ABSTRACT
Coragen 20 SC is a new insecticide that contains the active ingredient chlorantraniliprole, which has been approved for use against insects in many crops due to the presence of insect ryanodine receptors. The present study aims to evaluate the intrauterine exposure to coragen in pregnant rats and their fetuses from 6th to 20th day of gestation. The pregnant dams were orally administrated saline (control), 100 mg/kg (low dose) or 200 mg/kg (high dose) of coragen. The mothers of two treated groups showed teratogenic anomalies such as asymmetrical distribution of fetuses in the two uterine horns, as well as, observed resorption, bleeding in the uteri while, their fetuses had growth retardation, morphological malformations and some skeletal perversions in addition to some dead fetuses were recorded. Furthermore, multiple histopathological changes occurred and a significant DNA damage was induced by coragen in maternal and fetal liver and kidney tissues compared to the control. Gestational coragen treatment resulted in teratogenic and genotoxic effect on pregnant rats and their fetuses. The obtained results in the present study imply that women and their fetuses may have the same risk.
Introduction
Despite the potential adverse effects of pesticides on the living organisms including human and the environment, they are still widely used in agriculture due to their benefits to control insects-borne diseases, increase agricultural productivity and control of various pests [Citation1,Citation2]. Pesticides could be taken into the body through oral, inhalation or dermal routes after being ingested in food, drinking water, residential or occupational ways [Citation3]. The biological activity and severity of the pesticides’ toxicity impacts are determined by the type of chemical, the dose, the route and the period of exposure [Citation3]. There are numerous chemical classes of pesticides, which may be insecticide, fungicide or herbicide. Ryanodine is a toxic natural alkaloid isolated from plant Ryania speciose and is best used as insecticide [Citation4]. Chemically synthetic ryanodine compounds such as chlorantraniliprole, flubendiamide, cyantraniliprole, cyclaniliprole and tetraniliprole, are called diamide insecticides that opens muscular calcium channels [Citation5]. Chlorantraniliprole with 18.5% or 20% SC active ingredient in insecticide, has the trade name coragen, that we have investigated in our study. Coragen is being used to fight various types of flies and their larvae [Citation6–8].
It was reported that ryanoids are selectively targeting ryanodine receptors (RyRs) in the muscles of Lepidoptera and some Coleoptera, Diptera and Isoptera species [Citation9,Citation10]. Binding to ryanodine receptors resulting in an uncontrolled release of calcium from sarcoplasmic reticulum, leading to impaired regulation of muscle contraction, stop feeding, lethargy, muscle paralysis and death of the insect [Citation11].
Now it is well known that pesticide exposure during critical stages of development can have long-term negative consequences in early development and later life, where the developing brain and endocrine system are particularly vulnerable targets [Citation12].
It was thought that chlorantraniliprole is less toxic to non-target organisms including; mammals, birds and fish because ryanodine receptors in vertebrate are 400 to 3000 times less sensitive to it than are those of the target insects [Citation13]. Oral administration of chlorantraniliprole resulted in very low acute toxicity to birds; showing viable embryo reduction in Anas platyrhynchos (Mallard) and eggshell thinning in Colinus virginianus (Northern bobwhite) [Citation11,Citation14]. Another study confirmed the potential dangerous of coragen to the development of birds; where injected coragen into the yolk sac of eggs with doses of 0.1, 0.5, 1.0 and 1.5 μl/1000 μl/egg, induced a gradual increase of the mortality rate from lower to higher doses. As well as, high percentages of external malformations with appearance of several types of abnormalities in each embryo at 12th day of development were induced. Also, microcephaly, hydrocephaly, edematous swelling, hematoma formation, aberrant body color, microphthalmia, distorted beak, agnathia, micromelia, amelia, omphalocele and ectopic heart are among the qualitative defects that have been generated [Citation11].
Sub-acute oral administration of coragen (1000 mg/kg for 14 and 21 days) caused various signs of toxicity on hematological parameters with nausea, eye bulging, nose bleeding, tongue blackening, lack of body co-ordination and weakness of the body in Charles foster rats [Citation15]. Orally administered coragen in male albino rats every 2 days for 90 days, caused a decrease in body weight gain and organ weight (heart, kidney and brain), while an increase in spleen and liver was resulted. Moreover, increase in aspartate aminotransferase (AST) and alanine aminotransferase (ALT), hematological changes and slight increase in urea was detected. Furthermore, coragen 1/20LD50 induced chronic histopathological changes in liver and kidneys of male albino rats [Citation16]. Similarly, a recent study revealed that, oral administration of sub-lethal dose (2000 mg/kg three times/week/4 weeks) of coragen in male albino rats induced oxidative damage (inhibition of glutathione peroxidase and superoxide dismutase and enhanced lipid peroxidation), biochemical alterations and histopathological changes in liver, spleen and lung combined with symptoms of toxicity as closed eyes, nose bleeding, muscle paralysis and whole body weakness [Citation17]. In the same way, coragen (chlorantraniliprole) caused changes in the pathological parameters of the sub-acute and sub-chronic liver, kidneys, and protein profile, which resulted in increase in AST and ALT activities, urea, and creatinine concentrations. Also, there was a decrease in albumin, globulin and blood calcium content [Citation18]. Because slight work has been achieved on the teratogenesis, further studies are needed to evaluate the hazardous of coragen on the developmental stages of animals and humans.
This study aimed to investigate the teratogenic and genotoxic effects of coragen after intrauterine exposure to coragen in pregnant rats (Rattus norvegicus) from 6th to 20th day of gestation.
Materials and methods
Insecticide
Coragen 20% SC with structure formula [C18H14N5O2BrCl2] is the chemical used in this study, which is an anthranilic diamide insecticide containing chlorantraniliprole as an active substance with concentration 200 g/l [Citation19]. Coragen 20% SC was purchased from the central agriculture pesticides laboratory at agriculture research center, Dokki-Giza-Egypt. According to Lahm et al. [Citation20], and Shallan et al. [Citation16], LD50 for coragen in Albino rats is ≥5000 mg/kg body weight.
