ABSTRACT
The majority of cases of liver cancer are discovered late in the disease’s progression, making it the third leading cause of cancer death globally. Chemotherapy is the major treatment choice for advanced stages of hepatocellular carcinoma (HCC); however, drug resistance, adverse effects, and high cost are major problems for effective treatment. Isoquercetin (IQ) is a dietary flavonoid, which has an anti-proliferative effect against several cancer cell lines. It also has a prophylactic effect against the early stages of some cancer models including HCC. The purpose of this study was to assess the therapeutic anticarcinogenic effect of IQ against murine HCC. A single intraperitoneal (ip) dose of 100 mg/kg of diethylnitrosamine (DEN), followed by 18 weekly ip doses of 0.5 mg/kg of carbon-tetrachloride (CCl4) was used to induce HCC. After the induction of HCC, IQ (10 and 20 mg/kg bw) was given orally from week 21 to week 24. IQ most likely ameliorated the structure and function of livers with experimentally induced HCC by improving the survival rate, significantly reducing tumor marker alpha-fetoprotein (AFP), number of hepatic nodules and downstaging HCC. This was accompanied by an increase in endogenous antioxidant capacity and the tumor suppressor protein ‘p53’, as well as lower levels of the lipid peroxidation marker malondialdehyde (MDA), proliferation marker proliferating cell nuclear antigen (PCNA) and angiogenesis marker vascular endothelial growth factor (VEGF) in the tissues. IQ suppresses proliferation, oxidative stress, and angiogenesis in advanced HCC by activating the tumor suppressor protein p53 and inhibiting proliferation, oxidative stress, and angiogenesis.
Introduction
HCC accounts for 80–90% of primary liver cancer cases [Citation1]. According to the International Agency for Research on Cancer (2020), HCC is the third most common cause of cancer-related death and the fifth most common cancer in the world [Citation2]. Most HCC patients are diagnosed during the advanced stage of the disease when surgical options are no longer possible [Citation3]. Less than one-third of these patients benefit from chemotherapy [Citation4,Citation5], which is the primary treatment option for advanced HCC patients [Citation6]. However, drug resistance within six months of initiating the regimen [Citation4,Citation5], intolerable adverse effects, and high cost are still obstacles for effective chemotherapy treatment [Citation7]. This encouraged many researchers to discover and develop natural product-originated drugs for treating cancer with higher therapeutic efficacy, lower cost, and fewer side effects in comparison with chemical drugs [Citation8,Citation9].
IQ is a dietary flavonoid that is found in various plants such as onions, apples, grapes, ginkgo, and tea [Citation10,Citation11]. IQ has been attracting scientific interest because of its therapeutic potential, especially against cancer [Citation12–15]. IQ also has other medicinal effects including anti-inflammatory effects, antioxidant activities [Citation16–19] and hepatoprotective effect [Citation20,Citation21]. In addition, IQ is nontoxic and safe for humans [Citation22,Citation23]. Despite the extensive published research on the beneficial effects of IQ, studies carried out on its effectiveness against HCC are rare. Previous studies evaluating the anticarcinogenic effect of IQ in liver cells were mostly in vitro [Citation12,Citation15,Citation24]. However, those carried out in experimental animals studied its prophylactic effects on the early stages of HCC as the study of Yokohira et al. (2008) [Citation25], or studied the transplanted tumors in nude mice as the study of Huang et al. (2014) [Citation12]. Therefore, the present work was assessed to estimate the anticarcinogenic effects of IQ against advanced HCC in vivo, and to estimate the possible underlying mechanisms on cell proliferation, angiogenesis, and antioxidant status.
Materials and methods
Chemicals
DEN was purchased from Sigma-Aldrich (MO, USA). CCl4 was obtained from Research Lab (Cairo, Egypt). IQ (Quercetin 3- β-D-glucoside) was acquired from Sigma-Aldrich (MO, USA). Kits for determining alanine aminotransferase (ALT) and aspartate aminotransferase (AST) in serum were purchased from SPINREACT (Ctra. Santa. Coloma, Spain). Kits of Albumin and total bilirubin were from EGY-CHEM (Cairo, Egypt). Mouse AFP ELISA kit was from CUSABIO (CA, USA).
Animals
Fifty-five male albino mice of CD1 strain, six-week-old, weighing 15–18 g, were obtained from the Biological Unit of Theodore Bilharz Institute (Giza, Egypt). Mice were given a week to acclimate to laboratory settings before beginning the experiments. Water and standard chow pellets were available to the animals at all times. The National Institutes of Health guide for the care and use of laboratory animals is followed in all animal experiments (NIH Publications No. 8023, revised 1985).
HCC Induction
HCC was induced as described by Uehara et al. (2013) [Citation26]. Briefly, DEN (DEN; 100 mg/kg freshly diluted in sterile 0.9% sodium chloride saline) was injected once intraperitoneally. Two weeks later, the animals received repeated biweekly ip injections of CCl4 (CCl4; 0.5 ml/kg dissolved in sterile corn oil), for 18 weeks to promote the carcinogenic effect of DEN [Citation27,Citation28].
Experimental design
The animals were divided into four groups randomly, as follows: Group 1 (n = 10): received the vehicle and served as a negative control group; Group 2 (n = 15): HCC-control group. Group 3 and 4 (n = 15 each): were treated with IQ (10 and 20 mg/kg bw) doses respectively for four weeks, beginning from week 21 until week 24 of HCC induction.
