ABSTRACT
Glucosamine, an amino-polysaccharide, has been widely used for alleviating osteoarthritis. . In the present study, attempts have been made to evaluate the potential role of glucosamine, a caloric restriction mimetic (CRM), for erythrocyte membrane transporter protection in D-galactose (D-gal) induced accelerated and natural aging models of rat specifically Ca2+-ATPases (PMCA pump), Na+/K+-ATPases (NKA pump), and the Na+/H+ exchanger (NHE) and redox biomarkers during aging. The study comprised of young (3–4 months old; 150 ± 20 g), naturally aged (above 24 months; 420 ± 20 g) and D-galactose-induced aged (3–4 months old; 150 ± 20 g, administered with D-Gal at 300 mg/kg B.W., subcutaneously) male albino rats of Wistar strain. All the rats were supplemented with Glucosamine hydrochloride (300 mg/kg body weight) for 12 weeks. There was a significant (P < 0.05) decrease in the activity of Ca2+-ATPases, Na+/K+-ATPases and induced NHE activity in D-Gal and naturally old rats. Levels of redox biomarkers such as intracellular Ca2+ ion, protein carbonyl, and lipid hydroperoxides were also found to be increased significantly (P < 0.05). These results were found to be reversed in the rats supplemented with glucosamine. Our findings suggest that glucosamine supplementation improves ion homeostasis by protecting the erythrocyte membrane transporters against age-related alterations.
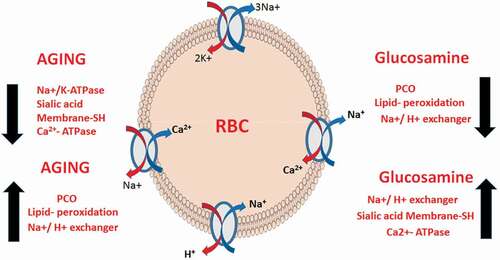
Introduction
Aging is a multifactorial process characterized by the progressive accumulation of molecular and cellular damages leading to functional deficits. Multiple molecular and cellular signaling events interface with environmental factors to maintain cellular homeostasis and longevity [Citation1]. The cumulative failure of these evolutionarily conserved pathways on account of external and endogenous stressors leads to a decline in various cellular functions and makes the organism vulnerable to several chronic diseases. There is a substantial amount of the literature detailing a decline in cellular homeostasis with increasing age [Citation2].
The plasma membrane regulates many aspects of cell physiology and signaling through the coordination of specific transporters and pumps [Citation3]. These membrane transporter proteins are crucial for the maintenance of ionic homeostasis between the extra- and intracellular environments [Citation4]. However, these proteins are vulnerable to oxidative modifications, which leads to changes in ionic concentrations inside the cell [Citation4]. This ionic imbalance leads to diverse cellular alterations that may contribute to the process of aging and age-related disorders [Citation5].
The mammalian erythrocyte membrane provides a valuable model to investigate aging-related changes [Citation6,Citation7]. Erythrocyte membrane-bound transporters or pumps such as Ca2+ATPase (PMCA pump), Na+/K+-ATPase (NKA pump), and Na+/H+ exchanger (NHE) use the energy of ATP hydrolysis to move ions or small molecules across the membrane against a chemical concentration gradient or electric potential [Citation8]. A close relationship has been established between the impairment in the activities of various membrane transporters and the development of pathologies [Citation9].
Glucosamine (GlcN), 2-amino-2-deoxy-D-glucose, is a naturally occurring amino sugar found in the human body. It is an important component of glycoproteins, proteoglycans, and glycosaminoglycans, which is a major component of joint cartilage [Citation10]. GlcN has a potent background as a glycolytic inhibitor [Citation11,Citation12]. Its entry into cells is stimulated by insulin and involves the glucose-transporter system [Citation13]. GlcN in its phosphorylated form (GlcN-6-phosphate), acts as an inhibitor of hexokinase, the first enzyme of glycolysis. Researchers have introduced a novel biological and pharmacological application of GlcN as a caloric restriction mimetic (CRM) [Citation14]. Recently, we have reported that GlcN supplementation results in an improvement in aging biomarkers in erythrocytes and plasma by inducing a transient mitohormetic increase in ROS [Citation15].
