ABSTRACT
Retinoic acid (RA), an active metabolite of vitamin A, plays essential signaling roles in mammalian embryogenesis. Nevertheless, it has long been recognized that overexposure to vitamin A or retinoic acid causes widespread teratogenesis in rodents as well as humans. However, the mechanism of its teratogenic effect is not yet understood. Here, we examined the effects of RA on the fetal and placental development as an attempt to find the mechanism of its teratogenicity through inhibition of utero-placental development. The pregnant mice were divided into three groups: RA treated group, control treated with dimethyl sulfoxide (DMSO) group and control non-treated group. The RA treated group received 15 mg/kg RA by intraperitoneal injection from 8.5 to 11.5 gestation days. The pregnant mice were sacrificed by cervical dislocation on days 9.5, 10.5, 12.5, 13.5 and 18.5 of gestation. The uteri were isolated, and the fetuses have been exposed to morphological examination and skeletal staining. Moreover, histological and immunohistochemical studies on placenta have been done. Morphologically, we recorded a reduction in the number of implanted fetuses besides hematoma in different parts of the uteri of the RA treated animals. Furthermore, RA was found to induce decreases in fetal weight and length, as well as malformations in the tail, forelimbs and hindlimbs, which were confirmed by skeletal examination. Interestingly, RA treatment showed a decrease in the expression level of epidermal growth factor receptor (EGFR) during placenta development, especially in junctional and labyrinth zones. We concluded that high dose of RA may have a direct teratogenic effect on the fetus besides an indirect effect through modulating EGFR signal during the placenta development.
Introduction
The placenta is a transient, but indispensable, organ during mammalian gestation [Citation1,Citation2]. Its functions range from nutrient and gaseous exchange to hormone and growth factor production to support normal fetal development [Citation3,Citation4]. In mice, the establishment of placental circulation gestation day (GD) 9.5 permits oxygen and nutrient delivery through the fetal vasculature to the growing embryo. The placental and embryonic circulatory system is regulated by the coordinated action of the genetic and physiologic factors [Citation5]. The murine placenta consists of three major cell populations: labyrinth trophoblasts, spongiotrophoblasts, and trophoblastic giant cells [Citation3]. The innermost labyrinth layer is composed of syncytial trophoblasts, which surround fetal blood vessels that invade the chorionic plate and interdigitate with maternal blood sinuses to enable nutrient and gas exchange. The intermediate layer is composed of the diploid spongiotrophoblasts. The outermost polyploid giant cells, derived from diploid trophoblasts in the ectoplacental cone, form the interface between maternal and embryonic tissues [Citation6].
Retinoic acid (RA), a bioactive vitamin A metabolite, is a signaling molecule, tightly regulated during embryogenesis; indispensable for the formation of many organs, including body axis, spinal cord, eyes, limbs, heart, and kidneys [Citation7–10]. RA is also a valuable compound in the therapy of cystic acne among other numerous dermatologic disorders [Citation11–13]. In target cells, RA acts as a ligand for nuclear retinoic acid receptors (RARs), which form heterodimers with retinoid X receptors (RXRs). The complex binds to a regulatory DNA segment, the retinoic acid response element (RARE), to control transcription of RA target genes. Maternal retinoic acid excess or deficiency during pregnancy cause abnormalities both in human infants [Citation9,Citation14] and in rodents [Citation15], indicating that retinoic acid levels must be within a specific range for normal development [Citation16]. Rat fetuses in mothers reared on vitamin A-deficient diets demonstrate an array of anomalies collectively known as ‘fetal vitamin A deficiency’ (VAD) syndrome, which comprises hind-brain, eye, ear, heart, lung, diaphragm, kidney, testis, limb and skeletal defects [Citation17]. Similarly, mice with compound null mutations of RA nuclear receptors [Citation18,Citation19] and RA-synthesizing enzymes have malformations resembling the VAD syndrome [Citation20]. Notably, excess vitamin A/RA in humans and animal models causes malformations resembling the fetal VAD syndrome [Citation21,Citation22].
Epidermal growth factor receptor (EGFR) is a receptor tyrosine kinase that couples the binding of extracellular ligands, such as EGF and transforming growth factor-α (TGF-α), to the initiation of intracellular signaling pathways [Citation23]. EGFR plays a role in many aspects of female reproduction and pregnancy. EGFR is expressed in the uterine stroma where it regulates not only uterine development but also embryo implantation. During implantation, expression of EGFR and ligands is observed in the uterus at the site of blastocyst attachment as well as on the surface of the implanting blastocyst [Citation24,Citation25]. In addition, EGFR activation is crucial for embryogenesis and organogenesis, and impairment of EGFR signaling during organogenesis may result in respective defect in different organs [Citation26–30]. EGFR regulates the growth and differentiation of the placenta. Egfrtm1Mag nullizygous embryos exhibit strain-dependent placental defects that range from minor reduction of the spongiotrophoblast layer to severe labyrinth dysmorphogenesis [Citation27,Citation31,Citation32]. The mice with increased levels of EGFR signaling during placental development using the hypermorphic EgfrDsk5 allele resulted in larger placental size with a more prominent spongiotrophoblast layer and increased expression of glycogen cell-specific genes [Citation32]. RA administration has been found to decrease the level of expression and the phosphorylation of the EGF receptor in human trophoblast cell cultures (in vitro), suggesting that retinoids could modulate the effect of this growth factor during growth and differentiation [Citation33]. Moreover, RA inhibited EGFR activity in human glioma cells [Citation34].
However, the mechanism of RA teratogenic effect is not yet understood. Here, we examined the effects of RA on the fetal and placental development in an attempt to find the mechanism of its teratogenicity by modulating the EGFR signal in vivo during the utero-placental development.