Sample collection
Twenty-four weeks after the beginning of the experiment, the animals were weighed, then euthanized by diethyl ether inhalation. Blood samples were withdrawn from the retro-orbital vein of each animal after a fasting period of 12–14 h. To obtain sera, the samples were allowed to clot at room temperature before being centrifuged at 6000 g for 15 minutes at 4°C. For further biochemical analyses, aliquots of serum were stored at −20°C. After the collection of the blood samples, the animals were sacrificed and the livers were quickly excised, washed in saline, and blotted dry. The livers were then weighed and evaluated for gross lesions. For histological and immunohistochemical research, liver portions were preserved in 10% neutral buffered formalin.
Biochemical assays
Serum ALT, AST, total bilirubin, albumin, in addition to liver MDA, and total antioxidant capacity (TAC), were assayed according to manufacturer’s instructions using commercially available colorimetric kits. Serum AFP which is an HCC-specific tumor marker was quantified using the ELISA technique.
Histopathological estimation
For 24 hours, liver samples from the left median lobes were fixed in 10% neutral buffered formalin. The samples were then washed overnight in running tap water, dehydrated, and embedded in paraffin wax. Hematoxylin and eosin were used to stain 5 μm thick transverse sections [Citation29].
Immunohistochemistry
Hepatic tissues were prepared by a conventional process. The following antibodies were used: Mouse monoclonal P53 antibody (IR616) at 1:100 (DO-7, Dako, Glostrup, Denmark), Rabbit monoclonal PCNA antibody (EPR3821) at 1:100 (Abcam, Cambridge, UK), and mouse monoclonal VEGF antibody (A17877) at 1:150 (AB clonal, China). Immunohistochemical analysis was applied according to the guidelines of each kit.
Statistical analysis
Mean values and standard error of means were used to express numerical data. GraphPad Prism (version 5.0, GraphPad Software, San Diego, CA, USA) was used to conduct all statistical analyses. Data were analyzed statistically by one-way ANOVA and then by post hoc multiple comparisons (Tukey’s test) for comparative analysis between the groups. P< 0 · 05 was regarded as statistically significant.
Results
Survival rate
During the 24 weeks of the experiment, there were no deaths in the blank control group. HCC group showed a high mortality rate, reached 78.6% at week 24. Treating HCC animals with IQ, which started at week 21, had lower mortality rate compared with HCC group. The mortality rate of the high dose group (20 mg/kg bw) was 56% vs 62.5% of low dose group (10 mg/kg bw) ().
Biochemical results
HCC-bearing mice showed significant (P< 0.05) increase in serum AST, ALT and total bilirubin (), as well as in serum AFP and liver MDA concentrations (), and significant (P< 0.05) decrease in serum albumin () and liver TAC () when compared with those of the blank control group. Treating HCC mice with IQ (10 mg/kg) showed no significant ameliorative effect on the elevated hepatic enzymes, bilirubin, or nodular count, but it significantly reduced the elevated AFP and MDA, and significantly elevated the serum albumin and liver TAC. On the other hand, treating HCC mice with IQ (20 mg/kg) significantly ameliorated all the biochemical results, nodular count, and AFP in comparison with HCC control group and IQ 10 mg/kg group.
Table 1. Effect of IQ on serum hepatic biomarkers in HCC-bearing mice
Table 2. Effect of IQ on the nodular count, tumor marker AFP, hepatic MDA and TAC in HCC-bearing mice
Histological results
Examination of hematoxylin and eosin-stained liver sections obtained from the negative control mouse showed normal architecture, with defined central vein and peripheral portal triad with hepatic portal vein, bile ductule and hepatic artery (). Each hepatic lobule has a central vein and radiated hepatic strands with 1–2 cell-thick hepatocytes, separated by sinusoids. Hepatocytes were polygonal with granular and eosinophilic cytoplasm. Rounded one or two nuclei (binucleated) were also seen. Blood sinusoids are lined with endothelial cells and scattered Kupffer cells ().
Figure 2. A photomicrograph of a section of the liver of negative control mouse revealing normal architecture, with defined central vein (CV), and peripheral portal triad with hepatic portal vein (PV), bile ductule (BD), and hepatic artery (HA) (H & E x100).
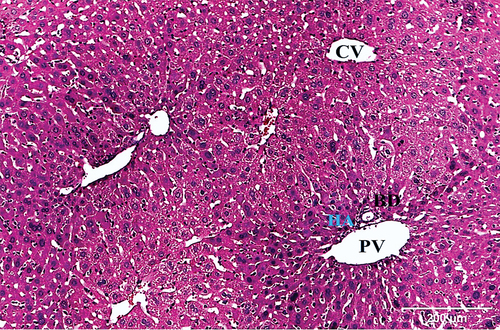
Figure 3. A photomicrograph of a liver section of negative control mouse showing hepatic lobule with central vein (CV), and radiated hepatic strands with 1–2 cell-thick polygonal hepatocytes (H), separated by sinusoids (S). The hepatocytes have either one nucleus (N) or binucleated (DN). Blood sinusoids are lined with endothelial cells (E) and scattered Kupffer cells (K) (H & E x400).