Rodents chronically injected with D-galactose (D-Gal) have been widely used as an animal aging model for anti-aging pharmacology research [Citation16,Citation17]. In several recent papers, we have used this accelerated senescence model of rat to study the efficacy of various compounds acting as CRMs [Citation18,Citation19]. Based on the property of GlcN to inhibit glycolysis, we thought it would be pertinent to assess its CRM effect on naturally aged and accelerated aging model of rat. The present study investigated the modulatory role of GlcN on the regulation of erythrocyte membrane integrity and membrane-bound transporters during the aging process in natural and D-gal induced aging models of rats. It is important to mention that although some previous studies have reported the effect of GlcN on membrane transporters in conditions such as obesity and diabetes, no study has yet been done on aged rats.
Material and methods
Reagents and chemicals
Glucosamine hydrochloride was purchased from TCI Chemicals (India) Pvt. Ltd. DIDS (4, 4-diisothiocyanatostilbene-2, 20-disulphonic acid), amiloride hydrochloride, and ouabain were acquired from Sigma-Aldrich (USA). Every other chemical used in the experiments was of the highest purity available from Merck (Germany) and HIMEDIA Labs (Mumbai, India).
Animal model and the study protocol
All the protocols and procedures involved in this study were approved by the Ethical Committee of University of Allahabad. Male Wistar rats of two age groups, 4 months old (B.W. 150 ± 20 g, considered young) and 24 months old (B.W. 350 ± 20 g, considered old) were used for the study and collected from the CSIR-Indian Institute of Toxicology Science, India. They were housed in polypropylene cages in a controlled environment (12 hr light/dark cycle, at 22 ± 2°C). Rats were fed upon nutrient-rich laboratory pallets with free access to drinking water. After acclimatization, 36 rats were divided randomly into the following 6 groups (n = 6):
Group I Young control: young rats received 0.9% saline orally as a vehicle once daily for 12 weeks.
Group II Young GlcN: young rats received glucosamine hydrochloride (GlcN) (300 mg/kg B.W. dissolved in 0.9% saline) orally for 12 weeks [Citation15].
Group III D-Gal induced senescence: young rats were administered with D-galactose (300 mg/kg B.W.) subcutaneously once daily for 12 weeks [Citation16].
Group IV D-Gal GlcN: young rats were administered D-galactose (300 mg/kg B.W., subcutaneously) and GlcN (300 mg/kg B.W. dissolved in 0.9%saline, orally with gavage) once daily for 12 weeks.
Group V Old control: old rats received 0.9% saline orally with gavage once daily for 12 weeks as a vehicle.
Group VI Old GlcN: old rats were administered GlcN (300 mg/kg B.W. dissolved in 0.9% saline) once daily for 12 weeks.
Isolation of packed red blood cells (PRBCs) and preparation of erythrocyte membrane
After the completion of 12 weeks, rats were sacrificed under light ether anesthesia. The blood was collected by cardiac puncture using heparinized syringes. It was immediately centrifuged at 800xg for 10 min at 4°C. After removing plasma and buffy coat, the PRBCs were washed twice using phosphate buffer saline (PBS) and were resuspended into glucose-PBS. The erythrocyte membrane (ghosts) was prepared following the protocol of Marchesi and Palade [Citation20].
Na+/K+-ATPase (NKA) activity
NKA activity was measured by following the protocol described earlier by us [Citation21]. The protocol involves spectrophotometric measurement of inorganic phosphate (Pi), released from the ATP hydrolysis in the presence of ouabain, which is a specific enzyme inhibitor. The assay buffer contains 140 mmol/L NaCl, 20 mmol/L KCl, 3 mmol/L MgCl2 and 30 mmol/L imidazole at pH 7.25. The assay buffer and erythrocyte membrane (0.4–0.9 mg protein/ml) were incubated with or without ouabain (5 × 10−4 mol/l) and in parallel ATP (6 mmpl/L) at room temperature for 30 minutes. After the specified time, the reaction was put to an end by adding 3.5 ml of stop solution containing 0.5 mM H2SO4, 0.5% ammonium molybdate, and 2% sodium dodecyl sulfate (SDS). The amount of Pi released, which is expressed as µmol Pi/mg protein/hour at 37°C.