Materials and methods
Materials
Experimental design
The experiment was approved by the Ethics Committee for Animal Experimentation of the Faculty of Science of Al-Azhar University. Adult female and male albino mice (Swiss strain) were obtained from the Animal House of Faculty of Medicine, Assiut University. The environment in which Swiss mice were housed throughout the experiment is kept under rigorous control at the Animal House of Faculty of Science, Al-Azhar University, Assiut, with the availability of adequate food and water. Adult virgin female mice (25–27 g) at an age of 8–10 weeks were mated with fertile males at an age of 5–6 months and tested for the presence of a vaginal plug. The morning of vaginal plug was designated as day 0.5 of gestation. Test compound retinoic acid (RA) was purchased from Sigma (St. Louis, MO, USA). Stock solution of RA was prepared by dissolving it into dimethyl sulfoxide (DMSO) at a dose of 15 mg/kg according to Collins et al., 1994 [Citation35] diluted with sunflower oil for in-vivo experiments. A stock solution of RA was kept in aliquots at 8°C in the dark bottle. The pregnant mice (n = 75) were divided into three groups of 25 mice each; (1) RA treated group which received 15 mg/kg dose of RA, (2) control treated with DMSO diluted with sunflower oil group, and (3) control non-treated group. Each group was subdivided into five subgroups of five mice each. The RA and control with DMSO treated groups were received a daily intraperitoneal injection dose with a volume of 0.2 ml on gestation days 8.5, 9.5, 10.5 and 11.5 at darkroom being for each group. The animals were killed by cervical dislocation on days 9.5, 10.5, 12.5, 13.5 and 18.5 of gestation.
Both uterine horns were removed and photographed. On day 18.5 of gestation, the number of live fetuses was recorded from the control (n = 50), and RA treated (n = 25) groups, as well as their weights and lengths.
Live, dead and resorped fetuses on gestation day 18.5 of RA treated group:
Histology and immunohistochemistry
The implantation sites of uterine horns were fixed in Carnoy’s fluid for 2 hours and directly dehydrated in absolute ethyl alcohol for about three times (15 min each) then cleared in methyl benzoate for three times (8 hours each) then embedded in paraffin wax at 60°C in oven for three times (2 hours each). Paraffin wax blocks were sectioned at 7µ thickness by Leica RM microtome, and then sections were mounted on glass slides, dewaxed by xylene, rehydrated by descending ethyl alcohol (100%, 90%, 70%, 50% and distilled water) and stained with Hematoxylin and Eosin (Hx & E), and then dehydrated by ascending series of ethyl alcohol (50%, 70%, 90% and 100%) and cleared by xylene and mounted by DPX [Citation36]. Stained sections were photographed by Tucson image acquisition camera.
Immunohistochemical investigations were performed on tissue preparations embedded in paraffin using the EconoTek Kit (code: 800–729-8350, EconoTek Kit, ScyTek Laboratories company, Logan, USA) and Horseradish peroxidase (HRP) method. Endogenous peroxidase activity was quenched by means of treatment with 3% H2O2 (v/v) for 5 min. The preparations were rinsed twice for 5 min in 0.1 M phosphate buffer saline (PBS). To prevent nonspecific staining, the sections were incubated in a protein blocking solution (EconoTek Super block) for 10 min at room temperature. Subsequently, the preparations were incubated with primary antibody (Anti-EGFR Rabbit polyclonal IgG, Spring Bioscience, Canada, 1:200 dilution in phosphate buffer) for 2 h at room temperature. After being washed twice for 5 min in 0.1 M PBS, the preparations were this time incubated with biotinylated secondary antibodies (EconoTek Biotinylated Anti-Polyvalent) for 30 min at room temperature and were once again washed twice for 5 min in 0.1 M PBS. Later, the preparations were incubated in HRP conjugate for 30 min and rinsed for a final twice for 5 min in 0.1 M PBS. In order to visualize the reaction, the preparations were treated with 303-diaminobenzidine hydrochloride (DAB) for 5–15 min. The sections were dehydrated and cleared by xylene [Citation37]. The slides were mounted by DPX, and the sections were examined and photographed using a Tucson image acquisition camera.
Image analysis
Tissue sections stained by immunohistochemistry were visualized by light microscopy, and digital images were captured using a Tucson image acquisition camera. The digitized images were analyzed using Imaging J software. Ten areas of decidual basalis (DB), junctional zone (JZ) and labyrinth zone (LB) were analyzed to determine the optical density of positive staining (average positive areas) in each area. At least two utero-placental units from two to three pregnant mice of each group were analyzed on days 9.5, 10.5, 12.5, 13.5 and 18.5 of gestation.
Skeleton staining
Opened lengthwise to expose the gestational sacs for the removal of live fetuses. Fetuses who suffered late fetal death and reabsorptions, when present, were also removed. Fetuses were examined externally by stereomicroscope, photographed and fixed in absolute ethanol, defatted with acetone, again passed through 95% ethanol, and transferred to 2% potassium hydroxide until the bones became visible. The fetuses were then stained with 5% Alizarin Red prepared in 1% potassium hydroxide until the bones were stained red. Next, the fetuses were placed in 1:1 glycerin and 1% potassium hydroxide, and then placed in a pure glycerin solution, analyzed under a stereoscopic microscope and photographed.
Statistical analysis
The computer program, the statistical package for social science (SPSS 25), was used for statistical analysis. Statistical analysis was performed using Student’s t test. P values less than 0.05 and 0.01 were considered to be statistically significant.
Results
Effect of RA on pregnancy outcomes
Since results from both control groups (non-treated and dimethyl sulfoxide (DMSO) treated groups) were almost the same, we here referred the control pregnant mice to both groups.
