ABSTRACT
The knowledge of the root nodule microbiome is still beyond the minimum. This study has been designed to isolate and characterize plant growth promoting bacteria and testing their ability to promote plant growth. In addition to Rhizobium MAP7, four other strains have been isolated from Phaseolus vulgaris nodules, and they have been molecularly identified as Bacillus MAP3, Brevibacillus MAP4, Pseudomonas MAP5, and Pseudomonas MAP8. The obtained strains showed the ability to produce IAA, siderophores, and HCN with different capabilities. A pot experiment was conducted to investigate the effect of the obtained strains on the physical growth parameters of Vicia faba. The inoculation with Rhizobium MAP7 along with Brevibacillus MAP4 and Pseudomonas MAP5 led to four times increase in the number of nodules (57/plant) compared with that of the control in addition to a significant increase in the physical growth parameters . Additionally, different nitrogen fractions increased significantly in response to this inoculum (226 and 206 mg/g dry weight total nitrogen for shoot and root respectively compared with 175 and 92.5 for control plants). The obtained strains in this study showed a good potentiality as plant growth promotors that might be tried with further crops on wider scale.
Introduction
Symbiotic nitrogen fixation, which is positioned as a major part of biological nitrogen fixation, is an important alternative source of chemical nitrogen fertilizers not only for leguminous but also for non-leguminous plants. The interaction between legumes and rhizobia leads to root nodule organogenesis, an organ that is produced in response to bacterial nod factors and plant developmental signals leading to the formation of a plant stem cell niche [Citation1]. Recently, rhizobia have been shown to improve the nutrition of non-leguminous crops, such as barley, wheat and canola [Citation2]. It has been established that the legume nodule is exclusively inhabited by the rhizobium. Meanwhile, in 2001, this concept has changed dramatically when non-rhizobial strains were regarded for their ability to nodulate legumes, such as Methylobacterium and Burkholderia that have been isolated from Crotalaria [Citation3] and Mimosa [Citation4], respectively. In addition to nodule-inducing bacteria, several bacterial strains have been isolated from nodules as co-inhabitants with rhizobium, such as Klebsiella, Pseudomonas [Citation5], Bacillus [Citation6] and Streptomyces [Citation7]. Interestingly, a review titled ‘the nodule microbiome: N2-fixing rhizobia do not live alone’ has been published in 2017 to conclude that some of these non-rhizobial bacteria might be nitrogen fixer or participate in nodule genesis and the others, more striking, might neither participate in nodulation nor fix nitrogen [Citation8].
Generally, plant genotype, environmental conditions, and growth-stage govern bacterial endophytic colonization inside plant tissues [Citation9]. Despite the recognized importance of the rhizosphere microbiome, the knowledge about the inhabitants of this selective region is still beyond the minimum as the culture-independent methods for exploring this community show that the number and diversity of rhizosphere microbiomes are highly underestimated. Besides plant beneficial bacteria, the rhizosphere zone might also be occupied with plant pathogens as well as human pathogens those which transfer via plant tissue to the host [Citation10]. Plant growth promoting bacteria would be beneficial to plants either directly or indirectly. The distinction between the two is not always clear: indirect mechanisms are usually those that occur outside the plant, while direct mechanisms are those that occur inside the plant and directly influence the metabolism of the plant. Direct mechanisms therefore include those that influence the balance of plant growth regulators, either because the microorganisms themselves release plant-integrated growth regulators or because the micro-organisms function as a sink of plant-released hormones, and those that induce the metabolism of the plant leading to an improvement in its adaptive ability. This includes phytohormones production, phosphate solubilization and nitrogen fixation. However, indirect mechanisms include plant defensive metabolic processes, which respond to the signal sent by the plant-influencing bacteria. This reaction comprises two essential mechanisms: induction of systemic resistance to plant pathogens (biotic stress) and defense against unhealthy environmental conditions (abiotic stress) in addition to siderophores and antibiotics production [Citation11].
Broad bean (Vicia faba L.) is considered one of the most important legumes in Egypt. It has become one of the strategic crops due to its income to the farmers. Increasing faba bean production and improving yield quality is the major target to meet the demand of the increasing population since faba bean constitutes a major part of the diet [Citation12].
Upon that, this study aims to isolate, characterize, and test the plant growth promoting activity of the bacterial strains co-inhabiting Phaseolus vulgaris nodules via assessing phytohormones, ammonia and siderophores production. Furthermore, their effect, either alone or in combination, will be tested on broad bean growth, pigmentation, nodulation and biochemical parameters.