Plasma membrane Ca2+ -ATPase (PMCA) activity
The erythrocyte PMCA activity was estimated by following the protocol of Pandey and Rizvi [Citation9]. The erythrocyte membrane (200 µl) was incubated with a reaction medium (containing 3 mM MgCl2, 80 mM NaCl, 15 mM KCl, 0.1 mM EGTA, 50 mM Tris-HCl (pH 7.4) and 0.5 mM ouabain) with or without CaCl2 (0.2 mM) at 37°C for 30 minutes. Calmodulin was present at the concentration of 40 units/ml. 6 mM ATP was used to initiate the reaction. The stop solution contains 1.4 ml of a cold solution containing 0.5 M H2SO4, 0.5% ammonium molybdate, and 2% SDS. The samples were shaken for 10 min and 0.04 ml of a solution containing 1.2% sodium metabisulphite, 1.2% sodium sulfite and 0.2% of 1-amino-2-naphthol-4-sulfonic acid (ANSA) was added to each sample. After incubating the reaction mixture for 30 minutes, the samples were centrifuged at 800 × g for 5 minutes and the absorbance was taken at 650 nm. The activity of PMCA was expressed as µmol Pi/mg protein/hour at 37°C.
Na+/H+ exchanger (NHE) activity
NHE activity was measured by following the protocol described by Matteucci et al [Citation22]). Freshly isolated PRBCs were suspended in a medium containing 1 mM KCl, 150 mM NaCl, 1 mM MgCl2, and 10 mM glucose for 5 minutes under magnetic stirring at room temperature. 0.2 M HCl was used to bring the pH of the solution to 6.35–6.45. Then, 0.2 mM DIDS was added to the reaction mixture and the pH was brought to 7.95–8.00 by adding 0.05 M NaOH. In a parallel set of experiments, amiloride was added along with DIDS. The rate of NHE was expressed as proton efflux mmol/L RBC/hour at 37̊C.
Determination of intracellular Ca2+ levels
Intracellular Ca2+ ion concentration was determined by following the protocol [Citation8]. In brief, PRBCs were resuspended in assay buffer containing 120 mmol/L NaCl, 5 mmol/L KCl, 1.2 mmol/L MgCl2, 5 mmol/L NaHCO3, 6 mmol/L glucose, 5 mmol/L CaCl2, 25 mmol/L Hepes with pH 7.4). Suspended RBCs were stained with Quin-2AM (25 mmol/L) for 60 minutes in absence of light. After washing thrice, the cells were resuspended in a reaction buffer and the fluorescence was measured at excitation/emission wavelengths of 330 and 492 nm, respectively, and expressed in nM.
Determination of membrane protein carbonyl
The protocol by Levine et al [Citation23] was used to express the erythrocyte membrane protein carbonyl. The absorbance of the sample was measured at 370 nm and was expressed in µmol/mg membrane protein. The extinction coefficient used was 22,000 M−1 cm1.
Determination of membrane sialic acid
Erythrocyte membrane sialic acid content was determined by the method described by spyridaki and siskos [Citation24]. The sialic acid concentration was estimated by preparing a calibration curve with a standard solution of SA. The concentration of SA has expressed µg/mg protein.
Determination of lipid hydroperoxides
Erythrocyte membrane lipid hydroperoxides were determined according to the method of Firuzi et al [Citation25]. In two separate test tubes, 180 µl of the membrane was taken. In the first tube, 20 µl methanolic triphenyl phosphine (TPP) was added, while in the other methanol was added and both the tubes were kept in the dark for 30 minutes. Then, 1.8 ml of FOX solution was added to both the tubes and kept for incubation for 1 hr at room temperature in the dark. The tubes were vortex every 10 minutes. After the incubation period, tubes were centrifuged at 16,000 × g for 7 minutes and the supernatant was used to measure the absorbance was measured at 560 nm. A calibration curve of H2O2 (range 1–10 µM) was used in calculating the results. The value of LH was expressed as nmol/mg membrane protein.
Determination of membrane thiol (-SH) group
The membrane-bound total thiol group was determined by following the protocol of Kitajima et al [Citation26]. The method exploits the ability of the thiol group to reduce 5, 5’-dithiobis (2-nitrobenzoic acid) into a yellow colored compound. The optical density of this anionic compound was measured at 412 nm. The concentration of membrane total thiol was expressed as nmol/mg protein.
