XL prevent apoptosis leads to the development of tumor cells resistant to chemotherapy [Citation18].
Therefore, the aim of this study was to evaluate the antitumor activities of CGA alone and in combination with DOX in solid Ehrlich carcinoma (SEC) model and explore the molecular mechanism. Moreover, the present study aimed to explore whether CGA has a protective effect against DOX-induced cardiotoxicity in treated mice.
Materials and methods
Drugs and chemicals
DOX HCl (Adricin) was obtained from EIMC United Pharmaceuticals (Badr city, Cairo, Egypt). CGA was obtained from Sigma-Aldrich (St Louis, MO, USA, C3878).
Animals
Fifty-four Swiss female albino mice weighing 20–25 g were used in this study. Mice were maintained on a regular 12-h light/12-h dark cycle with controlled temperature 25 ± 2°C and humidity 55 ± 5% and allowed to diet and water ad libitum. They were kept 2 weeks for acclimatization. The study protocol complies with the ethical principles and guidelines for the care and use of laboratory animals adopted by the Scientific Research Ethics Committee, Faculty of Pharmacy, Mansoura University which is in accordance with ‘Principles of laboratory Animal Care’ (National Materials Institute of Health publication No. 85-23, revised 1985).
Ehrlich solid tumor model
Ehrlich ascites carcinoma (EAC) cells were obtained from the National Cancer Institute, Cairo University, Egypt, 87032503. The cells were maintained by weekly serial intraperitoneal transplantation of cells in female Swiss albino mice [Citation19,Citation20]. The ascites fluid was obtained from the peritoneal cavity after 7 days of inoculation under aseptic conditions using needle aspiration. Ascitic tumor cell counts were performed in a hemocytometer and tested for viability using trypan blue. Then, they were suspended in normal saline so that each 100 µL contained 5 × 105 viable EAC cells.
Induction of solid tumor
Solid tumors were induced in mice by subcutaneous (S.C.) injection of 100 µL containing 5 × 105 viable EAC cells/mice into the right hind limb (thigh) of all animals [Citation21] except normal control group.
The solid tumor volume was measured using a digital caliper to assess tumor growth and was calculated by the following formula: A× B2 × 0.5, where A is the longest diameter of the tumor and B is its shortest perpendicular diameter [Citation22].
Animals were divided into the following groups after 5 days of tumor induction (day 0) when solid tumor reached size (50–100 mm3):
Normal control group (n = 8): mice received 100 µL (0.5% carboxy methyl cellulose (CMC)) per day orally for 21 days from the first day of the experiment.
SEC group (n = 13): mice received no treatment all over the experiment.
CGA group (n = 11): mice received CGA (60 mg/kg suspended in 0.5% CMC) per day orally [Citation23] for 21 days from the first day of the experiment.
DOX group (n = 11): mice received DOX (2 mg/kg) per day by intraperitoneal (i.p.) injection [Citation24] for 21 days from the first day of the experiment.
CGA/DOX group (n = 11): mice received CGA (60 mg/kg suspended in 0.5% CMC per day, orally) 2 h before receiving DOX (2mg/kg/day, i.p.) for 21 days from the first day of the experiment.
Measurement of tumor volume was carried out from the 5th day of tumor inoculation and evaluated every 5 days for a period of 21 days. Then, mice were sacrificed on day 22. Three mice were left in each group for observing survival time.
Mean survival time (MST) and percentage increase in life span (%ILS) were estimated by allowing animals to reach natural death and were calculated through the following formulas:
MST = Σ [survival time (days) of each mouse in a group]/(total number of mice)
% ILS = [(MST of treated mice)/(MST of control group)] × 100
Collection of samples
On day 22, animals were sacrificed under thiopental anesthesia and blood was collected via retro-orbital puncture. The serum was separated by centrifugation of clotted blood samples and stored at −80°C for further analysis.
Next, tumors were removed, weighed and divided into three sections. One section was preserved in buffered formalin (pH 7.2) for histopathological examination and immunohistochemistry. The second section was kept in liquid nitrogen for RT-PCR. The third part was homogenized in phosphate-buffered saline for further biochemical analysis.
Hearts of mice were isolated and fixed in 10%formalin for histopathology.
Histopathological analysis
Tumor and heart tissues were removed and preserved in 10% neutral buffered formalin solution; then, they were incorporated in paraffin for histological and immunohistochemical analyses. Five-micrometer sections were stained with hematoxylin and eosin. The slides were examined for histopathological changes using a BX51 Olympus optical microscope (Olympus Corporation, Tokyo, Japan) and photographed using a digital camera (Nikon digital camera, Japan).