Materials and Methods
IAA, Chromeazurol S (CAS), and hexadecyltrimethylammonium bromide were all obtained from Sigma-Aldrich. All other chemicals were of analytical grade.
Isolation and identification of bacteria isolated from the root nodules
Healthy Phaseolus vulgaris cultivated plants were carefully uprooted from the clay soil of the field to avoid nodules detaching or bursting. The plant samples were collected from Bani-Abeed, Dakahlia Governorate, Egypt. Under aseptic conditions, the obtained clean nodules were subjected to surface sterilization by 0.2 M HgCl2 in 50% ethanol for 4 minutes. These nodules were further washed by sterilized distilled water and then a sterilized needle was used to cut each nodule in 5 ml sterilized distilled water. Yeast extract mannitol agar media was inoculated with 200 µl of the obtained suspension [Citation13]. The inoculated plates were incubated at 28°C for 24 h. Five strains with different shapes, morphology, and color were obtained and given the names P3, P4, P5, P7, and P8. They were obtained and kept in 30% glycerol stocks at −80°C for further tests.
The DNA of the isolates was extracted according to the procedure described previously [Citation14]. Amplification of the gene was carried out by PCR using primer 5ʹ-AGTTTGATCATGGTCAG-3ʹ and reverse primer 5ʹ-GGTTACCTTGTTACGACT-3ʹ universal primers [Citation15] and the sequence of 16s rRNA was obtained by using DyeEx™ 2.0 Spin Kit (Qiagen PN 63204). The generated sequences were analyzed using Finch TV (version 1.4.0) software, and the phylogenetic tree was generated via Sea view software using the closest published type strain sequences. The isolates were further characterized for their plant growth promoting criteria.
The plant growth promoting criteria of the obtained isolates
Indole acetic acid (IAA) production
Indole acetic acid produced by bacteria was determined as described by [Citation16]. Bacterial cultures were grown in NB amended with tryptophan (0.01 g/l) at 30°C for 48 h in a shaker incubator (120 rpm). After centrifugation, the supernatant was mixed with two drops of o-phosphoric acid and 4 ml of Salkowski reagent. The development of pink color indicated IAA production.
Ammonia production
Bacterial isolates were grown in peptone water and incubated for 72 h at 30°C. Nessler’s reagent (0.5 ml) was added to each tube. The development of brown to orange color was a positive test for ammonia production [Citation17].
Hydrogen cyanide (HCN) production
The nutrient broth was amended with glycine (4.4 g/l), and bacteria were streaked on modified agar plates. Whatman filter paper no. 1 soaked in 2% sodium carbonate in 0.5% picric acid was placed at the inner surface of the lid of the Petri plate. Plates were sealed and incubated at 30°C for 4 days. The development of orange to red color indicated HCN production [Citation18].
Siderophores production
The Chrome Azurol Sulfonate assay (CAS) was used to screen the production of bacterial siderophore. The change of CAS agar medium containing the blue dye complex of CAS, Fe+3 and HDTMA, from blue to orange or dark purplish-red color indicates the Fe-binding compound production [Citation19].
Protease activity
30% skimmed milk-agar media with pH 7.0 was prepared by adding 30 ml packed liquid skim milk (the used skim milk nutrition label on the pack: fats 0.44 g, carbohydrates: 10.6 g, protein: 6.9 g, vitamin: B1 0.1 mg and vitamin: B2 0.14 mg). The plates were streaked by isolates and tightly sealed with parafilm and then incubated at 30°C for 48 h. The formation of a transparent zone around the growing isolates was considered as a positive result [Citation20].
Amylase activity
Amylase production was evaluated on starch agar plates containing (g/l) peptone 5 g, beef extract 3 g, soluble starch 2 g, and agar 15 g [Citation21]. The isolates were streaked on starch agar plates and incubated for 48 hours at 30°C. Amylase production was detected by flooding the plates with iodine solution.
Lipase activity
The cultures were streaked on tributyrene agar (1% tributyrene, 0.5% peptone, 0.3% beef extract and 2% w/v agar) and incubated at 30°C for 72 h. The development of clear zones around the microbial colonies indicated lipase activity [Citation22].
Cellulase activity
The isolates were grown on CMC (Carboxyl methylcellulose) agar containing (g/l) KH2PO4 1 g, MgSO4.7H2O 0.5 g, NaCl 0.5 g, FeSO4.7H2O 0.01 g, MnSO4.H2O 0.01 g, NH4NO3 0.3 g, CMC 10 g and Agar 15 g. The pH was adjusted to 7. The streaked CMC agar plates were incubated at 30°C for 5 days. The medium was flooded with an aqueous solution of Congo red (1% w/v). The formation of a clear zone indicated cellulose degradation [Citation23].
