ABSTRACT
Diabetes mellitus is the most common metabolic disease. Ceramide pathway is a vital intracellular signal transmission factor, and it has been regarded as a mediator to the development of diabetes. In the present study, the effects of OL-E and OL-L alkaloids in Portulaca oleracea are examined concerning ceramide generation and ceramide metabolizing enzymes.
The beta-TC-6 pancreatic cell line was treated with various concentrations of OL-E and OL-L. cell viability, insulin secretion, glucose reuptake, Pentosidine level, total cellular ceramide and the activities of acid ceramidase (AC), neutral sphingomyelinase (NS) and glucosylceramide synthase (GCS) assayed with spectrophotometry and fluorescence evaluation.
OL-E was more potent than OL-L, with the highest insulin secretion of 100 μM. Increased cytotoxicity and glycation index appeared at concentrations of 200 μM and higher. Higher concentrations of studied compounds were associated with ceramide accumulation, NS activation, and AC and GCS suppression .
These findings indicated that the consentration greater than 100 µM might be involved in improving glucose homeostasis. Based on insulin secretion and glucose uptake, high concentrations of investigated compounds are correlated with ceramide generation, and this event could attenuate the Oleracein hypoglycemic effect.
Introduction
Diabetes mellitus (DM) is a widespread and increasing metabolic disorder distinguished by the lack of the body’s ability to regulate glucose and insulin homeostasis [Citation1]. The long-term hyperglycemia of DM is associated with severe public health problems, multiple dysfunctions, and failure of various organs, especially the urinary, nervous, and cardiovascular systems [Citation2]. Thus, investigating the cellular and molecular DM-related pathways and developing new antidiabetic medicines are substantial.
The insulin secretion of the pancreas beta cells plays a key role in all types of DM, especially type one [Citation3]. Because insulin ineffectiveness causes hyperglycemia, glucose uptake can be an accurate and rational index for insulin action [Citation4]. Furthermore, advanced glycosylation end products (AGEs) such as Pentosidine may play a considerable function in the progress of diabetic microvascular complications. Hence, the Pentosidine level is a reliable biomarker for tissue levels of glycosylation [Citation5,Citation6].
Many efforts have been made to comprehend this disorder and its underlying molecular mechanism. Ceramide generation as a cellular second messenger affects blood sugar regulation and insulin signal transduction [Citation7,Citation8]. Ceramide-induced insulin resistance and β-cell apoptosis occur due to multiple mechanisms, including increasing cytochrome c release and inhibition of Akt (protein kinase B) [Citation9].
Ceramide content elevation is associated with the induction of insulin resistance and may be toxic in a variety of different cell types [Citation7,Citation10]. So, determining the effects of antidiabetic treatment on the ceramide pathway is essential. The biosynthesis and biodegradation of ceramide arise from the function of different enzymatic systems, and they can be affected by several endogenous and exogenous agents. Acid ceramidase (AC) and glucosylceramide synthase (GCS) (as major ceramide degradation factors), and neutral sphingomyelinase (NS) (as a ceramide generator) are the critical enzymes in ceramide metabolism [Citation11].
The crude extracts of herbal medicine are traditionally used in the treatment of DM, and usually, they have fewer harmful properties than common drugs. Furthermore, many current drugs are modeled from the plants [Citation12]. Portulaca oleracea (is commonly known as Ma-Chi-Xian in Chinese or Purslane in English) is a relatively well-known potherb that has been mentioned in the World Health Organization (WHO) [Citation13,Citation14]. It contains different phytochemicals substances, including glycosides, flavonoids, terpenoids, and alkaloids (including Oleraceins) [Citation15–17].
Purslane, as a tropical plant, is a source of isoquinoline alkaloids, which has exhibited several medical benefits, including anti–bacterial, anti–tumor, and antidiabetic effects with undefined targets [Citation18–20].
