ABSTRACT
The present study evaluated the effect of Centella asiatica L. Urban (CA) extracts on ultrastructure and oxidative stress parameters on chronic unpredictable mild stress (CUMS) rat’s hippocampi. Rats were divided into six groups (n=6) and treated as follows: Group 1, Unstressed + normal saline (control); Group 2, CUMS + normal saline (Model); Group 3, CUMS + Flx (10 mg/kg) (FLX); Groups 4, CUMS + CA (200 mg/kg) (CA 200); Group 5, CUMS + CA (400 mg/kg) (CA 400) and Group 6, CUMS + CA (800 mg/kg) (CA 800). Nine different stressors were administered to the rats for 8 weeks consecutively, with concurrent administration of fluoxetine or CA. The hippocampi of the rats were evaluated by transmission electron microscopy (TEM) and oxidative stress biomarkers were also determined. Qualitative TEM results showed defective mitochondria, nucleus, myelin sheaths and reduced numbers of synapses in CUMS rats as well as increased oxidative stress biomarkers. Administration of CA prevented some of the structural and biochemical aberrations observed in response to stress in a manner comparable with fluoxetine, a selective serotonin-reuptake inhibitor. Conclusively, CA ameliorates structural defects induced by CUMS in the rat hippocampus, with a potential therapeutic importance in the treatment of stress and depression.
Introduction
Stress is a process where the physical and psychological demands of an individual, which are called the stressors, affect the ability of the individual or organism to adapt to the ensuing challenges [Citation1]. There are different types of stressors which include acute and chronic, major and minor, and desirable and undesirable, which highlight the diversity of stresses that affect the life of an individual. Chronic stress occurs when a stressor persists or occurs as a repetition of an initial stressful event [Citation2]. Postmortem studies on patients diagnosed with chronic stress-induced depression and on animal models of depression have demonstrated various changes including a decrease in the size of parts of the limbic system of the brain such as the hippocampus and amygdala [Citation3]. In a study carried-out on a chronic unpredictable mild stress (CUMS) rat model of depression, the CA1 region of the hippocampus showed irregularly arranged pyramidal neurons and different shapes of the mitochondria, including swollen mitochondria [Citation4]. It has been demonstrated that when rats are subjected to chronic restraint stress, their neurons exhibited shrinkage of the nucleus, pyknosis of nuclei, with irregular and dispersed chromatin. In addition, the neuronal mitochondria appeared deformed, while the synaptic clefts were blurred [Citation5].
The CUMS protocol involved successive application of different mild stressors such as 1-min tail pinch, 5-min cold swimming, overnight illumination, food and water deprivation, 15-min forced swimming etc on rats. These stressors are randomly arranged over a period of 1-week and repeated throughout the period of experiment 6-weeks, 8 = weeks or 10-weeks [Citation6]. CUMS is a well-established protocol being used for the induction of depressive-like behaviors and cognitive impairments in rat models, it is also used for studying the underlying mechanisms [Citation7]. The CUMS protocol is as classical as it could induce stress in an unpredictable routine which can be compared to the unpredictable stressors of human life with good predictive, face and construct validity [Citation8–10]. Hence, CUMS is used widely for studying antidepressants since its highly reliable and it is also associated with several neuropsychiatric disorders, like anxiety and depression [Citation11–13]. Meanwhile, the hippocampus is sensitive to CUMS, as a result it leads to the impairments of its structures and functions. Decreased expression of brain-derived neurotrophic factor (BDNF), reduced neurogenesis and altered synaptic morphology in the hippocampus has been reported in CUMS rats [Citation14,Citation15].
The current drug treatment for depression is based on selective serotonin reuptake inhibitors (SSRI’s), monoamine oxidase inhibitors (MAOI’s), and tricyclic antidepressants (TCA’s). These treatments have significantly contributed to enhancing the quality of life of individuals with depression, but they are not without their limitations. The current medications do not produce a uniform response among patients, it takes weeks for their effects to be observed and many treatments have significant side effects [Citation16]. The concurrent use of multiple drugs complicates the problems through complex interactions and in particular gives rise to uncertainty regarding their safe use in pregnancy [Citation17]. Fluoxetine is a commonly used antidepressant, and as an SSRI, it inhibits the serotonin transporters at the synaptic cleft. Though in wide use, fluoxetine has side effects including fatigue, weight gain, and sexual dysfunction [Citation18,Citation19]. Thus, though there is a wide range of medications available for the treatment of depression, none of them are universally effective or without side effects. Consequently, there is a need for new therapeutic agents with lesser side effects and broader efficacy [Citation20]. In order to achieve this, it is necessary to consider the critical physiological processes that contribute to stress and depression.
One of the major mechanisms by which stress affects the human body is through the generation of reactive oxygen species (ROS) [Citation21–23]. Malondialdehyde (MDA) is a product of degradation of polyunsaturated fatty acids and has a positive correlation with the severity of lipid peroxidation [Citation24]. Wistar rats exposed to chronic restraint stress, demonstrated increased levels of MDA in the brain, highlighting the importance of lipid peroxidation induced by chronic stress [Citation25]. In a study on patients with recurrence of depression, elevated plasma levels of MDA were observed which correlated with the severity of working memory, short-term and delayed memory [Citation26].
The ROS produced by mitochondrial oxidation within the cells in response to stress is neutralized by both non-enzymatic and enzymatic antioxidant defense systems [Citation27]. For the enzymatic antioxidant mechanisms, the most studied markers are the levels of catalase (CAT) and superoxide dismutase (SOD) in Wistar rat models [Citation23]. The principal marker for the non-enzymatic antioxidant defense mechanism is the glutathione (GSH) levels. SOD acts by converting superoxide anion radicals to hydrogen peroxide, thereby the process of superoxide anion interacting with nitric oxide to form reactive peroxynitrite is reduced [Citation23].