Statistical analysis
Values are expressed as mean ± standard deviation. The statistical analyses were performed using Graph-Pad Prism version 5.01 software. Difference between each groups were analyzed by one-way analysis of variance (ANOVA) followed by post hoc Tukey’s multiple comparison test, while ‘t’ student test was used to make comparison between two groups. P < 0.05 was considered significant.
Results
(a) and (b) show a significant (p < 0.05) decrease in both NKA and PMCA activity in D-gal induced and naturally aged rats as compared to young control. The treatment of GlcN significantly (p < 0.05) increased the activity of both transporters in D-gal induced and naturally aged rats with respect to their control groups. Moreover, the administration of GlcN also improved the NKA and PMCA activity significantly (p < 0.05) in young rats when compared to young control rats.
Figure 1. Effect of glucosamine on the activity of erythrocyte membrane transporters: (A) Na+/K+-ATPase activity (µmol Pi/mg protein/hour), (B) Ca2+ -ATPase activity (µmol Pi/mg protein/hour) in young, D-gal induced and naturally aged rats. The values are given as mean ± SEM (where n = 6). *p < 0.05 when the value is compared with young control, $p < 0.05 when the value is compared with D-Gal control, #p < 0.05 when the value is compared with naturally old control rats.
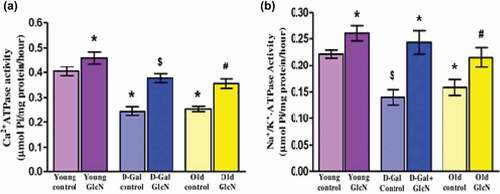
represents the activity of NHE. GlcN supplementation decreased the value of NHE significantly (p < 0.05) in D-gal induced aged rats when compared to D-gal controls. A similar result was found in the naturally aged rat group, where GlcN supplementation decreased the NHE activity significantly (p < 0.05) in comparison to old control rats. However, in GlcN treated young rats, there was a non-significant change in NHE activity. The erythrocyte intracellular Ca2+ ion concentration in all experimental groups has been depicted in . Our finding suggests a significant (p < 0.05) increase in intracellular Ca2+ ions in both D-gal induced and naturally aged groups. However, the GlcN supplementation results in a significant decline in intracellular Ca2+ levels in both D-gal induced and naturally aged rats as compared to their respective control groups. There was no significant change in intracellular Ca2+ levels in young GlcN treated rats.
Figure 2. (A) Effect of glucosamine on erythrocyte NHE activity (mmol/L RBC/hour at 37°C)], (B) intracellular calcium ion (nM) in young, D-gal induced and naturally aged rats. The values are given as mean ± SEM (where n = 6). Where * p < 0.05 when the value is compared with young control, $ p < 0.05 when the value is compared with D-Gal control, # p < 0.05 when the value is compared with naturally old control rats.
![Figure 2. (A) Effect of glucosamine on erythrocyte NHE activity (mmol/L RBC/hour at 37°C)], (B) intracellular calcium ion (nM) in young, D-gal induced and naturally aged rats. The values are given as mean ± SEM (where n = 6). Where * p < 0.05 when the value is compared with young control, $ p < 0.05 when the value is compared with D-Gal control, # p < 0.05 when the value is compared with naturally old control rats.](/cms/asset/bb1a8c3c-f769-4c0c-9479-4730ce3ad554/teba_a_2099116_f0002_oc.jpg)
(a) and (b) represent the protein carbonyl (PCO) and lipid hydro peroxides (LHP) respectively. A significant (p < 0.05) increase in both membrane PCO and LHP levels was observed in D-gal induced and naturally aged rats when compared with young control. The supplementation of GlcN results in a significant decrease (p < 0.05) in both PCO and LHP levels in D-gal induced aged rats. A similar result has been found in both GlcN treated young and old age rat groups with respect to their controls. Membrane Sialic acid and total thiol levels depicted in (c) and (d) respectively. An age-dependent significant (p < 0.05) decrease has been found in both Sialic acid and total thiol levels in both D-gal and naturally aged rats, respectively, as shown in (c) and (d). However, GlcN supplementation results in a remarkable increase in both sialic acid and total thiol levels significantly (p < 0.05) in D-gal induced as well as naturally aged rats with respect to their control groups. In the young GlcN treated group, the level of total thiol is improved non-significantly when compared with young control rats. Conversely, the membrane sialic acid is increased significantly (p < 0.05) upon GlcN treatment in young rats.