Oleracein E (OL-E) and Oleracein L (OL-L) are the main bioactive components in Purslane, and evaluating their antidiabetic effect regarding ceramide generation, insulin secretion, and response to insulin at different concentrations on pancreatic beta cells are necessary [Citation21]. In the current study, the effects of OL-E and OL-L have been studied using biological assessment in the beta-TC-6 pancreatic cell line. Therefore, in the present study, cell viability, glucose uptake, insulin secretion levels, total cellular ceramide, and the activity of major ceramide metabolizing enzymes (AC, NS, and GCS) were measured after the treatment with different concentrations of OL-E and OL-L.
Chemical structures of the examined isoquinoline alkaloids are presented in [Citation22].
Material and methods
Preparation of beta-TC-6 pancreatic cell line
Mouse β-TC6 pancreatic cell line was purchased from the Pasteur Institute of Iran (IPI). The pancreatic cells were grown in Dulbecco’s Modified Eagle Medium (DMEM), and 10% fetal bovine serum (FBS), 1% penicillin and streptomycin antibiotics were used to prevent bacterial contamination of cell culture. The cultures were maintained in a moist incubator at 37 °C in 5% CO2, and the culture medium was renewed every three days once [Citation1]. The other used compounds, OL-E, and OL-L were bought from Sigma Chemical Co., St. Louis, MO, USA.
Glucose uptake assay
Pancreatic cells were incubated at a seeding density of 1.5 × 104 viable cells/ml in a 96-well plate at 37° C in 5% CO2. After overnight incubation, the medium was removed, washed with phosphate-buffered saline (PBS) twice, and substituted with 2.5 mM glucose and L-glutamine in 15% (v/v) PBS. The cells were incubated again for 60 min, and then, the medium was replaced with 2-NBD glucose (10 mM). Finally, the medium was discarded, and the cells were washed with PBS and Hoechst 33,342 nucleic dye for 30 min. ArrayScan High Content Screening (HCS) system (Cellomics Inc., Pittsburgh, PA, USA) and Target Activation BioApplication software (Cellomics Inc.) were used for the evaluation of the plates [Citation1].
Cell viability assay
The cell viability was determined using the 3-(4,5-dimethylthiazol-2-yl)-2,5-diphenyl tetrazolium bromide (MTT) colorimetric assay to measure cell toxicity. The assay was based on the production of a blue formazan by the dehydrogenase enzymes, which found in cells were alive. Briefly, after incubation of the β-TC6 pancreatic cells over-night at 37°C in 5% CO2 with different doses of OL-E and OL-L, cells were washed and placed in 96 well plates. MTT solution was added to all wells of an assay, and plates were incubated again for three hours. Then, the culture medium was removed, and dimethyl sulfoxide was added to dissolve formazan. Experiments were repeated three times. Finally, the optical density was obtained using a spectrophotometer at 540 and 630 nm. The results are presented as survival percentages compared to viable cells [Citation1].
Insulin secretion assay
The medium of β-TC6 pancreatic cell lines contained 11.1 mM glucose and was changed every other day. For insulin secretion assay, the cells were washed and placed in Krebs-Ringers bicarbonate (KRB) buffer for 30 min. KRB contains 115 mM NaCl, 4.7 mM KCl, 2.56 mM CaCl2, 1.2 mM KH2PO4, 1.2 mM MgSO4, 20 mM NaHCO3, 16 mM 4-(2-hydroxyethyl)-1-piperazineethanesulfonic acid (HEPES), and 0.3% of serum albumin. The cells were then incubated with KRB, which included 11.1 mM glucose for 1 hour at 37 °C. Afterward, the contents were centrifuged, and the supernatants were equally isolated and reserved at −20 °C. To evaluate the rate of insulin secretion, the serologic mouse insulin enzyme-linked immunosorbent assay kit was used [Citation1].
Pentosidine level
After placing the samples (the pancreatic cells treated with Oleracein derivatives) in the phosphate buffer, the cells were separated, and the remaining solution was gently condensed by solvent evaporation. The remaining solution was hydrolyzed with hydrochloric acid (HCl), and then the HCl was also neutralized with 1 N NaOH solution. After the filtration, the solution was injected into a reverse phase C18 column chromatography in the HPLC system, and according to the fluorescence absorbance of standard pentosidine at 335/385 nm (excitation/emission wavelengths), the concentration of pentosidine was determined in the unknown sample [Citation23].