Oxidative stress is triggered by the imbalance between the generation of ROS in the cells and the clearance of its intermediates or repair of resulting damage. It has been reviewed that postmortem studies on oxidative stress in frontal lobes of the brain in patients with depression was increased compared to those of matched controls [Citation28]. Studies on different animal models including rats have revealed that chronic stress could contribute to depression by damaging mitochondrial function, impairing neurogenesis and affecting cell survival [Citation22,Citation29].
Centella asiatica L. Urban (CA) is a green leafy herb that is valued for its medicinal properties and has been used in traditional medicines of many countries since ancient times [Citation21]. The health benefits of CA have been described in Unani medicine in Sri Lanka, Ayurvedic medicine in India, Chinese traditional medicine, folk medicine in South Asian countries, and African traditional medicine [Citation30]. Centella asiatica has been used for its neurological actions including memory-enhancing effects [Citation31], and as a revitalizer for nerves and brain cells [Citation32]. More broadly, CA has also been used for the treatment of headache and leprosy [Citation33] wound healing, and memory enhancement [Citation34]. Centella asiatica is most commonly used in many traditional medicinal practices for its neuroprotective effects [Citation26,Citation35Citation36]. The ameliorative effects of CA on d-galactose and aluminum chloride-induced oxidative stress and cholinergic dysfunction causing neuronal degeneration, and cognitive impairment in male Wistar rats [Citation37] and lead-induced toxicity in rats [Citation38] have also been documented.
This present work explored the neuroprotective effects of CA in a CUMS rat model of depression, by studying the ultrastructure of the hippocampus and the expression of biomarkers for stress.
Materials and methods
Animals
Thirty-six male albino Wistar rats, aged 8–10 weeks and weighing 180–22 0 g, purchased from Bistari Ltd, Serdang, Selangor, Malaysia, were used in this study. The choice of males was made to exclude the influence of gender variability and the sex-dependent response to stress. The rats were kept in the Animal House, Faculty of Medicine and Health Sciences, Universiti Putra Malaysia and maintained under standard laboratory conditions (in 12:12 light/dark cycle, with the light on from 07.00 hrs to 19.00 hrs, the ambient temperature was maintained at 25 ± 2°C, and the relative humidity 50 ± 10% with access to food and water ad libitum). The rats were acclimatized to the laboratory conditions for one week and were housed in pairs per home cage. The number of rats used and the protocols followed for the study were approved by the Institutional Animal Care and Use Committee, Universiti Putra Malaysia, on 23 November 2018, with project reference number UPM/IACUC/AUP-R078/2018.
Centella asiatica and fluoxetine
The ethanolic extract of CA (Reference Number: AuRins-MIA-1-0, Atta-ur-Rahman Institute for Natural Product Discovery, Universiti Teknologi Mara (UiTM), Puncak Alam, Selangor, Malaysia) [Citation39] and fluoxetine (Flx) (Cadila Pharmaceuticals Ltd, Ahmedabad, India), were acquired from the sources indicated. The CA and Flx were administered to the rats in the morning between 9:00 a.m and 11:00 a.m. Flx was administered orally at a dosage of 10 mg/kg body weight and CA was also administered orally in dosages of 200 mg/kg, 400 mg/kg, and 800 mg/kg body weight. This dosage was chosen based on previous studies [Citation40,Citation41]. Oral administration was considered the most representative of how drugs would be administered during the treatment of psychiatric patients.
Induction of CUMS in rats
The rats were subjected to CUMS for 8 weeks by exposing them to both psychosocial and environmental stressors as previously described by Jagadeesan and Tong [Citation6,Citation20]. The rats which were not subjected to CUMS, were left in their home cages except for normal handling and cleaning. The rats that were subjected to CUMS experienced a course of nine types of mild stressors (S), with a minimum of two stressors per day for eight consecutive weeks (). These stressors were randomly administered for the first week and the same schedule was repeated throughout the rest of the experimental period. In order to avoid prophesy and adaptation, no stressor was applied consecutively.
Table 1. Chronic unpredictable mild stress procedures.
Experimental design
Following one week of acclimatization, rats were randomly divided into six groups (n = 6): Group 1, Unstressed + normal saline (control); Group 2, CUMS + normal saline (CUMS); Group 3, CUMS + Flx (10 mg/kg) (Flx); Groups 4, CUMS + CA (200 mg/kg) (CA 200); Group 5, CUMS + CA (400 mg/kg) (CA 400) and Group 6, CUMS + CA (800 mg/kg) (CA 800). Flx and CA were administered starting from day zero of the study once a day for eight weeks. The rats were euthanized on day 64, and their brain tissues were harvested for morphological studies using electron microscopy and oxidative stress examination using photospectrometry ().
Transmission electron microscopy (TEM)
The hippocampus was excised from the brains of decapitated rats and was cut into approximately 1 x 1 × 1 mm sections on a cold plate. The tissue sections were fixed in an electron microscopy (EM) grade primary fixative of 5% glutaraldehyde (Agar Scientific Ltd, UK) for 12 hours at 4°C for TEM studies. The tissue sections on the grids were stained first with uranyl acetate (Agar Scientific Ltd, UK), by placing the grid in 20 µl of uranyl acetate on parafilm for 10 minutes. The grids were then washed with filtered distilled water 3 times for 10 minutes each time. The grids were then stained with lead acetate (Agar Scientific Ltd, UK) for 10 minutes, then washed with filtered distilled water 3 times for 10 minutes each time. The grids were dried on filter papers and viewed with a TEM (LEO LIBRA-120, Japan).