Experimental animals and treatments
Forty-five albino rats (Rattus norvegicus); 15 males and 30 virgin females, weighing 170 ± 20 g were provided from the animal house of the National Research Center, Egypt. Animals were housed in polypropylene plastic cages covered by metal wire at 25 ± 2°C and 55 ± 5% humidity with a 12 light–12 dark cycle. The protocols for animal use and the experimental procedures described here were approved by the Cairo University Institutional Animal Care and Use Committee (CU-IACUC) (Egypt) (approval no.: CU/I/F/56/19). Females with regular estrous cycle were selected for the treatment studies. Two adult females were caged with one male overnight. In the next morning; fertilization was confirmed by detecting sperms in the vaginal smears (by staining the vaginal smears with methylene blue). Females with positive vaginal smears were regarded as pregnant and the first day of gestation was considered to be the day of detection [Citation21].
Thirty pregnant rats were separated in cages and randomly classified into three groups, ten females per group, orally received the corresponding treatment by gavage once daily from 6th day to 20th day of gestation as follows:
Group A (Control group): Pregnant rats received orally 0.9% saline solution.
Group B (Low dose group; LD): Pregnant rats received orally 100 mg/kg (1/50 LD50) of coragen.
Group C (High dose group; HD): Pregnant rats received orally 200 mg/kg (1/25 LD50) of coragen.
Pregnant rats were checked daily to determine any signs of toxicity or behavioral change. The weights of the pregnant rats were weekly recorded.
Assessment of reproductive toxicity and teratogenicity
On 20th day of gestation, dams were euthanized and subjected to ectomy. The uteri were detached, washed with 0.9% saline solution, dried on absorbed paper and weighed. The numbers of implantation sites were counted at all uterine swelling containing embryos (alive, dead and resorbed) in both control and treated rats. Also, the pregnant rats and their fetuses were then dissected; liver and kidney were excised for histopathological examinations.
Fetal evaluation
Fetuses were examined for any external anomalies, and their body weight and body lengths were determined. Fetuses were examined for skeletal malformations through skeletal staining with alcian blue and alizarin red double staining as stated by Young et al. [Citation22], with some modifications, followed by skeletal examination and photography.
Histopathological studies
Fetal and maternal specimens of liver and kidney of the control and treated groups were firstly fixed in 10% buffered formalin solution for 24 hr, then, dehydrated using ascending series of ethyl alcohol. Specimens were cleared in xylol and embedded in paraffin wax. The samples were sectioned at 5 µm and stained with H&E for routine examination and photomicrography by the light microscope [Citation23].
Comet assay
Comet assay was carried out according to the protocol described by Nandhakumar et al. [Citation24]. It was carried out for the maternal and fetal liver and kidney tissues of the three studied groups; control, LD and HD. Briefly, A small piece of each tissue was minced in 0.5 ml of Hank’s balanced salt solution (HBSS). Then, 10 µl of cell suspension was mixed with 70 µl of 0.5% low melting agarose (Sigma) and spread on a slide layered with 1% normal melting agarose. Slides were immersed in cold lysis buffer (2.5 M NaCl, 100 mM EDTA, 10 mM Tris base, 1% Triton X-100 and 10% DMSO) for 24 h at 4°C in darkness. Subsequently; slides were washed for 10 min in dH2O, incubated for 20 min in an alkaline buffer (300 mM NaOH and 1.0 mM EDTA, pH 13) and electrophoresed for 20 min at 300 mA and 25 V (0.90 V/cm). After electrophoresis, slides were neutralized in 0.4 M Tris (pH 7.5), fixed in 100% cold ethanol, dried and stained with ethidium bromide (0.2 μg/ml). For scoring; slides were examined by fluorescent microscope (Carl Zeiss Axioplan). The extent of DNA migration was determined by imaging using a Closed-Circuit Digital (CCD) camera and scoring of 50 cells at magnification 400× using Comet 5 image analysis software (Kinetic Imaging, Ltd., Liverpool, UK). Tail length, percentage of DNA in tail and tail moment was evaluated and used as indicators for DNA damage.
Statistical analysis
Data were analyzed using the statistical package for social sciences software (SPSS) version 20.0. Analysis of variance (ANOVA) was used to illustrate the effect of each treatment with the control and to determine differences among groups. The results are presented as mean ± standard error (SE) for three samples from each group. P-value ≤0.05 was considered as statistical significance.
Results
Effect of coragen on maternal toxicity
Pregnant albino rats treated orally with low dose (100 mg/kg) or high dose (200 mg/kg) of coragen survived until the end of the experiment and no mortality or abortion cases were reported throughout the work. Other than a loss of appetite, the dams showed no obvious signs of illness or abnormal behavior. Pregnant female rats treated with low or high doses of coragen showed significant reduction in their body weight gain (BWG) compared with the control (P < 0.05) ().
Table 1. Effect of coragen on mother’s body weight gain (BWG), uterus weight, resorption per dam and placental weight in the control and different treated groups
Gravid uteri from pregnant dams were weighed and checked carefully for any abnormalities through cesarean sections. Fetuses derived from control dams showed that fetuses were normally distributed among the two horns with no anomalis (). Gravid uteri from LD treated dams showed an asymmetrical distribution of their fetuses in the two horns. In addition, dead fetuses and bleeding were observed (&c). Bleeding, dead fetuses and resorption were recorded in several cases in uterine horns of dams in the HD treated group (&e). A significant decrease in registered uterine weights of the dams of both treated groups was observed in comparison with the control one. Also, significant resorption of the fetuses was observed in HD treated group compared to the control and LD treated groups (P > 0.05), while non-significant resorption was induced in LD treated group compared to the control (P > 0.05). In addition, a significant decrease in the average weight of placenta of treated pregnant rats was observed compared with the control group (P > 0.05). No significant difference was found in BWG, uterine weight, resorption per dam and placental weight between LD and HD groups (P > 0.05) ().