Effect of the bacterial strains on Vicia faba growth and metabolism
A bioassay-based determination of the plant growth promotion ability of the isolates was conducted using Vicia faba seedlings cultivated in a mixture of clay and sand soil (2:1). The used clay soil was with a previous history for Vicia faba cultivation. The study was conducted in the greenhouse of the Faculty of Science, Mansoura University, in normal field conditions of humidity, temperature, light, and day/night patterns. The Vicia faba seeds Giza3 were sterilized in 70% ethanol for 2 minutes followed by 0.2% HgCl2 for 5 minutes and finally washed by sterile distilled water. The pots used in this study were filled with 4 Kg soil and ten seeds were added and left per pot. Before cultivation, the soil was supplied with superphosphate fertilizer (1 g/ pot) and urea (1 g/pot) was considered as a control group, and other seven groups were subjected to bacterial treatments one of them with a single type (P7) and the others with different combinations as follows; P7 + P3, P7 + P4, P7 + P5, P7 + P8, P7 + P4 + P5, and P7 + P3 + P4 + P5 + P8. For all these treatments, the seeds were primed by presoaking with bacterial mentioned treatments for one hour before sowing.
The strains were obtained in LB media incubated at 30°C with 150 rpm for 1–2 days to get O.D = 1. The media effect was eliminated by centrifugation at 6000 rpm for 20 min at 4°C and the cells were resuspended in tap water and the O.D was checked to be = 1. In combined treatments, equal volumes of bacterial cultures were mixed after re-suspension in tap water. The whole experiment was conducted in three independent trials. The plants of each group were harvested after 50 days, and the morphological characteristics of each plant were recorded. The number of nodules, dry shoot and root weights, photosynthetic pigments [Citation24], carbohydrates fractions in shoot and root as glucose [Citation25], sucrose [Citation26] and polysaccharides [Citation27] and nitrogen fractions in shoot and root ammonia nitrogen [Citation28], amino nitrogen [Citation29] and total nitrogen [Citation30]. All the experiments were performed in triplicate. The data collected were statistically analyzed using a completely randomized design in the case of the pot trials. The means were compared using the least significant difference test. The correlation coefficients between a pair of the trait were determined and the significance of the correlation determined using ANOVA. All the statistical tests were performed at p < 0.05, using the software COSTAT.
Studying the interaction between isolates
The treatment (P4+ P5+ P7) was found to cause the maximum level of nodulation, and it was very important for us to study their interaction to pave the way for their co-cultivation in the same fermentation batch.
Generation time and antagonism
First, the growth curve was constructed for the selected isolates to calculate their generation times. After that, the antagonism between them was evaluated on LB plates. The three isolates were inoculated in a cross-over manner, and then the plates were incubated at 28°C overnight.
Co-culture set up and bioreactor batch cultivation
Co-culture set-up was carried out by using two levels of factorial design, via Minitab 17 statistical software, which gave a matrix composed of eight trials. The generated matrix is dependent on a presence/absence methodology, where the (−1) symbol indicates the absence of the isolate in the trial and the (1) symbol indicates the presence of that isolate in the performed trial. Eight flasks containing 100 ml LB medium are inoculated with 0.5 ml of the seed cultures of the tested isolates according to the generated matrix. The OD600 for all the seed cultures were adjusted to be ≈1. The flasks were incubated at 28°C for 48 h with 170 rpm orbital shaking. The runs were centrifuged at 6000 rpm for 15 min, and the pellets were collected and dried for 48 h at 37°C and weighted. A batch run was carried out using Eppendorf – New Brunswick 7 L Rushton turbine Stirred Tank Bioreactor (STR) with a working volume of 5 L. After sterilization, the bioreactor was set up according to the manufacturer’s instructions. From P4, P5, and P7 liquid cultures, 200 ml LB media mixed seed culture containing P4, P5, and P7 were obtained in an OD = 1 after incubation at 28°C to inoculate 5 L LB culture. Batch cultivation was carried out at 28°C, pH 7, and 150 rpm stirring. Culture pH was maintained at 6.5 by automatic addition of acid and base (4 N) by pH–mV controller M 7832 N. Samples were withdrawn at regular time intervals to be analyzed for growth (optical density at 600 nm). Airflow was disabled all over the batch run.