Immunohistochemical analysis of active caspase-3
Five-micrometer-thick solid tumor sections were dissected, de-paraffinized, rehydrated, incubated with citrate buffer for antigen retrieval and eventually blocked with 3% hydrogen peroxide solution. The slides were then incubated overnight with primary antibodies against activated caspase-3 in 1:100 dilution (Diagnostic Biosystems, USA, Mob 309, IgG2a) in a humidified chamber. Then, the slides were incubated with horseradish peroxidase– conjugated secondary antibodies (Vector Laboratories INC., CA, USA, catalog no. PI-2000, IgG). The visualization of the immune reaction was performed using diaminobenzidine reagent (Genemed, Biotechnologies INC., USA) and then counter-staining with hematoxylin. Subsequently, slides were visualized under a light microscope (Olympus BX51, Olympus, Tokyo, Japan). The number of positive cells were counted in 10 high-power fields and the apoptotic index was calculated.
Serum C-Reactive Protein (CRP) level
CRP was detected using a kit purchased from Spinreact Co, Spain (Ref. 1200301). The CRP reagent is a suspension of polystyrene latex particles, coated with goat IgG anti-CRP. Latex particles are agglutinated when mixed with samples (mouse samples) containing CRP. The presence of agglutination refers to a level of CRP equal to or greater than 6 mg/L.
Colorimetric assay
Tissue homogenates were used for measurement of malondialdehyde (MDA) and glutathione (GSH) levels for estimation of oxidative stress, following the manufacturer’s instructions (Biodiagnostic Company, Dokki, Giza, Egypt). Moreover, the serum levels of creatine kinase-MB (CK-MB) (Spectrum Diagnostics company, Egypt, Catalog no. 239 001) and lactate dehydrogenase (LDH) (Human Diagnostics company, Germany, Catalog no. 12214) were estimated by the colorimetric method, according to manufacturer’s protocols for assessing cardiotoxicity induced by DOX.
Real-time PCR (RT-PCR)
Total RNA was extracted from tumor tissues by Direct-zolTM RNA Miniprep extraction kit (Zymoresearch Co., Irvine, CA, USA), following the instructions of the manufacturer. The isolated RNA concentration and purity were estimated spectrophotometrically by Nanodrop System (Thermo Fisher Scientific Inc., MA, USA, 2000/2000c) at 260 nm and 260/280 nm ratio, respectively. One microgram of the total RNA was converted into c-DNA by reverse transcription using SensiFASTTM cDNA Synthesis Kit (Bioline, Massachusetts, USA, Catalog no. BIO-65,053), following manufacturer’s protocols. RT-PCRs were done by SensiFAST™ SYBR® No-ROX Kit (Bioline, Massachusetts, USA, Catalog no. BIO-98,002) in Piko Real-PCR System (Thermo Fisher Scientific Inc.), according to the instructions of the manufacturer. The primers were designed as shown in by using premier Biosoft (USA) and Pubmed (Entrez Gene) for getting the specific gene sequences. The mRNA expression levels of TRAILR2, TRAIL, Fas, FasL, caspase-3 and Bcl-2 genes were normalized to the housekeeping gene Beta-actin (β-actin). mRNA expression levels were quantified using the 2−ΔΔCt method [Citation25].
Table 1. Gene-specific primer sequence.
Statistical analysis
For data analysis, Graph Pad Prism 6.01 (Graph Pad Software, SanDiego, CA, USA) was used. One-way analysis of variance followed by Tukey’s post-hoc test was used for the analysis of quantitative variables. Statistical significance was predefined at a P-value of less than 0.05. Results were presented as the mean ± standard error of the mean (mean±SE). The Kaplan–Meier method was used to demonstrate the survival of mice.
Results
Effect of CGA on solid tumor growth inhibition
Last tumor volume was measured on the 21st day of treatment, and SEC group showed an increase from 74.3 ± 3.9 mm3 to 1070.6 ± 56.7 mm3. Administration of CGA, DOX and their combination caused a marked reduction in tumor volume by 58.9% (P < 0.0001), 57.2% (P < 0.0001) and 85.8% (P < 0.0001), respectively, in comparison with SEC group. Furthermore, CGA/DOX combination exhibited a significant reduction in tumor volume as compared to both DOX group (P < 0.0001) and CGA group (P < 0.0001), respectively ()), F (4, 168) = 262.7,P < 0.0001.
Figure 1. Effect of chlorogenic acid (CGA) treatment alone and in combination with doxorubicin (DOX) on (a) tumor volume (n = 11 except SEC group n = 13), (b) tumor weight (n = 8 except SEC group n = 10) and (c) survival (Kaplan–Meier survival curve) (n = 3). SEC, solid Ehrlich carcinoma. Values are expressed as mean ± SEM. **** Significant difference as compared to the SEC group at P < 0.0001 and #### significant difference as compared to the DOX group at P < 0.0001.
