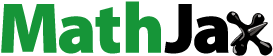
ABSTRCT
A simple and efficient technique for quantitative solvent-free synthesis of Ni (II) and Co (II) complexes by using ball milling as a green strategy is studied. The isolated solid compounds will be characterized using micro-analytical, thermo-gravimetric, magnetic and spectroscopic studies. Theoretical studies were carried out by applying DFT theory to predict the optimized configuration for all isolated solid complexes. Moreover, the in vitro antimicrobial activity assay versus fungi and bacteria via MIC technique has higher potency of Ni (II) than Co (II). The in vitro cytotoxicity of human muscle rhabdomyosarcoma (RD) via MTT technique and the antioxidant by DPPH free radical scavenging has higher inhibitory effect on complexes than free ligand. The cyclic voltammetry of Co(II) in absence/presence of H2BHNH was investigated to realize the significant effect of complex formation on the electrochemical manners of Co(II).
Introduction
Cancer is one of the most feared diseases in the world today because it is not easy to treat; this is due to cancer that comes as a result of uncontrolled multiplication of modified normal cell of human. So, the potential way of cancer treatment is drug therapy. Whereas, the drugs used to battle cancer join one of two wide types. The first is cytotoxic drugs in which the cell is killed and the second is cytostatic in which the cell stabilizes the drugs. Both types cause a decrease in the tumor size because cancer cell has a high humanity rate, which only preventing them from isolating will cause a decrease in the inhabitants [Citation1–4].
Metals complexes are fundamental cellular components chosen to function in numerous essential biochemical processes for living organisms. Generally, metals have unique characteristics that involve reactivity toward organic substrates, variable coordination modes, and redox activity. For these reasons, the design of special coordination complexes, whichever as drug or pro-drug, is considered the main target to get a powerful tool in cancer diagnosis [Citation5–8]. Based on the interface between molecular bioinorganic chemistry and biological system, the design of coordination complexes for cancer treatment in the current research is extensive in scope, targets multiple cellular and biological properties of many types of tumor. Recently, the improvement of anticancer drugs stimulated from cytotoxicity toward the design of selective agents, which act on definite cellular targets [Citation9–11].
So according to the previous facts, Ni (II) and Co(II) complexes with high biological efficiency will be prepared in an efficient and environmentally friendly way, which is to grind the reactors using ball milling technique as a green approach [Citation12,Citation13]. The current advance in nanotechnology led to the improvement of new metallic nanoparticles that eventually increase the environmental hazards. The main aim is to minimize the hazard of synthetic procedures, which are associated with derivative compounds and chemicals used.
Experimental
Apparatus
The apparatus used to propose the configuration for prepared compounds was displayed in Scheme 1S.
Synthesis of H2BHNH and its metal complexes
The Schiff base ligand was formed as displayed in . Also, the waste-free and facile solid state ball milling technique was used to prepare Ni (II) and Co (II) complexes as a green strategy of chemistry in pure state with high yield (99%) as shown in .
Conformational calculations
The quantum chemical parameters were estimated by applying DMOL3 program [Citation14] in Materials Studio package [Citation15]. The configuration of produced ligand and its metal complexes was proposed by applying Density Functional [Citation16].
Antimicrobial activity assay
In microbiology, the MIC technique was generally the initial point for larger pre-clinical evaluations of novel antimicrobial agents [Citation17,Citation18]. So, for drug discovery, the first step is the screening of a records drug candidate for MICs versus tested bacteria or fungi as shown in Scheme 2S.
In vitro cytotoxicity assay
The cytotoxic effect of tested compounds on Rhabdomyosarcoma cell lines (RD) were performed by determining the absorbance of each well at 570 nm via applying ELISA (Enzyme-linked immunosorbent assay) microplate reader (Thermo Electron Corporation Multiskan-EX reader) [Citation19] and mean optical density for each concentration was measured (Scheme 3S). The effect is expressed as % cell viability as shown in this relation:
Antioxidant Activity
The free radical-scavenging activity of tested compounds was determined by applying Blois method [Citation20] using DPPH as a stable radical scavenging (Scheme 4S). The ability to scavenge the DPPH radical was determined by applying this equation [Citation21]:
Acontrol: The absorbance of control
Asample: The absorbance of the sample
Cyclic voltammetry
The cyclic voltammetry was estimated for Co(II) in absence/presence of ligand by using three types of electrodes which are reference electrode (Ag/AgCl/KCl), the glassy carbon working electrode (GCWE) and an auxiliary electrode (platinum wire) that immersed in 30 ml 0.l M KCl as supporting electrolyte which connect with potentiostat of the type DY 2100.
