ABSTRACT
Aim: The aim of the current research work was to isolate, characterize and evaluate the hepatoprotective activity of Garuga pinnata leaves in both acute and chronic hepatic disorder.
Materials and methods: The ethanolic extract of the leaves was evaluated for phytochemical screening, acute oral toxicity studies and In-vivo hepatoprotective activity. Hepatotoxicity was induced by administration of paracetamol, thioacetamide and ethanol in albino wistar rats. Serum and tissue biochemical parameters were estimated. Histopathological studies were performed using hematoxylin and eosin staining. The active principle from plant was isolated using column chromatography and identified by UV, FTIR, NMR, and GC-MS studies.
Results: Animal groups treated with hepatotoxicant showed marked alteration in serum and tissue biochemical parameters. However, pretreatment with ethanolic extract significantly restored the biochemical parameters. Garuga pinnata leaves extract showed the dose-dependant hepatoprotective effect. Histopathology of the groups treated with leaves extract showed normal hepatic architecture confirming hepatoprotective activity. Analytical techniques including UV, FTIR, NMR and mass confirmed the presence of rutin flavonoids and hence the hepatoprotective activity was attributed to chemical compound rutin.
Conclusion: Ethanolic leaves extracts can serve as promising herbal medicine for the treatment of both acute and chronic hepatotoxicity due to the presence of flavonoids rutin.
Graphical abstract
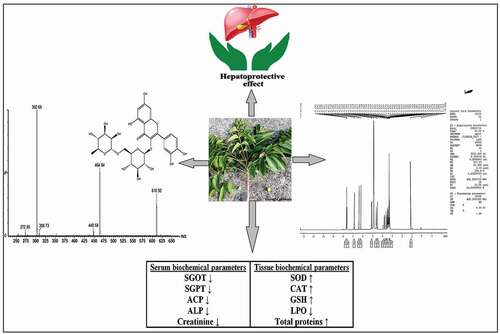
Introduction
The acute and chronic liver diseases, and cirrhosis is one of the major causes of morbidity and mortality worldwide. However, the burden and primary factors differ across regions and population groups [Citation1,Citation2]. Per year, about 25,000 deaths occur due to liver diseases, and maximum around 62.6% of global deaths occurs in the Asia-Pacific region [Citation3,Citation4]. Hepatocellular carcinoma contributes to 72.7% of global deaths, and more than two-thirds of the global load of acute viral hepatitis occurred in this area, among these cirrhosis leads to 54.3% of global deaths [Citation5,Citation6]. Thus, liver disorders are a significant health problem and are commonly associated with oxidative stress, tissue injury, fibrosis and inflammation [Citation1,Citation7].
The liver damage mainly caused by the chronic use of alcohol, chemicals (carbon tetrachloride, paracetamol, rifampicin, ethanol, notrosamines, thioacetamide) and infections [Citation8]. PCM is most commonly used analgesic antipyretic drug and its widespread use in various disease treatment resulted severe hepatic injury. In addition, toxic doses of PCM could cause alteration in the morphology and function of liver mitochondria [Citation9]. TAA is an organosulfur, white crystalline compound having liver damaging and carcinogenic activity by causing cytomegaly [Citation10]. Alcohol is a psychoactive substance metabolized mainly by the liver and its chronic consumption is associated with hepatotoxicity [Citation11]. Thus, substances like TAA, PCM and ethanol are used to induce liver injury in rats to study the hepatoprotective activity of different drugs.
Therefore, there is an unmet need to use suitable treatment strategies to treat liver diseases successfully. Nowadays, herbal medicines are playing an important role in protecting human health and enhancing the quality of human life [Citation12]. Many potent local herbal medicines are available in various parts of the world for the healing of liver diseases though most of them have not yet been clinically confirmed [Citation13]. The different chemical components such as phenols, coumarins, monoterpenes, glycosides, alkaloids and xanthenes are found in plants exhibiting hepatoprotective behavior [Citation14]. Moreover, these medicinal plants primarily produce phenolic substances that are flavonoids, which are liable for several biological acts, with the capacity to scavenge antioxidants and free radicals [Citation15]. Furthermore, varieties of plant products have long been used for the prevention of liver disease across the globe [Citation16].
G. pinnata Roxb [Citation17–19]. is a medium size plant widely distributed in India, Southeast Asia and few parts of the China. It belongs to the family of Burseraceae and found in mixed deciduous forests with ethnobotanical and ethanopharmacological background [Citation20,Citation21]. Traditionally, the plant has been used as stomachic, cooling agent, astringent, diuretic, tonic, aphrodisiac etc. Besides, it is used for better management of ulcers, leprosy and vaginal complications. Moreover, it shows wide pharmacological, antimicrobial, antioxidant, antidiabetic, anticancer activities [Citation22–24].
The extensive literature review shows that the hepatoprotective potential of G. pinnata Roxb. has not been evaluated for experimental assessment. Recently, our research group has done preliminary study and proved the hepatoprotective activity of ethanolic extract of G. pinnata Roxb. leaves against CCl4-induced hepatotoxicity in rats and found very promising results [Citation25]. So, we are extending our research work to isolate, identify the active principle from leaves and to prove and confirm the hepatoprotective activity of ethanolic extract of G. pinnata Roxb. leaves in both acute and chronic hepatotoxicity using three different types of hepatotoxic animal model using paracetamol, ethanol and thioacetamide.