Total cellular ceramide
In order to measure the total ceramide level in a cellular extract, a recombinant ceramidase enzyme was used according to the method proposed by Xingxuan H et al., which made the ceramide in the sample completely hydrolyzed and converted to sphingosine. A particular substance called Naphthalene-2,3-dialdehyde (NDA) with fluorescence properties and the ability to connect specifically to the sphingosine was added to the sample. Then, sphingosine-NDA was separated by HPLC using BetaBasic-18 columns. All chromatographic proceedings were done at room temperature using a mobile phase of methanol. The fluorescent of sphingosine-NDA was evaluated at the excitation/emission wavelengths of 252 and 483 nm [Citation24].
Acid ceramidase (AC) activity assays
After 24 h incubation of pancreatic cells with Oleracein derivatives, to evaluate the enzyme activity of AC, 7-nitro-2-1,3-benzoxadiazol-4-yl- ceramide (NBD-ceramide) in sodium acetate buffer and Triton X-100 were added, and the reaction was stopped by methanol-chloroform. The fluorogenic substrate was NBD-ceramide. Then centrifugation was performed, and the supernatant was gathered. The supernatant was regarded as an uncleaved substrate, and the fluorescence absorbance was determined in the UV range 400 excitation/550 nm emission in a spectrophotometer. Simultaneously, another test tube was made as described above and was added by the cellular extract suspension. Finally, the fluorescence yields were evaluated in the UV range of 430 excitation/550 nm emission. The absorption ratio of cleavaged/uncleaved fluorogenic substrate was demonstrated as an AC activity index [Citation25].
Neutral sphingomyelinase (NS) activity assays
The activity of NS starts by adding sphingomyelin to the cellular extracts, and sphingomyelin is transformed into ceramide and phosphorylcholine. Then, alkaline phosphatase produces choline from the phosphorylcholine, and afterward, the newly generated choline is used to obtain hydrogen peroxide. This reaction is catalyzed by choline oxidase. In the final stage, in the presence of peroxidase, which acts as a catalyst, hydrogen peroxide reacts with sodium N-ethyl-N-(2-hydroxy-3-sulfopropyl)-3,5-dimethoxyaniline (DAOS) and 4-aminoantipyrine to produce a blue color with a suitable absorption at 595 nm [Citation26].
Glucosylceramide synthase (GCS) activity assays
To the consideration of GCS activity, the C6-4-nitrobenzo-2-oxa-1,3-diazole (NBD)-ceramide as a fluorescent substrate and a normal phase HPLC were applied. Acceptor substrate, C6-NBD-Ceramide, and lecithin were mixed in 100 μL of ethanol, and then the solvent was evaporated. After adding water, the obtained mixture was sonicated to form liposomes. For the GCS assay, the reaction mixture contains UDP-Glc, EDTA, C6-NBD- ceramide liposome, and the appropriate amount of enzyme in the lysis buffer. Assays were carried out for one hour at 37°C. After stopping the reaction by adding chloroform/methanol, fluorescence was determined at excitation and emission wavelengths of 470 and 530 nm, respectively. The fluorescent peaks were determined by comparing their retention times with standards [Citation27].
Statistical analysis
The experiments were performed separately in 5 groups (triplicate) and in the range of 0–400 μM of both studied compounds. The obtained data were analyzed by ANOVA test using SPSS software (version 19.0), and for all analyses, p-value<0.05 was considered significant. The 50% of maximum inhibitory concentration (IC50) and 50% of maximum effective concentration (EC50) values for OL-E and OL-L were defined by GraphPad Prism software (version 6.07).
Results
Evaluation of anti-diabetic indicators levels in β-TC6 cell line
Glucose uptake
The glucose uptake capability was directly correlated with the concentration of OL-E and OL-L, and the highest glucose uptake was observed in the concentration of 200 micromolar (). It seems that OL-E is more potent than OL-L (P < 0.05). The high concentrations (400 micromolar) did not show a remarkable difference in glucose uptake than a concentration of 200.