Results and discussion
General characteristics
The isolated solid complexes of Ni (II) and Co (II) were physically and analytically characterized to set up their chemical formula and also have non-hygroscopic nature. The non-conducting aspect was proposed for Ni (II) and Co (II) complexes, owing to molar conductivity values for 10−3M are 4, 7 Ω−1cm2mol−1, respectively [Citation22].
1H NMR and IR spectra studies
The 1H NMR spectrum of H2BHNH in DMSO is displayed in have two signals at 11.98 and 11.30 ppm assignable to the protons of (OH)naphthoic & (NH) correspondingly. The intramolecular hydrogen bonding was suggested to be due to the appearance of (OH)naphthoic signal at high value downfield from TMS. Also, the aromatic and (-N = C-H) protons have multiplet signals displayed in 7.34–8.47 ppm range.
In the IR spectrum of H2BHNH, the bands of υ(C = N) & υ(C = O) were displayed at 1622 & 1646 vibrations [Citation23], correspondingly (). The bands of υ(OH)naphthoic & υ(NH) were observed at 3449 & 3271 cm−1, respectively [Citation23]. The intramolecular hydrogen bonding was suggested to be due to the existence of υ(OH) band at 3449 cm−1. Also, there is another confirmation for the existence of intramolecular hydrogen bonding (O-H··O) which is the presence of broad weak bands in the 1900–2050 and 2100–2230 cm−1 regions [Citation23].
Table 1. Analytical and physical data of H2BHNH and its metal complexes
Table 2. Most important IR spectral bands of H2BHNH and its metal complexes
In IR spectra of Ni(II) as well as Co(II) complexes the H2BHNH behaved as a mononegative tridentate ligand coordinating via (C = N)azo, (C = O) & (OH)naphthoic with the displacement of the hydrogen atom from it. This manner of complexation was recommended depending on; (i) the absence (OH)naphthoic group; (ii) the lower shift of υ(C = O) as well as υ(C = N) and (iii) the appearance of new bands at (508, 511) & (416, 430) cm−1 that ascribed to υ(M-O) & υ(M-N) [Citation23], correspondingly.
Electronic spectra, magnetic properties and ligand field parameters
The UV-visible data in DMSO, magnetic moments and ligand field parameters of Ni(II) & Co(II) complexes are collected in and represented graphically in Figure S1. The outcomes results suggested octahedral configuration for Ni(II) & Co(II) complexes [Citation24].
Table 3. Magnetic moments, electronic spectra and ligand field parameters of H2BHNH metal complexes
XRD
The diffraction patterns of investigated Ni(II) & Co(II) complexes as shown in were determined in of 10° < 2θ <80° region at RT by X-ray diffraction using (Cu, Kα) radiation [Citation25]. The patterns displayed sharp peaks in two investigated complexes reflecting crystalline nature [Citation25]. The crystallite size was determined through FWHM for characteristic peaks by applying the equation of Deby–Scherrer:
θ: The diffraction angle, Cu/Kα (λ) = 1.5406 Ao
S: The crystallite size
B: The line width at half maximum height
Also, by applying Bragg equation, the values of inner crystal plane d-spacing are calculated as follows:
The particle size as well as lattice parameter values are displayed in for examined complexes. The particle size appeared within 0.740 & 0.741 nm for Ni(II) & Co(II) complexes, respectively.
Table 4. XRD data for Co(II) and Ni(II) complexes
Scanning electron microscope studies
The SEM of the Co(II) & Ni(II) complexes displayed that the presence of small grains of non-uniform size and the particles were agglomerated with controlled morphological structure as shown in . Also, the SEM images of Co(II) & Ni(II) complexes reveal irregular shaped grains [Citation26].
Thermal studies of Ni(II) complex
The TG curves of [Ni(HBHNH)2].2H2O complex are thermally stable up to 137°C, above that point the dehydration of complex begins [Citation27,Citation28]. This low temperature indicated that the two molecules of water are not coordinated with the nickel ion. In the temperature range 137°C–198°C, the TG curve displayed 5.3% weight loss that related to two water molecules as shown in Figure S2. The degradation of organic moiety started >198°C. In the TG curve, the temperature ranges 198°C–329°C, the weight loss 23.0% was ascribed to the partial decomposition of the chelating agent with the removal of the two loosely bound phenyl moieties (2C6H5). The second weight loss stage (329°C–410°C) which could be correlated with the elimination of the two (C2H2N2) portions. Finally, at 618°C the weight loss of 44.4% was mainly ascribed to complete decomposition of the remaining more tightly bound fragment of the organic moiety, besides breaking the chelate bond, leaving nickel oxide (11.2%) of the complex initial mass.