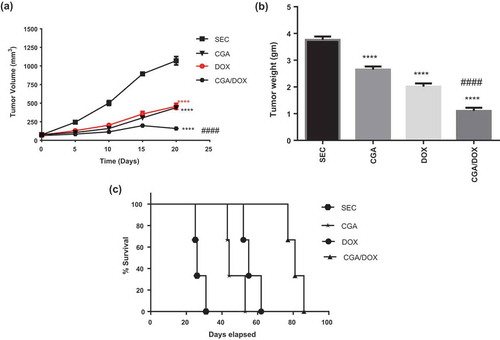
As shown in ), administration of CGA, DOX and CGA/DOX combination caused a remarkable reduction in tumor weight by 29.5% (P < 0.0001), 46.5% (P < 0.0001) and 70.7% (P < 0.0001), respectively, in comparison with SEC group. Moreover, the combination therapy markedly reduced tumor weight by 1.9-fold (P < 0.001) as compared to DOX-treated group, F (3, 30) = 88.16, P < 0.0001.
Effect of CGA on the survival of tumor-bearing mice
Both CGA and DOX treatments showed a marked increase in the mean survival time by 1.7-fold (P < 0.01) and 2.1-fold (P < 0.001) in comparison with the SEC group, respectively. Moreover, the combination therapy exhibited a marked increase in the survival of mice by 2.9-fold (P < 0.0001) and 1.4-fold (P < 0.001) as compared to SEC and DOX groups, respectively (; )), as obtained by Kaplan–Meirer survival analysis.
Table 2. Effect of chlorogenic acid (CGA) treatment alone and in combination with doxorubicin (DOX) on the survival of tumor-bearing mice (n = 3).
Effect of CGA on histopathological changes
As shown in and ), cross-sectional images of tumors revealed high mitotic figures and little apoptotic bodies in the SEC group. While treatment with CGA, DOX and their combination showed a significant reduction in mitotic activity and an elevation in apoptosis as compared to the SEC group, F (3, 36) = 46.17, P < 0.0001.
Table 3. Effect of chlorogenic acid (CGA) treatment alone and in combination with doxorubicin (DOX) on the histopathological changes and immunohistochemical staining of tumor tissues for apoptotic marker (active caspase-3) in mice bearing solid Ehrlich carcinoma (SEC) (n = 3).
Figure 2. Effect of chlorogenic acid (CGA) treatment alone and in combination with doxorubicin (DOX) on (a) histopathological analysis of tumor sections stained with H&E, arrows indicate mitotic figures and asterisks indicate apoptotic cell and (b) immunohistochemical staining of caspase-3 in tumor sections. Caspase-3-positive areas appeared as brown granules. A: SEC group, B: CGA group, C: DOX group, D: CGA/DOX group. n = 3.
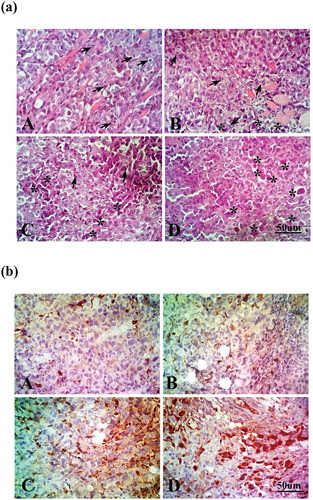
Effect of CGA on the tissue expression of active caspase-3
Using immunohistochemical analysis and measuring apoptotic index, CGA and/or DOX treatments were able to markedly increase the expression of active caspase-3 in comparison with the SEC group (P < 0.0001). Furthermore, the combination therapy of CGA and DOX revealed a marked increase in the tissue expression of active caspase-3, compared to the DOX-treated group (P < 0.001) (; )), F (3, 36) = 128.0, P < 0.0001.
Effect of CGA on caspase-3 mRNA expression levels
Administration of CGA, DOX and their combination markedly increased caspase-3 gene expression, compared to SEC group. Furthermore, CGA/DOX combination increased caspase-3 gene expression significantly by 42.3% (P < 0.0001) in comparison with the DOX group ()), F (3, 30) = 217.6 P < 0.0001.
Figure 3. Effect of chlorogenic acid (CGA) treatment alone and in combination with doxorubicin (DOX) on gene expression of (a) caspase-3 and (b) Bcl-2. Values are expressed as mean ± SEM. n = 8 except SEC group, n = 10. **** Significant difference as compared to the SEC group at P < 0.0001 and #### significant difference as compared to the DOX group at P < 0.0001.
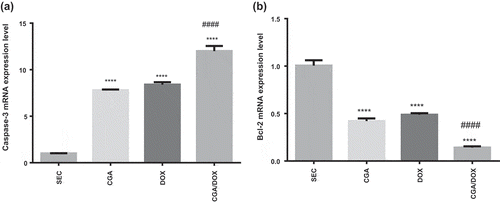
Effect of CGA on antiapoptotic Bcl-2 mRNA expression levels
CGA and/or DOX treatments significantly downregulated Bcl-2 gene expression, compared to the SEC group. Further, CGA/DOX combination caused a marked reduction in the gene expression of Bcl-2 in comparison with the DOX group ()), F (3, 30) = 116.5, P < 0.0001.