Figure 2. (A) The glucose uptake, (B) the insulin levels, (C) the cell viability as the percent of control, and (D) the pentosidine concentrations of β-TC6 pancreatic cells after 24 h treatment with different concentrations of OL-E and OL-L. The biological response of each substance was evaluated separately in cell lysate samples, and all data are presented as mean ± SD; n = 3
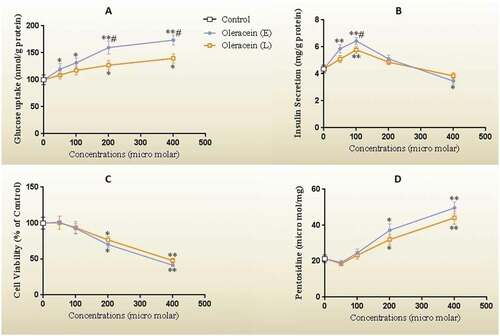
Insulin secretion
In the low to moderate concentrations, the studied compounds were considered to be dose-dependent and effective in evaluating insulin secretion (). The highest insulin secretion stimulation rate was observed at 100 micromolar, in which OL-E was more potent than OL-L (P < 0.05). The higher concentrations had no significant effects on insulin secretion compared to control and even reduced insulin secretion.
Cell viability
The effects of the investigated Oleracein derivatives on Beta-TC-6 pancreatic cells viability with MTT assay (presented in percentage of control) are shown in . No significant cellular toxicity was observed at low to moderate concentrations of the studied compounds. However, at concentrations higher than 100 micromolar, cell survival was attenuated with both OL-L and OL-E, and there was no notable difference between them in this regard (P < 0.05).
The advanced glycation end product (pentosidine)
Pentosidine levels regarded as a glycation index, and we observed that low to moderate concentrations of OL-E and OL-L did not produce any notable effect on this glycation end product (). However, we revealed that the higher concentrations of our investigated alkaloids (200 micromolar and higher) increased pentosidine, in which OL-E and OL-L were not significantly different from each other.
*Significant difference at P < 0.05 compared with the control according to one-way ANOVA, followed by Tukey’s post-hoc test.
** Significant difference at P < 0.001 compared with the control.
# Significant difference at P < 0.05 compared with OL-L.
Total cellular ceramide levels
Data revealed that low to moderate concentrations of the investigated compounds do not have a remarkable effect on total cellular ceramide (). However, in higher concentrations, ceramide generation was induced, and it was reached up to 2 times compared to the control group. OL-E and OL-L were not significantly different in this respect.
Figure 3. (A) Total cellular ceramide levels, (B) activity of acid ceramidase (AC), (C) activity of neutral sphingomyelinase (NS), (D) the activity of glucosylceramide synthase (GCS), and dose-response curve for each studied parameters of β-TC6 pancreatic cells after 24 h treatment with different levels of OL-E and OL-L. The biological response of each substance is evaluated separately in cell lysate samples, and all data are presented as mean ± SD; n = 3
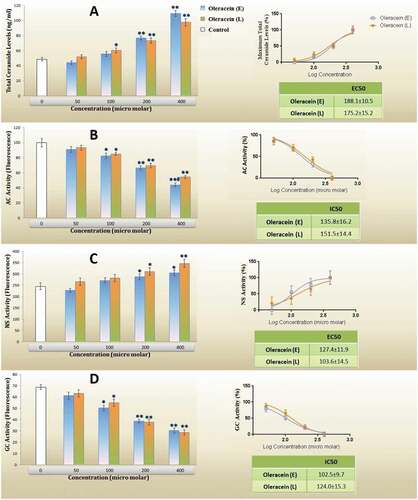
The activity of acid ceramidase
The AC activity and IC50 values are presented in . The treatment of β-TC6 cells with moderate to high concentrations of Oleracein derivatives reduced AC activity. In the concentrations of 400 micromolar, this enzyme activity decreased approximately by half the activity of the base, and OL-E and OL-L were not different.
The activity of neutral sphingomyelinase
There was a considerable increase in NS activity when the β-TC6 cells were treated with 200 micromolar and higher concentrations of the investigated compounds. However, there was no significant difference between OL-E and OL-L at the studied concentrations ().