Thermodynamic and kinetic studies
Kinetic parameters
Horowitz–Metzger and Coats–Redfern equations [Citation29,Citation30] were applied to estimate the kinetic as well as thermodynamic parameters of the decomposition steps for Ni(II) complex as shown in and . The outcomes data from the two applied methods were nearly similar to a certain extent, so the results can be concluded as follows:
Figure 4. Coats–Redfern and Horowitz–Metzger plots for [Ni(HBHNH)2].2H2O (a) first step, (b) second step, (c) third step and (d) fourth step
![Figure 4. Coats–Redfern and Horowitz–Metzger plots for [Ni(HBHNH)2].2H2O (a) first step, (b) second step, (c) third step and (d) fourth step](/cms/asset/bdb82743-7ba3-4a95-8f82-44660d186eec/teba_a_1909208_f0004_oc.jpg)
Table 5. Kinetic parameters evaluated by Coats–Redfern and Horowitz–Metzger equations for Ni(II) complex
The higher values of ∆G demonstrated the lower elimination rate of H2BHNH from one step to another.
The decomposition step was non-spontaneous process due to positive sign of ∆G referred to the higher quantity of residue than the beginning.
The positive values of ΔS referred to the disordered reaction, while the negative values indicated ordered reaction.
DTG curves referred to high activation energies that indicated the high stability of Ni(II) complex.
The decomposition steps are endothermic processes due to positive sign of ∆H.
Computational studies
Optimized molecular structure
The optimized molecular structures for H2BHNH and its Ni(II) & Co(II) complexes are represented in . The bond length as well as bond angle are renowned for the following explanations:
On complexation the bond angles of H2BHNH were altered where the major change observed through ‘C(14)-C(8)-O(11), O(13)-C(14)-N(15), N(15)-C(17)-H(31)’ angles.
The bond angles of the isolated solid complexes were referred to an octahedral geometry with d2sp3 or sp3d2 [Citation31].
The bond angles of the investigated complexes were longer than free ligand due to donor atoms shared in coordination [Citation32].
On chelation, a large difference in bond lengths observed through C(8)-O(11), O(11)-H(12), C(14)-O(13), N(15)-H(30), N(15)-N(16) and C(17)-H(31) atoms of free ligand than complexes. Anywhere, the bond length of investigated complexes became more longer than the ligand [Citation33].
In addition, DFT theory was applied to calculate the dipole moment, sum of atomic energies, electrostatic energy, spin polarization energy, binding energy and kinetic energy, total energy, LUMO, HOMO as represented in and .
Table 6. The molecular parameters of the H2BHNH and its complexes
MEP
Both electrophilic and nucleophilic attack points were resolute by electrostatic potential. The MEP of H2BHNH and its Ni(II) & Co(II) complexes that were shown in displayed three characteristic colors: blue color that referred to electron-poor area which prefers the nucleophilic attack. On the other hand, red color referred to electron rich which preferred electrophilic attack. While, the green color referred to electrostatic potential area [Citation34].
Biological studies
Antimicrobial studies
A lot of studies were established an increase in antimicrobial activity following the interaction of active organic compounds with metal ions. The current study used a methodology modified for antimicrobial bioassays against Gram-positive (+)ve as well as Gram (-)ve bacteria. The results obtained were considered appropriate for determining MIC for performing antimicrobial sensitivity testing with good quality reproducibility as well as efficiency.
The antimicrobial activities of H2BHNH and its Ni(II) & Co(II) complexes (0.2 ml of 10 μg/ml DMSO) versus P. Aeurginosa & B. Subtillis are represented in . Generally, the studies ligand showed variable antibacterial activity against either B. Subtillis & P. Aeurginosa. Moreover, the activities were dependent upon the type of the organic ligand used. On the other hand, the antimicrobial study of the isolated metal complexes versus the tested bacteria which arranged in the subsequent order: Ni(II) > Co(II) > H2BHNH. The data also showed the dependence of the antimicrobial activity on the size of metal cation used where the higher the size of the metal ion, the higher the antibacterial activity.