Results
Identification and characterization of the obtained strains
From the root nodules of Phaseolus vulgaris, five bacterial isolates have been obtained (). The 16S rRNA sequence of the isolate P3 showed a high level of sequence identity (99%) to the strain Bacillus amyloliquefaciens () and it has been given the name Bacillus MAP3. The isolate P4 showed a high sequence similarity (99.6%) to Brevibacillus parabrevis () and it has been given the name Brevibacillus MAP4. The isolates P5 and P8 showed a high level of sequence similarity (98.7% and 96.6% respectively) to Pseudomonas putida and Pseudomonas hibiscicola (Figures 3S &43) and the names Pseudomonas MAP5 and Pseudomonas MAP8, respectively, were given to them. All five bacterial isolates are submitted to NCBI with a specific accession number as shown in . P7 isolate showed a high level of sequence similarity (98.3%) to Rhizobium leguminosarum and it has been given the name Rhizobium MAP7 (Fig. 5S).
Table 1. The identification and characterization of plant growth promoting strains (P3, P4, P5, P7 and P8) obtained from Phaseolus vulgaris nodules. (+) mean +ve result and (-) mean – ve result
Figure 2. Time trajectory for growth of the isolates under investigation within bioreactor on the optimized cheap production medium under precise controlled conditions (28°C, pH 7 and 150 rpm stirring). The dissolved oxygen DO was started from 100% and decreased with time. The microbial growth in fermentor is fold than that in flask.
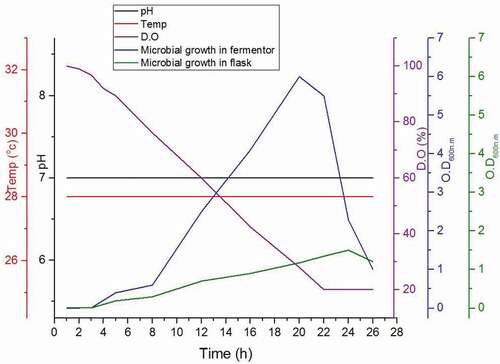
For the plant growth-promoting criteria tested in this study, all the obtained strains showed the ability to produce ammonia, lipase, and cellulase. However, different patterns have been recorded for other traits as shown in . The biochemical characteristics of these isolates, using Vitek2c1 automatic compact system, are recorded as shown in .
Effect of treatment with bacterial strains on plant growth and metabolism
Plants inoculated with the isolated strains were found to be comparably different from control ones in shoot and root lengths, the number of nodules, and shoot and root dry weights. The results, as shown in , revealed that all the designed treatments significantly improved plant growth parameters, particularly the number of nodules, shoot length, and dry weight that showed a significant increase. The number of nodules for plants treated with P7+ P4+ P5 showed four times increase compared to that of the control, and the shoot and root lengths and shoot dry weights were the maximum in response to this treatment too. The highest values of chlorophyll a, chlorophyll b, and carotenoids were recorded for plants subjected to P7+ P5, P7+ P4+ P5, and P7+ P3+ P4+ P5+ P8 treatments respectively as shown in .
Table 2. Effect of bacterial treatments on growth parameters of Vicia faba plant during the pre-flowering stage. P3: Bacillus MP3, P4: Brevibacillus MP4, P5: Pseudomonas MP5, P7: Rhizobium MP7 and P8: Pseudomonas MP8.
Table 3. The effect of bacterial treatments on photosynthetic pigments of Vicia faba during the pre-flowering stage. P3: Bacillus MP3, P4: Brevibacillus MP4, P5: Pseudomonas MP5, P7: Rhizobium MP7 and P8: Pseudomonas MP8.
shows that all treatments led to an increase in glucose content in both shoot and root either significantly or non-significantly. The treatments P7+ P8 and P7+ P4+ P5 led to the highest contents of glucose in shoot and root, respectively. Concerning sucrose concentrations, plants treated with P7+ P3+ P4+ P5+ P8 kept the highest concentration of sucrose in both shoot and root. It was observable that the lowest concentration of sucrose was in response to P7 and P7+ P4+ P5 treatments in shoot and root, respectively. The amount of total soluble sugars decreased in the shoot in response to all treatments, however, root amounts of polysaccharides generally increased either significantly or non-significantly in response to the aforementioned treatments. It was observable that P7+ P4+ P5 treatment kept the highest value of polysaccharide.
Table 4. Effect of bacterial treatments on carbohydrates content of Vicia faba shoot and root during the pre-flowering stage. P3: Bacillus MP3, P4: Brevibacillus MP4, P5: Pseudomonas MP5, P7: Rhizobium MP7 and P8: Pseudomonas MP8.