The activity of glucosylceramide synthase
GCS activity decreases at concentrations greater than or equal to 100 μM of alkaloids, as presented in . Although low concentrations of Oleracein derivatives were not impressive for GCS activity, more substantial suppression of GCS was showed in the concentration of 400 micromolar of OL-E or OL-L (more than 50% of basal activity). Based on IC50 analysis, there was no significant difference between the investigated compounds in this parameter.
*Significant difference at P < 0.05 compared with the control according to one-way ANOVA, followed by Tukey’s post hoc test.
** Significant difference at P < 0.001 compared with the control.
Also, IC50 and EC50 of the investigated parameters confirmed no statistically significant difference between OL-E and OL-L.
Discussion
In traditional medicine, Portulaca oleracea has been applied as a favorable and harmless herbaceous treatment for DM without any severe complications such as hypoglycemic and insulin resistance [Citation14,Citation28]. Oleracein-E and Oleracein-L are introduced as the main components of this plant that present the antidiabetic effects.
In the current study, the effects of various concentrations of OL-E and OL-L on ceramide generation, ceramide associated metabolizing enzymes, glucose uptake, insulin secretion, cell survival, and glycation index levels in β-TC6 pancreatic cell line were studied.
Our data revealed that low to moderate concentrations of OL-E and OL-L had a positive effect on insulin secretion. Also, in pancreatic cells, glucose uptake increased in a dose-dependent manner with both studied compounds (P < 0.05). Phenolic alkaloids, including OL-E and OL-L, are a remarkable class of natural antioxidants in Purslane, and several studies have shown its potential therapeutic effects on diabetes and hypoglycemia [Citation29,Citation30]. Furthermore, the aqueous extract of Purslane is associated with the attenuation of elevated blood glucose and the prevention of diabetic microvascular dysfunction and damage [Citation31]. It has been reported that Purslane decreases blood sugar and induces insulin secretion by rehabilitating the defective pancreatic cells in diabetic rats. The hypoglycemic effects of Portulaca oleracea make it utilizable for the treatment of a disorder such as DM [Citation32,Citation33].
Another study showed that Portulaca oleracea had beneficial effects on the recovery of type 2 diabetes. In this study, the plant was administered orally to diabetic rats. Based on these studies, it was found that treatment with this plant reduced blood glucose, levels of plasma triglycerides, cholesterol, and LDL, and systolic blood pressure in mice with type 2 diabetes. This plant also improves the function of acetylcholine. Therefore, it is suggested that the consumption of this plant inhibits hyperglycemia, vascular inflammation, and endothelial dysfunction in diabetics [Citation31].
The mechanism of action of this plant can be related to the increase in insulin secretion through the closure of K + -ATP channels, causing depolarization of the membrane and stimulation of Ca2 + penetration into the membrane, which is the first step for insulin secretion. Therefore, it can be concluded that P. oleracea has anti-diabetic properties by increasing the stimulation of insulin secretion and can help in the healing process of diabetic patients [Citation34].
According to the results of the present study, the amount of glycation index changes at different concentrations of E-oleracin and L-oleracin alkaloids. Pentosidine levels in pancreatic BTC6 cell line showed a significant increase compared to the control due to the increased treatment with E-oleracin and L-oleracin.
The results showed that high concentrations of OL-E and OL-L suppressed insulin secretion and the formation of pentosidine as AGEs.
AGEs are proteins or lipids that undergo glycation as a result of exposure to glucose. Among the AGEs, Pentosidine is a determining factor in senescence and the progress of many degenerative disorders, such as DM [Citation35]. Hence targeting AGEs is a theoretical approach to reduce diabetic complications [Citation36].
Ceramide molecules are generated by different enzymes, which add free fatty acid with the various carbon numbers to sphingosine as a backbone [Citation37]. The biosynthesis and cleavage of ceramide are controlled by separate enzymes whose activities may modify cellular ceramide content [Citation38].
This basic sphingolipid mediator is a key player in the pathogenesis of type 1 and type 2 DM and its related complications [Citation9].