The uteri of the control pregnant mice showed a normal distribution of the live fetuses between the two horns, with an increase in the size of implantation sites gradually from day 9.5 to day 13.5 of gestation. In RA treated group, the uterine horns showed clearly visible hematoma at different parts and abnormal size of some implantation sites ()). Moreover, there are clearly visible fetal resorption sites at 18.5 days of gestation ()). When we treated with RA daily on days 8.5, 9.5, 10.5 and 11.5 of gestation, our results showed that there was a significant (P ˂ 0.01) reduction in the number of live fetuses on day 18.5 of gestation from RA treated group ()) indicating that some fetuses may be resorbed or miscarriaged (data not shown). The fetuses from the control group had normal body weights and lengths. On the other hand, RA treatment caused a significant (P ˂ 0.05) reduction in fetal body weights and lengths (growth retardation) when compared with the control group ().
Figure 1. (a) Photographs of the mice uteri on gestation days (GDs) 9.5, 10.5, 12.5, 13.5 and 18.5 in control and retinoic acid (RA) treated groups. Hematoma (white arrows), abnormal size of implantation sites (black arrows) and resorped fetuses (arrowheads). (b, c and d) Histograms represent the mean number of live fetuses, the mean of fetal lengths and fetal weights, respectively on GD 18.5 of control and RA treated groups. *P < 0.05, **P < 0.01 statistically different.
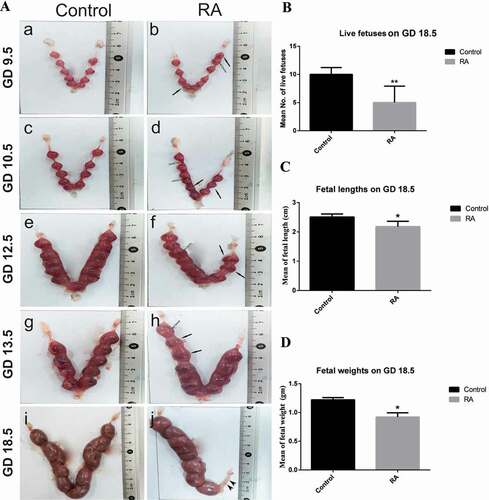
Morphological examination of the fetuses from control group on day 18.5 of gestation showed normal morphology ()), while the fetuses from RA treated group showed some malformations such as curved body, small head size, fore-and hind-limbs defects, opened eye, short tail and syndactyly ().
Figure 2. Photographs of mice fetuses on gestation day 18.5 showing external morphology. (a) normal fetus with correct shape and length from control group. (b), (c) and (d) the feuses from RA treated group showing some malformations as curved body, small head size, fore-and hind-limbs defects, opened eye, short tail and syndactyly.
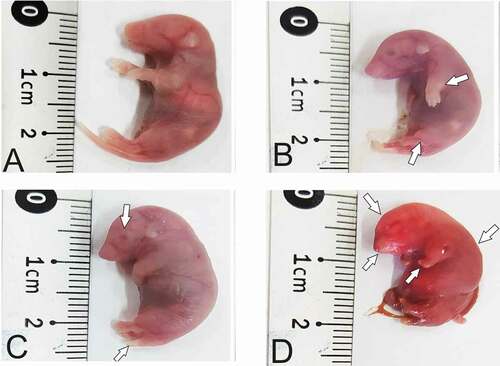
Next, the morphological malformations of the fetuses from RA treated group permitted us to examine the skeletal anomalies of the fetuses on day 18.5 of gestation, ossification process of the axial and appendicular skeletons in the control mice fetuses mostly has been obviously completed ()). The fetal skeleton from maternal RA treated group revealed incomplete ossification and non-ossified different parts like vertebral centrum thoracic, vertebral centrum lumbar and vertebral centrum sacral ()). The skeletal anomalies mostly appeared as incomplete ossification of the frontal, parietal, and interparietal ()), occipital condyles agenesis ()), caudal vertebrae agenesis ()), scapula and humerus hypoplasia ()), radius, ulna, carpus, meta carpus agenesis ()), femur hypoplasia ()), tibia, fibula, tarsus, meta tarsus agenesis ()), pelvic hypoplasia ()).
Figure 3. Photographs of the fetal skeletons of control and (RA) treated groups on gestation day 18.5. Normal ossification of the fetal skeleton from the control group (a,c,e,g and i). The fetal skeleton from (RA) treated group showing that incomplete ossification the skull (d and f), occipital condyles agenesis (f), caudal vertebrae agenesis (j), scapula and humerus hypoplasia (h), radius, ulna, carpus, meta carpus agenesis (h), femur hypoplasia (j), tibula, fibula, tarsus, meta tarsus agenesis (j), pelvic hypoplasia (b). Carpal (C), caudal vertebrae (Cv), fibula (E), femur (Fe), frontal (Fr), inter parietal (Ip), meta carpal (Mc), meta tarsus (Mt), occipital condyle (OC), parietal (p), pelvic element (Pe), radius (R), scapula (S), tarsus (T), tibia (Tb), ulna (U), vertebral centrum lumbar (Vcl), vertebral centrum sacral (Vcs), vertebral centrum thoracic (Vct). Alizarin staining. White arrows point to skeleton malformation (the analogous structure of control).
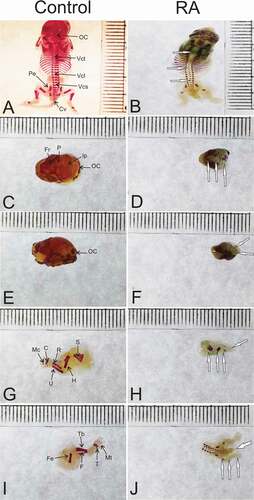
Effects of RA on the utero-placental development
Histologically, on gestation day 9.5 the placenta became mature and consisted of two parts: maternal and fetal parts, the maternal part consisted of maternal triangle and decidual basalis, the decidual basalis was composed of regression of mesometrial decidua, containing decidual cells, fibers, and vascular channels. The fetal part consisted of three layers: the trophoblastic giant cell layer, the spongiotrophoblast layer, and the labyrinth layer. Trophoblastic giant cells, identified by their large nuclei, were present as a layer separating the spongiotrophoblasts from decidual basalis, and the spongiotrophoblast layer consisted of large trophoblast cells with small blood sinuses. Both the trophoblast giant cells and spongiotrophoblast cells form the junctional zone. The labyrinth layer is formed by contact between the chorionic trophoblast and allantoic mesoderm, and contains blood sinuses ().