Materials and methods
The fresh leaves of the G. pinnata were collected from the local region of Katyayani, Kolhapur (District), Maharashtra, India and were identified and authenticated by the Botanical Survey of India, Pune (BSI/WRC/IDEN.CER./2018/H3–69) and the herbarium specimens SDC-01 and SDC-02 deposited at the same. Paracetamol (PCM), Thioacetamide (TAA), and Ethanol were obtained from Research Lab, Islampur (Sangli). Silymarin was obtained from Ranbaxy Laboratories Ltd. Baddi, H.P. India. Serum glutamic-pyruvic transaminase (SGPT), serum glutamic oxaloacetic transaminase (SGOT), alkaline phosphatase (ALP), acid phosphatase (ACP) and creatinine were estimated by using diagnostic kit obtained from Pathozyme Diagnostic Ltd., Kagal, Kolhapur and Medsource Ozone Biomedicals Pvt. Ltd. All reagents were of analytical grade.
Animal handlings and maintenance
Hepatoprotective activity was studied in Albino Wistar rats of either sex weighing 150–180 gm which were purchased from Crystal Biological Solutions, Uruli Devachi, Pune, Maharashtra India. All animal handlings were performed as per approved guidelines of the ‘Committee for the Purpose of Control and Supervision of Experiments on Animals’ (CPCSEA, India), and the protocol was approved by the Institutional Animal Ethical Committee, Rajarambapu College of Pharmacy, Kasegaon (1290/PO/Re/S/09/CPCSEA, Protocol No. RCP/P–18/18–19 Dated: 16/03/2019). All animals were housed in standard polypropylene cages (3 animals per cage) and were maintained under standard hygienic conditions at 25–28°C with 12 hr light/dark cycle and provided with standard pallet diet procured from Pranav Agro, Sangli and water and food was given ad libitum throughout the course of study.
Preparation of plant extract
The leaves of the plants were separated from stems and shade dried at room temperature until it becomes completely dry and finally made in powder form by pulverization with electric grinding machine. The dried powder was passed through 100 mesh sieve to get uniform size and then stored in sealed polythene bags. The powdered drug (100 g) was extracted using ethanol (500 ml) as solvent for the period of 6 hr in Soxhlet extractor followed by filtration using Whatman no. 1 filter paper. The ethanolic extract was concentrated to small volume by means of rotary evaporator and vacuum dryer. The plant yield was reported (5.1%) and deposited for further use at −20°C. The ethanolic extracts were further used for preliminary phytochemical evaluation and animal studies.
Phytochemical analysis
The ethanolic extract was used for preliminary phytochemical screening of alkaloids (Meyer’s tests), carbohydrates (Molish’s, Benedict’s and Fehling’s test) [Citation26], saponins (foam and froth tests) tannins (gelatin test), flavonoids (Alkaline reagent and lead acetate tests [Citation27]. The screening was done as per the standard methods including tests for alkaloids, carbohydrates, glycosides, flavonoids, saponins, steroids, triterpenoids, and phenolic compounds [Citation28].
Acute oral toxicity study
The acute oral toxicity study was carried out as per the set guidelines by Organization for Economic Cooperation and Development (OECD), received from Committee for the purpose of Control and Supervision of Experiments on Animals (CPCSEA), Ministry of Social Justice and Empowerment, Government of India. Female non-pregnant Albino Wistar rats were used for acute oral toxicity studies. The test substance was given orally by oral feeding tube. The animals were fasted before dosing. The animals were weighed during the fasting time, and the test substance was administered. Food was delayed for 3–4 h in rats after the dosage was delivered. Since dosing, the animals were monitored at least daily for the first 30 min, then frequently for the first 24 h. Additional observations like changes in skin, fur, eyes and mucous membranes, respiratory, circulatory, autonomic central nervous systems, somatomotor activity and behavior pattern. Attention was also given to the observation of tremors and convulsions. All observations lasted for a cumulative period of 14 days [Citation29,Citation30].
In vivo hepatoprotective activity in acute and chronic hepatotoxic model
Acute and chronic hepatotoxic models were developed by using the administration of PCM, TAA and ethanol in Albino Wistar rats, respectively.
Thioacetamide induced acute hepatotoxic model
All animals were divided into five groups, each group consisting of six animals. Group I (control group) received the vehicle viz. normal saline (2 ml/kg). Group II received TAA 50 mg/kg bw s.c., every 72 h for 21 days. Group III received standard drug (Silymarin 100 mg/kg p.o.) [Citation31] for 21 days and simultaneously administered TAA 50 mg/kg bw s.c. 1 h after the respective assigned treatments every 72 h. Groups IV–V received plant extract (200 mg/kg and 400 mg/kg p.o.) for 21 days and simultaneously administered TAA 50 mg/kg bw s.c. every 72 h.
Paracetamol induced acute hepatotoxic model
Animals, after acclimatization (6–7 days) in the animal quarters, were randomly divided into five groups of six animals each. Group I (Normal control) animals received distilled water for 7 days. Group II (Positive control) animals were induced hepatotoxicity by using PCM (3 g/kg p.o.). The animals received distilled water for 7 days and given PCM single dose, orally on day 8. Groups III animals received pretreatment with Standard Drug (silymarin 100 mg/kg p.o.) for 7 days followed by a single dose of PCM on day 8. Groups IV and V animals were pretreated with drug extract for 7 days (200 mg/kg and 400 mg/kg p.o) followed by a single dose of PCM on day 8.