For nitrogen fractions, as shown in , different bacterial inoculums led to decrease in nitrate amount in shoot either significantly or non-significantly except P7+ P5 treatment that showed a significant increase in this fraction. Root nitrate generally increased in response to different treatments and P7+ P4+ P5 treatment kept the highest nitrate concentration. Ammonia and amino nitrogen concentrations increased in response to almost all treatments in both shoot and root, and it was observable that P7+ P4+ P5 treatment kept the highest ammonia and amino-N contents. The expected total nitrogen values were on the same trend.
Table 5. Effect of bacterial treatments on nitrogenous constitute of Vicia faba shoot and root during the pre-flowering stage. P3: Bacillus MP3, P4: Brevibacillus MP4, P5: Pseudomonas MP5, P7: Rhizobium MP7 and P8: Pseudomonas MP8.
Microbial interaction and co-culture
It is shown that the bacterization with P7+ P4+ P5 led to the highest nodule number along with better physical growth. This result inspired us to study the interaction between these strains to pave the way for producing them together and use the final product as a bio-fertilizer. shows the growth behavior of the isolates even at the cross over sections confirming the absence of any possible antagonism between the tested three microbes. The generation time was found to be 4, 4.1 and 4.3 h for P4, P5 and P7, respectively.
A 2-level factorial design matrix was applied () with eight different trials, each with its own unique combination to study the positive interaction between the targeted strains. The highest dry weight was attained with the trial number (5) where the three isolates (P7+ P4+ P5) were grown together leading to (2.4 g/L) of biomass. The combination of Rhizobium MAP7 (P7), Brevibacillus MAP4 (P4) in the trial number (3) gave the lowest dry weight (0.4 g/L). The data obtained was exposed to a Multi-Way ANOVA analysis to explore the statistical significance of the effect of each main factor and two and three ways interactions on the combination between isolates (). According to Multi-Way ANOVA statistical analysis, the organisms, Brevibacillus MAP4 (P4), Pseudomonas MAP5 (P5) and Rhizobium MAP7 (P7) were found to be the most significant factors, Brevibacillus MAP4 (P4), Pseudomonas MAP5 (P5) and Rhizobium MAP7 (P7) are significantly positive factors but Brevibacillus MAP4 (P4) and Rhizobium MAP7 (P7) are a significantly negative factor, in term of the main effect as the P-value of their main effect was less than α = 0.1, which was used as a cut off to test the significance at 90% level of confidence. Besides, all the tested two ways interactions, and most of the three ways interactions were found to play a significant role in the growth and metabolism of common bean.
Table 6. The obtained biomass (g/L) in co-culturing experiments and Multi-Way ANOVA for the results
A batch run was carried out using Eppendorf – New Brunswick 7 L Rushton turbine, Stirred Tank Bioreactor (STR) with a working volume of 5 L to study the growth kinetics of the culture study growth and determine O.D600 at time intervals and also determine the final dry weight. shows the growth of the combination between P4+ P5+ P7 on the LB medium, the bacterial growth increased and OD600 reached 6 after 22 h compared with that of 1.2 in the case of 1 liter LB media at the flask level.
Discussion
Characteristics of the isolated strains
Four bacterial isolates in addition to rhizobium were isolated from the root nodules of Phaseolus vulgaris plants cultivated in the field. Besides their morphological and biochemical differences, they were found to have different capabilities as plant promoting bacteria.
The isolate P3 obtained in this study, which is phylogenetically very close to Bacillus amyloliquefaciens, was found to be an IAA producer in addition to its ability to produce ammonia, HCN, siderophores, amylase, cellulase protease, and lipase. Additionally, it could sequestrate for its ability to produce siderophores. Bacillus amyloliquefaciens that is belonging to firmicutes is famous for its activity as plant growth promoting rhizobacteria. It has been reported that B. amyloliquefaciens can produce IAA and GA3 [Citation31]. Additionally, it is considered as a potential biocontrol agent against several plant pathogens, such as Fusarium oxysporum [Citation31] because of its ability to produce several volatile organic compounds (VOCs).
Brevibacillus parabrevis, which belongs to firmicutes, is the closest homologue to P4 that is known for its ability to produce IAA, phosphate solubilization in addition to ACC-deaminase activity [Citation32]. This species has been isolated from citrus root [Citation32] as well as the sugarcane rhizosphere [Citation33]. The isolate P4 showed the ability to produce IAA, siderophores, ammonia, and HCN. Hydrolytic activity against cellulose, lipids, and proteins was also recorded for this isolate. These activities would nominate this isolate as plant growth promoting bacteria. Some Brevibacillus species have been recognized as microaerophiles [Citation33].