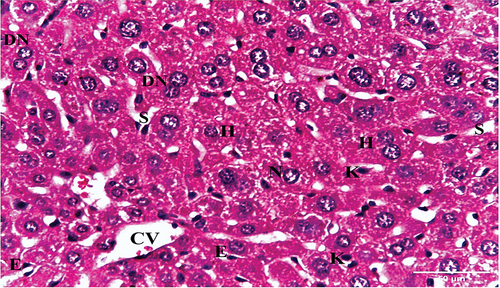
On the other hand, liver sections of HCC-control group exhibited architectural characteristics of HCC, with significantly altered structure of liver tissue. Several nodules with indefinable radiated cell plates and portal veins surrounded by inflammatory infiltrates were seen (). The hepatic strands were disorganized and collapsed, and the hepatocytes were well-differentiated with eosinophilic cytoplasm. The nuclei were pleomorphic, relatively large, and some were dividing. Portal veins with inflammatory infiltrate and sinusoids were also seen ().
Figure 4. Photomicrograph of a liver section of HCC control group showing several nodules (asterisks) with indefinable radiated cell plates, and portal veins (PV) surrounded by inflammatory infiltrates (arrows) (HX&E X100).
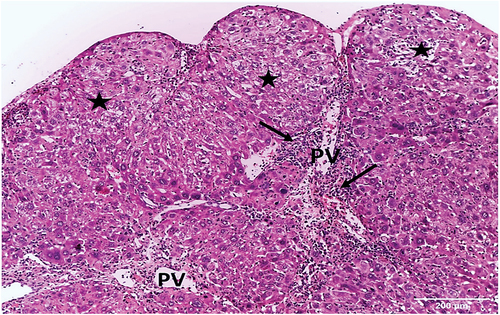
Figure 5. A photomicrograph of a liver section of HCC control group mouse showing altered liver tissue. The hepatic strands are disorganized, and the hepatocytes (H) are well-differentiated with eosinophilic cytoplasm. The nuclei are pleomorphic, relatively large, and some are dividing (DN). Portal vein (PV) with inflammatory infiltrate (arrows) and sinusoids (S) are also seen (HX & E x400).
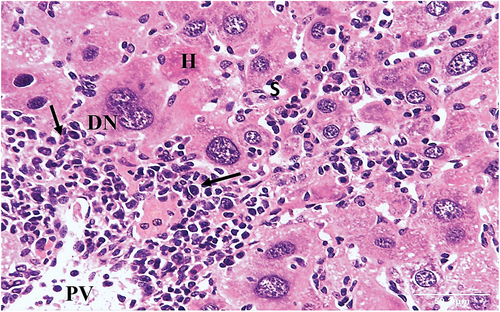
Figure 6. A photomicrograph of section of liver of HCC control group mouse showing significantly altered liver tissue. The hepatic strands are disorganized. The hepatocytes (H) are well-differentiated with eosinophilic cytoplasm. The nuclei are pleomorphic with prominent nucleoli (PN). Portal vein (PV) and compressed sinusoids (S) are also seen (HX & E x400).
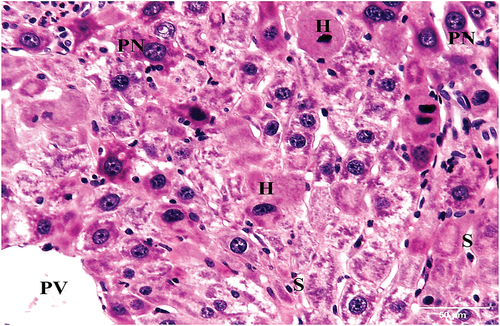
HCC group treated with 10 mg/kg bw IQ exhibited disorganized hepatic cords. Central veins and portal veins were surrounded by small number of sinusoids, some of these sinusoids were congested. Normal bile ductules, hepatocytes with vacuolated cytoplasm and central rounded nuclei, as well as mild inflammatory infiltrates were also seen ().
Figure 7. A photomicrograph of a section of liver of HCC treated with IQ (10 mg/kg bw) showing disorganized hepatic cords. Central vein (CV), Portal vein (PV) with bile ductule (BD) and congested sinusoids (S) are seen. Hepatocytes with vacuolated cytoplasm and mild inflammatory infiltrate (arrow) are also seen (H&E x100).
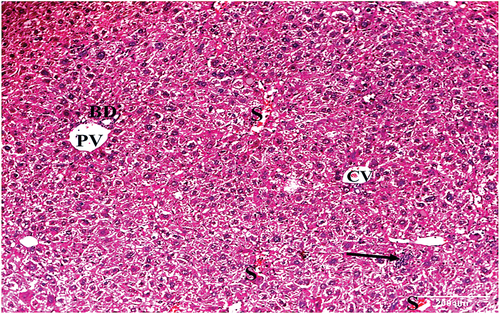
Figure 8. A photomicrograph of a liver section of HCC animals treated with IQ (10 mg/kg bw) showing portal vein (PV) with bile ductule (BD). The portal vein is surrounded by sinusoids (S) and hepatocytes (H) with vacuolated cytoplasm and central rounded nuclei (N) (H&E x400).