Effect of CGA on TRAILR2, TRAIL, Fas and Fasl mRNA expression levels
Groups treated with CGA, DOX and their combination exhibited a marked upregulation in TRAILR2, TRAIL, Fas and FasL mRNA expression levels as compared to SEC group (P < 0.0001). Moreover, the combination of CGA and DOX significantly increased the gene expression of TRAILR2, TRAIL, Fas and FasL as compared to DOX-treated group (P < 0.0001) (). TRAILR2 (F (3, 30) = 205.2, P < 0.0001), TRAIL (F (3, 30) = 96.45, P < 0.0001), Fas (F (3, 30) = 261.3,P < 0.0001) and FasL (F (3, 30) = 222.8, P < 0.0001).
Figure 4. Effect of chlorogenic acid (CGA) treatment alone and in combination with doxorubicin (DOX) on gene expression of (a) TRAILR2, (b) TRAIL, (c) Fas and (d) FasL. Values are expressed as mean ± SEM. n = 8 except SEC group, n = 10. **** Significant difference as compared to the SEC group at P < 0.0001 and #### significant difference as compared to the DOX group at P < 0.0001.
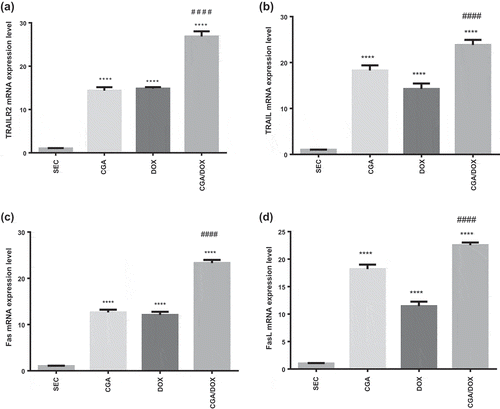
Effect of CGA on serum CRP level
As shown in ), the SEC group revealed a significant increase in serum CRP level by 10.1-fold (P < 0.0001), compared to the normal control group. CGA group showed a significant reduction in CRP level in comparison with the SEC group (P < 0.0001). Furthermore, CGA/DOX combination therapy exerted a significant decrease in serum CRP level by 4.3-fold (P < 0.0001) and 1.5-fold (P < 0.05) in comparison with SEC and DOX groups, respectively, F (4, 37) = 187.3, P < 0.0001.
Figure 5. Effect of chlorogenic acid (CGA) treatment alone and in combination with doxorubicin (DOX) on (a) serum CRP, (b) tissue MDA and (c) tissue GSH levels. Values are expressed as mean ± SEM. n = 8 except SEC group, n = 10. ++++ Significant difference as compared to the normal control group at P < 0.0001, **** significant difference as compared to the SEC group at P < 0.0001, #### significant difference as compared to DOX group at P < 0.0001 and # significant difference as compared to DOX group at P < 0.05.
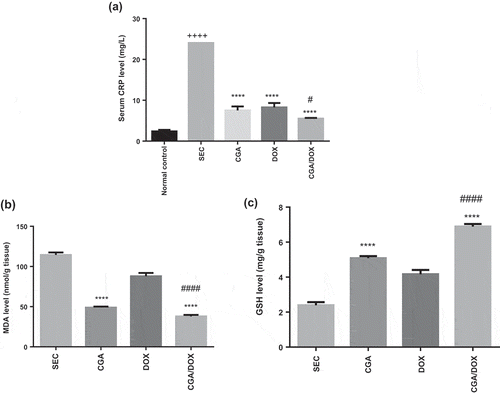
Effect of CGA on oxidative stress level
As shown in ), treatment with CGA resulted in a significant reduction in tissue MDA level, compared to the SEC group. Moreover, CGA/DOX combination markedly reduced tissue MDA level, compared to SEC and DOX groups, F (3, 30) = 161.1,P < 0.0001.
Treatment with CGA significantly increased tissue GSH level as compared to the SEC group. Moreover, the group treated with a combination of CGA and DOX revealed a remarkable elevation in tissue GSH level, compared to SEC and DOX groups ()), F (3, 30) = 113.1, P < 0.0001.
Effect of CGA on cardiotoxicity induced by DOX
As shown in ,), treatment with DOX resulted in a significant increase in serum levels of CK-MB and LDH in comparison with the normal control group. Moreover, there was a nonsignificant change between the CGA group and the normal control group regarding both CK-MB and LDH enzymes activity. Furthermore, CGA/DOX combination group exhibited a marked reduction in serum levels of CK-MB and LDH by 1.56-fold (P < 0.0001) and 1.36-fold (P < 0.001), respectively, in comparison with DOX-treated group. CK-MB (F (3, 28) = 80.75, P < 0.0001) and LDH (F (3, 28) = 25.24,P < 0.0001).