The isolates P5 and P8 with close homology to Pseudomonas putida and Pseudomonas hibiscicola respectively showed the ability to produce ammonia. Additionally, the isolate P5 was able to produce IAA in addition to its ability to hydrolyze lipid, cellulose, and starch, merits that make it a potential plant growth promoting bacteria. On the other hand, the isolate P8 able to produce siderophore and showed the ability to hydrolyze all the tested macromolecules. Interestingly, both Pseudomonas putida and Pseudomonas hibiscicola were isolated from rice and regarded as potential growth-promoting endophytic bacteria for the ability to produce IAA and solubilize phosphate [Citation34]. Several Pseudomonas species have been isolated from check pea nodules [Citation35] although no report about the isolation of our isolates homologues from nodules. Several reports have shown the significant effect of Pseudomonas putida on rhizobia-legume symbiosis [Citation36,Citation37].
The isolate P7, with close homology to Rhizobium leguminosarum, has been regarded to nodulate a wide range of leguminous hosts. It has been reported to nodulate Phaseolus vulgaris [Citation38] as well as Vicia faba [Citation39]. However, in this study it has been used as a PGPB that would synergize the nodulation of Vicia faba with its specific symbiont present in soil. The obtained P7 isolate has been found to produce IAA, siderophores, and ammonia in addition to its ability to hydrolyze protein, lipid, and cellulose.
Effect of the isolated strains on Vicia faba growth and metabolism
To evaluate their potentiality as plant growth promoting bacteria and to assess their effect on legume nodulation, a pot experiment was designed as mentioned previously. Generally, there is an observed increase in the growth parameters of plants treated with rhizobia, either alone or in combination with other bacteria to various degrees over un-inoculated control. Additionally, the use of the tested PGPB committed a positive effect on root nodules and this is evidenced from the increase in nodulation of the infected plants in case of combined inoculations. The observed increase in nodulation might be attributed to the production of siderophores by the tested PGPB that make iron available, an element that is important for nodule formation [Citation40]. The increase in nodulation was accompanied with an observed increase in fixed nitrogen as well as growth parameters, a fact that has been shown by several studies [Citation41,Citation42]. The increase in nodule was noticeable with an increase in root system ‘the increase in root length & dry weight’ in this study as it has been observed before in chickpea when treated with PGPB in phosphate enriched compost [Citation43]. The increased supply of fixed nitrogen leads to a significant enrichment of different nitrogen fractions as reported in previous studies and in this study. The inoculation of rhizobia either alone or in combined treatments with other bacteria showed, in general, a significant increase in nitrate, ammonia, amino nitrogen and total nitrogen. The combined treatments of P7+ P4+ P5 that showed the highest nodulation results showed the highest values of the aforementioned nitrogen fractions. Treatment of soybean with rhizobia in combination with Serratia proteamaculans and Aeromonas hydrophila showed higher nodulation as well as higher nitrogen contents [Citation42].
Treatment of broad bean plants with rhizobia either alone or in combination with other bacteria led in general to a significant increase in chlorophylls and carotenoids, as well as total pigments. The sustainable supply of the fixed nitrogen that has been attained by increasing nodulation from one side and the ability of some bacterial strains to produce siderophores from the other side may together increase chlorophyll contents [Citation40] as the presence of siderophores enables the presence of iron, which is very important for nodulation.
The photosynthetic capacity of leaves is related to the nitrogen content primarily because of proteins of the Calvin cycle, and thylakoids represent the majority of leaf nitrogen [Citation44]. Nitrogen availability and internal distribution play a critical role in the regulation of various growth-related and morphogenetic aspects of plant development [Citation45]. One of the important factors indicating the efficiency of nitrogen fertilization is the performance of the photosynthetic apparatus that determines photosynthetic pigment contents in leaves [Citation46].
Conclusion
Summering up, Rhizobium is being accompanied by some helpful comrades inside the nodules, those helping it to do its function, which means nodules are not exclusively inhabited by rhizobia. Additionally, these strains showed a good potentiality as plant growth promotors. Treatment of broad bean with a combination of Rhizobium leguminosarum, Brevibacillus parabrevis, and Pseudomonas putida improved growth as well as nodulation, an effect that should improve the agronomical features of this crop. This improvement paves the way to use these tested strains on wider scale and for further figuring out of potential promotors among nodules inhabitants.
Disclosure statement
No potential conflict of interest was reported by the author(s).
References
- Crespi M, Frugier F. De novo organ formation from differentiated cells: root nodule organogenesis. Sci Signal. 2008;1(49):re11–re11.
- Lupwayi N, Clayton G, Hanson K, et al. Endophytic rhizobia in barley, wheat and canola roots. Can J Plant Sci. 2004;84(1):37–45.