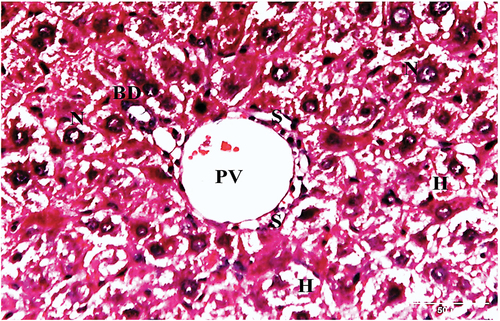
HCC group treated with 20 mg/kg bw IQ showed central vein, portal vein and bile ductule surrounded by sinusoids. Hepatocytes with moderately vacuolated cytoplasm and central rounded nucleus, few inflammatory infiltrates and Kupffer cells were also seen ().
Figure 9. A photomicrograph of a liver section of HCC animals treated with IQ (20 mg/kg bw) showing central veins (CV), portal vein (PV), and bile ductule (BD). Hepatocytes with moderately vacuolated cytoplasm and mild inflammatory infiltrate (arrow) are also seen (H&E x100). ) A photomicrograph of a liver section of HCC animals treated with IQ (20 mg/kg bw) showing central vein (CV), blood sinusoids (S), hepatocytes (H) with moderately vacuolated cytoplasm, and central rounded nuclei (N). Kupffer cells (K) are also seen (H&E x400).
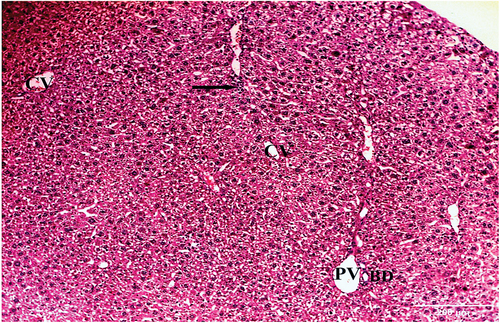
Immunohistochemical results
P53 immuno-expression in negative control and IQ-treated groups mice showed barely detectable P53 in the nuclei of hepatocytes. In HCC liver section, the nuclear immuno-expression of P53 was observed in hepatocytes (, ).
Figure 11. Photomicrographs showing P53 immuno-expression in (a) negative control, (b) HCC control group, (c) HCC+IQ [10 mg/kg bw] -treated group, and (d) HCC+ IQ [20 mg/kg bw]-treated group. Negative control (a) and HCC+IQ-treated groups (c, d) show barely detectable P53 in the hepatocytes nuclei, in comparison with the dense expression in HCC control group (b).
![Figure 11. Photomicrographs showing P53 immuno-expression in (a) negative control, (b) HCC control group, (c) HCC+IQ [10 mg/kg bw] -treated group, and (d) HCC+ IQ [20 mg/kg bw]-treated group. Negative control (a) and HCC+IQ-treated groups (c, d) show barely detectable P53 in the hepatocytes nuclei, in comparison with the dense expression in HCC control group (b).](/cms/asset/4d5c049f-4dcb-40a0-82da-da2d5a4bf724/teba_a_2079843_f0011_oc.jpg)
Table 3. Effects of IQ on the immunohistochemical expression in hepatic tissues of HCC-bearing mice
The distribution of PCNA showed weak nuclear immunoreactivity in negative control mice liver sections, while HCC control group showed strong positive nuclear immunoreactivity of PCNA. IQ (10 mg/kg bw)-treated group showed moderately positive nuclear immunoreactivity of PCNA, while IQ (20 mg/kg bw)-treated group showed positively mild nuclear immunoreaction (, ).
Figure 12. Photomicrographs showing PCNA immuno-expression in (a) negative control, (b) HCC control, (c) HCC+IQ [10 mg/kg bw] -treated and (d) HCC+IQ [20 mg/kg bw]-treated groups. Negative control section shows weak nuclear PCNA immunoreaction, while HCC control section shows strong positive nuclear immunoreaction. HCC+IQ [10 mg/kg bw]-treated group shows moderately positive nuclear immunoreaction, while HCC+IQ [20 mg/kg bw]-treated group shows positively mild nuclear immunoreaction.
![Figure 12. Photomicrographs showing PCNA immuno-expression in (a) negative control, (b) HCC control, (c) HCC+IQ [10 mg/kg bw] -treated and (d) HCC+IQ [20 mg/kg bw]-treated groups. Negative control section shows weak nuclear PCNA immunoreaction, while HCC control section shows strong positive nuclear immunoreaction. HCC+IQ [10 mg/kg bw]-treated group shows moderately positive nuclear immunoreaction, while HCC+IQ [20 mg/kg bw]-treated group shows positively mild nuclear immunoreaction.](/cms/asset/4ad45607-4b31-4e44-a3de-1b3d57886a1b/teba_a_2079843_f0012_oc.jpg)
VEGF showed localized and weak immunoreactivity along the sinusoids and portal vessels in negative control mice, while in HCC control group VEGF diffused in strong positive immunoreactivity along with hepatocytic cytoplasm, endothelial cells, sinusoids, and portal vein. IQ (10 mg/kg bw)-treated group showed localized VEGF immunoreactivity along the sinusoids and there were some sporadic cells still had the stain, while in IQ (20 mg/kg bw)-treated group there was weak localized VEGF immunoreactivity along the sinusoids and portal vessels (, ).