According to the results obtained from experiments related to pancreatic cell line BTC6, ceramide and sphingomyelinase levels increased significantly at high concentrations of E-oleracin, while ceramidase and sphingomyelinase enzyme levels decreased significantly. At high concentrations of L-oleracein, the amounts of ceramide and sphingomyelinase also showed a significant increase and the levels of ceramidase and glycosyl ceramide synthase decreased significantly.
Therefore, high concentrations of E-oleracin and L-oleracin in the pancreatic cell line increase the amounts of ceramide and sphingomyelinase and decrease the levels of ceramidase and glycosyl ceramide synthase enzymes.
Our data indicated that although low to moderate concentrations of investigated phenolic alkaloids do not affect ceramide levels, the high concentrations of OL-E and OL-L are a positive stimulus for ceramide generation.
Several in vitro and in vivo studies have demonstrated that elevated ceramide biosynthesis correlates to the development of insulin resistance, attenuation of insulin gene expression, and pancreatic β-cell loss with apoptosis during the expansion of diabetes [Citation39,Citation40]. Ceramide-induced insulin resistance and β-cell apoptosis occur due to multiple mechanisms, including increasing cytochrome c release and inhibition of Akt (protein kinase B) [Citation9].
Sphingolipids, such as ceramide and sphingosine, are important components of cell membranes that have a messaging function in cell division, life, and death. Exogenous use of ceramide can cause cytotoxicity caused by the increased catabolism of sphingomyelin to ceramide. Catabolism of ceramide into sphingosine and then its conversion to sphingosine-1-phosphate prevent ceramide-induced apoptosis. Therefore, inhibition of ceramide catabolism can help improve the quality of cancer cell treatment. Today, many anticancer drugs kill cancer cells and prevent cancer progression by increasing the re-synthesis of ceramide in these cells [Citation41].
In this study, hypoglycemic and anti-diabetic effects were observed in alkaloids E-oleracein and L-oleracein from P. oleracea plant by increasing the stimulation of insulin secretion and glucose uptake, which can help in the recovery process of diabetic patients. Since an increase in ceramide at the cell surface leads to cell death, inhibition of ceramide catabolism can help improve the quality of cancer cell treatment. Because ceramide suppresses the progression of cancer cells, defects in ceramide production and sphingolipid metabolism lead to cancer cell survival and cancer resistance. Therefore, it can be assumed that E-oleracin and L-oleracin in P. oleracea can kill pancreatic cancer cells and maintain the survival of other remaining cells.
According to the results of this study, this plant can be used as a good nutritional source, which can protect the body due to its high alkaloid content.
Conclusion
The present study results demonstrated that as major isoquinoline alkaloids in Portulaca oleracea, low to moderate concentrations of both OL-E and OL-L have a considerable stimulating effect on insulin secretion and improving glucose uptake. These findings are in accordance with the results of other related investigations. The important point is that a high dose of Oleracein derivative is associated with increasing the ceramide generation and cellular toxicity and decreasing insulin secretion. Consequently, OL-E and OL-L can be recommended as a potential natural antidiabetic agent in preventing or treating DM and its multiple complications. Nevertheless, it should be regarded that the excessive consumption of these compounds may result in inconsistent and undesirable responses with possible involvement of the ceramide pathway. Further studies are recommended to augment and confirm our suggestions.
Disclosure statement
The authors declare no conflicts of interest.
References
- Roozi H, Bojar MA, Eidi V, et al. Effects of oleracein E and oleracein L from portulaca oleracea on cell survival, antioxidant and antidiabetic efficacy on beta-TC-6 pancreatic cell line. Indian J Pharm Sci. 2019;81(4):681–689.
- Chawla A, Chawla R, Jaggi S. Microvasular and macrovascular complications in diabetes mellitus: distinct or continuum? Indian J Endocrinol Metab. 2019;81(4):546.
- Fu Z, Gilbert ER, Liu D. Regulation of insulin synthesis and secretion and pancreatic Beta-cell dysfunction in diabetes. Curr Diabetes Rev. 2013;9(1):25–53.