- Sy A, Giraud E, Jourand P, et al. Methylotrophic Methylobacteriumbacteria nodulate and fix nitrogen in symbiosis with legumes. J Bacteriol. 2001;183(1):214–220.
- Moulin L, Munive A, Dreyfus B, et al. Nodulation of legumes by members of the β-subclass of Proteobacteria. Nature. 2001;411(6840):948.
- Pandya M, Naresh Kumar G, Rajkumar S. Invasion of rhizobial infection thread by non-rhizobia for colonization of Vigna radiata root nodules. FEMS Microbiol Lett. 2013;348(1):58–65.
- Zakhia F, Jeder H, Willems A, et al. Diverse bacteria associated with root nodules of spontaneous legumes in Tunisia and first report for nifH-like gene within the genera Microbacterium and Starkeya. Microb Ecol. 2006;51(3):375–393.
- Tokala RK, Strap JL, Jung CM, et al. Novel plant-microbe rhizosphere interaction involving Streptomyces lydicus WYEC108 and the pea plant (Pisum sativum). Appl Environ Microbiol. 2002;68(5):2161–2171.
- Martínez-Hidalgo P, Hirsch AM. The nodule microbiome: N2-fixing rhizobia do not live alone. Phytobiomes. 2017;1(2):70–82.
- Sturz A, Christie B, Matheson B, et al. Biodiversity of endophytic bacteria which colonize red clover nodules, roots, stems and foliage and their influence on host growth. Biol Fertili Soils. 1997;25(1):13–19.
- Mendes R, Garbeva P, Raaijmakers JM. The rhizosphere microbiome: significance of plant beneficial, plant pathogenic, and human pathogenic microorganisms. FEMS Microbiol Rev. 2013;37(5):634–663.
- Goswami D, Thakker JN, Dhandhukia PC. Portraying mechanics of plant growth promoting rhizobacteria (PGPR): a review. Cogent Food Agric. 2016;2(1):1127500.
- Zeidan M. Effect of sowing dates and urea foliar application on growth and seed yield of determinate faba bean (Vicia faba L.) under Egyptian conditions. J Agron. 2003;24:93–102.
- Bhatt S, Vyas R, Shelat H, et al. Isolation and identification of root nodule bacteria of mung bean (Vigna radiata L.) for biofertilizer production. Int J Res Pure App Microbiol. 2013;3(4):127–133.
- Joseph S, David WR. Molecular cloning: a laboratory manual. Q Rev Biol. 2001;76(3):348–349.
- Mathews DH, Sabina J, Zuker M, et al. Expanded sequence dependence of thermodynamic parameters improves prediction of RNA secondary structure1. J Mol Biol. 1999;288(5):911–940.
- Banerjee S, Palit R, Sengupta C, et al. Stress induced phosphate solubilization by’Arthrobacter’Sp. And’Bacillus’ sp. isolated from tomato rhizosphere. Aust J Crop Sci. 2010;4(6):378.
- Cappuccino J, Sherman N. Serial dilution agar plating procedure to quantitate viable cells. In: Microbiology: a laboratory manual. 3rd ed. Bedwood: The Benjamin Cummings Publishing Co., Inc; 1992. p. 77–82.
- Lorck H. Production of hydrocyanic acid by bacteria. Physiol Plant. 1948;1(2):142–146.
- Schwyn B, Neilands J. Siderophores from agronomically important species of the Rhizobiacae. Comments Agri Food Chem. 1987;1(2):95–114.
- Chaiharn M, Chunhaleuchanon S, Kozo A, et al. Screening of rhizobacteria for their plant growth promoting activities. KMITL Sci Technol J. 2008;8(1):18–23.
- Kathiresan K, Manivannan S. Amylase production by Penicillium fellutanum isolated from mangrove rhizosphere soil. Afr J Biotechnol. 2006;5(10).
- Sierra G. A simple method for the detection of lipolytic activity of micro-organisms and some observations on the influence of the contact between cells and fatty substrates. Antonie van Leeuwenhoek. 1957;23(1):15–22.
- Stewart J, Stewart C, Heptinstall J. The use of tritiated cellulose in screening for cellulolytic microorganisms. Biotechnol Lett. 1982;4(7):459–464.
- Taylor WI, Achanzar D. Catalase test as an aid to the identification of Enterobacteriaceae. Appl Microbiol. 1972;24(1):58–61.
- Glickmann E, Dessaux Y. A critical examination of the specificity of the salkowski reagent for indolic compounds produced by phytopathogenic bacteria. Appl Environ Microbiol. 1995;61(2):793–796.