Figure 13. Photomicrographs showing VEGF immuno-expression in (a) negative control, (b) HCC control group, (c) HCC+IQ 10 mg/kg bw -treated group and (d) HCC+IQ [20 mg/kg bw]-treated group. Negative control showing localized and weak VEGF immunoreactivity along the sinusoids and portal vessels, while in HCC control group VEGF is diffuse in strong positive immunoreactivity along with hepatocytic cytoplasm, endothelial cells, sinusoids, and portal vein. HCC+IQ [10 mg/kg bw] -treated group showing localized VEGF immunoreactivity along the sinusoids and there are some sporadic cells still have the stain, while in HCC+IQ [20 mg/kg bw]-treated group there is weak localized VEGF immunoreactivity along the sinusoids and portal vessels.
![Figure 13. Photomicrographs showing VEGF immuno-expression in (a) negative control, (b) HCC control group, (c) HCC+IQ 10 mg/kg bw -treated group and (d) HCC+IQ [20 mg/kg bw]-treated group. Negative control showing localized and weak VEGF immunoreactivity along the sinusoids and portal vessels, while in HCC control group VEGF is diffuse in strong positive immunoreactivity along with hepatocytic cytoplasm, endothelial cells, sinusoids, and portal vein. HCC+IQ [10 mg/kg bw] -treated group showing localized VEGF immunoreactivity along the sinusoids and there are some sporadic cells still have the stain, while in HCC+IQ [20 mg/kg bw]-treated group there is weak localized VEGF immunoreactivity along the sinusoids and portal vessels.](/cms/asset/91005d66-411f-4f74-8f4f-38939ac8b78b/teba_a_2079843_f0013_oc.jpg)
Discussion
Oxidative stress is the major cause of HCC. It has emerged as a crucial factor in the initiation and development and progression of liver carcinogenesis [Citation30]. This ability of oxidative stress to develop HCC is due to its ability to induce mutations, generate free radicals, which can somatically alter key oncogenes and tumor suppressor genes, such as p53. P53 is a transcription factor that regulates many genes regulating cell cycle, apoptosis, DNA repair, senescence, and metabolism [Citation31]. P53 is one of the most commonly mutated genes in HCC [Citation32]. Down-expression of p53 was observed in liver sections of HCC animals here and in earlier studies [Citation9,Citation33].
Endogenous antioxidants were decreased significantly in DEN-induced HCC rats, compared with that of the normal one. The decline in the activities of these enzymes could be attributed to the excessive utilization of these enzymes in inactivating the free radicals generated during the metabolism of DEN [Citation34]. Increased Reactive oxygen species (ROS) levels also cause damage to lipids by lipid peroxidation, with an increase in cell membrane permeability and cell death [Citation35]. In this work, MDA was elevated in HCC animals, which is in agreement with a study of Kuznietsova et al. (2020) [Citation36] and Mahmoud et al. (2021) [Citation9].
In addition to lipid peroxidation, increased ROS levels also cause damage to proteins, which causes alterations in the cell structure [Citation35]. In our study, HCC- bearing mice exhibited altered architecture and missed definite hepatic strands. The normal liver tissue was replaced by multiple nodules variable in size and shape with inflammatory infiltrate and the hepatocytes were pleomorphic with eosinophilic cytoplasm and most of them had large nuclei. Similar histological observations were reported by Ding et al. (2017) [Citation37] at 20 weeks after HCC introduction.
These pathological alterations in hepatocytes of HCC-bearing mice, lead to functional alterations such as increased liver enzymes. In individuals with liver diseases such as hepatocellular predominant disorders, serum liver enzymes such as ALT and AST are typically high [Citation38]. In our study, HCC control group showed significant elevations in serum levels of ALT and AST enzymes, compared with the normal control group. The same results were reached by Ding et al. (2017) [Citation37], Abozaid et al. (2018) [Citation39] and Elsayed et al. (2019) [Citation40].
Albumin levels decrease in hepatotoxic conditions due to defective protein biosynthesis in the liver [Citation41]. The DEN intoxication causes disruption and disassociation of polyribosomes on the endoplasmic reticulum and thereby reduces the biosynthesis of protein [Citation39]. Levels of serum albumin have an important prognostic indicator for HCC [Citation42]. In this study, albumin was significantly decreased in HCC control group compared with the normal group. The same result was obtained by Abozaid et al. (2018) [Citation39] and Elsayed et al. (2019) [Citation40].
VEGF is an endothelial growth factor that regulates angiogenesis and is critical for HCC growth and development [Citation43]. In DEN-induced carcinogenesis is overexpressed [Citation44,Citation45]. Angiogenesis can be triggered by activation of oncogenes like ras or inactivation of tumor suppressor genes like p53 [Citation46]. An increased level of PCNA expression has been described to signify an aggressive tumor [Citation47]. Many studies reported overexpression of PCNA in DEN-induced carcinogenesis [Citation48].
IQ is well known for its anticancer effect [Citation49] and inhibit the growth of HepG2 cells via endoplasmic reticulum stress [Citation24] and liver preneoplastic lesion development in vivo [Citation25] and it has a significant, dose-dependent, inhibitory effect [Citation12,Citation13] on pancreatic cancer progression.
Studies regarding its mechanism of action against HCC remain limited. It is well established that one of the mechanisms for cancer development is oxidative stress and therefore, the chemo-preventive effects of IQ could be attributed to its antioxidant and oxidative damage preventive activities. IQ inhibits lipid peroxidation by interfering with enzyme function (xanthine oxidase), chelating redox-active metals, increasing vitamin C absorption, and directly scavenging ROS such as singlet oxygen, hydroxyl, peroxyl, peroxynitrite, and superoxide radicals [Citation50–52]. IQ possesses ROS and nitrite-scavenging capacities by increasing the levels of superoxide dismutase or catalase and reducing the MDA in the livers and kidneys of mice exposed to Cd2+ [Citation53].