Biochemical assay of oxidative stress markers
Hippocampal tissue from the study groups was weighed and homogenized in phosphate buffered saline (PBS) (tissue weight (g): PBS (mL) volume 1:9) using a tissue homogenizer. The homogenates were then subsequently centrifuged at 5000 x g for 5 minutes to get the supernatant. The total protein concentration of the rat brain tissues was measured using the bicinchoninic acid assay (BCA assay) kit, purchased from Thermo Fisher Scientific (Carlsbad, CA, USA). Bovine serum albumin (BSA) (1 mg/ml) was used as the standard in the range of 0.01–0.1 mg/ml for its comparison with the total protein.
The oxidative stress markers (MDA and SOD) were quantified using their respective assay kits by colorimetric methods. Measurements of the MDA levels were done following the manufacturer’s guide, Cayman Chemical Company, Ann Arbor (Michigan, USA). The MDA levels were expressed as nmol per mg protein. The SOD activities were measured by the water soluble tetrazolium −1 (WST-1) method. Measurements of SOD were also conducted according to the manufacturer’s manual, (Elabscience, USA). The optical density (OD) readings were done using a microplate reader at 450 nm, and the SOD activities expressed as U/mg protein.
Statistical analysis
Using the GraphPad Prism version 6 (ISI, San Diego, CA, USA) software, the data obtained were analyzed using one way ANOVA. Tukey’s post hoc comparison was used where applicable, with p < 0.05 considered as being significant and the results presented as mean ± SD.
Results
CA attenuated mitochondrial aberrations in the brains of rats exposed to CUMS
Representative electron micrographs of mitochondria in the hippocampal sections of rats from all treatment groups were recorded (). The hippocampi of the control group of rats exhibited healthy mitochondria which appeared round or ovoid-shaped with a dense matrix and parallel-arranged cristae (). Mitochondrial changes were observed in the brains of the CUMS rats in the form of elongated mitochondria, with some ruptured and others exhibiting fragmentation of the cristae (). The CUMS rats co-administered with Flx showed less mitochondrial aberrations, maintaining their parallel-arranged cristae and round or ovoid shapes (). Further, CUMS rats co-administered with CA 200, 400 and 800 mg/kg exhibited fewer damages to mitochondria of neuronal cells, although a few vacuolated mitochondria were observed in sections from rats administered with CA at the lowest dose, 200 mg/kg ()).
Figure 2. TEM images of rat’s brains showing mitochondrial aberrations in CUMS rats and those co-administered with fluoxetine and CA. a) Control group of rats showing normal mitochondria (blue arrow) with dense matrix, b) CUMS rats showing elongated and burst mitochondria (yellow arrow), c) fluoxetine group of rats showing normal mitochondria like those of the control group of rats, d) CA 200 group of rats showing normal mitochondria (blue arrow) and vacuolated mitochondria (red arrow), and e-f) CA 400 and 800 groups of rats, showing normal mitochondria.
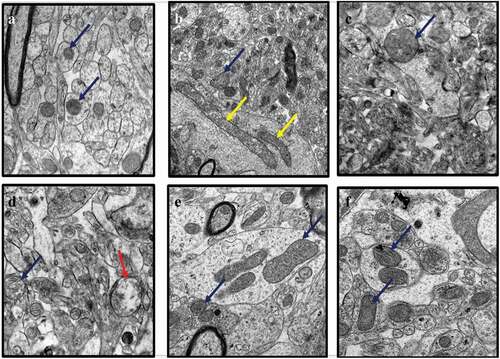
CA prevented nuclear abnormalities in the brains of rats exposed to CUMS
The effects of treatment with CA on CUMS-induced nuclear damage of the hippocampus of rats was evaluated through TEM (). In the control hippocampus tissue, the nuclei appeared normal with prominent nucleoli, double-layered nuclear membrane, and a well-distributed chromatin (). The hippocampi of the CUMS rats showed pyknotic nuclei, degenerated chromatin and nucleoli (). Administration of fluoxetine to CUMS rats prevented some nuclear abnormalities as the sections showed normal nucleus with nucleoli in the hippocampi (). Administration of CA (200, 400, and 800 mg/kg) prevented some of the morphological changes seen in CUMS rats ()). However, again, the hippocampal sections from the lowest dose CA treatment group (200 mg/kg) showed some distorted nuclear membrane and degenerated chromatin with less prominent nucleoli ()).
Figure 3. TEM micrographs of rat’s brains showing nuclear abnormalities in CUMS rats and those co-administered with Fluoxetine and CA. a) Control group of rats showing nucleoli (red arrow), evenly distributed chromatin and double nuclear membrane (blue arrow), b) CUMS rats showing pyknotic nucleus and crescent formation, c) fluoxetine group of rats showing normal nucleus with double nuclear membrane (blue arrow), d) CA 200 group of rats showing nucleus with degenerated chromatin and gaps in their nuclear membrane (purple arrow), e-f) CA 400 and 800 groups of rats with normal nucleus showing intact nuclear membrane (blue arrow) and nucleoli (red arrow).