Ethanol induced chronic hepatotoxic model
Animals, after acclimatization (6–7 days) in the animal quarters, were randomly divided into five groups of six animals each. Group I served as normal control and received distilled water orally once daily for 28 days. Group II served as toxicant control and received ethanol (5 g/kg, 20% w/v p.o.) once daily for 21 days from day 8 to day 28. Group III animals received standard drug (silymarin 100 mg/kg) p.o. daily for 28 days and ethanol (5 g/kg, 20% w/v p.o.) daily for 21 days from day 8 to day 28. Groups IV and V received plant extract (200 mg/kg and 400 mg/kg p.o.) daily for 28 days and ethanol (5 g/kg, 20% w/v p.o.) daily for 21 days from day 8 to day 28.
After 48 h, following PCM, TAA and ethanol administration, blood samples were collected from all groups of animals by the heart puncture/ puncturing the retro-orbital plexus. Serum was separated by centrifugation at 4000 rpm at 4°C for 15 min and analyzed for various biochemical parameters. Afterward, the rats were sacrificed under light ether anesthesia. The liver from all the animals were immediately removed and small piece was fixed in 10% formalin for histopathological assessment.
Serum biochemical parameter estimation
The biochemical parameter levels of liver-specific marker enzymes were estimated by reported methods viz, SGOT and SGPT by Modified Reitman & Frankel’s method [Citation32], ALP by Modified Kind & King’s method [Citation33], ACP by Modified King’s method [Citation34] and creatinine by Modified Jaff’s Method [Citation35]. Enzyme activities were measured by using the diagnostic strips and were read on colorimeter.
Tissue biochemical parameter estimation
Tissue biochemical parameter such as lipid peroxidation (LPO) was determined by measuring malondialdehyde (MDA) by Ohkawa’s method [Citation36]. Glutathione was measured by its reaction with 5, 5ʹ-dithiobis (2-nitrobenzoic acid) DTNB by Ellman’s method [Citation37,Citation38]. Catalase by Aebi’s method [Citation39] and superoxide dismutase was determined by Marklund’s method [Citation40] and the total protein content was measured by Lowry’s method [Citation41].
Histopathological studies
Liver tissue was collected and fixed in 10% buffered formalin for histopathological analysis. Liver tissue sections were taken with the help of microtome and stained with hematoxylin and eosin. The sections were observed under electronic microscope [Citation42].
Isolation and identification of phytoconstituents from G. pinnata
Nearly, 200 gm of dried leaves powder was macerated with acetone: water (7:3) for the period of 3 days followed by filtration and concentration of filtrate. This concentrated filtrate was extracted three times with dichloromethane solvent. This fraction was discarded and again extracted with acetone: water system three times with ethyl acetate solvent. The acetone: water fraction was tested for flavonoids tests. Ethyl acetate extract concentrated and evaporated to dryness in water bath (crude ethyl acetate fraction). Ethanol extract of G. pinnata containing flavonoids with potent hepatoprotective activity against chemically mediated liver damage is subjected to chromatography for the separation and isolation of flavonoids.
Characterization of active principle from G. pinnata
UV spectrum
The five fractions obtained by column chromatography were subjected to UV analysis after proper dilutions. The UV spectrum was performed by using Jasco UV spectrometer.
IR study
The yellow band isolated from column chromatography was taken; the solvent was evaporated and dried in desiccators so as to get the dry powder. The dried compound mixed with KBr and pellet was prepared using KBr press and an IR spectrum was obtained. The analysis was carried out by using Thermo Nicolet, Avatar 370 at SAIF, Cochin.
NMR study of isolated compound from G. pinnata
The isolated, dried compound was subjected to NMR spectroscopic analysis. The compound was solubilized in DMSO and the spectrum was obtained at 400 MHz. The NMR was performed at SAIF, Cochin. The instrument Bruker model AV 400 MHz was used for analysis.
Mass analysis of isolated compound from G. pinnata by GC-MS
GC-MS analysis of the ethanol extract of G. pinnata was done using a Perkin–Elmer GC Clarus 500 system comprising an AOC-20i auto-sampler and a Gas Chromatograph interfaced to a Mass Spectrometer (GC-MS) equipped with a Elite-5 MS (5% diphenyl/95% dimethyl poly siloxane) fused a capillary column (30 × 0.25 µm ID × 0.25 µm df). For GC-MS detection, an electron ionization system was operated in electron impact mode with ionization energy of 70 eV. Helium gas (99.999%) was used as a carrier gas at a constant flow rate of 1 ml/ min, and an injection volume of 2 µl was used.
Results and discussion
Phytochemical analysis of G. pinnata
The phytochemical analysis of ethanolic extract confirmed the presence of alkaloids, sterols, triterpenoids, glycosides, flavonoids, and tannins in varying amount. Proteins, carbohydrates and saponins were found to be absent in all the extracts. Previous studies also revealed the presence of several triterpenoids, flavonoids and glycosides etc., through phytochemical screening of this plant [Citation21,Citation24]. The percentage of extraction yield was found to be 18%. The loss on drying was 0.03 g, total ash of 0.23, water soluble extractive value of 4.5%, alcohol soluble extractive value of 1.25% (for 4 g), acid insoluble ash of 0 g, and water insoluble ash of 0.1 g was observed.
Acute toxicity study
Acute toxicity study was carried out to determine the safe dose for pharmacological studies. The limit test at a dose of 2000 mg/kg body weight was performed for ethanolic extracts. Oral administration at this dose level did not show any sign of behavioral toxicity, neurological toxicity or mortality. Also all animals were alive, active and healthy during the observation period hence, 1/5th and 1/10th dose of the maximum tolerated dose was selected for the efficacy studies. Based on results obtained, the 200 and 400 mg/kg body weight was considered as safe dose for In-vivo pharmacological studies.