Figure 4. Photomicrographs of the transverse section of the mouse utero-placental unit on gestation day (GD) 9.5 from control (a, e, i, c, g, k) and RA treated (b, f, j, d, h, l) groups. (a, e, i) Histological structure of the utero-placental unit, maternal triangle (MT), decidual basalis (DB), junctional zone (JZ), labyrinth zone (LB). (e) a magnified part of the decidual basalis, (i) a magnified part of the junctional zone (JZ), labyrinth zone. (b, f, and j) Showing effect of RA treatment on the developing utero-placental unit, note that irregular shape with reduction of the thickness of both the junctional and labyrinth zones (Hx & E staining). (c, g, and k) illustrate the immunohistochemical localization of EGFR of the utero-placental unit from control group, note that EGFR is expressed at high level in the boundary and blood sinuses of decidual basalis and the junctional zone, and at low level in the labyrinth zone. (d, h, and l) showing the effect of RA on the expression level of EGFR. note that EGFR is expressed in the decidual basalis and the junctional zone at moderate level, while the labyrinth zone is devoid of immunoreaction. (m) Optical density of EGFR-positive areas in decidual basalis (DB), junctional zone (JZ) and labyrinth zone (LB). Density is expressed as the number of positive areas. **P < 0.01 compared with the control group. Allantois (All), Blood sinuses (BS), chorion (Ch) and trophoblast giant cell (TGC). Scale bar (a–d): 100 µm, (e–l): 50 µm.
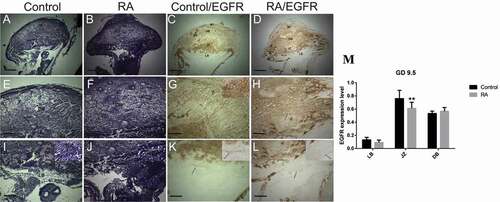
On gestation day 10.5, there was no change in the thickness of the decidual basalis, while junctional and labyrinth zones were developed compared to that of the previous stage (GD 9.5) (). On gestation day 12.5, the decidual basalis was regressed, while the junctional zone and labyrinth zones were developed more. The fetal mesenchyme progressively invaded the trophoblast cells and established a widespread network of fetal blood vessels in the developing labyrinth (). On gestation day 13.5, the thickness of decidual basalis was reduced more. In contrast, the junctional zone became well developed and reached its maximum development. The labyrinth zone showed continuous growth (). By the end of gestation day 18.5, the decidual basalis was restricted to a thin layer, thickness of junctional zone was reduced also, indicating that both decidual basalis and junctional zone have been degraded during the late placental development, while the labyrinth zone became widespread with extensive area of blood sinuses, indicating its continuous growth and development ().
Figure 5. Photomicrographs of the transverse sections of the mice utero-placental units on gestation day (GD) 10.5 from control (a, e, i, c, g, k) and RA treated (b, f, j, d, h, l) groups. (a, e, i) Histology of the developed utero-placental unit from the control group showing, reduction of the thickness of decidual basalis (DB), and increase of the thickness of both junctional zone (JZ) and labyrinth zone (LB) comparing to the previous stage (GD9.5). (e) a magnified part of the decidual basalis and junctional zone (JZ). (i) a magnified part of labyrinth zone. (b, f, and j) Showing effect of RA treatment on the utero-placental development, note that irregular shape of placenta with inhibition of the development of both the junctional and labyrinth zones (Hx & E staining). (c, g, and k) illustrate the immunohistochemical localization of EGFR of the utero-placental unit from control group, note that EGFR is expressed at high level in the decidual basalis and the junctional zone, and at moderate level in the labyrinth zone. (d, h, l) showing the effect of RA on the expression level of EGFR. note that EGFR is expressed at moderate level in the decidual basalis and the giant cell layer of junctional zone, while the rest of junctional zone and labyrinth zone are devoid of immunoreaction. (m) Optical density of EGFR-positive areas in decidual basalis (DB), junctional zone (JZ) and labyrinth zone (LB). Density is expressed as the number of positive areas. **P < 0.01 compared with the control group. Blood sinuses (BS). Scale bar (a–d): 100 µm, (e–l): 50 µm.
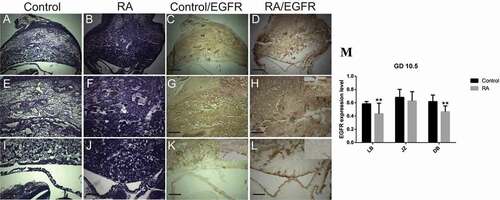
Figure 6. Photomicrographs of the transverse sections of the mice utero-placental units on gestation day (GD) 12.5 from control (a, e, i, c, g, k) and RA treated (b, f, j, d, h, l) groups. (a, e, i) Histology of the developed utero-placental unit from the control group showing, reduction of the thickness of decidual basalis (DB), while the thickness of junctional zone (JZ) and labyrinth zone (LB) is increased comparing to the previous stage (GD10.5). (e) a magnified part of the decidual basalis and junctional zone. (I) a magnified part of labyrinth zone. (b, f, and j) Showing effect of RA treatment on the utero-placental development, note the placenta is abnormal, with inhibition of the development of the labyrinth zones (white arrows), which is not demarcated from the junctional zone (Hx & E staining). (c, g, and k) illustrate the immunohistochemical localization of EGFR of the utero-placental unit from control group, note EGFR is weakly expressed in decidual basalis and junctional zone, and strongly expressed in the labyrinth zone. (d, h, l) showing the effect of RA on EGFR expression level; note RA induces reduction of EGFR expression level in decidual basalis(DB), junctional zone (JZ), and labyrinth zone (LB). (M) Optical density of EGFR-positive areas in decidual basalis (DB), junctional zone (JZ) and labyrinth zone (LB). Density is expressed as the number of positive areas. *P < 0.05 compared with the control group. Blood sinuses (BS). Scale bar (a–d): 100 µm, (e–l): 50 µm.