Figure 6. Effect of chlorogenic acid (CGA) treatment alone and in combination with doxorubicin (DOX) on (a) serum CK-MB and (b) LDH levels. (c) Histopathological analysis of heart sections stained with H&E and arrows indicates cardiac myocyte vacuolization and infiltration by mixed chronic inflammatory cells. A: normal control group, B: CGA group, C: DOX group, D: CGA/DOX group. Values are expressed as mean ± SEM. n = 8. +++ Significant difference as compared to the normal control group at P < 0.001, ++++ significant difference as compared to the normal control group at P < 0.0001, ### significant difference as compared to DOX group at P < 0.001 and #### significant difference as compared to DOX group at P < 0.0001.
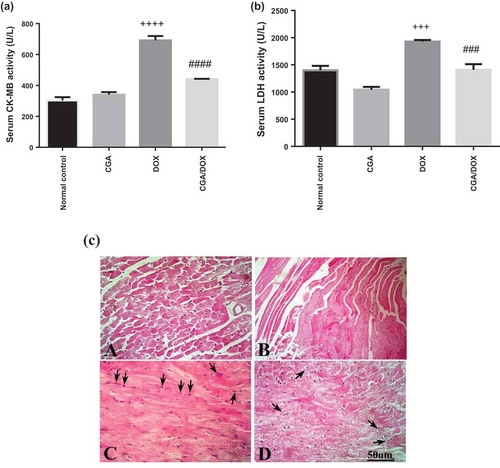
Histopathological examination of heart tissues showed the normal architecture of myocardium in the normal control group and CGA-treated group, while treatment with DOX showed severe vacuolization of cardiac myocytes and chronic inflammatory cells. Meanwhile, CGA treatment in combination with DOX decreased the vacuolization and inflammation of cardiac myocytes and showed a well-protected myocardium ()).
Discussion
Natural products are an attractive source to identify new compounds for the prevention and treatment of cancer [Citation26]. Previous studies presented that CGA exerts different biological activities such as antitumor activity and chemosensitizing activity [Citation10,Citation27–Citation29]. Therefore, we studied the molecular mechanism of CGA as an effective chemosensitizer that enhances DOX-induced apoptosis in the SEC model in mice.
According to our results, oral administration of CGA significantly decreased tumor volume and weight as compared to the SEC group. This effect was near to the effect of DOX treatment. Interestingly, coadministration of CGA with DOX revealed a more significant reduction in tumor burden, compared to the DOX group. Furthermore, these results were approved by the capability of CGA alone and in combination with DOX to markedly increase %ILS and MST in comparison with the SEC group. Our results were further confirmed by histopathological investigation of tumor mass that revealed decreased mitotic figures and increased apoptotic bodies with CGA either alone or in combination with DOX.
Induction of apoptosis in different types of cancer is one of the possible mechanisms by which CGA exerts its antitumor effect [Citation30,Citation31]. According to our results, CGA either alone or in combination with DOX caused the upregulation of TRAILR2 and Fas expressions. In addition, it increased the gene expression of their corresponding ligands TRAIL and FasL. In agreement with the present work, Rakshit, et al. (2010) reported the ability of CGA to increase TRAILR2 expression in vitro [Citation32]. To our knowledge, our study is the first to investigate the ability of CGA to upregulate TRAILR2, Fas, TRAIL and FasL in SEC in vivo model. Death ligands such as TRAIL and FasL have the ability to induce apoptosis after binding to their corresponding death receptors TRAILR2 and Fas, respectively. Activation of TRAILR2 and Fas receptors leads to the recruitment of Fas-associated protein with death domain and further processing of caspases and splitting of procaspase 3 to the active caspase-3 that further induces apoptosis [Citation33–Citation36]. Our study revealed an increase in caspases-3 gene expression in the CGA group. Furthermore, CGA/DOX combination therapy significantly increased the gene expression level of caspase-3 in comparison with each treatment alone. Moreover, the immunohistochemical study of active caspase-3 in tumor tissue revealed that CGA alone and in a combination with DOX significantly increased active caspase-3 tissue expression.
The Bcl-2 gene family which includes anti-apoptotic Bcl-2 is significant in the regulation of cell apoptosis [Citation37]. Here, we found that Bcl-2 gene expression in tumor tissue was reduced by CGA. In addition, the combination of CGA and DOX significantly decreased the Bcl-2 gene expression level in comparison with each treatment alone. Thus, the reduction in gene expression of Bcl-2 leads to disruption of mitochondrial outer membrane permeability and the release of cytochrome-c into the cytoplasm. Cytochrome-c triggers the formation of apoptosome by binding to apoptosis protease activating factor-1 (Apaf-1) and pro-caspase-9, allowing the release of caspase-9. This process in turn activates downstream executor caspases-3, caspase-6 and caspase-7 [Citation38,Citation39].