Figure 1. Photographs of the uteri of pregnant albino rats secluded at 20th day of gestation. (A) Control group showing uterus with normal distribution of fetuses in both horns. (B, C) LD group, (D, E) HD group. Uterine bleeding (arrow), dead fetuses (lines), resorbed fetus (head arrow). F = fetus, Pl = placenta, V = vagina
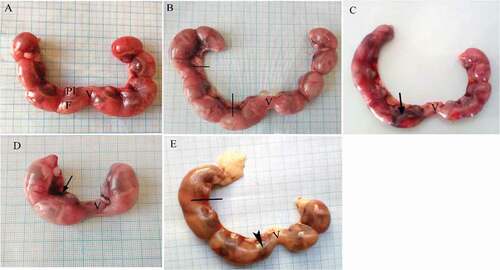
Morphological fetal examination
The morphological examination of the fetuses maternally treated with low or high dose of coragen showed significant appearance of hematoma compared with the control group ( & ). It was observed that fetuses maternally treated with LD or HD of coragen from 6th to 20th day of gestation showed a significant decrease in their mean body weight and body length compared with the fetuses from the control group (p < 0.05), while no significant difference was observed between LD and HD groups (P > 0.05). HD of coragen significantly increased the number of dead fetuses in comparison to the control and LD groups (P < 0.05) ().
Table 2. Effect of coragen on fetal weight and length, number of hematoma and dead fetuses of mothers in the control and different treated groups
Fetal skeletal examination
and revealed several ossification defects in both coragen treated groups. The fetuses maternally treated with LD group showed several skeletal anomalies including; decreased degree of ossification in the bones of the median dorsal series (), shortage of the last rib, loss of chondrification of some tail vertebrae (&c), sternum ossification is incomplete () and absence of fore limb ossification (). Hind limb showed variety in degree of ossification from unossified of metatarsals only to all hind bones (&c).
Table 3. Effect of coragen on skeletal examination of fetuses in the control and different treated groups (N = 32)
Figure 3. Photographs of the cranial skeleton of fetuses at 20th day of gestation (Alcian blue–Alizarin red double stain). (A) Control group with normal ossification of the cranial bones. (B) LD group: incompletely ossified cranial bones. (C) HD group: unossified cranial bones. N = nasal, Mx = maxilla, Mn = mandible, Fr = frontal, and Pr = parietal
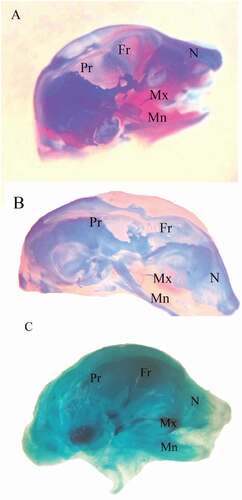
Figure 4. Photographs of the vertebral column and ribs of fetuses at 20th day of gestation (Alcian blue–Alizarin red double stain). (A) Control group: vertebral column showed complete ossification of all vertebrae and normal appearance of ribs. (B, C) LD group: vertebral column showed shortage of the last rib (arrow), and unossified vertebrae (head arrow) and loss of chondrification of some tail vertebrae (wavy arrow). (D) HD group: unossification of vertebral column and centra. (head arrow). ThV = thoracic vertebra and LV = lumbar vertebra
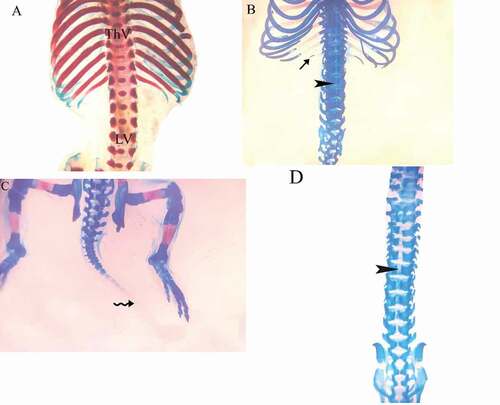
Figure 5. Photographs of the sternum of fetuses at 20th day of gestation (Alcian blue–Alizarin red double stain). (A) Control group: ossification of all sternebrae. (B) LD group: incomplete ossification of the sternum. (C) HD group: unossified sternebrae
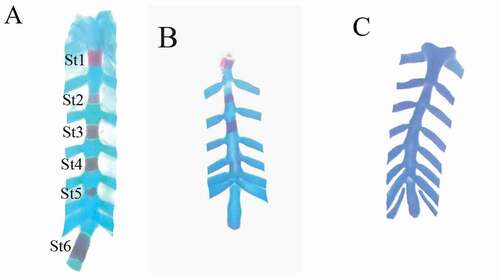
Figure 6. Photographs of the pectoral girdle and forelimb of fetuses at 20th day of gestation (Alcian blue–Alizarin red double stain). (A) Control group: pectoral girdle and forelimbs showed complete ossification of all bones. (B) LD group. (C) HD group: showed incomplete ossification of all bones. Sc = scapula, C = clavicle, Hu = humerus, R = radius, U = ulna, Mc = metacarpals, and Ph = phalanges
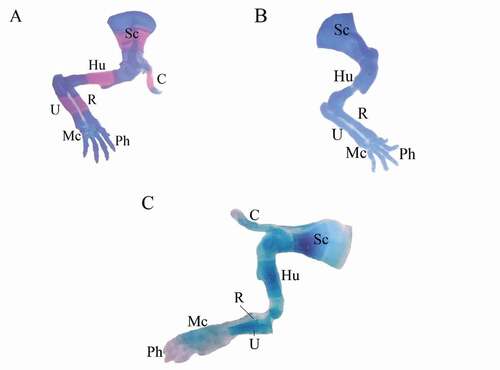
Figure 7. Photographs of the pelvic girdle and hind limbs of fetuses at 20th day of gestation (Alcian blue–Alizarin red double stain). (A) Control group: showed ossification of bones of pelvic girdle and hind limbs. (B, C) LD group: showed unossification of the metacarpals (arrow) or unossification of all hind limb bones (tibia, fbula, metatarsals, and phalanges). (D) HD group: completely unossified bones. I = ilium, Is = ischium, Ps = pubis, Fe = femur, Fi = fbula, Ti = tibia, Mt = metatarsals, and Ph = phalanges
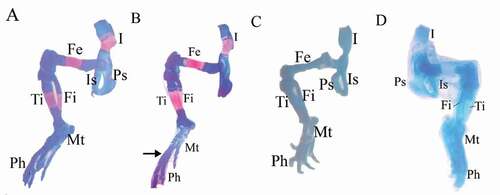
The fetuses maternally treated with HD group showed several skeletal anomalies including; completely unossified skulls (), centra of vertebrae (), sternal bones (), fore and hind limbs with their corresponding girdles ().