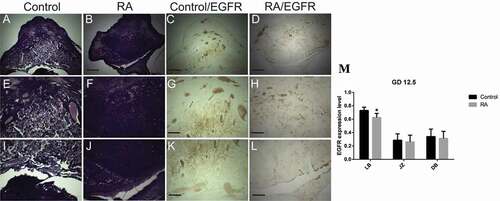
Figure 7. Photomicrographs of the transverse sections of the mice utero-placental units on gestation day (GD) 13.5 from control (a, e, i, c, g, k) and RA treated (b, f, j, d, h, l) groups. (a, e, i) Histology of the developed utero-placental unit from the control group showing, reduction of the thickness of both decidual basalis (DB) and junctional zone (JZ), while the thickness of the labyrinth zone (LB) is increased comparing to the previous stage (GD10.5). (e) a magnified part of the decidual basalis and junctional zone (JZ). (i) a magnified part of labyrinth zone. (b, f, and j) Showing effect of RA treatment on the utero-placental development, note small size of the placenta with inhibition of the development of the labyrinth zones (Hx & E staining). (c, g, and k) illustrate the immunohistochemical localization of EGFR of the utero-placental unit from control group, note weak expression of EGFR in decidual basalis and junctional zone, while in labyrinth zone the expression is strong. (d, h, l) showing the effect of RA on EGFR, the expression level of EGFR is moderate in decidual basalis (DB), junctional zone (JZ), and labyrinth zone (LB). (M) Optical density of EGFR-positive areas in decidual basalis (DB), junctional zone (JZ) and labyrinth zone (LB). Density is expressed as the number of positive areas. *P < 0.05, **P < 0.01 compared with the control group. Scale bar (a–d): 100 µm, (e–l): 50 µm.
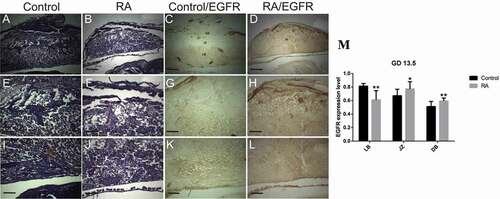
Figure 8. Photomicrographs of the transverse sections of the mice placentae on gestation day (GD) 18.5 from control (a, e, i, c, g, k) and RA treated (b, f, j, d, h, l) groups. (a, e, i) Histology of the placenta from the control group showing, reduction of the thickness of both decidual basalis (DB) and junctional zone (JZ), while the thickness of the labyrinth zone (LB) is increased comparing to the previous stage (GD13.5). (e) a magnified part of the spongiotrophoblast layer (ST). (i) a magnified part of labyrinth zone. (b, f, and j) Showing effect of RA treatment on placental development, note small size of the placenta with inhibition of the development of the labyrinth zones (Hx & E staining). (c, g, and k) illustrate the immunohistochemical localization of EGFR of the placenta from control group, note weak expression of EGFR in spongiotrophoblast layer, while in labyrinth zone the expression is strong. (d, h, l) showing the effect of RA on EGFR, the expression level of EGFR is moderate in spongiotrophoblast layer (ST), and labyrinth zone (LB). (m) Optical density of EGFR-positive areas in spongiotrophoblast layer (ST) and labyrinth zone (LB). Density is expressed as the number of positive areas. **P < 0.01 compared with the control group. Scale bar (a–d): 100 µm, (e–l): 50 µm.
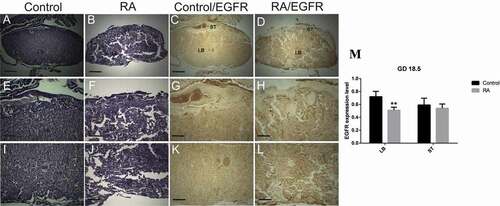
In RA treated group, our results showed that RA induced irregular shape of placenta with defects that range from minor reduction of the spongiotrophoblast and labyrinth layers to severe labyrinth dysmorphogenesis; RA induced inhibition of the development of both the junctional and labyrinth zones with less numerous and small blood sinuses compared to control group on gestation day 9.5 (). On gestation day 10.5, the placentae from RA treated group were abnormal in shape, especially at junctional zone which was not clearly demarcated from the labyrinth zone with less numerous and small blood sinuses compared to that of the control group (). Moreover, on gestation day 12.5, there was no clear differentiation of the fetal layers with inhibition of blood sinus formation. Interestingly, RA induced failure of the allantois to fuse with the chorion (). On gestation days 13.5 and 18.5, the placentae from RA treated group showed small sizes with a gross reduction in the fetal vasculature compared to those of the control ().