Table 7. Diameter of Inhibited Zones (I. Z. D.) in mm as a criterion of antibacterial activity of the H2BHNH and its complexes at concentration level of mg/l
Cytotoxicity
The cytotoxicity of H2BHNH and its Ni(II) & Co(II) complexes assayed at various concentrations (50, 150, 200 and 400 μg/mL) on RD (ATCC) human muscle cell line was shown to have cell inhibition in range of 51–68%, 41–56% and 46–58%, correspondingly (note that: the number of observed viable cells was decreased). The cytotoxic potential of Ni(II) is greater than H2BHNH as shown in ( and ). The cytotoxicity was observed to increase with the increase in concentration.
Table 8. % Cell viability
Antioxidant studies
Various researchers have used scavenging effect of synthesized compounds on DPPH radical as a quick and reliable parameter to measure the in vitro antioxidant activity. DPPH is stable nitrogen radical that bears no similarity to the highly reactive and temporary peroxyl radicals concerned with the peroxidation of lipids. A lot of antioxidants that react quickly to peroxyl radicals may even be inert to DPPH owing to steric seclusion. Also, DPPH was decolorized by reducing agents as well as H transfer that contributes to inaccurate interpretations of antioxidant capacity.
The antioxidant activity of the H2BHNH and its Ni(II) & Co(II) complexes studied by DPPH method at 20 µL – 140 µL showed scavenging potential (∼19-43%), (∼23-48%) and (∼27-51%), correspondingly ( and ) substantiating the fact that complexation leads to enhanced antioxidant activity [Citation35–37]. The discoloration from purple DPPH radical solution to yellow may be ascribed to hydrogen donation from the (O-H) and (N-H) groups in line with earlier reports on different metals [Citation38]. The resultant free radical formed in complexes through the exchange mechanism was more stabilized due to charge delocalization than that in case of the ligand thereby affording increased antioxidant activity of complexes over ligand.
Table 9. % DPPH scavenging activity data at T = 60 min
Cyclic voltammetry of CoCl2
The cyclic voltammetry technique was applied within the potential window of (0.8 to −0.8 V) using scan rate 0.1 V/sec at 298.15 K to study the electrochemical redox mechanism of Co2+ in 0.1 M KCl as a supporting electrolyte and GC as working electrode (). In the forward scan, (Co3+/Co2+) cathodic potential peak (Epc1) and (Co2+/Co1+) cathodic potential peak (Epc2) come into view at 0.121 V and – 0.325 V, correspondingly. While, in the reverse scan the (Co1+/Co2+) anodic potential peak (Epa2) exists at 0.035 V followed by (Co2+/Co3+) anodic potential peak (Epa1) at 0.212 V. Thus, the redox mechanism of the reaction can be represented as follows:
After that, the peak current is given by the Randles-Sevcik equation [Citation39].
Ip: the current in ampere
D: diffusion coefficient in cm2/s
A: surface electrode area (cm2)
C: molar concentration of metal ion (mol/cm3)
Ν: scan rate in volts/sec
Furthermore, the difference potential (ΔEP) was determined from the following equation [Citation40]
The above quantities (Epa1, Epa2, Epc1, Epc2, Ip) can be used to estimate numerous cyclic voltammetric parameters such as Q, Γ, αna & ks. The surface concentration of the redox ion was determined by applying the following equation [Citation41]
iP: the peak current
F: faraday constant (96485.33 coulomb)
T: temperature in K
N: the number of e− in redox reactions
R: gas constant (8.314 J.mol−1.K−1)
A: the surface area of working electrode in cm2
Ν: the scan rate in Vsec−1
The charge transfer coefficient of electrons was evaluated by applying the following equation [Citation42]
In case of cathodic peak, Epc/2 is the half-wave potential.
The charge consumed during the redox reaction was determined by applying the following equation [Citation43].