- Singh N, Luthra R. Sucrose metabolism and essential oil accumulation during lemongrass (Cymbopogon flexuosus Stapf.) leaf development. Plant Sci. 1988;57(2):127–133.
- Thayermanavan V. Qual Plant Foods Hum Nutr., 34, 253-257. In: Sadasivam S, Manickam A, editors. Quoted from biochemical methods. New Delhi: New ag. inter.” Limit. Publ; 1984. p. 11–12.
- El-Saht H, Hasaneen M, Bassyoni F. Effects of metribuzin herbicide on nitrogen, pigments, protease and nitrate reductase activity of normal and NaCl-stressed castor bean and maize plants. Biol Plant. 1994;36(2):267.
- Muting D, Kaiser E. Spectrophotometric method of determining of-amino-N in biological materials by means of the ninhydrin reaction. Hoppe-Seyler’s Zeitschrift für Physiologische Chemie. 1963;332:276–289.
- Chibnall A, Rees M, Williams E. The total nitrogen content of egg albumin and other proteins. Biochem J. 1943;37(3):354.
- Yuan J, Ruan Y, Wang B, et al. Plant growth-promoting rhizobacteria strain Bacillus amyloliquefaciens NJN-6-enriched bio-organic fertilizer suppressed Fusarium wilt and promoted the growth of banana plants. J Agric Food Chem. 2013;61(16):3774–3780.
- Trivedi P, Spann T, Wang N. Isolation and characterization of beneficial bacteria associated with citrus roots in Florida. Microb Ecol. 2011;62(2):324–336.
- Ratón T, Yano R, Rodríguez Gámez O, et al. Isolation and characterisation of aerobic endospore forming Bacilli from sugarcane rhizosphere for the selection of strains with agriculture potentialities. World J Microbiol Biotechnol. 2012;28(4):1593–1603.
- Nhu V, Diep C. Isolation, characterization and phylogenetic analysis of endophytic bacteria in rice plant cultivated on soil of Phu Yen Province, Vietnam. Am J Life Sci. 2014;2(3):117–127.
- Gopalakrishnan S, Srinivas V, Prakash B, et al. Plant growth-promoting traits of Pseudomonas geniculata isolated from chickpea nodules. 3 Biotech. 2015;5(5):653–661.
- Grimes HD, Mount MS. Influence of Pseudomonas putida on nodulation of Phaseolus vulgaris. Soil Biol Biochem. 1984;16(1):27–30.
- Rosas SB, Andrés JA, Rovera M, et al. Phosphate-solubilizing Pseudomonas putida can influence the rhizobia–legume symbiosis. Soil Biol Biochem. 2006;38(12):3502–3505.
- Dar GH, Zargar M, Beigh G. Biocontrol of Fusarium root rot in the common bean (Phaseolus vulgaris L.) by using symbiotic Glomus mosseae and Rhizobium leguminosarum. Microb Ecol. 1997;34(1):74–80.
- Zahran H, Sprent J. Effects of sodium chloride and polyethylene glycol on root-hair infection and nodulation of Vicia faba L. plants by Rhizobium leguminosarum. Planta. 1986;167(3):303–309.
- Tang C, Robson AD, Dilworth MJ. The role of iron in nodulation and nitrogen fixation in Lupinus angustifolius L. New Phytol. 1990;114(2):173–182.
- Elkoca E, Kantar F, Sahin F. Influence of nitrogen fixing and phosphorus solubilizing bacteria on the nodulation, plant growth, and yield of chickpea. J Plant Nutrit. 2007;31(1):157–171.
- Zhang F, Dashti N, Hynes R, et al. Plant growth promoting rhizobacteria and soybean [Glycine max (L.) Merr.] nodulation and nitrogen fixation at suboptimal root zone temperatures. Ann Bot. 1996;77(5):453–460.
- Shahzad S, Khalid A, Arshad M, et al. Integrated use of plant growth promoting bacteria and P-enriched compost for improving growth, yield and nodulation of chickpea. Pakistan J Bot. 2008;40(4):1441–1735.
- Shepherd M, McLean S, Hunter CN. Kinetic basis for linking the first two enzymes of chlorophyll biosynthesis. FEBS J. 2005;272(17):4532–4539.
- Vadeboncoeur Y, Devlin SP, McIntyre PB, et al. Is there light after depth? Distribution of periphyton chlorophyll and productivity in lake littoral zones. Freshwater Sci. 2014;33(2):524–536.
- Kopsell DA, Kopsell DE, Lefsrud MG, et al. Variation in lutein, β-carotene, and chlorophyll concentrations among Brassica oleracea cultigens and seasons. HortScience. 2004;39(2):361–364.