Histopathological examination
Maternal liver
The hepatic architecture of control group revealed a standard lobular structure with tightened hepatic cords extend from the central vein to the periphery. The hepatocytes are large polygonal cells, regular in shape and contain large spherical nucleus. The hepatic strands were separated by a sinusoidal system converging with the normal dilation into the central vein ().
Figure 8. Photomicrographs of liver sections of pregnant dams (stained with H&E) obtained on the 20th day of gestation. (A) Control group: normal lobular architecture with regular arrangement of hepatocytes around a central vein. Hepatocytes and their nuclei and blood sinusoids are normal in shape. (B) LD group, (C, D) HD group: loss of hepatocyte architecture, wide sinusoids (lines), lymphatic infiltration (arrow), cytoplasmic vacuoles (wavy arrow). hemorrhage (asterus), apoptotic cells (curved arrow). H = hepatocytes, CV = central vein, S = sinusoids, and K = Kupffer cells
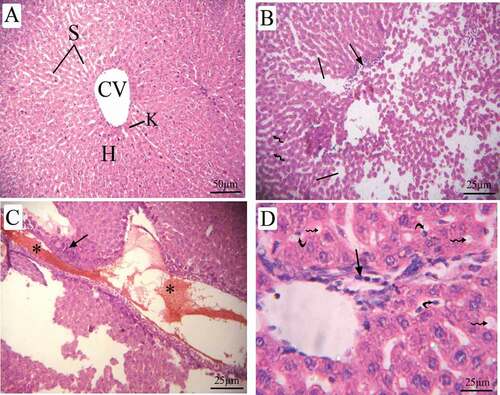
The liver sections of pregnant rats treated with LD of coragen displayed loss of hepatocyte architecture, wide sinusoids, lymphatic infiltration and cytoplasmic vacuoles (). The dams treated with HD of coragen showed disorganization in their hepatic architecture with large dilatation in the sinusoid, hemorrhage and apoptotic cells (&d).
Fetal liver
The fetal liver sections of 20th day of gestation of the control group are distinguished by hepatic lobules with irregularly ramified and interconnected hepatic strands, which run from the central vein to the periphery (). Fetal liver maternally treated with LD or HD of coragen showed distinct cytoplasmic vacuoles and degeneration in the hepatic cells (). In addition, degeneration in the endothelial lining the central vein, loss of the hepatocyte architecture, dilated sinusoids and increasing the megakaryocytes were observed in fetal liver maternally treated with HD of coragen ().
Figure 9. Photomicrographs of fetal liver sections (stained with H&E) at 20th day of gestation (A) Control group: normal structure of liver with normal hepatocyte nuclei. (B) LD group, (C) HD group: showed cytoplasmic vacuoles (arrow), vacuolated hepatocytes (head arrow), degenerated hepatocytes (asterus), Central vein with degenerated endothelial lining (lines), Megakaryocytes (M) and dilated sinusoid (wavy arrow)
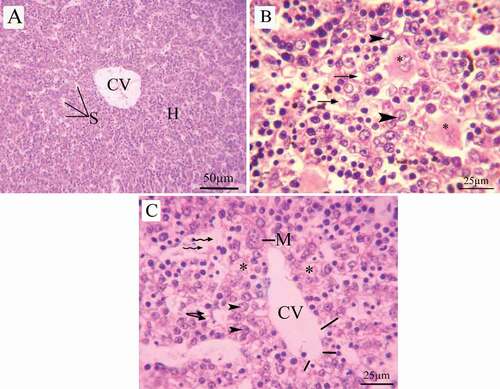
Maternal kidney
Histopathological examination of renal tissue of control pregnant rats revealed normal histological appearance. The renal corpuscle was made up of a glomerulus surrounded by the Bowman’s capsule and normal urinary space. There were proximal convoluted tubules with narrow lumens among the renal tubules, and distal convoluted tubules with wide lumen (). Pregnant rats treated with LD of coragen showed some histopathologic changes including; shrunken of glomeruli, urinary space dilation and basement membrane of Bowman’s capsule deterioration, shredded nuclei into the lumen of the tubule, hypertrophy and hyperplasia of the tubular epithelial lining in addition to disappearance of the lumen of renal tubules (). Pregnant rats treated with HD of coragen exhibit inflammatory infiltration, fragmentation in tubular epithelial lining and hemorrhage (&d).
Figure 10. Photomicrographs of a transverse section of maternal kidney tissue (stained with H&E) at the 20th day of gestation. (A) Control group: normal histologic pattern of renal tissues; normal glomeruli surrounded by Bowman’s capsule and normal capsular space and normal renal tubules (RT). (B) LD group, (C,D) HD group: shredded nuclei into the tubule lumen (arrow), hypertrophy (line), and hyperplasia of the tubular epithelial lining (wavy arrow), disappearance of lumen (arrow head), inflammatory infiltration (curved arrow), fragmented tubular epithelial lining (U-shaped arrow), hemorrhage (asterus) and distortion in the epithelial lining of Bowman’s capsule (blue arrow). G = glomeruli, BC = Bowman’s capsule, CS = capsular space, and RT = renal tubules
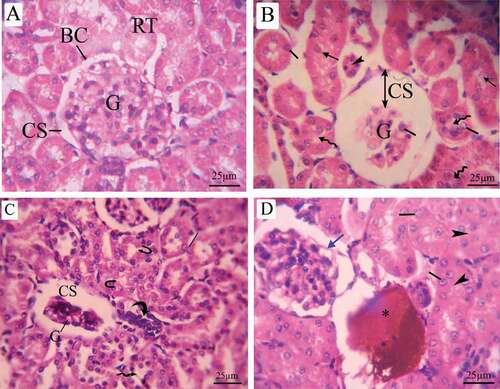
Fetal kidney
Histopathological examination of the fetal kidney at 20th day of gestation of the control group revealed normal tissue structures including; a prominent Bowman’s capsule encircling the glomerulus with normal cells and capillaries, as well as normal tubular structure and interstitial parenchyma (). The kidney of fetus maternally treated with LD of coragen showed shrinkage in the glomerulus with dilated inter-capsular space and distortion in the epithelial lining of Bowman’s capsule, in addition to disappearance of the lumen of the tubules and appearance of necrotic cells (). While the kidney of fetus maternally treated with HD of coragen revealed damage in the renal tubules, necrosis, karyolysis and increases of parenchymal cells (&d).