Serum biochemical parameter estimation
The change in serum biochemical parameters in PCM, TAA and ethanol-induced acute and chronic hepatotoxicity is presented in , and , respectively. PCM and TAA are well-known chemical agents responsible for the development of acute hepatotoxicity at higher doses and widely used in experimental animals [Citation43,Citation44]. Similarly, ethanol at very higher doses leads to chronic cellular as well as tissue necrosis and abnormalities and causes cellular leakage of enzymes and other clinical markers so it is widely used in induction of chronic hepatotoxicity in animal models [Citation45,Citation46]. Administration of PCM (3 g/kg), TAA (50 mg/kg) and ethanol (5 g/kg, 20% w/v p.o.) orally, induced marked increase in the serum biochemical markers (SGOT, SGPT, ACP and ALP) level compared with normal control. Similar observations related to rise in such biochemical markers were observed by various groups of researchers [Citation38,Citation47,Citation48]. As an indicative of hepatotoxicity, significant (P < 0.01) increase in the levels of SGOT, SGPT, ACP, ALP and creatinine in the group treated with PCM, TAA and ethanol was observed, and it was compared with normal control group. The observation of changes in serum biochemical parameters like SGOT, SGPT, ACP and ALP clearly indicated the occurrence of hepatotoxicity upon exposure of PCM, TAA and ethanol. The hepatotoxicity was caused due to the damage of liver cells, tissues or might be due to the fact of increased cellular permeability leading to the appearance of these markers in serum [Citation49]. Thus, marked rise in serum levels of biochemical parameters confirms the induction of acute and chronic hepatotoxicity in experimental animals. Serum biochemical parameters like SGOT, SGPT, ACP, ALP and creatinine levels of animals who received EEGP at a dose of 200 mg/kg and 400 mg/kg extracts were significantly decreased (P < 0.01) as comparable with the standard group, treated with Silymarin (, and ). Previously our research group conducted the study with G. pinnata and found similar observations in CCl4-induced hepatotoxicity model [Citation25]. This effect is in agreement with the commonly accepted view that serum levels of transaminase and other biochemical markers return to normal with the healing of hepatic parenchyma and regeneration of hepatocytes [Citation35]. These findings of the normalization of different biochemical markers serve as excellent proof of the hepatoprotective ability of G. pinnata in both acute and chronic hepatotoxicity.
Table 1. Effects of ethanolic extract of Garuga pinnata on blood biochemical parameters by Paracetamol induced hepatotoxicity (SGOT, SGPT, ACP, ALP and creatinine)
Table 2. Effects of ethanolic extract of Garuga pinnata on blood biochemical parameters by Thioacetamide induced hepatotoxicity (SGOT, SGPT, ACP, ALP and creatinine)
Table 3. Effects of ethanolic extract of Garuga pinnata on blood biochemical parameters by Ethanol induced hepatotoxicity (SGOT, SGPT, ACP, ALP and creatinine)
Tissue biochemical parameter estimation
The change in tissue biochemical parameters in PCM, TAA and ethanol-induced acute and chronic hepatotoxicities are presented in , respectively. Administration of PCM (3 g/kg), TAA (50 mg/kg) and ethanol (5 g/kg, 20% w/v p.o.) orally, induced marked alteration in the tissue biochemical parameters level compared with normal control. As an indicative of hepatotoxicity, significant (P < 0.01) decrease in total serum protein, SOD and GSH was observed. In addition, there were subsequently increase (P < 0.01) in the levels of CAT and lipid peroxidation (LPO) in the group treated with PCM, TAA and ethanol compared with normal control. The pre-treatment of EEGP extracts at dose levels of 200 and 400 mg/kg body weight significantly controlled the change in the serum and tissue biochemical parameters in dose dependant manner. All the extracts at dose levels of 400 mg/kg exhibited significant activity compared to lower doses. Pretreatment of EEGP extract significantly increased (P < 0.01) total proteins, SOD and GSH levels as compared to toxic control group and the effect as comparable with the standard group, treated with Silymarin, simultaneously CAT and LPO levels were significantly (P < 0.01) decreased as observed in tissue biochemical estimations. In current research, the rise in MDA levels indicates increased LPO leading to tissue damage and free radical development as a result of antioxidant defense mechanism failure. LPO biomembrane depletion impacts the liver’s antioxidant function, and is a significant source of liver damage [Citation50]. CAT, SOD and GSH are responsible for protecting the liver from harmful and toxic effects of reactive oxygen species (ROS), free radical scavengers and chain reaction terminators [Citation51]. But it has been observed that administration of PCM, TAA and ethanol resulted in to decrease in the activity of CAT, SOD and GSH in livers leading to the increase in concentration of ROS, free radicals leading to the hepatic cell injury [Citation52]. In contrast to this, the administration of ethanolic extract of the plant, increased the activity of CAT, SOD and GSH and their by decreased the levels of LPO in both acute and chronic hepatotoxic rat models. The hepatoprotective activity of the extract might be due to the antioxidant potential of flavonoids and phenolic compounds present in ethanolic extracts. In our previous study, similar results were observed in CCl4-induced hepatotoxic rat models [Citation25]. The hepatoprotective potential of G. pinnata in both acute and chronic hepatotoxicity is strengthened by these results associated with increased CAT, SOD and GSH activity.