Our results indicate that treating HCC-bearing mice with IQ restored the decreased activities of the TAC and prevented the peroxidation of the lipids of the cell membranes of the hepatocytes, and consequently, preventing the leakage of the liver enzymes to the bloodstream, indicating that IQ has an antioxidant potential against HCC-correlated oxidative stress. This is in agreement with a previous study of Li et al. (2011) [Citation53] and Chen et al. (2016) [Citation54]. The antioxidative mechanism of IQ is largely dependent on the number and position of phenolic hydroxyl groups linked to the structural backbone of IQ [Citation53]. The phenolic hydroxyl moieties may easily react with O2−· and ·OH, reducing the accumulation of ROS [Citation53]. Our results indicate that IQ exhibits a hepatoprotective potential by reducing elevated serum levels of AST and ALT and increasing albumin levels after 30 days of therapy, and the change occurred in a dose-dependent manner this result is in agreement with the study of Huang et al. (2017) [Citation21].
In our result IQ induce apoptosis and inhibit tumor growth, this is in agreement with previous studies of Li et al. (2018) [Citation14] and Shui et al. (2020) [Citation15]. Molecular mechanism of IQ might be closely associated with the mitogen-activated protein kinase and protein kinase C signaling pathways [Citation14,Citation52]. Antioxidants could induce apoptosis in HCC animals [Citation9,Citation55,Citation56], indicating that the anticarcinogenic effect of IQ is possibly linked to apoptosis induction. This finds support in the increased immunohistochemical expression of P53 and PCNA in IQ- treated mice. There was downregulation of immunohistochemical expression of VEGF in IQ -treated mice that support the anti-angiogenesis activity of IQ. The anticarcinogenic effect of IQ could also be attributed to its antiangiogenic effect, manifested by suppressing the overexpressed angiogenic marker VEGF that starves the tumors of their blood supply to avoid the refueling of oxygen and nutrients [Citation9,Citation57], which are crucial to this highly vascular solid tumor with a rapid growth pattern.
In the current study, the livers of HCC control animals emerged nodules of different sizes on the surface of the liver of this group. Also, Luo et al. (2012) [Citation58] stated that HCC livers showed multinodular or coarsely granular surfaces at week 20. In our study treating HCC mice with IQ significantly decreased the nodular count.
In conclusion, IQ exhibited an anticarcinogenic effect against HCC manifested by tumor regression, increased survival rate, AFP tumor marker suppression, ameliorating liver biomarkers, and downstaging HCC pathology. IQ anticarcinogenic effect was induced by restoring the antioxidant capacity, activating the tumor suppressor protein P53 and suppressing PCNA, angiogenic marker VEGF, and oxidative stress. IQ, being a natural product with antioxidant, angiogenic properties, may be a promising candidate for the treatment of HCC.
Disclosure statement
No potential conflict of interest was reported by the author(s).
References
- Rasool M, Rashid S, Arooj M, et al. New possibilities in hepatocellular carcinoma treatment. Anticancer Res. 2014;34(4):1563–1571.
- International agency for research on cancer globocan: cancer fact sheets. (2020) https://gco.iarc.fr/today/data/factsheets/cancers/11-Liver-fact-sheet.pdf. (cited 2020 May 18).
- Hiraoka A, Kawamura T, Aibiki T, et al. Prognosis and therapy for ruptured hepatocellular carcinoma: problems with staging and treatment strategy. Eur J Radiol. 2015;84(3):366–371.
- El-Serag HB, Marrero JA, Rudolph L, et al. Diagnosis and treatment of hepatocellular carcinoma. Gastroenterol. 2008;134(6):1752–1763.
- Anwanwan D, Singh SK, Singh S, et al. Challenges in liver cancer and possible treatment approaches. Biochim Biophys Acta Rev Cancer. 2020;1873(1):188314.
- Jung KH, Rumman M, Yan H, et al. An ethyl acetate fraction of Artemisia capillaris (ACE‐63) induced apoptosis and anti‐angiogenesis via inhibition of PI3K/AKT signaling in hepatocellular carcinoma. Phytother Res. 2018;32(10):2034–2046.
- Ren F, Li J, Yuan X, et al. Dandelion polysaccharides exert anticancer effect on hepatocellular carcinoma by inhibiting PI3K/AKT/mTOR pathway and enhancing immune response. J Funct Foods. 2019;55:263–274.
- Kefayat A, Ghahremani F, Safavi A, et al. Spirulina extract enriched for braun‐type lipoprotein (Immulina®) for inhibition of 4T1 breast tumors’ growth and metastasis. Phytother Res. 2020;34(2):368–378.
- Mahmoud YI, Shehata AM, Fares NH, et al. Spirulina inhibits hepatocellular carcinoma through activating p53 and apoptosis and suppressing oxidative stress and angiogenesis. Life Sci. 2021;265:118827.
- Zhou J, Yoshitomi H, Liu T, et al. Isoquercitrin activates the AMP–activated protein kinase (AMPK) signal pathway in rat H4IIE cells. BMC Complement Altern Med. 2014;14(1):42.