Figure 11. Photomicrographs of fetal kidney transverse sections (stained with H&E) at 20th day of gestation. (A) Control group: distinct Bowman’s capsule-enclosed normal glomeruli with normal urinary space. Renal tubules with normal nuclei were observed. (B) LD group, (C,D) HD group: damaged glomeruli surrounded by a decomposed Bowman’s capsule basement membrane (head arrow)and increase in the intercapsular space (line), disappearance of lumen of renal tubules (arrow), damaged tubules (wavy arrow), necrosis, Karyolysis, increase in the number of parenchymal cells and renal tubule hypertrophy (curved arrow). BC = Bowman’s capsule, G = glomeruli, RT = renal tubules, N = necrosis, K = karyolysis, and Pa = parenchymal cells
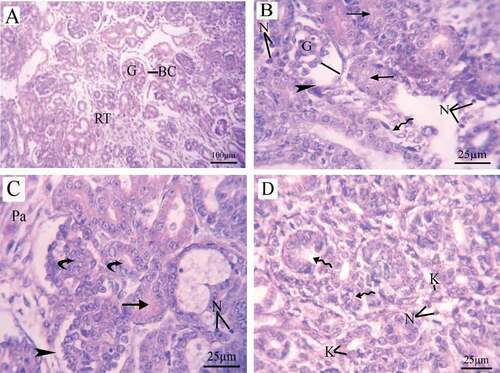
Genotoxicity
Coragen induced DNA damage in liver and kidney of pregnant rats
and 14 show that treatment with coragen resulted in induction of DNA damage in maternal liver and kidney tissues as indicated by statistically significant increase in tail length, percentage of DNA in tail and tail moment in coragen-treated groups (LD or HD) in comparison with the control untreated group (P < 0.05).
Figure 12. Effect of coragen on DNA damage induced in maternal liver and kidney tissues represented as (A) tail length, (B) % DNA in tail and (C) tail moment in different treated groups. *Significant difference as compared to the control group (P < 0.05), #Significant difference as compared to LD group (P < 0.05)
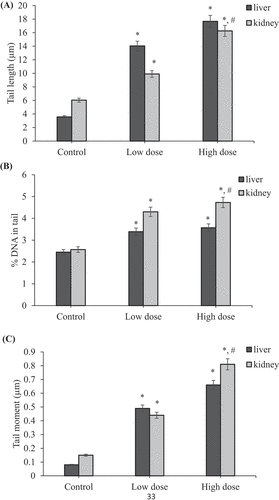
It was noticed that the HD of coragen induced more DNA damage than that induced by the LD of coragen in maternal liver but not statistically significant (P > 0.05). Furthermore, the maternal kidney showed a statistically significant increase in tail length and tail moment induced by the HD of coragen compared with the LD (P < 0.05).
Coragen induced DNA damage in liver and kidney tissues of fetus maternally treated with LD or HD of coragen
As shown in ; statistically significant increase in tail length, percentage of DNA in tail and tail moment in liver and kidney tissues of fetuses maternally treated with LD or HD of coragen compared with those of fetuses maternally untreated with coragen (P < 0.05). Moreover, the HD of coragen induced more DNA damage in the fetal kidney tissues than that induced by the low dose of coragen as indicated by statistically significant increase in tail length and percentage of DNA in tail (P < 0.05). The fetal liver showed no statistically significant difference in DNA damage induced by the HD of coragen compared with the LD treated group (P > 0.05).
Figure 13. Effect of coragen on DNA damage induced in fetal liver and kidney tissues represented as (A) tail length, (B) % DNA in tail and (C) tail moment in different treated groups. *Significant difference as compared to the control group (P < 0.05), #Significant difference as compared to LD group (P < 0.05)
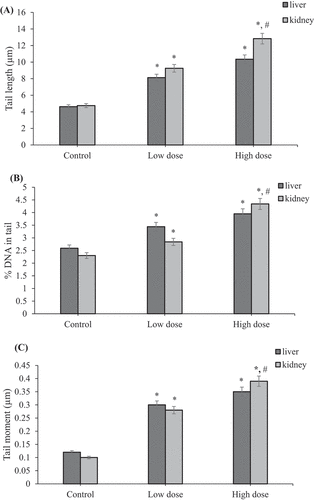
Figure 14. Representative photomicrographs showing typical nuclei with various degrees of DNA damage observed as comet induced by coragen in maternal and fetal liver and kidney tissues. LD group and HD group were compared with undamaged DNA of control group. 1, maternal liver cells; 2, fetal liver cells; 3, maternal kidney cells and 4, fetal kidney cells. Magnification x400
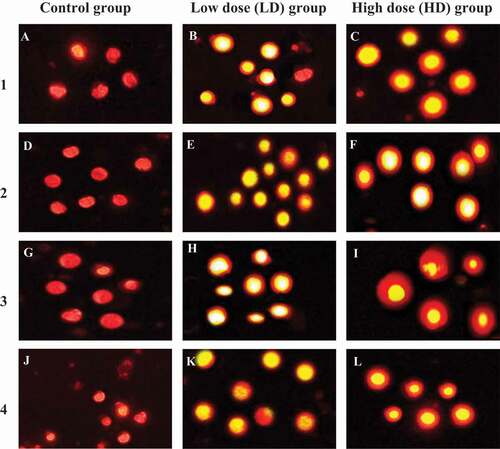
Discussion
Pesticides enter the human and animal bodies through breathing, swallowing, or skin and get access into the cells and nuclei inducing cytotoxic effects. Workers with long-term exposure to pesticides have a high risk for diseases such as: cancer, diabetes, genetic, cardiovascular and neurodegenerative disorders [Citation3].