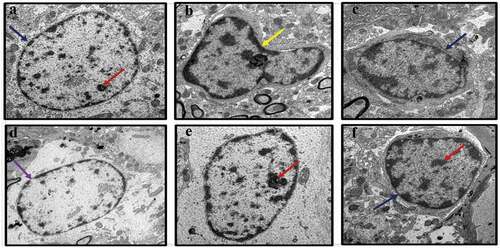
CA treatment prevented myelin sheath defects in the brains of rats exposed to CUMS
The effects of treatment with CA on CUMS-induced myelination changes in rat hippocampal tissue were investigated (). The myelin sheaths of neurons in the sections from the untreated control group of rats were highly electron-dense, thick, continuous, and tightly wrapped around the axonal cytoplasmic membranes. The CUMS rats model exhibited myelin defects which included the loose wrapping of the myelin sheath around the cytoplasmic membranes of axons, bulging of the sheaths, and discontinuous myelination of axons (. The administration of Flx to the CUMS rats prevented some of the morphological aberrations caused by CUMS. The administration of CA (400 and 800 mg/kg) protected the rats from the myelin sheath defects ()).
Figure 4. TEM micrographs of rat brains showing myelin sheath defects in CUMS rats and those co-administered with fluoxetine and CA. a) Control group of rats showing normal myelin sheaths appeared dense, thick and tightly wrapped around their axons (blue arrow). b) The CUMS rats showing discontinuous myelin (purple arrow) and onion-like bulging (red arrow) of their myelin sheaths. c) fluoxetine group of rats showed normal myelin sheaths. d) CA 200 group of rats showing distorted myelin sheaths (yellow arrow). e) CA 400 group of rats showing normal myelin sheath tightly wrapped around an axon (blue arrow) and f) CA 800 group of rats showing normal myelin sheath.
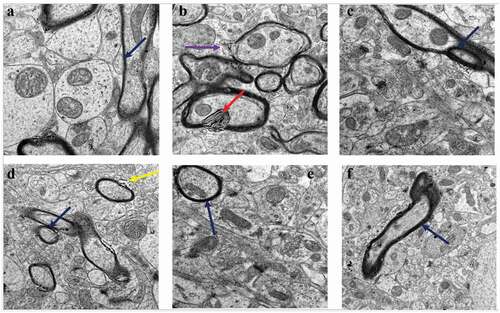
CA treatment prevented synaptic irregularities in the brains of rats exposed to CUMS
The synaptic morphology in the hippocampus sections of the rats was evaluated to determine the protective effects of CA in the CUMS rats (). Sections from the untreated control group of rats () exhibited an abundance of normal synapses with intact pre and postsynaptic vesicles. However, the sections from CUMS rats showed a marked reduction in the number of synapses, which also had blurred synaptic clefts and irregular synaptic connections (). Administration of Flx to the CUMS rats prevented these morphological aberrations (). While the hippocampal sections from CUMS rats that were treated with CA (400 and 800 mg/kg) had higher numbers of normal synapses compared to those of the CUMS rats model ()).
Figure 5. TEM micrographs of rat’s brains showing synaptic abnormalities in CUMS rats and those co-administered with fluoxetine and CA. a) Control group of rats showing abundant synapses (red arrows). b) CUMS rats showing few synapse. c) fluoxetine group of rats showing many normal looking synapses. d) CA 200 group of rats showing less numbers of synapses. e-f) CA 400 and 800 groups of rats showing abundant normal synapses.
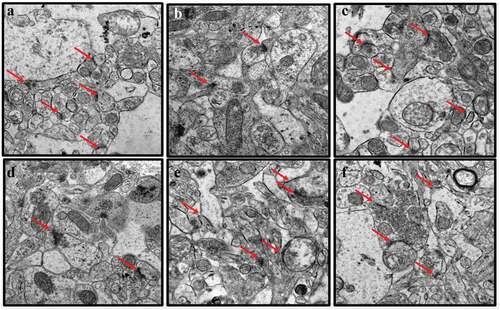
CA treatments prevented upsurge of MDA levels in CUMS rats brain tissues
The probable antioxidant capacity of CA in CUMS rats was evaluated by measuring the levels of MDA in their brains. There were significant differences in the levels of MDA among the various groups of rats [F (5, 12) = 38.94, p < 0.001] (). Significant increases in MDA levels were observed in the CUMS rats model (9.42 ± 0.456, p < 0.001) when compared to the control group of rats (5.24 ± 0.35). Significant decreases were observed in the levels of MDA in the CUMS rats administered with Flx (5.37 ± 0.35, p < 0.001), CA 200 (7.37 ± 0.701, p < 0.001), CA 400 (5.96 ± 0.503, p < 0.001) and CA 800 (5.36 ± 0.25, p = 0.0001), when compared to CUMS model group (9.42 ± 0.456). Significant increases of MDA levels in the CUMS model group (9.42 ± 0.456, p < 0.001) and CA 200 (7.37 ± 0.701, p < 0.001) groups of rats, when compared to the Flx (5.37 ± 0.35, p < 0.001) group of rats. No statistically significant differences were observed between Flx and CA (400 and 800) groups of rats.
CA treatment increased the levels of SOD in CUMS rats brain tissues
The levels of SOD in the rat brains were evaluated to check the protective effects of CA on CUMS rats. There were significant differences in the level of SOD among the various groups of rats [F (5, 12) = 9.792, p < 0.001] (). Significant decreases of the levels of SOD in the CUMS model group of rats (7.6 ± 1.07, p < 0.001) when compared to the control group of rats (14.67 ± 1.145). Conversely, significant increases in SOD levels were observed in the CUMS rats which were administered with Flx (11.97 ± 1.89, p = 0.016), CA 400 (12.3 ± 1.15, p = 0.009) and CA 800 (13.27 ± 1.51, p = 0.002), when compared to CUMS model group (7.6 ± 1.07). No significant differences were observed between Flx and CA (400 and 800) groups of rats.