CRP is a systemic inflammation biomarker produced in the liver and released into the circulation in response to elevations in interleukin-6 [Citation40]. Elevated levels of CRP are associated with tissue damage, inflammation during cancer, cardiac stress and infection [Citation41–Citation43]. Our study showed that induction of solid tumors in female Swiss albino mice was accompanied by a significant increase in CRP level that was in agreement with other previous studies [Citation19,Citation44,Citation45]. Interestingly, the anti-inflammatory effect of CGA was indicated by a significant reduction in CRP level in comparison with the SEC group. Also, CGA/DOX combination markedly reduced the CRP level.
Oxidative stress and ROS have been involved in the pathogenesis of cancer [Citation46]. Regarding our results, tumor cells were under oxidative stress indicated by an elevation in the MDA level in the SEC group which is a biomarker of lipid peroxidation [Citation47]. Furthermore, GSH decreased in the SEC group reflected the failure of the antioxidant defense mechanism. Although DOX treatment was effective in reducing tumor burden, it resulted in a marked increase in MDA and a significant decrease in GSH levels in comparison with the normal control group and that was in accordance with Qin et al. (2008) [Citation48]. Interestingly, administration of CGA alone and in combination with DOX significantly decreased MDA and increased GSH levels leading to a decreased level of oxidative stress and ameliorated the antioxidant capacity in treated mice, respectively. This antioxidant ability of CGA was reported in other previous studies [Citation49,Citation50].
DOX is an effective chemotherapeutic agent that is widely used in different types of cancers [Citation51–Citation54], but its clinical use is often limited by induction of cardiotoxicity [Citation55]. In this study, mice treated with DOX showed a marked elevation in serum CK-MB, LDH activities in comparison with a normal control group and supported by a histopathological report, which showed severe cardiac myocyte vacuolization and infiltration by mixed chronic inflammatory cells that proved cardiotoxicity induced by DOX. Interestingly, a combination of CGA with DOX significantly reduced the activity of both serum CK-MB and serum LDH to a level near normal and showed a well-protected myocardium. This study demonstrates for the first time the cardioprotective effect of CGA against DOX in SEC in vivo model. The combination therapy caused a marked reduction in oxidative stress and inflammation indicated by low MDA, high GSH and low CRP levels as compared to the DOX group. Thus, the antioxidant and anti-inflammatory activities of CGA are considered as the possible mechanisms by which it protects against DOX-induced cardiotoxicity. Nevertheless, further studies are recommended to determine the exact mechanism behind CGA’s cardioprotective effect.
In conclusion, CGA exhibits antitumor activity by that it suppresses the growth of SEC in vivo model. As summarized in , the antitumor effect of CGA may be due to the induction of apoptosis by upregulating the expression of death receptors (TRAILR2 and Fas), their legends (TRAIL, FasL) and downregulating the expression of antiapoptotic protein Bcl-2. Moreover, CGA combination with DOX was able not only to enhance apoptosis induced by DOX, but it also reduces oxidative stress level and attenuates DOX-induced cardiotoxicity.
Disclosure statement
No potential conflict of interest was reported by the authors.
References
- Siegel RL, Miller KD, Jemal A. Cancer statistics, 2019. CA. 2019.
- Perez-Herrero E, Fernandez-Medarde A. Advanced targeted therapies in cancer: drug nanocarriers, the future of chemotherapy. Eur J Pharm Biopharm. 2015;93:52–79.
- Pan ST, Li ZL, He ZX, et al. Molecular mechanisms for tumour resistance to chemotherapy. Clin Exp Pharmacol Physiol. 2016;43(8):723–737.
- Cortes-Funes H, Coronado C. Role of anthracyclines in the era of targeted therapy. Cardiovasc Toxicol. 2007;7(2):56–60.
- Momparler RL, Karon M, Siegel SE, et al. Effect of adriamycin on DNA, RNA, and protein synthesis in cell-free systems and intact cells. Cancer Res. 1976;36(8):2891–2895.
- Wang S, KOTAMRAJU S, KONOREV E, et al. Activation of nuclear factor-kappaB during doxorubicin-induced apoptosis in endothelial cells and myocytes is pro-apoptotic: the role of hydrogen peroxide. Biochem J. 2002;367(Pt 3):729–740.
- Chatterjee K, Zhang J, Honbo N, et al. Doxorubicin cardiomyopathy. Cardiology. 2010;115(2):155–162.
- Bekedam EK, Schols HA, Van Boekel MA, et al. Incorporation of chlorogenic acids in coffee brew melanoidins. J Agric Food Chem. 2008;56(6):2055–2063.