The heterogeneous charge transfer rate constant (ks) was estimated by applying the following equation:
DC: the diffusion coefficient of oxidized particles
N: electrons number in redox reactions
R: the gas constant
F: Faraday Constant
T: the temperature in K
Α: charge transfer coefficient
ΔEP: the difference potential
na: scan rate and the number of electrons transfer in the rate determining step
Figure 10. Cyclic voltammogram of CoCl2 in the presence of different concentration of H2BHNH at 298.15 K
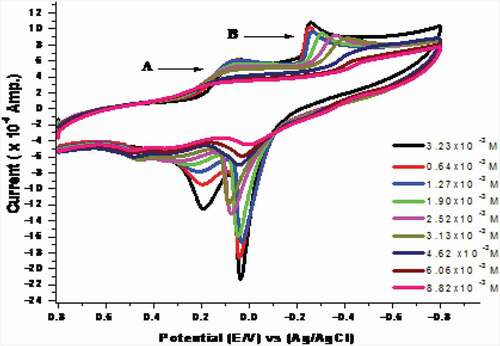
The previous parameters were estimated for the anodic as well as cathodic peak and tabulated in . Most of the evaluated parameters for the anodic and cathodic peaks increase as the concentration of CoCl2 increases. The increase in all peak currents supported the suggested diffusion mechanism [Citation44].
Table 10. Solvation and kinetic parameters D, kS, Γ and Q of CoCl2 at 298.15 K for wave (B) in absence of H2BHNH
Cyclic voltammetry response of Co(II) in presence of H2BHNH
The electrochemical behavior was studied for complexation between H2BHNH and CoCl2 in 0.1 M KCl solution at GCE within (+0.8 to −0.8 V) potential window (). The result current redox peaks are decreased by increasing the ligand concentration that favoring the covalency between the cobalt ions and H2BHNH. Furthermore, the cathodic & anodic potential peaks were shifted. These outcomes results recommend a strong interaction between cobalt and H2BHNH.
Figure 11. Cyclic voltammogram of CoCl2 at different scan rates at final adding in absence of H2BHNH at 298.15 K
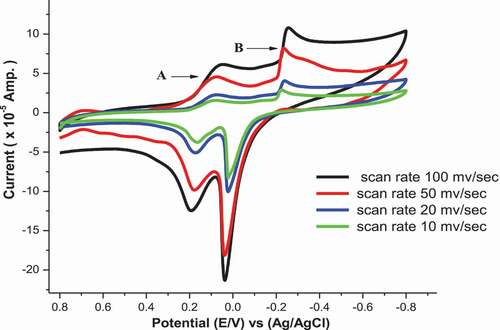
In addition to the previous variations in the shape of voltammogram, the calculated values of EPc, EPa, ∆Ep, iPa, iPc, (iPa/iPc), E°, ks, αna, Γ and Q for CoCl2 in the presence of H2BHNH ().
Table 11. Solvation and kinetic parameters D, ks, Γ and Q of CoCl2 at 298.15 K for wave (B) in presence of H2BHNH
Effect of scan rate in (absence/presence) of H2BHNH
The cyclic voltammograms of Co2+ in (absence/presence) for H2BHNH at different scan rates 10, 20, 50 & 100 mV/s in 0.1 M KCl at 298.15 K are displayed in and . The outcomes data showed that both cathodic & anodic peak currents increase in linear manner with the increasing in the scan rate. Also, the cathodic peak was slightly shifted toward the negative potential while, the anodic peak was slightly shifted toward positive direction with the extent of the scan rate. These remarks suggest the diffusion controlled redox mechanism and supported by the linear relation between square root of scan rate and peak currents as displayed in . The ratio of peak current is >1 that continue a correspondence to a quasi reversible system [Citation45]. The solvation and kinetic parameters for CoCl2 in absence/presence of H2BHNH at various scan rates were shown in .