- Huang Q, Chen L, Teng H, et al. Phenolic compounds ameliorate the glucose uptake in HepG2 cells' insulin resistance via activating AMPK: anti-diabetic effect of phenolic compounds in HepG2 cells. Journal of Functional Foods. 2015;19:487–494
- Beisswenger PJ, Moore LL, Brinck-Johnsen T, et al. Increased collagen-linked pentosidine levels and advanced glycosylation end products in early diabetic nephropathy. J Clin Invest. 1993;92(1):212–217.
- Nowotny K, Jung T, Höhn A, et al. Advanced glycation end products and oxidative stress in type 2 diabetes mellitus. Biomolecules. 2015;5(1):194–222.
- Summers SA. Ceramides in insulin resistance and lipotoxicity. Prog Lipid Res. 2006;45(1):42–72.
- Boojar MMA, Hassanipour M, Mehr SE, et al. New aspects of silibinin stereoisomers and their 3-O-galloyl derivatives on cytotoxicity and ceramide metabolism in Hep G2 hepatocarcinoma cell line. Iran J Pharm Res. 2016;15(3):421.
- Galadari S, Rahman A, Pallichankandy T, et al. Role of ceramide in diabetes mellitus: evidence and mechanisms. Lipids Health Dis. 2013;12(1):98.
- Haus JM, Kashyap SR, Kasumov S, et al. Plasma ceramides are elevated in obese subjects with type 2 diabetes and correlate with the severity of insulin resistance. Diabetes. 2009;58(2):337–343.
- Boojar MA, Masoud Mashhadi Akbar Boojar M, Golmohammad S. Ceramide pathway: a novel approach to cancer chemotherapy. Egypt J Basic Appl Sci. 2018;5(4):237–244.
- Kooti W, Farokhipour M, Asadzadeh Z, et al. The role of medicinal plants in the treatment of diabetes: a systematic review. Electron Physician. 2016;8(1):1832. .
- Karimi G, Alireza A, Abbas O, et al. Protective effect of aqueous and ethanolic extracts of Portulaca oleracea against cisplatin induced nephrotoxicity. Iran J Basic Med Sci. 2010;13(2):31–35.
- Zhou Y-X, Xin H-L, Rahman K, et al. Portulaca oleracea L.: a review of phytochemistry and pharmacological effects. Biomed Res Int. 2015; 2015. doi:https://doi.org/10.1155/2015/925631
- Xiang L, Wang W, Wang R-F, et al. Alkaloids from Portulaca oleracea L. Phytochemistry. 2005;66(21):2595–2601.
- Chen T, Wang J, Li Y, et al. Sulfated modification and cytotoxicity of Portulaca oleracea L. polysaccharides. Glycoconj J. 2010;27(6):635–642.
- Dong C-X, Hayashi K, Lee J-B, et al. Characterization of structures and antiviral effects of polysaccharides from Portulaca oleracea L. Chem Pharm Bull. 2010;58(4):507–510.
- Zidan Y, Bouderbala S, Djellouli F, et al. Portulaca oleracea reduces triglyceridemia, cholesterolemia, and improves lecithin: cholesterol acyltransferase activity in rats fed enriched-cholesterol diet. Phytomedicine. 2014;21(12):1504–1508.
- Pakdel R, Niazmand S, Mouhebati M, et al. A comparison between the effects of Portulaca oleracea seeds extract and valsartan on echocardiographic and hemodynamic parameters in rats with levothyroxine-induced thyrotoxicosis. Avicenna J Phytomed. 2018;8(3):276.
- Lei Q, Liu H, Peng Y, et al. In silico target fishing and pharmacological profiling for the isoquinoline alkaloids of Macleayacordata (Bo Luo Hui). Chin Med. 2015;10(1):37.
- Sun H, He X, Liu C, et al. Effect of Oleracein E, a neuroprotective tetrahydroisoquinoline, on rotenone-induced Parkinson’s disease cell and animal models. ACS Chem Neurosci. 2017;8(1):155–164. .