Discussion
The hippocampus as a part of the limbic system has a role in the modulation of depressive mood, processing of information, and behavioral changes in depression [Citation42]. Decreased hippocampal volumes have been detected in humans based on postmortem studies and MRI studies on patients suffering from major depression (MD) [Citation43,Citation44]. Shrinkage of the dendrites of CA3 and dentate gyrus neurons and the loss of spines of CA1 neurons have also been reported in the hippocampus of the rat brains subjected to stress-induced depression [Citation45]. Further, studies have reported ultrastructure aberrations in CUMS rodents, including mitochondrial atrophy, enlarged endoplasmic reticulum, condensation of the chromatin material, distortion of mitochondrial structure, and damages of other structures in their hippocampus [Citation46]. Decreased synaptic density in mice hippocampus [Citation47] and decreased dendritic spine and synaptic density in medial prefrontal neurons [Citation48] were also observed in stress-induced rat models. Decreased postsynaptic density in the CA1 region of the hippocampus was also observed in CUMS mice models of depression [Citation49]. In conformity with the preceding reports, marked ultrastructure aberrations were observed in the brain of the CUMS rats model in the present study. Based on the available literature to date, the present study is the first to report on the protective effects of CA on the neuronal ultrastructural aberrations observed in the mitochondria, myelin sheaths, nucleus, and synapses of the hippocampus of CUMS-induced depressive-like rats.
The structural integrity of mitochondria is of paramount importance for effective energy generation and cell survival. The CUMS rats have been reported to exhibit mitochondrial alterations like enlargement, fragmentation, and disappearance of cristae which resulted in empty intermembrane space [Citation50Citation51]. Similarly, TEM images in the present study also revealed some unique mitochondrial alterations, including elongation of mitochondria, fragmentation, and loss of cristae, vacuolation, and breach of the outer membrane of mitochondria in the hippocampus of CUMS rats models. The aforementioned changes could disturb the normal mitochondrial functions such as the electron transport chain [Citation49]. Of the particular significance in the present study is that the administration of CA prevented the mitochondrial aberrations which could be the reason for the reversal of the depressive-like behaviors in the rats as previously reported [Citation6].
Structural damages to the nucleus in CUMS exposed rats model of depression, including chromatin aggregation, condensation, margination, and nuclear karyopyknosis, have been reported and were considered as indicators of apoptotic changes [Citation44]. In the present study, the CUMS rats also exhibited nuclear structural changes like pyknosis, degeneration of chromatin, and disappearance of nucleoli. The administration of fluoxetine or CA (400 and 800 mg/kg) to CUMS rats prevented some of the nuclear changes. This finding is similar to those of earlier reports demonstrating that CA prevented nuclear abnormalities in the prefrontal cortex of the D-gal/AlCl3 mediated model of AD-rats [Citation41].
The myelin sheath plays a vital role in the central nervous system because of its role in the trophic support of axons and rapid signal conduction [Citation52]. The myelin sheath also has a role in the protection and insulation of axons. Hence, damage to the myelin sheaths could result in severe neurological deficits affecting the normal signaling, and leading therefore to neurological dysfunction. An increase in the number of demyelinated axons and destruction of the myelin sheath around axons were reported in rats exposed to either physiological or physical stressors [Citation53]. It has been reviewed that demyelination is associated with anxiety- and depression-related behaviors in rodents [Citation54]. In this study, CUMS rats also exhibited defects of the myelin sheaths which include detachment from the axons, discontinuous, and bulging of myelin sheath. These structural anomalies of the myelin sheath could be attributed to the depression-like behaviors and cognitive deficits observed in the CUMS rats [Citation6]. Prevention of the structural alterations was seen in fluoxetine and CA (400 and 800 mg/kg) treated groups of rats in the current study. Rao and his team have also reported that CA extract significantly increased the dendritic arborization of hippocampal CA3 neurons in vivo studies on rats [Citation55]. In another study on chronic mild stress-induced rat models of depression, it was observed that fluoxetine delayed the development of white matter demyelination [Citation56].
Cumulative evidence has shown that biological mechanisms of depression lie in the dysfunction of synaptic plasticity, particularly in the hippocampus and prefrontal cortex [Citation5,Citation42]. In a previous study, a significant loss of postsynaptic density was reported in CUMS rats [Citation39]. Similarly, CUMS rats from the present study revealed synaptic morphological alterations such as less number of synapses with fewer pre and post-synaptic vesicles, blurry synapses, and irregular synaptic clefts which were all prevented by the administration of CA.
To further understand the mechanisms of ROS in the pathogenesis of depression, researchers have subjected rats to CUMS, as an appropriate model of depression, widely accepted as a clinically relevant model for depression [Citation57,Citation58]. It has been revealed that in humans and rat models of depression there was a significant decrease in plasma concentrations of antioxidants such as SOD with an increase in the levels of MDA [Citation28]. In conformity with previous research, marked changes in the levels of the oxidative stress marker MDA and antioxidant SOD were observed in the brain of CUMS rat models of depression in the current study. The present study is the first to report the neuroprotective effect of CA on the oxidative stress in CUMS rat models of depression. MDA is highly reactive and produced as an end result of lipid peroxidation of polyunsaturated fatty acids by ROS, which causes toxic neuronal damage [Citation59]. It has also been observed that chronic stress increases level of MDA in various parts of the brain including hippocampus [Citation60,Citation61]. In a study, rats subjected to chronic unpredictable stress showed increases in the level of MDA in the prefrontal cortex and hippocampus [Citation62]. In the current study, increase in the levels of MDA in the hippocampus of CUMS rat models of depression were demonstrated in comparison to the control group of rats. It was also observed that the levels of MDA in the hippocampus were significantly lower in the rat groups which were administered with Flx and CA (400 and 800 mg/kg) in comparison to CUMS rat models. There were no significant difference in the levels of MDA between the Flx and CA (400 and 800 mg/kg) treated groups, respectively.