Table 4. Effects of ethanol extract of Garuga pinnata on tissue biochemical estimations by Paracetamol induced hepatotoxicity (SOD, CAT, GSH, LPO and Total proteins)
Table 5. Effects of ethanol extract of Garuga pinnata on tissue biochemical estimations by Thioacetamide induced hepatotoxicity (SOD, CAT, GSH, LPO and total proteins)
Table 6. Effects of ethanol extract of Garuga pinnata on tissue biochemical estimations by Ethanol induced hepatotoxicity (SOD, CAT, GSH, LPO and total proteins)
Histopathological studies
In addition, the hepatoprotective role of EEGP extracts on hepatotoxicity-induced PCM, TAA and ethanol model was verified by contrasting the histopathology of extracts treated with usual regulated liver tissue, toxic control and typical drug-treated animals. The histological characteristics of liver tissues in normal group showed well managed architecture without any damage to hepatocytes (). The histological architecture of the liver segment treated with PCM, TAA and ethanol revealed massive fatty modifications, necrosis and cellular boundary degradation (). EEGP (200 and 400 mg/kg) extracts administered at higher doses effectively normalized these defects () approximately to the extent of the Silymarin treated groups in the histological architecture of the liver, indicating their strong hepatoprotective capacity (). There was a microvasular fat improvement in the regulation of ethanol. The group treated with EEGP extract and Silymarin () showed less microvasular fatty changes, and their structures were regular, suggesting that hepatoprotection was greater for these extracts than for other extracts.
Figure 1. Histopathology of PCM induced hepatotoxicity model; (1A) Normal control group- Showing well defined liver architecture with normal hepatic cells without any damage, (1B) PCM Treated group- showed the degenerative hepatocytes with necrosis causing severe degree of liver damage, (1 C) Standard silymarin (100 mg/kg) treatment group- showed the mild degree of liver damage and restoring hepatocytes, (1D and 1E) Group treated with Garuga pinnata (200 mg/kg and 400 mg/kg) showed the regeneration of liver cells with mild degree of liver damage
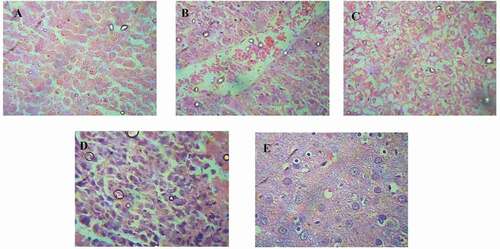
Figure 2. Histopathology of TAA induced hepatotoxicity model; (2A) Normal control group- Showing well defined liver architecture with normal hepatic cells with conserved cytoplasm, (2B) TAA Treated group- showed the degenerative hepatocytes with necrosis causing severe degree of liver damage, (2 C) Standard silymarin (100 mg/kg) treatment group- showed the mild degree of liver damage and restoring hepatocytes, (2D and 2E) group treated with Garuga pinnata (200 mg/kg and 400 mg/kg) showed the regeneration of liver cells with mild degree of liver damage
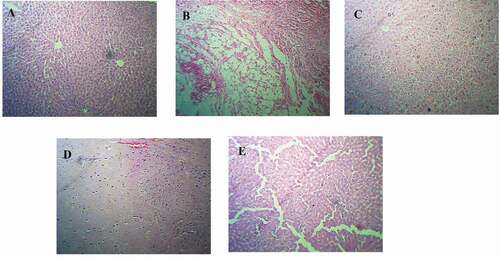
Figure 3. Histopathology of Ethanol induced hepatotoxicity model; (3A) Normal control group- showing well defined liver architecture with normal hepatic cells with conserved cytoplasm, (3B) ethanol treated group- showed the degenerative hepatocytes with necrosis causing severe degree of liver damage, (3 C) standard silymarin (100 mg/kg) treatment group- showed the mild degree of liver damage and restoring hepatocytes, (3D and 3E) group treated with Garuga pinnata (200 mg/kg and 400 mg/kg) showed the regeneration of liver cells with mild degree of liver damage
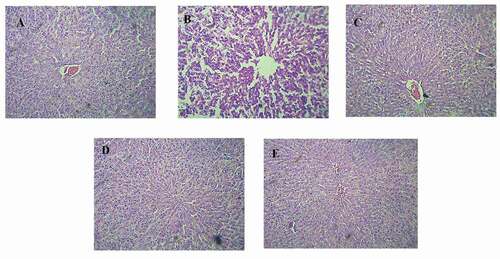
Mixed hepatic cellular injury may be due to the organ disruption and elevated serum enzyme levels. Increased serum levels of SGOT, SGPT, ACP, ALP and creatinine revealed hepatotoxicity as a mixed hepatic cell form injury in the present research. But G. pinnata ethanolic extracts reduced serum enzyme levels, indicating that they were hepatocytes-defensive and maintained normal liver physiology, further causing plasma membrane stabilization and regeneration of damaged liver cells by restoring hepatic parenchymal cells and normalizing the permeability of the altered cells as shown in both acute and chronic hepatotoxic models. In this way, the ethanolic extract of G. pinnata showed hepatoprotective activity, which was confirmed by histopathological observations.
Identification and characterization of active phytoconstituents
The isolated compound form the Garuga pinnta was subjected to UV spectral analysis, and it showed characteristic bands in chloroform at λ = 251.The UV spectra of standard showed one characteristic peak at λ = 271.As compared with standard it was found that compound belongs to the group of flavonoids.