Figure 12. Cyclic voltammogram of CoCl2 at different scan rates at final adding in presence of H2BHNH at 298.15 K
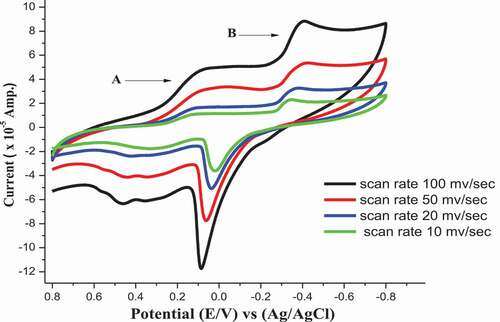
Figure 13. Relation (ip vs. ν1/2) for CoCl2 at final adding in absence of H2BHNH at 298.15 K and different scan rate
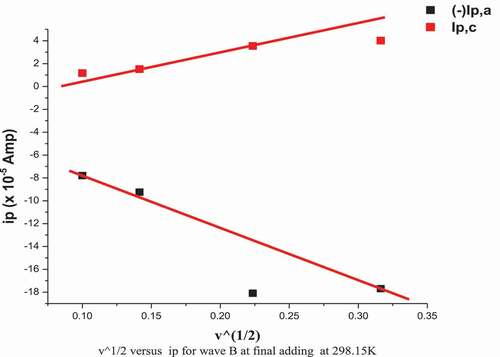
Figure 14. Relation (ip vs. ν1/2) for CoCl2 in presence of H2BHNH by molar ratio (1:1) at 298.15 K and different scan rate
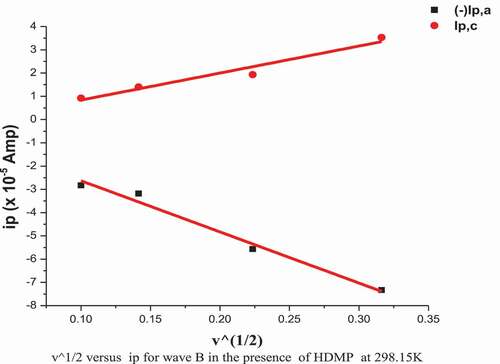
Table 12. Effect of scan rate on CoCl2 [3.23x10−3 M] for wave (B)
Table 13.. Effect of scan rate on co-complex for wave (B)
Conclusion
The bivalent Ni (II) and Co (II) complexes of N’-benzylidene-3-hydroxy-2-naphthohydrazide ligand were synthesized by ball milling as an efficient technique for quantitative solvent-free synthesis. The isolated solid compounds will be characterized using several spectroscopic techniques. The theoretical studies represented in the molecular modeling of isolated solid compounds were investigated using the DFT method which showed the compatibility with the practical outcomes data. Moreover, the in vitro antimicrobial activity assay versus fungi and bacteria via MIC technique has higher potency of Ni (II) than Co (II). The in vitro cytotoxicity on human muscle rhabdomyosarcoma (RD) via MTT technique and the antioxidant by DPPH free radical scavenging have higher inhibitory effect of complexes than free ligand. Voltametric studies explained the effect of Co(II) in absence/presence of H2BHNH on the electrochemical behavior of cobalt solution through the change in the values of (iP, E, Γ, αna, rate ks, and Q).
Supplemental Material
Download MS Word (4.9 MB)Disclosure statement
No potential conflict of interest was reported by the authors.
Supplementary material
Supplemental data for this article can be accessed here.
References
- Zaky R, Fekri A, Abou El-Reash Y, et al. Bivalent transition metal complexes of 3-(2-(4-(dimethylamino)benzylidene)hydrazinyl)-3-oxo-N-(thiazol-2-yl)propanamide: structural, spectral, DFT, ion-flotation and biological studies. EJBAS. 2016;3:3272–3286.
- Al-Hazmi GAA, Abou-Melha KS, El-Metwaly NM, et al. Green synthesis for 3-(2-Benzoylhydrazono)-N-(pyridin-2-yl) butanamide complexes: spectral, analytical, modelling, MOE docking and biological studies. J Inorg Organomet Polym Mater. 2020;30:1519–1536.
- Baile B, Kolhe S, Deotarse P, et al. Metal ion complex -potential anticancer drug- A review. Int J Pharm Sci Rev Res. 2015;4:59–66.
- Yousef TA, Abu El-Reash GM, El-Gammal OA, et al. Characterization, quantum, antibacterial, antifungal and antioxidant studies on Hg(II) and Cd(II) complexes of allyl and ethyl thiosemicarbazides derived from 2- aminothiazole-4-yl acetohydrazide. EJBAS. 2016;3:40–60.
- Al-Janabi ASM, Zaky R, Yousef TA, et al. Synthesis, characterization, computational simulation, biological and anticancer evaluation of Pd(II), Pt(II), Zn(II), Cd(II), and Hg(II) complexes with 2-amino-4-phenyl-5-selenocyanatothiazol ligand. J Chin Chem Soc. 2020;67:1–13.
- Tella AC, Owalude SO, Alimi LO, et al. Facile synthesis and vapochromic studies of Co(II) complexes bearing NO and OO donor ligands. EJBAS. 2016;3:125–135.
- Kenawy I, Hassanien M, Abdel-Rhman M, et al. Synthesis and characterization of Hg(II) and Cd(II) complexes derived from the novel acenaphthaquinone-4-phenyl thiosemicarbazone and its CPE application. EJBAS. 2016;3:106–117.