Superoxide dismutase is an important enzyme involved in the first line of defense against the ROS (Hajipour et al., 2016) [Citation63]. Lower levels of SOD could be responsible for an increase in lipid peroxidation of the brain of rats. A study on Wistar rats subjected to stress with chronic restraint revealed a reduction in activity of SOD in their brains, thereby enhancing an oxidative stress [Citation64]. Depressive-like behavioral changes and decreased levels of SOD were reported in rats subjected to unpredictable chronic stress [Citation65,Citation66]. The result of the current study confirmed that the levels of SOD in the hippocampus were significantly reduced in comparison with the control group. The findings of this study agree with those of the previous studies [Citation58,Citation59]. The present study revealed an increase in the levels of SOD in the hippocampus among rats administered Flx and CA (400 and 800 mg/kg) and prevented the manifestations of stress. No significant differences were observed between the rats administered Flx and those administered CA (400 and 800 mg/kg).
Conclusion
The present study indicated that CA prevented neuronal damage in the hippocampus of CUMS rat models. Therefore, treatment with CA could be a promising approach for the corrections of neuronal defects in CUMS rats. The study supports the hypothesis that CA prevents oxidative stress-induced neuronal damage in the hippocampus of CUMS rat models ().
Ethical Issues
The study was approved by the Institutional Animal Care and Use Committee, Universiti Putra Malaysia, on 23 November 2018, with a project reference number UPM/IACUC/AUP-R078/2018.
Acknowledgments
The authors acknowledged the contributions made by Imaging Centre UiTM Puncak Alam and Imaging Centre University of Malaya.
Disclosure statement
The authors declared no conflict of interest.
Additional information
Funding
References
- Cano-Lopez I, Gonzalez-Bono E. Cortisol levels and seizures in adults with epilepsy: a systematic review. Neurosci Biobehav Rev. 2019;103:216–229.
- Segerstrom SC, Miller GE. Psychological stress and the human immune system: a meta-analytic study of 30 years of inquiry. Psychol Bull. 2004;130(4):601–630.
- Masi G, Brovedani P. The hippocampus, neurotrophic factors and depression: possible implications for the pharmacotherapy of depression. CNS Drugs. 2011;25(11):913–931.
- Gu HF, Nie YX, Tong QZ, et al. Epigallocatechin-3-gallate attenuates impairment of learning and memory in chronic unpredictable mild stress-treated rats by restoring hippocampal autophagic flux. PLoS ONE. 2014;9(11):11.
- Liang Y, Guo XL, Chen JX, et al. Effects of the Chinese traditional prescription Xiaoyaosan decoction on chronic immobilization stress-induced changes in behavior and ultrastructure in rat hippocampus. Evid Based Complement Alternat Med. 2013;2013:1–8.
- Jagadeesan S, Musa Chiroma S, Baharuldin MTH, et al. Centella asiatica prevents chronic unpredictable mild stress-induced behavioral changes in rats. Biomed Res Ther. 2019;6(6):3233–3243.
- Jia KK, Zheng YJ, Zhang YX, et al. Banxia-houpu decoction restores glucose intolerance in CUMS rats through improvement of insulin signaling and suppression of NLRP3 inflammasome activation in liver and brain. J Ethnopharmacol. 2017;209:219–229.
- Wang GL, Wang YP, Zheng JY, et al. Monoaminergic and aminoacidergic receptors are involved in the antidepressant-like effect of ginsenoside Rb1 in mouse hippocampus (CA3) and prefrontal cortex. Brain Res. 2018;1699:44–53.
- Gupta GL, Fernandes J. Protective effect of Convolvulus pluricaulis against neuroinflammation associated depressive behavior induced by chronic unpredictable mild stress in rat. Biomed Pharmacother. 2019;109:1698–1708.
- Willner P. The chronic mild stress (CMS) model of depression: history, evaluation and usage. Neurobiol Stress. 2017;6:78–93.
- Pekala K, Budzynska B, Biala G. Utility of the chronic unpredictable mild stress model in research on new antidepressants. Curr Issues Pharm Med Sci. 2014;27(2):97–101.
- Karson A, Demirtaş T, Bayramgürler D, et al. Chronic administration of Infliximab (TNF‐α Inhibitor) decreases depression and anxiety‐like behaviour in rat model of chronic mild stress. Basic Clin Pharmacol Toxicol. 2013;112(5):335–340.
- Zhao Y, Wang Q, Jia M, et al. (+)-Sesamin attenuates chronic unpredictable mild stress-induced depressive-like behaviors and memory deficits via suppression of neuroinflammation. J Nutr Biochem. 2019;64:61–71.
- Stockmeier CA, Mahajan GJ, Konick LC, et al. Cellular changes in the postmortem hippocampus in major depression. Biol Psychiatry. 2004;56(9):640–650.
- Feng SF, Shi TY, Wang WN, et al. Long-lasting effects of chronic rTMS to treat chronic rodent model of depression. Behav Brain Res. 2012;232(1):245–251.