- Heitman E, Ingram DK. Cognitive and neuroprotective effects of chlorogenic acid. Nutr Neurosci. 2017;20(1):32–39.
- Hongtao L, Xiaoqi G, Junni L, et al. Chlorogenic-induced inhibition of non-small cancer cells occurs through regulation of histone deacetylase 6. Cell Mol Biol (Noisy-le-grand). 2018;64(10):134–139.
- Santana-Galvez J, Cisneros-Zevallos L, Jacobo-Velazquez DA. Chlorogenic acid: recent advances on its dual role as a food additive and a nutraceutical against metabolic syndrome. Molecules. 2017;22(3):358.
- Liu CC, Zhang Y, Dai B-L, et al. Chlorogenic acid prevents inflammatory responses in IL1betastimulated human SW1353 chondrocytes, a model for osteoarthritis. Mol Med Rep. 2017;16(2):1369–1375.
- Akila P, Vennila L. Chlorogenic acid a dietary polyphenol attenuates isoproterenol induced myocardial oxidative stress in rat myocardium: an in vivo study. Biomed Pharmacother. 2016;84:208–214.
- Lukitasari M, Nugroho DA, Widodo N. Chlorogenic acid: the conceivable chemosensitizer leading to cancer growth suppression. J Evid Based Integr Med. 2018;23:2515690x18789628.
- Leonard BC, Johnson DE. Signaling by cell surface death receptors: alterations in head and neck cancer. Adv Biol Regul. 2018;67:170–178.
- Vanden Berghe T, Kaiser WJ, Bertrand MJ, et al. Molecular crosstalk between apoptosis, necroptosis, and survival signaling. Mol Cell Oncol. 2015;2(4):e975093.
- Reed JC. Mechanisms of apoptosis. Am J Pathol. 2000;157(5):1415–1430.
- Verma S, Singh A, Kumari A, et al. Natural polyphenolic inhibitors against the antiapoptotic BCL-2. J Recept Signal Transduct Res. 2017;37(4):391–400.
- El-Mesery M, Al-Gayyar MM, Salem HA, et al. Chemopreventive and renal protective effects for docosahexaenoic acid (DHA): implications of CRP and lipid peroxides. Cell Div. 2009;4:6.
- Devi PU, Rao BS, Solomon FE. Effect of plumbagin on the radiation induced cytogenetic and cell cycle changes in mouse Ehrlich ascites carcinoma in vivo. Indian J Exp Biol. 1998;36(9):891–895.
- Sathisha MP, Revankar VK, Pai KS. Synthesis, structure, electrochemistry, and spectral characterization of bis-isatin thiocarbohydrazone metal complexes and their antitumor activity against ehrlich ascites carcinoma in swiss albino mice. Met Based Drugs. 2008;2008:362105.
- Schirner M, Hoffmann J, Menrad A, et al. Antiangiogenic chemotherapeutic agents: characterization in comparison to their tumor growth inhibition in human renal cell carcinoma models. Clin Cancer Res. 1998;4(5):1331–1336.
- Chen L, Li Y, Yin W, et al. Combination of chlorogenic acid and salvianolic acid B protects against polychlorinated biphenyls-induced oxidative stress through Nrf2. Environ Toxicol Pharmacol. 2016;46:255–263.
- Awara WM, El-Sisi AE, El-Sayad ME, et al. The potential role of cyclooxygenase-2 inhibitors in the treatment of experimentally-induced mammary tumour: does celecoxib enhance the anti-tumour activity of doxorubicin? Pharmacol Res. 2004;50(5):487–498.
- Livak KJ, Schmittgen TD. Analysis of relative gene expression data using real-time quantitative PCR and the 2(-Delta Delta C(T)) method. Methods. 2001;25(4):402–408.
- Bishayee A, Sethi G. Bioactive natural products in cancer prevention and therapy: progress and promise. Semin Cancer Biol. 2016;40–41:1–3.
- Kang TY, Yang HR, Zhang J, et al. The studies of chlorogenic acid antitumor mechanism by gene chip detection: the immune pathway gene expression. J Anal Methods Chem. 2013;2013:617243.
- Yan Y, Liu N, Hou N, et al. Chlorogenic acid inhibits hepatocellular carcinoma in vitro and in vivo. J Nutr Biochem. 2017;46:68–73.
- Yan Y, Li J, Han J, et al. Chlorogenic acid enhances the effects of 5-fluorouracil in human hepatocellular carcinoma cells through the inhibition of extracellular signal-regulated kinases. Anticancer Drugs. 2015;26(5):540–546.
- Liu YJ, ZHOU C-Y, QIU C-H, et al. Chlorogenic acid induced apoptosis and inhibition of proliferation in human acute promyelocytic leukemia HL60 cells. Mol Med Rep. 2013;8(4):1106–1110.