The current study investigated the effect of exposure of the pesticide coragen (chlorantraniliprole) with two different doses on the pregnant albino rats from day 6 to day 20 of gestation. The results reported that the oral administration of coragen caused a significant reduction in the body weight gain, in coincidence with Shallan et al. [Citation16], who recorded a significant body weight reduction after coragen administration in male albino rats. Furthermore, Luckett [Citation25] noticed that the dogs those have received any dietary concentration of chlorantraniliprole causing 20% reduction in their body weight.
The current study reported a significant decrease in weight of uterine horn that could be because lower number of live fetuses and higher resorption percentages in two treatments compared to the control group. Similar results have been observed in rats after lufenuron exposure [Citation26] and co-exposure to cypermethrin and dimethoate [Citation27].
In the present study, coragen treatment induced fetal growth retardation through fetal body weight and fetal length, morphological disorders including; hematoma and skeletal malformation such as loss of chondrification of some tail vertebrae and shortage of last rib. In the same way, a previous study reported that consumption of coragen by rats had a negative impact on calcium and bone properties, resulting in osteoporosis [Citation28]. This might be related to the delay of ossification and skeletal anomalies in the current study. As well as, teratogenic development was observed in chick embryo upon exposure to chlorantraniliprole [Citation11]. Moreover, increased fetal resorption and a decrease in live fetal number, combined with skeletal anomalies, were recorded as indication of teratogenicity in the exposed pregnant rats to other insecticides such as lufenuron [Citation26], chlorpyrifos [Citation29], cypermethrin [Citation27,Citation30], dimethoate [Citation31], emamectin benzoate [Citation32], fipronil [Citation33] and organophosphorous pesticides [Citation34].
Coragen as an insecticide that selectively targeting ryanodine receptors, a group of calcium ion channels found in cardiac and skeletal muscles. Nanomolar concentration of coragen locks the receptor in a half-open state, whereas its micromolar concentration fully closes them [Citation35]. Furthermore, Abdel-Mobdy et al. [Citation18] proved that coragen affects the calcium content in serum of rats given both sub-acute and sub-chronic doses, where a slight decrease in serum calcium content was observed in sub-acutely treated rats, and the highest decrease in serum calcium in the group received 1/2 of the LD50 that showed the most toxic effects.
Regarding the histopathological examination; Liver plays an essential role in metabolism to preserve energy and metabolic stability of the body [Citation36], in addition to its role in biotransformation where toxic compounds are converted into less-toxic ones to reduce toxicity [Citation37]. Pathological variations in the maternal and fetal liver in which the severity of lesions is increasing with coragen dose were shown in the present study. These results agree with Shallan et al. [Citation16], who found lymphocytic infiltration, congestion of portal blood vessels and Kupffer cell activation in liver of male rats exposed to different doses of coragen. Again, Saafi et al. [Citation38], who found that pesticide intoxication resulted in severe histopathological changes including mononuclear cell infiltration in the parenchymatous tissue and portal area, congestion, enlargement of the hepatic sinusoids and enlargement of the central and the portal veins and hepatocellular damage.
Kidneys are almost developed in newborn rats, while are completely developed in adult rats. Nephrogenesis begins at 12–13 days of gestation and is completed at postnatal days 10 in rats [Citation39]. Normal fetal kidneys show corpuscles, glomeruli, and proximal and collective tubules at various stages of development with highest volume of distal and proximal tubules, but smaller volume of the cortex, medulla, glomerular and Bowman’s capsule and lower number of glomeruli than in newborn or adults. Glomeruli on the outer surfaces of kidney are less mature than those on the inner surface [Citation40].
Histopathological examination of kidney obtained from both coragen-treated dams and their fetuses revealed reduction in glomeruli, lesion in Bowman’s capsule, hypertrophy, and hyperplasia of the tubular epithelial lining, in addition to inflammatory infiltration and hemorrhage in the maternal kidney. Similar results were recorded for coragen [Citation41] and for chlorpyrifos [Citation42,Citation43] in previous studies. The decreased glomeruli size could have been caused by a nephron-toxicant that causes renal vasoconstriction [Citation41]. In fetal renal tissue, coragen disfigured the renal configuration, distorted the epithelial lining of Bowman’s capsule, damaged the renal tubules and increased the parenchymal cells. These results are in agreement with Dutta et al. [Citation19], and Mohammed [Citation44] who reported that coragen is causing toxicity to liver and kidney. Also, Hassan et al. [Citation41] emphasized that coragen (2000 mg/kg) elevated the level of urea, uric acid and creatinine in serum combined with a disturbance in kidney functions in rats.
Several studies have reported the cellular responses that result from exposure to pesticides including; DNA damage or chromosomal changes by induction of oxidative stress through production of free radicals that accumulate in the cell and affecting the biological macromolecules [Citation45–47].
Yahia et al. [Citation48] found that Lambda cyhalothrin and Dimethoate insecticides induced DNA damage in bone marrow of rats 48 h with mild histopathological changes. Also, Tsitsimpikou et al. [Citation45] mentioned that propoxur and diazinon induced an oxidative DNA damage in kidney and liver of rabbits after long-term exposure. Another study reported histopathological alterations in liver and significant increase in DNA damage among rats exposed to Chlorpyrifos with a dose-dependent effect by using the comet assay and the micronucleus test for the whole blood and bone marrow, respectively [Citation49]. Moreover, Ferri et al. [Citation50], noticed a DNA damage in lymphocytes from workers who were exposed to insecticides such as chlorpyriphos and deltamethrin.
Herein, as detected by comet assay; coragen induced a significant DNA damage in the liver and kidney tissues of the mother and their fetuses compared to the control. A significant and marked increase in all DNA damage parameters including; tail length, percentage of DNA in tail and tail moment was observed. However, the high-dose of coragen induced a significant more DNA damage in the kidney tissues of both the mother and their fetuses than the low-dose. On the other hand, no significant difference of DNA damage was detected in the liver tissues between high-dose and low-dose groups of both the mother and their fetuses. Our findings are in contiguous with a previous study that recorded a severe haemato-toxicity, hepato-renal toxicity and oxidative damage after oral administration of coragen (1000 mg/kg for 6 weeks) in Charles foster rats [Citation19]. Induced DNA damage may be attributed to the alkylating abilities of the pesticide chemicals that induce breaks in DNA and thus affecting DNA structure and functions [Citation51]. Many studies have mentioned that the main mechanism for toxicity of pesticides is the production of reactive oxygen species (ROS) including free radicals of oxygen, hydroxyl or hydrogen peroxide that induce oxidative stress in different tissues. Those free radicals could bind to and penetrate through the biological membranes and cause damage to DNA, RNA and protein macromolecules [Citation16].