- Yaman M, Kaya G, Yekeler H. Distribution of trace metal concentrations in paired cancerous and non-cancerous human stomach tissues. World J Gastroenterol. 2007;13:612–618.
- Jakupec MA, Galanski M, Arion VB, et al. Antitumour metal compounds: more than theme and variations. Dalton Trans. 2008;183–194. DOI:https://doi.org/10.1039/B712656P
- Munshi AM, Aa B, Abualnaja M, et al. Ball-milling synthesis technique for Cu(II)-Schiff base complexes with variable anions; characterization, potentiometric study and in-vitro assay confirmed by in-silico method. Inorg Chem Commun. 2021;127:108542.
- Mertz W. Essential trace metals: new definitions based on new paradigms. Nutr Rev. 1993;51:287–295.
- Zaky R, Fekri A. Solvent-free mechanochemical synthesis of Zn(II), Cd(II), and Cu(II) complexes with 1-(4-methoxyphenyl)-4-(2-(1-(pyridin-2-yl)ethylidene)hydrazinyl)-1H-pyrrole-3-carbonitrile. Green Process Synth. 2017;12–23.
- Zaky R, Fekri A. Solid state ball milling as a green approach to prepare Cu(II) complexes: structural, spectral, DFT, and DNA studies. New J Chem. 2017;41:4555–4563.
- Zhao Y, Geng WT, Freeman AJ, et al. Structural, electronic, and magnetic properties of α- and β-MnAs: LDA and GGA investigations. Phys Rev B. 2002;65:11–15.
- Modeling and simulation solutions for chemicals and materials research. Materials Studio (Version 5.0). San Diego, USA: Accelrys software Inc.; 2009. Available from: www.accelrys.com
- Matveev A, Staufer M, Mayer M, et al. Density functional study of small molecules and transition‐metal carbonyls using revised PBE functional. Int J Quantu Chem. 1999;75:863–873. .
- Balouiri M, Sadiki M. Ibnsouda SK Methods forin vitro evaluating antimicrobial activity: a review. J Pharm Anal. 2016;6:71–79.
- Greenwood D, Slack R, Peutherer J. Medical Microbiology: a guide to microbial infections: pathogenesis, immunity, laboratory diagnosis and control. 15th ed. 1997, Churchill Livingstone, London.
- Ali AM, Ismail NH, Mackeen MM, et al. Antiviral, cytotoxic and antimicrobial activities of anthraquinones isolated from the roots of morinda elliptica. Pharm Biol. 2000;38:298–301.
- Abu El-Reash GM, Zaky RR, El-Gamil MM, et al. Synthesis, characterization, computational, conductometric titration and DNA binding studies of N′ 1, N′ 2-bis (3-hydroxy-5,5-dimethylcyclohex-2-en-1-ylidene) oxalohydrazide complexes. J Mol Liq. 2019;288:111030.
- Corona-Bustamante A, Viveros-Paredes JM, Flores-Parra A, et al. Antioxidant activity of butyl- and phenylstannoxanes derived from 2-, 3- and 4-pyridinecarboxylic acids. Molecules. 2010;15:5445–5459.
- Sedaghat T, Naseh M, Khavasi HR, et al. Synthesis, spectroscopic investigations, crystal structures and antibacterial activity of 3-(3-hydroxypyridin-2-ylamino)-1-phenylbut-2-en-1-one and its diorganotin(IV) complexesedi. Polyhedron. 2012;33:435–440.
- Cotton FA, Wilkinson G, Murillo CA, et al. Adv Inorg Chem. 6th ed. John Wiley & Sons Inc; 2003, NJ USA.
- Fahem AA. Comparative studies of mononuclear Ni(II) and UO2(II) complexes having bifunctional coordinated groups: synthesis, thermal analysis, X-ray diffraction, surface morphology studies and biological evaluation. Spectrochim Acta Part A Mol Biomol Spectrosc. 2012;88:10–22.
- Shahrjerdi A, Davarani SSH, Najafi E, et al. Sonoelectrochemical synthesis of a new nano lead(II) complex with quinoline-2-carboxylic acid ligand: a precursor to produce pure phase nano-sized lead(II) oxide. Ultrason Sonochem. 2015;22:382–390.