Similarly, the structure of the isolated compound was elucidated by Fourier Transformed-Infra Red (FT-IR) spectrophotometer in KBr pellet method (Shimadzu-8400S). The FTIR spectra () of the isolated compound showed principal peaks at 3347.03 cm−1 corresponding to the OH stretching, 2937.85 cm−1 corresponding to the CH2 stretching, 1203.34 cm−1 corresponding to the C-O stretching, 1655.79 cm−1 corresponding to the – C = O stretching, 1503.89 cm−1 corresponding to the C = C aromatic ring. All these characteristic peaks correspond to the flavonoids [Citation53].
Figure 4. Characteristic UV peak of isolated compound at 251 nm indicating the compound belonging to flavonoids group
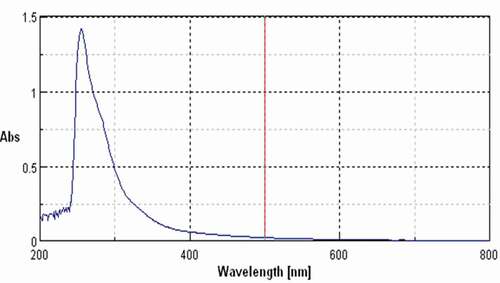
Further, structure of the isolated compound was elucidated by FT-NMR (BRUKER AMX 400 MHZ) analysis. The sample was dissolved in DMSO-d6 and value is measured in δ ppm. δ ppm values and the corresponding functional group are summarized in , and NMR spectra of the of isolated compound obtained from ethyl acetate fraction of G. pinnata is shown in .
The 1H-NMR revealed the chemical shifts of protons essentially identical with those reported in the literature for Rutin.
Table 7. Functional group characterization using δ ppm of ethyl acetate fraction of Garuga pinnata.
The structure of isolated compound was elucidated by GC-MS Spectrum (). The parent ions of isolated compound were observed at m/z 610.92 and production ion peaks were observed at m/z 302.69 and 464.84. The multiple reactions monitoring mass transition of rutin was at m/z 609.4→300.4 with collision energy of 70 eV. The reported parent ions of the rutin were found at m/z 609.4 and production ion peak at m/z 300.4. These observations clearly indicated the isolated compound as rutin.
The analytical techniques including UV, FTIR, NMR and GC-MS confirmed the presence of rutin as flavonoids a principle phytochemical in G. pinnata extract. Rutin is a flavonol abundantly present in plants such as passion flower, buckwheat, tea, and apple, and its chemical name is (3,3ʹ,4ʹ,5,7-pentahydroxyflavone-3-rhamnoglucoside). Chemically, it is a glycoside along with disaccharide rutinose, flavonolic aglycone quercetin. A variety of pharmacological behaviors have been shown, including antioxidant, cytoprotective, vasoprotective, anticarcinogenic, neuroprotective and cardio protective and hepatoprotective activities [Citation54]. Rutin is commonly studied in laboratory animals for hepatoprotective function. The protective role of rutin in carbon tetrachloride (CCl4)-induced liver injuries in rats was tested by [Citation55,Citation56]. Rutin administration resulted in reductions in serum levels of alanine aminotransferase, aspartate aminotransferase, alkaline phosphatase and gamma-glutamyl transpeptidase due to carbon tetrachloride elevation. Thus, current study demonstrated PCM, TAA and ethanol-induced acute and chronic hepatotoxicity in albino wistar rats was significantly treated by using ethanolic extracts of G. pinnata leaves due to presence rutin as antioxidant, hepatoprotective flavonoids constituent.
Conclusion
Ethanolic extract of G. pinnata exhibited great hepatoprotective activity against paracetamol, thioacetamide and ethanol-induced hepatotoxicity and showed significant decrease in SGOT, SGPT, ACP, ALP, Creatinine, LPO and significant increase in total proteins and protective enzymes such as SOD, CAT, GSH and restoration of histopathological structure of liver; damaged and hence can be used in the treatment of both acute and chronic hepatotoxicity. The hepatoprotective activity was attributed due to the presence of rutin which was confirmed by various analytical techniques.
Highlights
G. pinnata is well known traditional plant known for its various pharmacological properties and used as folk medicine in India and other countries
First report to prove the hepatoprotective activity in both acute and chronic hepatotoxicity.
Excellent hepatoprotective potential due to presence of flavonoids i.e. rutin confirmed by NMR and Mass spectroscopy.
Ethanolic leaves extracts as promising herbal medicine for the treatment of both acute and chronic hepatotoxicity due to the presence of flavonoids rutin.
Abbreviations
SGOT: Serum Glutamate Oxaloacetate Transaminase, SGPT: Serum Glutamate Pyruvate Transaminase, ALP: Alkaline Phosphatase, ACP: Acid Phosphatase, SOD: Superoxide dismutase CAT: Catalase, LPO: Lipid peroxidation, GSH: Glutathione, EEGP: Ethanolic extract of Garuga pinnata, PCM: Paracetamol, TAA: Thioacetamide.
Disclosure statement
No potential conflict of interest was reported by the author(s).
References
- Mohamed J, Nafizah AN, Zariyantey AH, et al. Mechanisms of diabetes-induced liver damage: the role of oxidative stress and inflammation. Sultan Qaboos Univ Med J. 2016;16(2):132–141.
- Younossi Z, Tacke F, Arrese M, et al. Global perspectives on nonalcoholic fatty liver disease and nonalcoholic steatohepatitis. Hepatology. 2019;69(6):2672–2682. .
- Ceresa CD, Nasralla D, Coussios CC, et al. The case for normothermic machine perfusion in liver transplantation. Liver Transpl. 2018;24(2):269–275.