Conclusion
Finally, it could be concluded that the gestational coragen treatment resulted in teratogenic and genotoxic effects on pregnant rats and their fetuses, implying the same risk for women and their fetuses in the human being.
Availability of data and materials
All data generated or analyzed during this study are included in this published article.
Declarations
Ethical approval
Experimental protocols and procedures used in this study were approved by the Cairo University Institutional Animal Care and Use Committee (CU-IACUC) at Cairo University, Faculty of Science (Egypt) (approval no. CU/I/F/56/19), in accordance with the international guidelines for care and use of laboratory animals.
Authors’ contributions
A.R.O., A.E.D. and H.B. conceived, designed, planned and supervised the study. A.R.O., M.M.A and H.B. performed the experiments, analyzed and graphed data, interpreted results and wrote initial draft of the manuscript. A.R.O., A.E.D. and H.B. critically revised and edited the manuscript. All authors read the final manuscript and approved submission.
Acknowledgments
Authors acknowledge the Genetics Laboratory, Department of Zoology, Faculty of Science, Cairo University, Giza, Egypt, where comet assay was performed.
Disclosure statement
No potential conflict of interest was reported by the authors.
Additional information
Funding
References
- Sharma A, Kumar V, Shahzad B, et al. Worldwide pesticide usage and its impacts on ecosystem. SN Appl Sci. 2019;1(11):1446.
- Sarkar S, Gil JDB, Keeley J, et al. The use of pesticides in developing countries and their impact on health and the right to food. European Union: European Parliament’s online database; 2021. p. 1–56 doi:10.2861/28995.
- Hernandez AF, Parron T, Tsatsakis AM, et al. Toxic effects of pesticide mixtures at a molecular level: their relevance to human health. Toxicology. 2013;307:136–145.
- Nauen R. Insecticide mode of action: return of the ryanodine receptor. Pest Manag Sci. 2006;62(8):690–692.
- Teixeira LA, Andaloro JT. Diamide insecticides: global efforts to address insect resistance stewardship challenges. Pestic Biochem Physiol. 2013;106(3):76–78.
- Kar A, Mandal K, Singh B. Environmental fate of chlorantraniliprole residues on cauliflower using QuEChERS technique. Environ Monit Assess. 2013;185(2):1255–1263.
- Ioriatti C, Anfora G, Angeli G, et al. Effects of chlorantraniliprole on eggs and larvae of Lobesia botrana (Denis & Schiffermuller) (Lepidoptera: tortricidae). Pest Manag Sci. 2009;65(6):717–722.
- Bacca T, Cabrera NJ, Gutiérrez Y. Toxic effect of chlorantraniliprole on new‐born larvae of the potato tuber moth Tecia solanivora (Lepidoptera: gelechiidae). Ann Appl Biol. 2021;179(2):169–175.
- Lahm GP, Cordova D, Barry JD. New and selective ryanodine receptor activators for insect control. Bioorg Med Chem. 2009;17(12):4127–4133.
- Sattelle DB, Cordova D, Cheek TR. Insect ryanodine receptors: molecular targets for novel pest control chemicals. Invertebrate Neuroscience: IN. 2008;8(3):107–119.
- Abbas S, Iqbal R, Butt MZ, et al. Teratogenic Effects of Chlorantraniliprole on chick embryos (Gallus gallus domesticus). Indian J Anim Res. 2017(OF). doi:10.18805/ijar.B-569.
- London L, Beseler C, Bouchard MF, et al. Neurobehavioral and neurodevelopmental effects of pesticide exposures. Neurotoxicology. 2012;33(4):887–896.
- Larson JL, Redmond CT, Potter DA. Comparative impact of an anthranilic diamide and other insecticidal chemistries on beneficial invertebrates and ecosystem services in turfgrass. Pest Manag Sci. 2012;68(5):740–748.
- Anwar K. Cypermethrin, a pyrethroid insecticide induces teratological and biochemical changes in young chick embryos. Pak J Biol Sci. 2003;6(19):1698–1705.
- Kumar A, Dutta K, Najam A, et al. Coragen causes haematological alterations in Charles foster rats | semantic scholar. Eur J Toxicol Sci. 2013;4:1–7.
- Shallan -MAA-MYE, Hamdi E, Abdel Rahim E. Coragen (Chlorantraniliprole) insecticide effects on male albino rats. Res J Pharm, Biol Chem Sci. 2016;7(6):1536–1545.
- Noha M, Meligi Hfhah SM. Coragen induced toxicity and the ameliorative effect of an Origanum majorana L. in male albino Rats. J Am Sci. 2019;15(9):33–44.
- Abdel-Mobdy YE, Moustafa M, Nahas AHA, et al. Sub-acute and sub-chronic effect of chlorantraniliprole (Coragen® 20% SC) on Albino Rat. J Plant Protection Pathol. 2017;8(6):297–303.
- Dutta K, Ali M, Najam A, et al. Ameliorative effect of seed extract of Pterocarpus santalinus on coragen induced haematological alterations and serum biochemical changes in Charles Foster rats. J Toxicol Environ Health Sci. 2014;6(10):194–202.
- Lahm GP, Stevenson TM, Selby TP, et al. Rynaxypyr: a new insecticidal anthr anilic diamide that acts as a potent and selec tive ryanodine receptor activator. Bioorg Med Chem Lett. 2007;17(22):6274–6279.
- Burdan F, Pliszczynska-Steuden M, Rozylo-Kalinowska I, et al. Developmental outcome after exposure to cyclooxygenase inhibitors during pregnancy and lactation. Reprod Toxicol. 2011;32(4):407–417.
- Young AD, Phipps DE, Astroff AB. Large-scale double-staining of rat fetal skeletons using Alizarin Red S and alcian blue. Teratology. 2000;61(4):273–276.