Effects of RA on EGFR during the utero-placental development
On day 9.5 of gestation, the utero-placental unit from control group showed that, in the decidual basalis, EGFR was expressed strongly in non-decidualized cells (fibroblasts) and some rounded cells of the central area and close to the smooth muscle coat. Strong signaling was also detected in the blood sinuses, while decidualized cells were negative. Moreover, EGFR was expressed strongly in the junctional zone, while in the labyrinth zone, the expression was weak (). By day 10.5 of gestation, the expression pattern of EGFR in the decidual basalis was stronger especially in fibroblast cells and endothelial cells of blood sinuses similar to that observed on day 9.5 of gestation. Strong signaling was also detected in the blood sinuses of the junctional zone, while in the spongiotrophoblasts, the expression was weak to moderate, and in the labyrinth zone, the expression was increased to moderate level (). Moreover, on gestation day 12.5, the strong signal of EGFR was restricted to the labyrinth zone, while the decidual basalis and junctional zone were negative for EGFR with the exception that the blood sinuses exhibited strong signaling for EGFR (). On gestation day 13.5, the expression pattern of EGFR in the decidual basalis and junctional zone was similar to that on gestation day 12.5, interestingly, the expression of EGFR was strong with widespread in the developed labyrinth layer (). By the end of gestation on day 18.5, the expression of EGFR was strong and restricted to the well-developed labyrinth layer ().
In RA treated group, on day 9.5 of gestation, the utero-placental unit showed that the expression of EGFR was negative in spongiotrophlasts and trophoblast giant cells of the junctional zone and labyrinth zone (), indicating that RA induced a reduction of EGFR expression level in the junctional and highly significant decrease in labyrinth zones. Moreover, the inhibitory effect of RA on the expression of EGFR was also appeared on day 10.5 of gestation, resulted in a significant reduction of the expression of EGFR in decidual basalis to moderate level, inhibition of EGFR in the junctional zone and highly significant decrease in labyrinth zone except the apical region of the labyrinth zone which showed a moderate-to-strong signaling (). By day 12.5 of gestation, the EGFR expression was mostly inhibited in decidual basalis, junctional zone, and significant decrease in labyrinth zone, except the blood sinuses in decidual basalis and some dispersed cells exhibited reactivity for EGFR ().
On gestation days 13.5 and 18.5, the placentae from RA treated group with minor defects showed that EGFR was expressed at moderate level throughout the undifferentiated layers (), indicating that the development of utero-placental unit was delayed.
Discussion
It has been shown that maternal RA excess during pregnancy causes abnormalities in human infants [Citation14] as well as in rodents [Citation15]. However, the mechanism of RA teratogenic effect is not yet understood. In this study, we examined the effects of RA on fetal and placental development in an attempt to find the mechanism of its teratogenicity by modulating the EGFR signal during the utero-placental development. In general, we found that RA induced small size of implantation sites and fetal resorption or miscarriage. In addition, RA induced morphological fetal malformation and placental abnormality. Moreover, RA inhibited the expression level of EGFR during utero-placental development.
First, we examined the effect of RA during mid-gestation at a dose of 15 mg/kg on pregnancy outcomes, and the uterine horns showed clearly visible hematoma at different parts with reduction in the sizes of the implantation sites. Moreover, RA induced fetal reabsorption or miscarriage during late stages of pregnancy, which in turn led to a reduction in the number of implanted fetuses as shown previously as a single intraperitoneal injection of RA (70 mg/kg) on the eighth gestational day of the mice induced reabsorptions [Citation38], indicating the fetal lethality or miscarriage of the RA treated group that could be related to the placental defects.
The current study showed that the RA treatment caused a significant (P˂ 0.05) reduction in fetal body weight and length (growth retardation). It coincides with the previous study, which showed that the general development of RA treated embryos was retarded [Citation16]. Morphological examination of the fetuses on day 18.5 of gestation from RA treated group showed some malformations such as curved body, small head size, fore-and hind-limbs defects, opened eye, short tail and syndactyly. According to a previous study, a 15 mg/kg/day of RA is an embryotoxic dose for rodents [Citation39]. The morphological features of RA treated embryos have been described in more detail [Citation38,Citation40].
The fetal skeletons from maternal RA treated groups revealed incomplete ossification and non-ossified different parts, the skeletal anomalies mostly appeared as incomplete ossification of the frontal, parietal, and interparietal, occipital condyles agenesis, caudal vertebrae agenesis, scapula and humerus hypoplasia, radius, ulna, carpus, meta carpus agenesis, femur hypoplasia, tibia, fibula, tarsus, meta tarsus agenesis, pelvic hypoplasia. As shown previously, the skeletal examination of the diaphanized fetuses showed bone changes, especially of the forelimbs, hindlimbs, pelvic hypoplasia, and tail agenesis in mice [Citation38] and chick [Citation7,Citation8]. Next, the morphological malformations and skeletal anomalies of the fetuses from RA treated groups permitted us to investigate the mechanism of RA teratogenic effect, either through the placental abnormality or direct effect on the fetus.
During pregnancy, the utero-placental unit undergoes a series of programmed morphological changes resulting in an extensive tissue reorganization in order to accommodate the growing fetus. These changes include sequential processes of growth, differentiation, and regression in different regions of the placenta. Here, we demonstrated that the utero-placental unit consists of four layers: maternal triangle, decidual basalis, junctional zone and labyrinth zone as shown by Cross et al. (1994). The decidual basalis was developed and reached its maximum development on gestation day 10.5, regressed by day 12.5 and this regression continued throughout the remainder of gestation as a thin layer [Citation3]. On gestation day 10.5, the junctional zone was expanded compared to that of the previous stage (GD 9.5), this expansion continued and reached its maximum development on gestation day 13.5, then followed by reduction up to gestation day 18.5. On gestation day 10.5, the labyrinth zone was developed and the expansion was significantly increased up to gestation day 18.5 indicating its continuous growth and development.
Interestingly, our results showed that the RA induced irregular shape of placenta with defects that range from minor reduction of the spongiotrophoblast and labyrinth layers to severe labyrinth dysmorphogenesis; RA induced inhibition of the development of both the junctional and labyrinth zones with less numerous and small blood sinuses compared to control group. Moreover, RA induced failure of the allantois to fuse with the chorion in severe cases. This result supports the previous study [Citation41], suggesting that the placental alterations could explain the inability of the mothers to carry fetuses (miscarriage/reabsorption) or to cause fetal death [Citation42].