- Stassen HH, Angst J, Hell D, et al. Is there a common resilience mechanism underlying antidepressant drug response? Evidence from 2848 patients. J Clin Psychiatry. 2007;68(5):9882.
- Källén B. Neonate characteristics after maternal use of antidepressants in late pregnancy. Arch Pediatr Adolesc Med. 2004;158(4):312–316.
- Bijlsma EY, Chan JS, Olivier B, et al. Sexual side effects of serotonergic antidepressants: mediated by inhibition of serotonin on central dopamine release? Pharmacol Biochem Behav. 2014;121:88–101.
- Bleakley S. Review of the choice and use of antidepressant drugs. Prog Neurol Psychiatry. 2013;17(6):18–26.
- Tong J, Zhou Z, Qi W, et al. Antidepressant effect of helicid in chronic unpredictable mild stress model in rats. Int Immunopharmacol. 2019;67:13–21.
- Sabaragamuwa R, Perera CO, Fedrizzi B. Centella asiatica (Gotu kola) as a neuroprotectant and its potential role in healthy ageing. Trends Food SciTechnol. 2018;79:88–97.
- Lucca G, Comim CM, Valvassori SS, et al. Effects of chronic mild stress on the oxidative parameters in the rat brain. Neurochem Int. 2009;54(5–6):358–362.
- Maritim AC, Sanders RA, Watkins JB. Diabetes, oxidative stress, and antioxidants: a review. J Biochem Mol Toxicol. 2003;17(1):24–38.
- Samarghandian S, Azimi-Nezhad M, Borji A, et al. Protective effects of carnosol against oxidative stress induced brain damage by chronic stress in rats. BMC Complement Altern Med. 2017;17(1):1–7.
- Samarghandian S, Farkhondeh T, Samini F, et al. Protective effects of carvacrol against oxidative stress induced by chronic stress in Rat’s Brain, Liver, and Kidney. Biochem Res Int. 2016;2016:1–7.
- Talarowska M, Gałecki P, Maes M, et al. Malondialdehyde plasma concentration correlates with declarative and working memory in patients with recurrent depressive disorder. Mol Biol Rep. 2012;39(5):5359–5366.
- Ray PD, Huang BW, Tsuji Y. Reactive oxygen species (ROS) homeostasis and redox regulation in cellular signaling. Cell Signal. 2012;24(5):981–990.
- Maes M, Galecki P, Chang YS, et al. A review on the oxidative and nitrosative stress (O&NS) pathways in major depression and their possible contribution to the (neuro)degenerative processes in that illness. Prog Neuro Psychopharmacol Biol Psychiatry. 2011;35(3):676–692.
- Li T, Liu H, Wang X, et al. Resveratrol exerts antidepressant properties in the chronic unpredictable mild stress model through the regulation of oxidative stress and mTOR pathway in the rat hippocampus and prefrontal cortex. Behav Brain Res. 2016;302:191–199.
- Jahan R, Hossain S, Seraj S, et al. Centella asiatica (L.) Urb.: ethnomedicinal uses and their scientific validations. Am-Eurasian J Sustainable Agric. 2012;6(4):261–270.
- Orhan IE. Centella asiatica (L.) Urban: from traditional medicine to modern medicine with neuroprotective potential. Evid Based Complement Alternat Med. 2012;2012:1–8.
- Seevaratnam V, Banumathi P, Premalatha MR, et al. Functional properties of Centella asiatica (L.): a review. Int J Pharm Pharm Sci. 2012;4(5):8–14.
- Shukla A, Rasik AM, Dhawan BN. Asiaticoside‐induced elevation of antioxidant levels in healing wounds. Phytother Res. 1999;13(1):50–54.
- Sari DCR, Aswin S, Susilowati R, et al. Ethanol extracts of Centella asiatica leaf improves memory performance in rats after chronic stress via reducing nitric oxide and increasing brain-derived neurotrophic factor (BDNF) concentration. GSTF J Psychol. 2014;1(1):1–7.
- Brinkhaus B, Lindner M, Schuppan D, et al. Chemical, pharmacological and clinical profile of the East Asian medical plant Centella aslatica. Phytomedicine. 2000;7(5):427–448.
- Chiroma SM, Moklas MAM, Norma CM, et al. Neuro-therapeutic benefits of Centella asiatica on some neurodegenerative diseases: a review. Res J Pharm Biol Chem Sci. 2017;8(6):549–556.
- Chiroma SM, Baharuldin MTH, Mat Taib CN, et al. Protective effects of centella asiatica on cognitive deficits induced by D-gal/AlCl3 via inhibition of oxidative stress and attenuation of acetylcholinesterase level. Toxics. 2019;7(2):19.
- Sainath SB, Meena R, Supriya C, et al. Protective role of Centella asiatica on lead-induced oxidative stress and suppressed reproductive health in male rats. Environ Toxicol Pharmacol. 2011;32(2):146–154.
- Binti Mohd Yusuf Yeo NA, Muthuraju S, Wong JH, et al. Hippocampal amino‐3‐hydroxy‐5‐methyl‐4‐isoxazolepropionic acid GluA1 (AMPA GluA1) receptor subunit involves in learning and memory improvement following treatment with Centella asiatica extract in adolescent rats. Brain Behav. 2018;8(9):e01093.
- Chiroma SM, Baharuldin MTH, Mat Taib CN, et al. Centella asiatica protects d-galactose/AlCl3 mediated Alzheimer’s disease-like rats via PP2A/GSK-3β signaling pathway in their Hippocampus. Int J Mol Sci. 2019;20(8):1871.