- Bancroft JD. LCSSK. Bancrof’s Teory and Practice of Histological Techniques. 7th ed. [updated 2021/09/12/21:18:11. London: Churchill Livingstone; 2013.
- Nandhakumar S, Parasuraman S, Shanmugam MM, et al. Evaluation of DNA damage using single-cell gel elect rophoresis (Comet Assay). J Pharmacol Pharmacotherapeutics. 2011;2(2):107–111.
- Luckett EM. DPX-E2Y45 technical: 90-day oral toxicity study in dogs. Unpublished report No12749 from MPI Research, Mattawan, Michigan, USA Submitted to WHO by EI du Pont de Nemours and Company, Wilmington, Delaware, USA. 2004; DPR Vol. #53042-0062; −0064, record #231678; 231680.
- Basal WT, Ahmed ART, Mahmoud AA, et al. Lufenuron induces reproductive toxicity and genotoxic effects in pregnant albino rats and their fetuses. Sci Rep. 2020;10(1):19544.
- Ramon-Yusuf SB, Aliu YO, Salawu OA, et al. Maternal and foetal toxicity induced by exposure to mixture of dimethoate and cypermethrin in albino rats. J Toxicol Environ Health Sci. 2017;9(6):59–65.
- Abdel-Mobdy AE, Abdel-Mobdy YE, Mabrok HB. Cow milk and its dairy products ameliorate bone toxicity in the Coragen-induced rat model. Open Agric. 2021;6(1):498–510.
- Tian Y, Ishikawa H, Yamaguchi T, et al. Teratogenicity and developmental toxicity of chlorpyrifos. Maternal exposure during organogenesis in mice. Reprod Toxicol. 2005;20(2):267–270.
- Emeka PM. Teratogenic and embryotoxic effects of orally administered cypermethrin in pregnant albino rats. J Toxicol Environ Health Sci. 2015;7(7):60–67.
- Farag AT, Karkour TA, El Okazy A. Developmental toxicity of orally administered technical dimethoate in rats. Birth Defects Res Part B, Dev Reprod Toxicol. 2006;77(1):40–46.
- Gabr GA, Soliman GA, Abdulaziz SS, et al. Teratogenic effects in rat fetuses subjected to the concurrent in utero exposure to emamectin benzoate insecticide. Pak J Biol Sci. 2015;18(7):333–340.
- Eisa AA, Abo-Elghar GE, Ammar IM, et al. Embryotoxicity and teratogenicity of fipronil in rats (Rattus Norvegicus). Zagazig J Agric Res. 2017;44(5):1851–1861.
- Yu Y, Yang Y, Zhao X, et al. Exposure to the mixture of organophosphorus pesticides is embryotoxic and teratogenic on gestational rats during the sensitive period. Environ Toxicol. 2017;32(1):139–146.
- Meer G. The structural basis of ryanodine receptor ion channel function. J Gen Physiol. 2017;149(12):1065–1089.
- Rui L. Energy metabolism in the liver. Compr Physiol. 2014;4(1):177–197.
- Singh D, Cho WC, Upadhyay G. Drug-induced liver toxicity and prevention by herbal antioxidants: an overview. Front Physiol. 2015;6:363.
- Saafi EB, Louedi M, Elfeki A, et al. Protective effect of date palm fruit extract (Phoenix dactylifera L.). on dimethoate induced-oxidative stress in rat liver. Exp Toxicol Pathol. 2011;63(5):433–441.
- Ortiz LA, Quan A, Weinberg A, et al. Effect of prenatal dexamethasone on rat renal development. Kidney Int. 2001;59(5):1663–1669.
- Şimşek N, Altunkaynak BZ, Ünal D, et al. A stereological and electron microscopic study of the develo pment of the neph ron in prenatal and postnatal rats (Turkey: The Eurasian Journal of Medicine). 2009. p. 84–90.
- Hassan HF, Mohammed HS, Meligi NM. Potential impact of marjoram on coragen-induced physiological and histological alterations in male albino rats. Egyptian J Zoology. 2021;75(75):25–38.
- Rekha R, Raina S, Hamid S. Histopathological effects of pesticide-cholopyrifos on kidney in albino rats. Int J Res Med Sci. 2013;1(4):465.
- El-Tawil MF. Toxicological effects of short-term feeding with chlorpyrifos and chlorpyrifos-methyl insecticides on adult albino rats (Pakistan: Middle East Journal of Agriculture Research) . 2014. p. 208–220.
- Mohammed EHA. Attenuating the toxicity effects of chlorontraniliprole in male albino rats using artemistia annua as natural antioxidant. Egypt: Department of Biochemistry, Faculty of Agriculture, Cairo University; 2016.
- Tsitsimpikou C, Tzatzarakis M, Fragkiadaki P, et al. Histopathological lesions, oxidative stress and genotoxic effects in liver and kidneys following long term exposure of rabbits to diazinon and propoxur. Toxicology. 2013;307:109–114.
- Ledda C, Cannizzaro E, Cina D, et al. Oxidative stress and DNA damage in agricultural workers after exposure to pesticides. J Occup Med Toxicol. 2021;16(1):1.
- Rapisarda V, Ledda C, Matera S, et al. Absence of t(14;18) chromosome translocation in agricultural workers after short-term exposure to pesticides. Mol Med Rep. 2017;15(5):3379–3382.
- Yahia D, Ali MF, El-Maguid DSA. Estimation of bone marrow DNA damage induced by lambda Cyhalothrin and dimethoate insecticides using alkaline comet assay. J Adv Vet Res. 2019;9(1):23–28.
- Ezzi L, Belhadj Salah I, Haouas Z, et al. Histopathological and genotoxic effects of chlorpyrifos in rats. Environ Sci Pollut Res Int. 2016;23(5):4859–4867.
- Ferri GM, Cavone D, Dambrosio M, et al. Lymphocytes DNA damages and exposure to chlorpyrifos, deltamethrin, penconazole, copper oxicloride. Biomarkers. 2019;24(2):186–198.
- Kaur R, Kaur S, Lata M. Evaluation of DNA damage in agricultural workers exposed to pesticides using single cell gel electrophoresis (comet) assay. Indian J Hum Genet. 2011;17(3):179–187.