Figure 3. Restorative potential of glucosamine on biomarkers of oxidative stress in erythrocyte membrane: (A) Protein carbonyl levels (µmol/mg protein), (B) Lipid hydroperoxides (nmol/mg protein), (C) Membrane sialic acid (µg/mg membrane protein), and (D) Membrane sulfhydryl group (nmol/mg protein) in young, D-Gal induced and naturally aged rats. The values are given as mean ± SEM (where n = 6). *p < 0.05 when the value is compared with young control, $p < 0.05 when the value is compared with D-Gal control, #<0.05 when the value is compared with naturally old control rats.
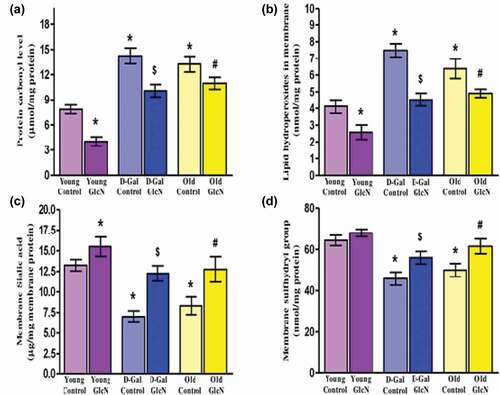
Discussion
The present study aims to investigate the potential therapeutic properties of glucosamine in an accelerated senescence model of rat (D-galactose induced aging) and natural aging rat models. D-gal was used to develop pathophysiological features similar to those found in the Cellular natural aging of rats. Recently, several studies have proposed Glucosamine as a promising caloric restriction mimetic [Citation14,Citation15]. GlcN has found therapeutic application in the treatment of age-related pathologies including osteoarthritis, cardiovascular diseases, neurological disorders, skin disorders, kidney diseases, and cancer [Citation27]. Studies on C.elegans and mice have shown that GlcN extends lifespan by mimicking caloric restriction [Citation28]. It has been hypothesized that in response to GlcN-mediated glucose deprivation, the AMPK pathway is activated which is a promoter of mitochondrial biogenesis. Increased mitochondrial respiration elevates the cellular level of reactive oxygen species (ROS) which, in turn, activates secondary stress defenses, cumulating in mitohormetically increased stress protection and extended lifespan [Citation28]. There has been a heightened interest in the role of GlcN in the activation of autophagy [Citation29]. Significantly oral administration of GlcN has been reported to help delay the aging process via autophagy induction [Citation30].
The present study has been undertaken to investigate the protective role of glucosamine in aged rats. With the progression of aging, increased oxidation of erythrocyte membrane-bound proteins, lipids, and carbohydrates was observed which has been confirmed in the present study by the significant increase in their biomarkers (PCO, LHs, and SA levels). Moreover, the activity of PMCA, NKA, and NHE of the RBC membrane were also studied as a marker of membrane function affected by age.
It has been suggested that biochemical and biophysical abnormalities of cell membranes may actively participate in the pathogenesis of age-related diseases [Citation27]. Membrane-bound calcium-transporting protein PMCA pump is important in regulating various signaling functions of calcium [Citation31]. Erythrocytes have deformability and elasticity properties which are affected by Ca2+ ions [Citation32]. Elevation in internal Ca2+ levels leads to changes in cell shape and volume, increased cellular rigidity, and hemolysis [Citation33]. Since this pump plays a crucial role in the maintenance of internal Ca2+ levels by creating a concentration gradient between cytosol and extracellular fluid, it can be predicted that aging results in alteration of PMCA pump activity [Citation34]. Altered activity of erythrocyte PMCA has been reported in aging [Citation35] which can be seen in our results too. However, upregulation of PMCA activity by glucosamine supplementation signifies its possible shielding effect against cellular redox imbalance in aging and associated diseases.
NKA is responsible for transmembrane Na+ and K+ concentration gradient and membrane potential [Citation21]. It also regulates excitatory neurotransmitters, RBC volume, and cytoplasmic viscosity via maintaining the ionic homeostasis across the cell membrane [Citation36]. It is widely believed that impairment in the activity of these transporters may play a major role at the cellular level in the pathophysiology of many age-associated diseases such as diabetes, vascular complications, cerebral ischemia, and Alzheimer’s disease [Citation37]. Alterations of the antioxidant status and increased lipid peroxidation which is a common biomarker of aging have been proposed to cause a reduction in NKA activity in erythrocyte membranes [Citation38]. Studies have shown that GlcN prevents the reduction of the cytosolic antioxidant glutathione in erythrocytes and can chelate ferrous ions and free radical scavenging [Citation39]. Thus, the treatment of glucosamine reversed the impaired NKA activity in both G-gal and naturally aged rats by restoring cellular antioxidant status.