- Jiao -Z-Z, Yue S, Sun H-X, et al. Indoline Amide glucosides from portulaca oleracea : isolation, structure, and DPPH radical scavenging activity. J Nat Prod. 2015;78(11):2588–2597. .
- Henle T, Schwarzenbolz U, Klostermeyer H. Detection and quantification of pentosidine in foods. Zeitschrift für Lebensmitteluntersuchung und-Forschung A. 1997;204(2):95–98.
- He X, Dagan A, Gatt S, et al. Simultaneous quantitative analysis of ceramide and sphingosine in mouse blood by naphthalene-2,3-dicarboxyaldehyde derivatization after hydrolysis with ceramidase. Anal Biochem. 2005;340(1):113–122. .
- Morad SAF, Levin JC, Tan S-F, et al. Novel off-target effect of tamoxifen—inhibition of acid ceramidase activity in cancer cells. Biochim Biophys Acta (BBA)-Mol Cell Biol Lipids. 2013;1831(12):1657–1664.
- Hojjati MR, Jiang X-C. Rapid, specific, and sensitive measurements of plasma sphingomyelin and phosphatidylcholine. J Lipid Res. 2006;47(3):673–676.
- Hayashi Y, Zama K, Abe E, et al. A sensitive and reproducible fluorescent-based HPLC assay to measure the activity of acid as well as neutral β-glucocerebrosidases. Anal Biochem. 2015;10(1):122–129.
- El-Sayed M-I. Effects of portulaca oleracea L. seeds in treatment of type-2 diabetes mellitus patients as adjunctive and alternative therapy. J Ethnopharmacol. 2011;137(1):643–651.
- Yang Z, Liu C, Xiang L, et al. Phenolic alkaloids as a new class of antioxidants in Portulaca oleracea. Phytother Res. 2009;23(7):1032–1035.
- Iranshahy M, Javadi B, Iranshahi M, et al. A review of traditional uses, phytochemistry and pharmacology of Portulaca oleracea L. J Ethnopharmacol. 2017;205:158–172.
- Lee AS, Lee YJ, Lee SM, et al. Portulaca oleracea ameliorates diabetic vascular inflammation and endothelial dysfunction in db/db mice. Evid Based Complement Alternat Med. 2012;2012.
- Gong F, Li F, Zhang L, et al. Hypoglycemic effects of crude polysaccharide from purslane. Int J Mol Sci. 2009;10(3):880–888.
- Ramadan BK, Schaalan MF, Tolba AM. Hypoglycemic and pancreatic protective effects of Portulaca oleracea extract in alloxan induced diabetic rats. BMC Complement Altern Med. 2017;17(1):37.
- Lei X, Barbour SE, Ramanadham S. Group VIA Ca2+-independent phospholipase A2 (iPLA2β) and its role in β-cell programmed cell death. Biochimie. 2010;92(6):627–637.
- Jakuš V, Rietbrock N. Advanced glycation end-products and the progress of diabetic vascular complications. Physiol Res. 2004;53(2):131–142.
- Goh S-Y, Cooper ME. The role of advanced glycation end products in progression and complications of diabetes. J Clin Endocrinol Metab. 2008;93(4):1143–1152.
- Yabu T, Shiba H, Shibasaki Y, et al. Stress-induced ceramide generation and apoptosis via the phosphorylation and activation of nSMase1 by JNK signaling. Cell Death Differ. 2015;22(2):258–273.
- Pettus BJ, Chalfant CE, Hannun YA. Ceramide in apoptosis: an overview and current perspectives. Biochim Biophys Acta (BBA)-Mol Cell Biol Lipids. 2002;1585(2–3):114–125.
- Boslem E, Meikle PJ, Biden TJ. Roles of ceramide and sphingolipids in pancreatic β-cell function and dysfunction. Islets. 2012;4(3):177–187.
- Mandrup-Poulsen T. beta-cell apoptosis: stimuli and signaling. Diabetes. 2001;50(suppl 1):S58.
- Patrick RC, Maurer BJ, Kolesnick RN. Ceramide synthesis and metabolism as a target for cancer therapy. Cancer Lett. 2004;206(2):169–180.