- Eslam M, Sarin SK, Wong VWS, et al. The Asian Pacific Association for the Study of the Liver clinical practice guidelines for the diagnosis and management of metabolic associated fatty liver disease. Hepatol Int. 2020;14:1–31.
- Wu TJ, Chan KM, Chou HS, et al. Liver transplantation in patients with hepatitis B virus-related hepatocellular carcinoma: the influence of viral characteristics on clinical outcome. Ann Surg Oncol. 2013;20(11):3582–3590. .
- Asrani SK, Devarbhavi H, Eaton J, et al. Burden of liver diseases in the world. J Hepatol. 2019;70(1):151–171.
- Cichoz-Lach H, Michalak A. Oxidative stress as a crucial factor in liver diseases. World J Gastroenterol. 2014;20(25):8082–8091.
- Zhang Q, Hu X, Hui F, et al. Ethanol extract and its dichloromethane fraction of Alpinia oxyphylla Miquel exhibited hepatoprotective effects against CCl4-induced oxidative damage in vitro and In vivo with the involvement of Nrf2. Biomed Pharmacother. 2017;91:812–822.
- Shanmugam S, Thangaraj P, BdS L, et al. Effects of luteolin and quercetin 3-b-D-glucoside identified from Passiflora subpeltata leaves against acetaminophen induced hepatotoxicity in rats. Biomed Pharmacother. 2016;83:1278–1285.
- Zargar S, Wani TA, Alamro AA, et al. Amelioration of thioacetamide-induced liver toxicity in Wistar rats by rutin. Int J Immunopathol Pharmacol. 2017;30(3):207–214.
- Rabelo ACS, KdP L, Araujo CM, et al. Baccharis trimera protects against ethanol induced hepatotoxicity In-vitro and In-vivo. J Ethnopharmacol. 2018;215:1–13.
- Gurib-Fakim A. Medicinal plants: traditions of yesterday and drugs of tomorrow. Mol Aspects Med. 2006;27(1):1–93.
- Rajaratnam M, Prystupa A, Lachowska-Kotowska P, et al. Herbal medicine for treatment and prevention of liver diseases. J Pre Clin Clin Res. 2014;8(2):55–60.
- Youssef FS, Ashour ML, Sobeh M, et al. Eremophila maculata—Isolation of a rare naturally-occurring lignan glycoside and the hepatoprotective activity of the leaf extract. Phytomedicine. 2016;23(12):1484–1493.
- Mukherjee PK, Sahoo AK, Narayanan N, et al. Lead finding from medicinal plants with hepatoprotective potentials. Expert Opin Drug Discov. 2009;4(5):545–576.
- Langmead L, Rampton DS. Herbal treatment in gastrointestinal and liver disease- benefits and dangers. Aliment Pharmacol Ther. 2001;15(9):1239–1252.
- Warrier PK, Nambiar VPK, Ramankutty C. Indian medicinal plants a compendium of 500 species. Chennai: Orient Longman; 2004.
- Khare CP. Indian medicinal plants: an illustrated dictionary. Verlag Berlin: Springer; 2007.
- Nadkarni KM, Nadkarni AK. Indian materia medica: with Ayurvedic, Unani-Tibbi, Siddha, allopathic, homeopathic, naturopathic & home remedies, appendices & indexes. In: Popular Prakashan, Bombay. 1955. p. 570.
- Shirwaikar A, Rajendran K, Barik R. Effect of aqueous bark extract of Garuga pinnata Roxb. in streptozotocin-nicotinamide induced type-II diabetes mellitus. J Ethnopharmacol. 2006;107(2):285–290.
- Sachan K, Singh PK, Kansal A, et al. Evaluation of anti-ulcer activity of hydroalchoholic stem bark extract of Garuga pinnata Roxb. J Pharm Chem Biol Sci. 2014;1(1):33–38.
- Thupurani MK, Reddy PN, Thirupathaiah A, et al. In vitro determination of anti-oxidant activities of Garuga pinnata Roxb. Int J Med Aro Plants. 2012;2(4):566–572.
- Thupurani MK, Reddy PN, Charya MAS, et al. In-vitro and In-vivo determination of anti-inflammatory activities of Garuga pinnata Roxb. Int J Pharm Sci Rev Res. 2013;22(2):310–314.
- Kulsum ARA, Mohammad AK, Mohammad SR, et al. Antimicrobial Constituents from Garuga pinnata Roxb. Lat Am J Pharm. 2012;31(7):1071–1073.
- Chavan S, Dias R, Magdum C. Evaluation of hepatoprotective activity of ethanolic extract of Garuga pinnata Roxburgh leaves against CCl4 induced hepatotoxicity in rats. Res J Pharm and Technol. 2021;14(5):2375–2380.
- Osborne TB, Harris IF. The carbohydrate group in the protein molecule. J Am Chem Soc. 1903;25(5):474–478.
- Ganesh P, Kumar RS, Saranraj P. Phytochemical analysis and antibacterial activity of Pepper (Piper nigrum L.) against some human pathogens. Eur J Exp Biol. 2014;3(2):36–41.
- Meckes M, Calzada F, Tortoriello J, et al. Terpenoids isolated from Psidium guajava hexane extract with depressant activity on central nervous system. Phytother Res. 1996;10(7):600–603.
- The organisation for economic co-operation and development. Guidelines on acute oral toxicity. Revised document. October 2005.
- Suneetha B, Prasad KVSRG, Nishanthi PD, et al. Hepatoprotective and antioxidant activity of methanolic extract of Actinodaphne madraspatana against carbon tetrachloride induced hepatotoxicity. Res J Pharmacogn Phytochem. 2014;6(4):176–180.