NHE is an N-methyl-N-isobutyl amiloride-sensitive, electroneutral exchange system, which exchanges Na+ for H+ in accordance with concentration gradients, favoring the regulation of cellular pH, growth, and volume [Citation40]. It catalyzes acid extrusion by electro-neutral ion-exchange, using the energy support provided by Na+ /K+ -ATPase. Altered NHE activity has been linked to the onset of several diseases, including hypertension, diabetes mellitus, tissue damage, and cancer [Citation41]. The elevation in NHE activity during aging creates a condition of metabolic imbalance and oxidation of glucose, proteins, and free fatty acids [Citation8]. It is a potent physiological system that reduces cell acidity by eliminating H+ from the cells. An age-dependent increase in NHE activity was observed in rats and humans due to the deoxygenation of hemoglobin [Citation42]. Glucosamine exerts a beneficial effect during aging by decreasing NHE activity significantly.
The evidence presented above clearly indicates that the free Ca2+ content of erythrocytes increases with cell aging due to the increased activity of NHE. Increased Intracellular Ca2+ levels have long been associated with the pathogenesis of myocardial injury during ischemia and reperfusion [Citation43]. Our result shows increased intracellular Ca2+ with aging, which is reduced upon the treatment with GlcN.
Lipids and proteins are the main constituents of plasma membranes which define membrane dynamics, integrity, permeability, and fluidity [Citation44]. Age-related oxidative modifications in proteins result in the conversion of their amino acid residues into carbonyl groups. These carbonyls react with an amino group of other proteins and form protein aggregates, resulting in protein structural and functional alterations. Studies have suggested that GlcN has an anticarbonylation function and provides protection to proteins against age-associated carbonyl stress [Citation45]. The erythrocyte membrane contains the thiol (-SH) group, which is maintained in reduced form by reduced glutathione. It is a major determinant of total antioxidant potential and maintains overall cellular redox homeostasis during aging [Citation46]. Studies have shown an age-dependent decrease in glutathione in aged rats [Citation47]. GlcN has been shown to prevent the reduction of the cytosolic level of glutathione in erythrocytes [Citation39]. It has also been found to protect proteins from oxidation and increase glutathione levels in stressed human chondrocytes [Citation48]. In our earlier studies, we have shown an increased level of lipid hydroperoxides with aging [Citation8] which corroborates to present study results. GlcN has been found to scavenge lipid peroxidation product malondialdehyde. Studies have reported that glucosamine hydrochloride can reduce the erythrocyte hemolysis and lipid/protein peroxidation in a concentration and time-dependent manner [Citation44]. Our findings also suggest GlcN supplementation decreases lipid peroxidation in aged rats, thus, potentiating the anti-peroxidative nature of GlcN.
Sialic acid, the carbohydrate moiety of glycoproteins, contributes to the negative charge on the erythrocyte membrane [Citation49]. Therefore, the loss of sialic acid moiety from the membrane causes altered biophysical properties of the erythrocyte membrane by changing surface charge. Studies have shown that age-dependent decrease in glycoproteins especially SA from erythrocyte membranes at a higher rate in older individuals compared to younger individuals [Citation50]. The supplementation of GlcN protects against age-induced modification in surface charge and conserves membrane SA levels.
The present study explored only a few parameters of erythrocyte membrane basically the membrane transporters in an effort to validate the CRM effect of GlcN, however these results have been interpreted taking into account our previous report wherein we have reported the mitohormetic effect of GlcN [Citation15]. Despite these results, the present study has not explored various rheological parameters which are known to be altered during aging [Citation51] .
Conclusion
Based on the above results, we conclude that aging results in impairment in the activity of erythrocyte plasma membrane transporters and pumps which plays a very important role in the maintenance of cellular redox homeostasis. The present study validates the role of GlcN treatment in preserving the erythrocyte membrane integrity and modulating membrane transporters in D-gal and naturally aged rats. These findings, viewed in conjunction with the evolving evidence of the role of GlcN in the induction of autophagy during aging, provide a strong intervention strategy to reduce aging-related ailments.