- Yamagata K, Izawa Y, Onodera D, et al. Chlorogenic acid regulates apoptosis and stem cell marker-related gene expression in A549 human lung cancer cells. Mol Cell Biochem. 2018;441(1–2):9–19.
- Rakshit S, Mandal L, Pal BC, et al. Involvement of ROS in chlorogenic acid-induced apoptosis of Bcr-Abl+ CML cells. Biochem Pharmacol. 2010;80(11):1662–1675.
- Mahmood Z, Shukla Y. Death receptors: targets for cancer therapy. Exp Cell Res. 2010;316(6):887–899.
- Lavrik I, Golks A, Krammer PH. Death receptor signaling. J Cell Sci. 2005;118(Pt 2):265–267.
- Elmore S. Apoptosis: a review of programmed cell death. Toxicol Pathol. 2007;35(4):495–516.
- El-Mesery M, Trebing J, Schafer V, et al. CD40-directed scFv-TRAIL fusion proteins induce CD40-restricted tumor cell death and activate dendritic cells. Cell Death Dis. 2013;4:e916.
- Reed JC, Miyashita T, Takayama S, et al. BCL-2 family proteins: regulators of cell death involved in the pathogenesis of cancer and resistance to therapy. J Cell Biochem. 1996;60(1):23–32.
- Danial NN, Korsmeyer SJ. Cell death: critical control points. Cell. 2004;116(2):205–219.
- Saelens X, Festjens N, Walle LV, et al. Toxic proteins released from mitochondria in cell death. Oncogene. 2004;23(16):2861–2874.
- Allin KH, Nordestgaard BG, Flyger H, et al. Elevated pre-treatment levels of plasma C-reactive protein are associated with poor prognosis after breast cancer: a cohort study. Breast Cancer Res. 2011;13(3):R55.
- Gabay C, Kushner I. Acute-phase proteins and other systemic responses to inflammation. N Engl J Med. 1999;340(6):448–454.
- Chandrashekara S. C-reactive protein: an inflammatory marker with specific role in physiology, pathology, and diagnosis. Internet J Rheumatol Clin Immunol. 2014;2(S1):SR3.
- Ansar W, Ghosh S. C-reactive protein and the biology of disease. Immunol Res. 2013;56(1):131–142.
- El-Mowafy AM, El-Mesery ME, Salem HA, et al. Prominent chemopreventive and chemoenhancing effects for resveratrol: unraveling molecular targets and the role of C-reactive protein. Chemotherapy. 2010;56(1):60–65.
- Said UZ, Neamat HA, Amina MM, et al. Effects of omega-3 fatty acids against Ehrlich carcinoma-induced hepatic dysfunction. J Cancer Res Exp Oncol. 2014;6(2):20–28.
- Waris G, Ahsan H. Reactive oxygen species: role in the development of cancer and various chronic conditions. J Carcinog. 2006;5:14.
- Tsikas D. Assessment of lipid peroxidation by measuring malondialdehyde (MDA) and relatives in biological samples: analytical and biological challenges. Anal Biochem. 2017;524:13–30.
- Qin XJ, He W, Hai C-X, et al. Protection of multiple antioxidants Chinese herbal medicine on the oxidative stress induced by adriamycin chemotherapy. J Appl Toxicol. 2008;28(3):271–282.
- Ali N, Rashid S, Nafees S, et al. Protective effect of Chlorogenic acid against methotrexate induced oxidative stress, inflammation and apoptosis in rat liver: an experimental approach. Chem Biol Interact. 2017;272:80–91.
- Sato Y, Itagaki S, Kurokawa T, et al. In vitro and in vivo antioxidant properties of chlorogenic acid and caffeic acid. Int J Pharm. 2011;403(1–2):136–138.
- Minotti G, Menna P, Salvatorelli E, et al. Anthracyclines: molecular advances and pharmacologic developments in antitumor activity and cardiotoxicity. Pharmacol Rev. 2004;56(2):185–229.
- Petrioli R, Fiaschi AI, Francini E, et al. The role of doxorubicin and epirubicin in the treatment of patients with metastatic hormone-refractory prostate cancer. Cancer Treat Rev. 2008;34(8):710–718.
- Tacar O, Sriamornsak P, Dass CR. Doxorubicin: an update on anticancer molecular action, toxicity and novel drug delivery systems. J Pharm Pharmacol. 2013;65(2):157–170.
- Carvalho C, Santos R, Cardoso S, et al. Doxorubicin: the good, the bad and the ugly effect. Curr Med Chem. 2009;16(25):3267–3285.
- Takemura G, Fujiwara H. Doxorubicin-induced cardiomyopathy from the cardiotoxic mechanisms to management. Prog Cardiovasc Dis. 2007;49(5):330–352.