- Chiroma SM, Baharuldin MTH, Taib CNM, et al. Protective effect of Centella asiatica against D-galactose and aluminium chloride induced rats: behavioral and ultrastructural approaches. Biomed Pharmacother. 2019;109:853–864.
- Fournier NM, Duman RS. Illuminating hippocampal control of fear memory and anxiety. Neuron. 2013;77(5):803–806.
- Keller J, Shen L, Gomez RG, et al. Hippocampal and amygdalar volumes in psychotic and nonpsychotic unipolar depression. Am J Psychiatry. 2008;165(7):872–880.
- Vakili K, Pillay SS, Lafer B, et al. Hippocampal volume in primary unipolar major depression: a magnetic resonance imaging study. Biol Psychiatry. 2000;47(12):1087–1090.
- McEwen BS. Stress and hippocampal plasticity. Annu Rev Neurosci. 1999;22(1):105–122.
- Fan C, Song Q, Wang P, et al. Neuroprotective effects of curcumin on IL-1β-induced neuronal apoptosis and depression-like behaviors caused by chronic stress in rats. Front Cell Neurosci. 2019;12(516). DOI:10.3389/fncel.2018.00516
- Shen J, Xu L, Qu C, et al. Resveratrol prevents cognitive deficits induced by chronic unpredictable mild stress: sirt1/miR-134 signalling pathway regulates CREB/BDNF expression in hippocampus in vivo and in vitro. Behav Brain Res. 2018;349:1–7.
- Hu F, Ge MM, Chen WH. Effects of lead exposure on dendrite and spine development in hippocampal dentate gyrus areas of rats. Synapse. 2016;70(3):87–97.
- Zhao Y, Wang Q, Jia M, et al. (+)-Sesamin attenuates chronic unpredictable mild stress-induced depressive-like behaviors and memory deficits via suppression of neuroinflammation. J Nutr Biochem. 2019;64:61–71.
- Zhang J, Zhang Z, Zhang J, et al. Electroacupuncture improves antidepressant effects in CUMS rats by protecting hippocampal synapse and mitochondrion: an ultrastructural and iTRAQ proteomic study. Evid Based Complement Alternat Med. 2019. 1-11.
- Gong Y, Chai Y, Ding JH, et al. Chronic mild stress damages mitochondrial ultrastructure and function in mouse brain. Neurosci Lett. 2011;488(1):76–80.
- Douglas Fields R. A new mechanism of nervous system plasticity: activity-dependent myelination. Nat Rev Neurosci. 2015;16(12):756–767.
- Meknatkhah S, Sharif Dashti P, Mousavi MS, et al. Psychological stress effects on myelin degradation in the cuprizone‐induced model of demyelination. Neuropathology. 2019;39(1):14–21.
- Edgar N, Sibille E. A putative functional role for oligodendrocytes in mood regulation. Transl Psychiatry. 2012;2(5):e109–e109.
- Gadahad MRK, Rao M, Rao G. Enhancement of hippocampal CA3 neuronal dendritic arborization by Centella asiatica (Linn) fresh leaf extract treatment in adult rats. J Chin Med Assoc. 2008;71(1):6–13.
- Gao Y, Yao Y, Liang X, et al. Changes in white matter and the effects of fluoxetine on such changes in the CUS rat model of depression. Neurosci Lett. 2019;694:104–110.
- Andrus BM, Blizinsky K, Vedell PT, et al. Gene expression patterns in the hippocampus and amygdala of endogenous depression and chronic stress models. Mol Psychiatry. 2012;17(1):49–61.
- Bondi CO, Rodriguez G, Gould GG, et al. Chronic unpredictable stress induces a cognitive deficit and anxiety-like behavior in rats that is prevented by chronic antidepressant drug treatment. Neuropsychopharmacology. 2008;33(2):320–331.
- Romero FJ, Bosch-Morell F, Romero MJ, et al. Lipid peroxidation products and antioxidants in human disease. Environ Health Perspect. 1998;106(SUPPL. 5):1229–1234.
- Abelaira HM, Réus GZ, Ribeiro KF, et al. Effects of lamotrigine on behavior, oxidative parameters and signaling cascades in rats exposed to the chronic mild stress model. Neurosci Res. 2013;75(4):324–330.
- Bhattacharya A, Ghosal S, Bhattacharya SK. Antioxidant activity of tannoid principles of Emblica officinalis (amla) in chronic stress induced changes in rat brain. Indian J Exp Biol. 2000;38(9):877–880.
- Che Y, Zhou Z, Shu Y, et al. Chronic unpredictable stress impairs endogenous antioxidant defense in rat brain. Neurosci Lett. 2015;584:208–213.
- Hajipour S, Sarkaki A, Farbood Y, et al. Effect of gallic acid on dementia type of Alzheimer disease in rats: electrophysiological and histological studies. Basic Clin Neurosci. 2016;7(2):97–106.
- Nawaz A, Batool Z, Shazad S, et al. Physical enrichment enhances memory function by regulating stress hormone and brain acetylcholinesterase activity in rats exposed to restraint stress. Life Sci. 2018;207:42–49.
- Kumar B, Kuhad A, Chopra K. Neuropsychopharmacological effect of sesamol in unpredictable chronic mild stress model of depression: behavioral and biochemical evidences. Psychopharmacology (Berl). 2011;214(4):819–828.
- Fernandes J, Gupta GL. N-acetylcysteine attenuates neuroinflammation associated depressive behavior induced by chronic unpredictable mild stress in rat. Behav Brain Res. 2019;364:356–365.