On day 9.5 of gestation, in the decidual basalis, EGFR was expressed strongly in non-decidualized cells (fibroblasts) of the central area and close to the smooth muscle coat. The strong signaling was extended up to gestation day 10.5, suggesting that the expression of EGFR is associated with the developed decidual basalis. On the other hand, by gestation day 12.5 the expression of EGFR was negative in the cells of the decidual basalis, and this negative expression extended up to the end of gestation, suggesting that the negative expression of EGFR is associated with the regression state of the decidual basalis. This suggestion is confirmed by a previous study, which showed that the decidual basalis undergoes cellular proliferation and apoptosis in distinct regions and at different stages of pregnancy [Citation43]. Moreover, in the labyrinth zone, the expression of EGFR was weak on gestation day 9.5 and increased gradually to moderate level by day 10.5, then increased to a strong level on day 12.5 which continued up to the end of pregnancy. This expression pattern of EGFR is associated with the development of labyrinth layer during pregnancy.
EGFR activation is crucial for embryogenesis and organogenesis, and impairment of EGFR signaling during organogenesis may result in respective defects in different organs [Citation26–30]. EGFR regulates the growth and differentiation of the placenta. Egfrtm1Mag nullizygous embryos exhibit strain-dependent placental defects that range from minor reduction of the spongiotrophoblast layer to severe labyrinth dysmorphogenesis [Citation27,Citation31].
To explain how RA induces this effect on the placenta, we have characterized the expression levels of EGFR as an important receptor for many growth factors during the utero-placental unit development under the effect of RA treatment. We found that RA induced a reduction of EGFR expression level in the junctional and labyrinth zones. Moreover, the inhibitory effect of RA on the expression of EGFR was also appeared on day 10.5 of gestation. By day 12.5 of gestation, the EGFR expression was mostly inhibited in decidual basalis, junctional zone, and labyrinth zone. A previous study showed that the excess of RA caused a reduction of TGFβ1 expression level, and the down regulation of TGFβ1 was continued up to 2 days after maternal administration [Citation16]. RA administration has been found to decrease the level of expression and the phosphorylation of the EGF receptor in human trophoblast cell cultures, suggesting that retinoids could modulate the effect of this growth factor during placental growth and differentiation [Citation33]. The mice with increased levels of EGFR signaling during placental development using the hypermorphic EgfrDsk5 allele resulted in larger placental size with a more prominent spongiotrophoblast layer and increased expression of glycogen cell-specific genes [Citation32]. On gestation days 13.5 and 18.5, the placentae from RA treated group with minor defects showed that EGFR was expressed at moderate level throughout the undifferentiated layers, suggesting that RA induced delay of the EGFR expression especially in the labyrinth zone.
Conclusions
We concluded that a high dose of RA may have a direct teratogenic effect on the fetus besides an indirect effect through modulating EGFR signal during the placenta development.
Recommendations
Any pregnant female, or even any female who will be pregnant, must be aware of the food and vitamins, which she deals with, because it is a critical period and any exogenous material may affect their fetuses not only during pregnancy but also this effect may continue with the newborn until maturation.
Disclosure statement
No potential conflict of interest was reported by the author(s).
References
- Deligdish L. Pathology of the placenta. In: Pasqualini JR, Scholler R, editors. Hormones and fetal patho-physiology. 5th ed. New York (NY): Marcel Dekker Inc; 1992. p. 203–249.
- Copp AJ. Death before birth: clues from gene knockouts and mutations. Trends Genet. 1995;11(3):87–93.
- Cross JC, Werb Z, Fisher SJ. Implantation and the placenta: key pieces of the development puzzle. Science. 1994;266(5190):1508–1518.
- Kaufmann P, Burton D. Anatomy and genesis of the placenta. In: Knobil E, Neill JD, editors. The physiology of reproduction. 2nd ed. New York (NY): Raven Press, Ltd.; 1994 :26 .
- Maltepe E, Simon MC. Oxygen genes, and development: an analysis of the role of hypoxic gene regulation during murine vascular development. J Mol Med. 1998;76(6):391–401.
- Watanabe S, Akagi R, Mori M, et al Marked developmental changes in Heme Oxygenase-1 (HO-1) expression in the mouse placenta: correlation between HO-1 Expression and placental development. Placenta. 2004;25(5):387–395.
- Ali RA, Wassif ET, Mostafa DF. Retinoic acid as a teratogen: III- axial shift and degeneration of nervous structures in the developing chick embryo. J Egypt Ger Soc Zool. 2007;52(B):57–82.
- Ali RA, Abdel-Tawab HS, Mustafa DF. Nigella sativa mitigative antioxidant properties against citral induced oxidative stress and biochemical changes during development of chick embryo. Biol Forum. 2018;10(2):74–85.
- Rhinn M, Dollé P. Retinoic acid signalling during development. Development. 2012;139(5):843–858.
- Yang X, Wright JR, Yu W, et al Parietal bone agenesis and athelia in retinoic acid embryopathy: an expansion of the phenotype. Birth Defects Res. 2021; 114 (1) :17–22.
- Bollag W. Vitamins and retinoids: from nutrition to pharmacotherapy in dermatology and oncology. Lancet. 1983;1(8329):860–863.
- Becherel PA, Le Goff L, Ktorza S, et al CD23-mediated nitric oxide synthase pathway induction in human keratinocytes is inhibited by retinoic acid derivatives. J Invest Dermatol. 1996;106(6):1182–1186.
- Duell EA, Kang S, Voorhees JJ. Retinoic acid isomers applied to human skin in vivo each induce a 4-hydroxylase that inactivates only trans retinoic acid. J Invest Dermatol. 1996;106(2):316–320.