Availability of data and material
The information that helps the finding of this study is accessible from the corresponding author upon reasonable request.
Author contribution
SIR conceived and designed research. KS conducted experiments, analyzed data, and wrote the manuscript, RK conducted experiments. All authors read and approved the manuscript and all data were generated in-house and that no paper mill was used.
Ethics approvals
All the protocols and procedures were followed as mandated by the Ethical Committee of the University of Allahabad, Allahabad, India. The work described has been also carried out in accordance with The Code of Ethics of the World Medical Association (Declaration of Helsinki) for experiments involving animal.
Acknowledgments
This work was supported by a research grant to Syed Ibrahim Rizvi from the Science and Engineering Research Board (SERB), Department of Science and Technology, Govt of India (EMR/2016/006470), the Department of Biochemistry is supported by a Fund for Improvement of S&T Infrastructure (FIST) grant of DST-SERB, and university grants commission- Special Assistance Programme (UGC-SAP) Grant. Financial funding from Department of Biotechnology, Government of India under the RRFSP is gratefully acknowledged.
Disclosure statement
No potential conflict of interest was reported by the author(s).
Additional information
Funding
References
- Hartl FU. Cellular homeostasis and aging. Annu Rev Biochem. 2016;85(1):1–4.
- Pomatto LCD, Davies KJA. The role of declining adaptive homeostasis in ageing. J Physiol. 2017;595(24):7275–7309.
- Pandey KB, Rizvi SI. Resveratrol up-Regulates the erythrocyte plasma membrane redox system and mitigates oxidation-Induced alterations in erythrocytes during aging in humans. Rejuvenation Res. 2013;16(3):232–240.
- Pandey KB, Rizvi SI. Markers of oxidative stress in erythrocytes and plasma during aging in humans. Oxid Med Cell Longev. 2010;3(1):2–12.
- Andrews NW, Corrotte M. Plasma membrane repair. Curr Biol. 2018;28(8):R392–R397.
- Bartosz G. Erythrocyte aging: physical and chemical membrane changes. Gerontology. 1991;37(1–3):33–67.
- Kaestner L, Minetti G. The potential of erythrocytes as cellular aging models. Cell Death Differ. 2017;24(9):1475–1477.
- Singh AK, Singh S, Garg G, et al. Rapamycin mitigates erythrocyte membrane transport functions and oxidative stress during aging in rats. Arch Physiol Biochem. 2018a;124(1):45–53.
- Pandey KB, Rizvi SI. Role of resveratrol in regulation of membrane transporters and integrity of human erythrocytes. Biochem Biophys Res Commun. 2014a;453(3):521–526.
- Bissett DL. Glucosamine: an ingredient with skin and other benefits. J Cosmet Dermatol. 2006;5(4):309–315.
- Ingram DK, Roth GS. Glycolytic inhibition: an effective strategy for developing calorie restriction mimetics. GeroScience. 2020; Preprint. doi:10.1007/s11357-020-00298-7
- Ingram DK, Roth GS. Glycolytic inhibition: an effective strategy for developing calorie restriction mimetics. GeroScience. 2021;43(3):1159–1169.
- Anderson JW, Nicolosi RJ, Borzelleca JF. Glucosamine effects in humans: a review of effects on glucose metabolism, side effects, safety considerations and efficacy. Food Chem Toxicol. 2005;43(2):187–201.
- Ingram DK, Roth GS. Calorie restriction mimetics: can you have your cake and eat it, too? Ageing Res Rev. 2015;20:46–62.
- Kumar R, Saraswat K, Rizvi SI. Glucosamine displays a potent caloric restriction mimetic effect in senescent rats by activating mitohormosis. Rejuvenation Res. 2021;24(3):220–226.
- Azman KF, Zakaria R. d-Galactose-induced accelerated aging model: an overview. Biogerontology. 2019;20(6):763–782.
- Song X, Bao M, Li D, et al. Advanced glycation in d-galactose induced mouse aging model. Mech Ageing Dev. 1999;108(3):239–251.
- Kumar R, Kumar M, Rizvi SI. Chitosan displays a potent caloric restriction mimetic effect in senescent rats. Rejuvenation Res. 2021;24(5):390–396.