- Ghawate VB, Shrivastava P, Bhambar RS. Hepatoprotective activity of Bridelia retusa bark extracts against carbon tetrachloride-induced liver damage rats. Res J Pharmacogn Phytochem. 2017;9(2):121–124.
- Crowley LV. The Reitman-Frankel colorimetric transaminase procedure in suspected myocardial infarction. Clin Chem. 1967;13(6):482–487.
- King EJ, Abul-Fadl MAM, Walker PG. King-Armstrong phosphatase estimation by the determination of liberated phosphate. J Clin Pathol. 1951;4(1):85–91. .
- Hansen PW. A simplification of Kind and King’s method for determination of serum phosphatase. Scand J Clin Lab Inv. 1966;18(3):353–356.
- Vaishya R, Arora S, Singh B, et al. Modification of Jaffe’s kinetic method decreases bilirubin interference: a preliminary report. Indian J Clin Biochem. 2010;25(1):64–66.
- Ohkawa H, Ohishi N, Yagi K. Assay for lipid peroxides in animal tissues by thiobarbituric acid reaction. Anal Biochem. 1979;95(2):351–358.
- Davies MH, Birt DF, Schnell RC. Direct enzymatic assay for reduced and oxidized glutathione. J Pharmacol Methods. 1984;12(3):191–194.
- Kumar G, Banu GS, Pappa PV, et al. Hepatoprotective activity of Trianthema portulacastrum L. against paracetamol and thioacetamide intoxication in albino rats. J Ethnopharmacol. 2004;92(1):37–40.
- Catalase AH. In: Bergmeyer HU, editor. In Methods of enzymatic analysis. New York: Academic press. 1974. p. 673–684.
- Marklund SL. Human copper-containing superoxide dismutase of high molecular weight. Proc Natl Acad Sci U S A. 1982;79(24):7634–7638.
- Lowry OH, Rosebrough NJ, Farr AL, et al. Protein measurement with the Folin phenol reagent. J Biol Chem. 1951;193(1):265–275.
- Alqasoumi SI, Al-Howiriny TA, Abdel-Kader MS. Evaluation of the hepatoprotective effect of Aloe vera, Clematis hirsute, Cucumis prophetarum and bee propolis against experimentally induced liver injury in rats. Int J Pharmacol. 2008;4(3):213–217.
- Galal RM, Zaki HF, Seif ENMM, et al. Potential protective effect of honey against paracetamol-induced hepatotoxicity. Arch Iran Med. 2012;15(11):674–680.
- Sharma A, Verma AK, Kofron M, et al. Lipopolysaccharide reverses hepatic stellate cell activation via modulation of cMyb, SMADs and C/EBP transcription factors. Hepatology. 2020;72(5):1800–1818.
- Cui Y, Ye Q, Wang H, et al. Hepatoprotective potential of Aloe vera polysaccharides against chronic alcohol induced hepatotoxicity in mice. J Sci Food Agric. 2014;94(9):1764–1771.
- Song X, Yin S, Huo Y, et al. Glycycoumarin ameliorates alcohol-induced hepatotoxicity via activation of Nrf2 and autophagy. Free Radic Biol Med. 2015;89:135–146.
- Subramanian M, Balakrishnan S, Chinnaiyan SK, et al. Hepatoprotective effect of leaves of Morinda tinctoria Roxb. against paracetamol induced liver damage in rats. Drug Invent Today. 2013;5(3):223–228.
- Abou Seif HS. Ameliorative effect of pumpkin oil (Cucurbita pepo L.) against alcohol-induced hepatotoxicity and oxidative stress in albino rats. Beni-Seuf Univ J Appl Sci. 2014;3(3):178–185.
- Kuamwat RS, Mruthunjaya K, Gupta MK. Hepatoprotective effect of Gallic acid and Gallic acid Phytosome against Carbon Tetrachloride induced damage in albino rats. Res J Pharm Tech. 2012;5(5):677–681.
- Goel A, Dani V, Dhawan DK. Protective effects of zinc on lipid peroxidation, antioxidant enzymes and hepatic histoarchitecture in chlorpyrifos-induced toxicity. Chem Biol Interact. 2005;156(2–3):131–140.
- Patil SA, Joshi VG, Sambrekar SN, et al. Hepatoprotective and antioxidant potential of extracts from whole plant of solanum surattense burm. Res J Pharmacogn Phytochem. 2011;3(1):17–21.
- Wu YH, Zhang XM, Hu MH, et al. Effect of Laggera alata on hepatocytes damage induced by carbon tetrachloride In-vitro and In-vivo. J Ethnopharmacol. 2009;126(1):50–56.
- Oliveira RN, Mancini MC, Oliveira FCSD, et al. FTIR analysis and quantification of phenols and flavonoids of five commercially available plants extracts used in wound healing. Matéria (Rio J.). 2016;21(3):767–779.
- Kreft S, Knapp M, Kreft I. Extraction of rutin from buckwheat (Fagopyrum esculentum Moench) seeds and determination by capillary electrophoresis. J Agric Food Chem. 1999;47(11):4649–4652.
- Khan RA, Khan MR, Sahreen S. CCl4-induced hepatotoxicity: protective effect of rutin on p53, CYP2E1 and the antioxidative status in rat. BMC Complement Altern Med. 2012a;12(1):178–183.
- Khan RA, Khan MR, Sahreen S. Protective effects of rutin against potassium bromate induced nephrotoxicity in rats. BMC Complement Altern Med. 2012b;12(1):204–215.