- Ran J, Wang Y, Zhang W, et al. Research on the bioactivity of isoquercetin extracted from marestail on bladder cancer EJ cell and the mechanism of its occurrence. Artif Cells Nanomed Biotechnol. 2016;44(3):859–864.
- Huang G, Tang B, Tang K, et al. Isoquercitrin inhibits the progression of liver cancer in vivo and in vitro via the MAPK signalling pathway. Oncol Rep. 2014;31(5):2377–2384.
- Chen Q, Li P, Xu Y, et al. Isoquercitrin inhibits the progression of pancreatic cancer in vivo and in vitro by regulating opioid receptors and the mitogen-activated protein kinase signalling pathway. Oncol Rep. 2015;33(2):840–848.
- Li S, Tan HY, Wang N, et al. The potential and action mechanism of polyphenols in the treatment of liver diseases. Oxid Med Cell Longev. 2018;2018 .
- Shui L, Wang W, Xie M, et al. Isoquercitrin induces apoptosis and autophagy in hepatocellular carcinoma cells via AMPK/mTOR/p70S6K signaling pathway. Aging (Albany NY). 2020;12(23):24318.
- Qin G, Ma J, Huang Q, et al. Isoquercetin improves hepatic lipid accumulation by activating AMPK pathway and suppressing TGF-β signaling on an HFD-induced nonalcoholic fatty liver disease rat model. Int J Mol Sci. 2018;19(12):4126.
- Chen GL, Fan MX, Wu JL, et al. Antioxidant and anti-inflammatory properties of flavonoids from lotus plumule. Food Chem. 2019;277:706–712.
- Liang S, Xu Z, Ruan Y, et al. Isoquercitrin attenuates renal ischemia/reperfusion injury through antioxidation, anti-inflammation, and antiapoptosis in mice. Transplant Proc. 2020;52(3):1014–1019.
- Park HJ, Kim HN, Kim CY, et al. Synergistic protection by isoquercitrin and quercetin against glutamate-induced oxidative cell death in HT22 cells via activating nrf2 and HO-1 signaling pathway: neuroprotective principles and mechanisms of dendropanax morbifera leaves. Antioxidants. 2021;10(4):554.
- Xie W, Chen C, Jiang Z, et al. Apocynum venetum attenuates acetaminophen-induced liver injury in mice. Am J Chin Med. 2015;43(3):457–476.
- Huang XL, He Y, Ji LL, et al. Hepatoprotective potential of isoquercitrin against type 2 diabetes-induced hepatic injury in rats. Oncotarget. 2017;8(60):101545.
- Liu M, Wei C. Pharmacology effect of isoquercetin and its clinical application prospect. Chinese Pharmacy. 2010;21:2581–2583.
- QU WT, W ZHU, Zhai GY, et al. Research progress of synthesis and biological activity of quercetin derivatives. Chem Res. 2010;23:101–110.
- Gao X, Yanan J, Santhanam RK, et al. Garlic flavonoids alleviate H2O2 induced oxidative damage in L02 cells and induced apoptosis in HepG2 cells by Bcl‐2/caspase pathway. J Food Sci. 2021;86(2):366–375.
- Yokohira M, Yamakawa K, Saoo K, et al. Antioxidant effects of flavonoids used as food additives (purple corn color, enzymatically modified isoquercitrin, and isoquercitrin) on liver carcinogenesis in a rat medium‐term bioassay. J Food Sci. 2008;73(7):C561–C568.
- Uehara T, Ainslie GR, Kutanzi K, et al. Molecular mechanisms of fibrosis-associated promotion of liver carcinogenesis. Toxicol Sci. 2013;132(1):53–63.
- Dapito DH, Mencin A, Gwak GY, et al. Promotion of hepatocellular carcinoma by the intestinal microbiota and TLR4. Cancer Cell. 2012;21(4):504–516.
- Tolba R, Kraus T, Liedtke C, et al. Diethylnitrosamine (DEN)-induced carcinogenic liver injury in mice. Lab Anim. 2015;49(1):59–69.
- Bancroft JD, Gamble M. Theory and practice of histological technique. 5th ed. London: Churchill Livingstone Edinburg London; 2002.
- McLoughlin MR, Orlicky DJ, Prigge JR, et al. TrxR1, Gsr, and oxidative stress determine hepatocellular carcinoma malignancy. Proc Natl Acad Sci U S A. 2019;116(23):11408–11417.
- Lane D, Levine A. p53 research: the past thirty years and the next thirty years. Cold Spring Harb Perspect Biol. 2010;2(12):a000893.
- Schulze K, Imbeaud S, Letouzé E, et al. Exome sequencing of hepatocellular carcinomas identifies new mutational signatures and potential therapeutic targets. Nat Genet. 2015;47(5):505–511.
- Ahmed OM, Ahmed AA, Fahim HI, et al. Quercetin and naringenin abate diethylnitrosamine/acetylaminofluorene-induced hepatocarcinogenesis in Wistar rats: the roles of oxidative stress, inflammation and cell apoptosis. Drug Chem Toxicol. 2019; 1–12.
- Veeraraghavan VP, Mohan SK, Jainu M, et al. Ameliorating effects of garcinia mangostana Linn pericarp extract on hepatic antioxidants in diethyl nitrosamine (DEN) induced hepatocellular carcinoma (HCC). Pharm Res. 2015;49(4):329–337.