- Shebl M. Synthesis, spectroscopic characterization and antimicrobial activity of binuclear metal complexes of a new asymmetrical Schiff base ligand: DNA binding affinity of copper (II) complexes. Spectrochim Acta Part A Mol Biomol Spectrosc. 2014;117:127–137.
- Rakha TH, Ibrahim KM, Khalifa MI. Thermochemical study of some transition metal complexes of isonicotinic hydrazide derivatives. Thermochim Acta. 1989;144:53–63.
- Zaky RR, Yousef TA, Abdelghany AM. Computational studies of the first order kinetic reactions for mononuclear copper (II) complexes having a hard–soft NS donor ligand. Spectrochim. Acta Part A Mol Biomol Spectrosc. 2014;130:178–187.
- Coats AW, Redfern JP. Kinetic parameters from thermogravimetric data. Nature. 1964;201:68–69.
- Horowitz HH, Metzger G. A new analysis of thermogravimetric traces. Anal Chem. 1963;35:1464–1468.
- El-Gammal OA, Abu El-Reash GM, Yousef TA, et al. Synthesis, spectral characterization, computational calculations and biological activity of complexes designed from NNO donor Schiff-base ligand. Spectrochim Acta Part A Mol Biomol Spectrosc. 2015;146:163–178.
- Liu L, Alam MS, Lee DU. Synthesis, antioxidant activity and fluorescence properties of novel europium complexes with (E)-2- or 4-hydroxy-N’-[(2-hydroxynaphthalen-1-yl)methylene]benzohydrazide Schiff Base. Bull Korean Chem Soc. 2012;33:3361–3367.
- Da REP, Rodrigues HA, Da Cunha EFF, et al. Probing kinetic and thermodynamic parameters as well as solvent and substituent effects on spectroscopic probes of 2-amino-1,4-naphthoquinone derivatives. Comput Theor Chem. 2016;1096:17–26.
- Da Silva RR, Ramalho TC, Santos JM, Figueroa-Villar JD. On the limits of highest-occupied molecular orbital driven reactions: the frontier effective-for-reaction molecular orbital concept. J Phys Chem A. 2006;110:1031–1040.
- Gabrielska J, Soczynska-Kordala M, Hladyszowski J, et al. Antioxidative effect of quercetin and its equimolar mixtures with phenyltin compounds on liposome membranes. J Agric Food Chem. 2006;54:7735–7746.
- Bukhari SB, Memon S, Tahir MM, et al. Synthesis, characterization and investigation of antioxidant activity of cobalt–quercetin complex. J Mol Struct. 2008;892:39–46.
- Chen W, Sun S, Cao W, et al. Antioxidant property of quercetin–Cr(III) complex: the role of Cr(III) ion. J Mol Struct. 2009;918:194–197.
- Zaky RR, Al-dawood AY. Chelation behavior of N′-(4-(dimethylamino)benzylidene) −2-oxo-2H-chromene-3-carbohydrazide towards Cd(П), Zn(П), Ni(П), Hg(П), Cu(П) and Co(П) metal ions in presence of SiO2. Silicon. 2020;12:1259–1277.
- Ong W, Kaifer AE. Unusual electrochemical properties of unsymmetric viologen dendrimers. J Am Chem Soc. 2002;124:9358–9359.
- Bamford CH, Compton RG, Thomas JDR. Electrode kinetics: principles and methodology (Comprehensive chemical kinetics. Vol. 26. Amsterdam: Elsevier; 1986.
- Gosser DK. Cyclic voltammetry: simulation and analysis of reaction mechanisms. New York: VCH; 1993.
- Hidalgo-Acosta JC, Climent V, Suárez-Herrera MF, et al. Enhanced electrochemical reversibility of ultrathin aniline oligomer films grown on Pt (111). Electrochem Commun. 2011;13:1304–1308.
- Brownson DAC, Banks CE. The handbook of graphene electrochemistry. Springer; 2014, Manchester UK.
- Shaikh AA, Begum M, Khan AH, et al. Cyclic voltametric studies of the redox behavior of iron (III)-vitamin B 6 complex at carbon paste electrode. Russ J Electrochem. 2006;42:691–697.
- Zaky RR, Yousef TA, Ibrahim KM. Co(II), Cd(II), Hg(II) and U(VI)O2 complexes of o-hydroxyacetophenone [N-(3-hydroxy-2-naphthoyl)] hydrazone: physicochemical study, thermal studies and antimicrobial activity. Spectrochim. Acta Part A Mol Biomol Spectrosc.. 2012;97:683–694. .