- Cunningham TJ, Duester G. Mechanisms of retinoic acid signaling and its roles in organ and limb development. Nat Rev Mol Cell Biol. 2015;16(2):110–123.
- Webster WS, Johnston MC, Lammer EJ, et al Isotretinoin embryopathy and the cranial neural crest, an in vivo and in vitro study. J Cranwfacial Gene. 1986;6:211–222.
- Mahmood R, Flanders KC, Morris-Kay GM. Interactions between retinoids and TGFβs in mouse morphogenesis. Development. 1992;115(1):67–74.
- Wilson JG, Roth CB, Warkany J. An analysis of the syndrome of malformations induced by maternal vitamin A deficiency. Effects of restoration of vitamin A at various times during gestation. Am J Anat. 1953;92(2):189–217.
- Mendelsohn C, Lohnes D, Decimo D, et al Function of the retinoic acid receptors (RARs) during development (II). Multiple abnormalities at various stages of organogenesis in RAR double mutants. Development. 1994;120(10):2749–2771.
- Kastner P, Mark M, Ghyselinck N, et al Genetic evidence that the retinoid signal is transduced by heterodimeric RXR/RAR functional units during mouse development. Development. 1997;124(2):313–326.
- Niederreither K, Subbarayan V, Dollé P, et al Embryonic retinoic acid synthesis is essential for early mouse post-implantation development. Nat Genet. 1999;21:444–448.
- Shenefelt RE. Morphogenesis of malformations in hamsters caused by retinoic acid: relation to dose and stage at treatment. Teratology. 1972;5(1):103–118.
- Rothman KJ, Moore LL, Singer MR, et al Teratogenicity of high vitamin A intake. N Engl J Med. 1995;333(21):1369–1373.
- Huang Y, Ognjenović J, Karandur D, et al A molecular mechanism for the generation of ligand-dependent differential outputs by the epidermal growth factor receptor. eLife. 2021;10:e73218.
- Das SK, Tsukamura H, Paria BC, et al Differential expression of epidermal growth factor receptor (EGF-R) gene and regulation of EGF-R bioactivity by proges- terone and estrogen in the adult mouse uterus. Endocrinology. 1994;134(2):971–981.
- Tong BJ, Das SK, Threadgill D, et al Differential expression of the full-length and truncated forms of the epidermal growth factor receptor in the preimplantation mouse uterus and blastocyst. Endocrinology. 1996;137(4):1492–1496.
- Miettinen PJ, Berger JE, Meneses J, et al Epithelial immaturity and multiorgan failure in mice lacking epidermal growth factor receptor. Nature. 1995;376(6538):337–341.
- Sibilia M, Wagner EF. Strain-dependent epithelial defects in mice lacking the EGF receptor. Science. 1995;269(5221):234–238.
- Threadgill DW, Dlugosz AA, Hansen LA, et al Targeted disruption of mouse EGF receptor: effect of genetic background on mutant phenotype. Science. 1995;269(5221):230–234.
- Sibilia M, Steinbach JP, Stingl L, et al A strain-independent postnatal neurodegeneration in mice lacking the EGF receptor. EMBO J. 1998;17(3):719–731.
- Wong RW, Guillaud L. The role of epidermal growth factor and its receptors in mammalian CNS. Cytokine Growth Factor Rev. 2004;15(2–3):147–156.
- Threadgill DW, Yee D, Matin A, et al Genealogy of the 129 inbred strains: 129/SvJ is a contaminated inbred strain. Mamm Genome. 1997;8(6):390–393.
- Dackor J, Li M, Threadgill DW. Placental overgrowth and fertility defects in mice with a hypermorphic allele of epidermal growth factor receptor. Mamm Genome. 2009;20(6):339–349.
- Roulier S, Rochette-Egly C, Rebut-Bonneton C, et al Nuclear retinoic acid receptor characterization in cultured human trophoblast cell: effect of retinoic acid on epidermal growth factor receptor expression. Mol Cell Endocr. 1994;105(2):165–173.
- Steck PA, Hardi A, Lotan R, et al Inhibition of epidermal growth factor receptor activity by retinoic acid in glioma cells. J Cell Biochem. 1990;42(2):83–94.
- Collins MD, Tzimas G, Hummler H, et al Comparative teratology and transplacental pharmacokinetics of all-trans-retinoic acid, 13-cis-retinoic acid, and retinyl palmitate following daily administrations in rats. Toxicol Appl Pharmacol. 1994;127(1):132–144.
- Drury R, Wallington E. Carleton’s histological technique. London (UK): Oxford University Press; 1976.
- Buchlowalow BI, Bocker W. Immunohisto-chemistry. Basics and Methods. Berlin Heidelberg: Springer Verlag; 2010. p. 48.
- Quemelo PRV, Lourenco CM, Peres LC. Teratogenic effect of retinoic acid in Swiss mice. Acta Cir Bras. 2007;22(6):451–456.
- Agnish ND, DiNardo B, Holmes M, et al. Reproduction study with Ro 4-3780 (administered orally) in rats. Phase II-Teratology study with postnatal evaluation. Arch Data F Holfmann-La Roche. 1980.
- Morriss-Kay GM, Murphy P, Hill RE, et al Effects of retinoic acid excess on expression of Hox-2.9 and Krox-20 and on morphological segmentation in the hindbrain of mouse embryos. EMBO J. 1991;10(10):2985–2995.
- Sapin V, Begue R, Dastugue B, et al Retinoids and mouse placentation. Trophoblast Res. 1998;12:57–76.
- Noback CR, Takahashi YI. Micromorphology of the placenta of rats reared on marginal vitamin-A-deficient diet. Acta Ant. 1978;102(2):195–202.
- Correia-da-Silva G, Bell SC, Pringle JH, et al Patterns of uterine cellular proliferation and apoptosis in the implantation site of the rat during pregnancy. Placenta. 2004;25(6):538–547.