- Ko E, Kim JS, Bae JW, et al. SERPINA3 is a key modulator of HNRNP-K transcriptional activity against oxidative stress in HCC. Redox Biol. 2019;24:101217.
- Kuznietsova H, Dziubenko N, Herheliuk T, et al. Water-soluble pristine C60 fullerene inhibits liver alterations associated with hepatocellular carcinoma in rat. Pharmaceutics. 2020;12(9):794.
- Ding YF, Wu ZH, Wei YJ, et al. Hepatic inflammation-fibrosis- cancer axis in the rat hepatocellular carcinoma induced by diethylnitrosamine. J Cancer Res Clin Oncol. 2017;143(5):821–834.
- Hann HW, Wan S, Myers RE, et al. Comprehensive analysis of common serum liver enzymes as prospective predictors of hepatocellular carcinoma in HBV patients. PloS one. 2012;7(10):47687.
- Abozaid O, Moawed F, Salah Kawara R. Protective effect of benzethonium chloride on diethylnitrosamine-induced hepatocellular carcinoma. Benha. Vet Med J. 2018;35(2):537–546.
- Elsayed MM, Mostafa ME, Alaaeldin E, et al. Design and characterisation of novel sorafenib-loaded carbon nanotubes with distinct tumour-suppressive activity in hepatocellular carcinoma. Int J Nanomedicine. 2019;14:8445.
- Clawson GA. Mechanisms of carbon tetrachloride hepatotoxicity. Pathol Immunopathol Res. 1989;8(2):104–112
- Bağırsakçı E, Şahin E, Atabey N, et al. Role of albumin in growth inhibition in hepatocellular carcinoma. Oncology. 2017;93(2):136–142.
- Morse MA, Sun W, Kim R, et al. The role of angiogenesis in hepatocellular carcinoma. Clin Cancer Res. 2019;25(3):912–920.
- Anwar HM, Moghazy AM, Osman AAE, et al. The therapeutic effect of myrrh (Commiphora molmol) and doxorubicin on diethylnitrosamine induced hepatocarcinogenesis in male albino rats. Asian Pac J Cancer Prev. 2013;22(7):2153–2163.
- Zakaria S, Helmy MW, Salahuddin A, et al. Chemopreventive and antitumor effects of benzyl isothiocynate on HCC models: a possible role of HGF/pAkt/STAT3 axis and VEGF. Biomed Pharmacother. 2018;108:65–75.
- Rak J, Mitsuhashi Y, Bayko L, et al. Mutant ras oncogenes upregulate VEGF/VPF expression: implications for induction and inhibition of tumor angiogenesis. Cancer Res. 1995;55(20):4575–4580.
- Bedeer A, El-Rashidy M, Elzayat S, et al. Evaluation of the prognostic significance of P53 and proliferating cell nuclear antigen (PCNA) in laryngeal squamous cell carcinoma. Int J Cancer Biomed Res. 2020;4(3): 169–176.
- Sánchez DI, González‐Fernández B, San‐Miguel B, et al. Melatonin prevents deregulation of the sphingosine kinase/sphingosine 1‐phosphate signaling pathway in a mouse model of diethylnitrosamine‐induced hepatocellular carcinoma. J Pineal Res. 2017;62(1):12369.
- Ramirez JE, Zambrano R, Sepúlveda B, et al. Anthocyanins and antioxidant capacities of six Chilean berries by HPLC–HR-ESI-ToF-MS. Food Chem. 2015;176:106–114.
- Appleton J. Evaluating the bioavailability of isoquercetin. Nat Med J. 2010;2(1):1–6.
- De Araújo MEMB, Franco YEM, Alberto TG, et al. Enzymatic de-glycosylation of rutin improves its antioxidant and antiproliferative activities. Food Chem. 2013;141(1):266–273.
- Di Camillo Orfali G, Duarte AC, Bonadio V, et al. Review of anticancer mechanisms of isoquercitin. World J Clin Oncol. 2016;7(2):189.
- Li R, Yuan C, Dong C, et al. In vivo antioxidative effect of isoquercitrin on cadmium-induced oxidative damage to mouse liver and kidney. Naunyn Schmiedebergs Arch Pharmacol. 2011;383(5):437–445.
- Chen F, Chen X, Yang D, et al. Isoquercitrin inhibits bladder cancer progression in vivo and in vitro by regulating the PI3K/Akt and PKC signaling pathways. Oncol Rep. 2016;36(1):165–172.
- Abolmaesoomi M, Aziz AA, Junit SM, et al. Effects of solvent polarity on antioxidant and anti-proliferative activities in breast and colon cancer cells. Eur J Integr Med. 2019;28:57–67.
- El-Far YM, Khodir AE, Noor AO, et al. Selective cytotoxic activity and protective effects of sodium ascorbate against hepatocellular carcinoma through its effect on oxidative stress and apoptosis in vivo and in vitro. Redox Rep. 2020;25(1):17–25.
- Liu K, Zhang X, Xu W, et al. Targeting the vasculature in hepatocellular carcinoma treatment: starving versus normalizing blood supply. Clin Transl Gastroenterol. 2017;8(6):98.
- Luo M, Yang F, Huang SX, et al. Two- stage model of chemically induced hepatocellular carcinoma in mouse. Cancer Commun. 2012;20(11):517–528.