- Tripathi SS, Kumar R, Bissoyi A, et al. Baicalein may act as a caloric restriction mimetic candidate to improve the antioxidant profile in a natural rodent model of aging. Rejuvenation Res. 2022;25(2):70–78.
- Marchesi VT, Palade GE. THE LOCALIZATION OF Mg-Na-K-ACTIVATED ADENOSINE TRIPHOSPHATASE ON RED CELL GHOST MEMBRANES. J Cell Biol. 1967;35(2):385–404.
- Suhail M. Na+, K+-ATPase: ubiquitous multifunctional transmembrane protein and its relevance to various pathophysiological conditions. J Clin Med Res. 2010; Preprint. doi:10.4021/jocmr2010.02.263w
- Matteucci E. ERYTHROCYTE SODIUM/HYDROGEN EXCHANGE INHIBITION BY (−) EPICATECHIN. Cell Biol Int. 2001a;25(8):771–776.
- Levine RL, et al. Determination of carbonyl content in oxidatively modified proteins. In Methods in Enzymology 186 Elsevier; 1990. p 464–478. DOI: 10.1016/0076-6879(90)86141-H
- Spyridaki M-HE, Siskos PA. An improved spectrophotometric method for the determination of free, bound and total N-acetylneuraminic acid in biological fluids. Anal Chim Acta. 1996;327(3):277–285.
- Firuzi O, Mladěnka P, Riccieri V, et al. Parameters of oxidative stress status in healthy subjects: their correlations and stability after sample collection. J Clin Lab Anal. 2006;20(4):139–148.
- Kitajima H, Yamaguchi T, Kimoto E. Hemolysis of human erythrocytes under hydrostatic pressure is suppressed by cross-linking of membrane proteins. J Biochem. 1990;108(6):1057–1062.
- Rao V, Kaja S, and Gentile S. Ion channels in aging and aging-related diseases. In Shiomi N, editor. Molecular mechanisms of the aging process and rejuvenation. Kobe college, Japan: InTech; 2016. doi:10.5772/63951
- Weimer S, Priebs J, Kuhlow D, et al. D-Glucosamine supplementation extends life span of nematodes and of ageing mice. Nat Commun. 2014;5(1):3563.
- Lv C, Wang L, Zhu X, et al. Glucosamine promotes osteoblast proliferation by modulating autophagy via the mammalian target of rapamycin pathway. Biomed Pharmacothe. 2018;99:271–277.
- Shintani T, Kosuge Y, Ashida H. Glucosamine extends the lifespan of caenorhabditis elegans via autophagy induction. J. Appl. Glycosci. 2018;65(3):37–43.
- Bogdanova A, Makhro A, Wang J, et al. Calcium in red blood cells—A perilous balance. Int J Mol Sci. 2013;14(5):9848–9872.
- Kim J, Lee H, Shin S. Advances in the measurement of red blood cell deformability: a brief review. J Cell Biotechnol. 2015;1(1):63–79.
- Viskupicova J, Blaskovic D, Galiniak S, et al. Effect of high glucose concentrations on human erythrocytes in vitro. Redox Biol. 2015;5:381–387.
- Stafford N, Wilson C, Oceandy D, et al. ‘The plasma membrane calcium ATP ases and their role as major new players in human disease’. Physiol Rev. 2017;97(3):1089–1125.
- Zaidi A. Plasma membrane Ca 2+ -ATPases: targets of oxidative stress in brain aging and neurodegeneration. World J Biol Chem. 2010;1(9):271.
- Clausen MV, Hilbers F, Poulsen H. The structure and function of the Na,K-ATPase isoforms in health and disease. Front Physiol. 2017;8:371.
- Rossi L, Fraternale A, Bianchi M, et al. Red blood cell membrane processing for biomedical applications. Front Physiol. 2019;10:1070.
- Namazi G, Asa P, Sarrafzadegan N, et al. Decreased Na+/K+-ATPase activity and altered susceptibility to peroxidation and lipid composition in the erythrocytes of metabolic syndrome patients with coronary artery disease. Ann Nutr Metab. 2019;74(2):140–148.
- Jamialahmadi K, Arasteh O, Matbou Riahi M, et al. Protective effects of glucosamine hydrochloride against free radical-induced erythrocytes damage. Environ Toxicol Pharmacol. 2014;38(1):212–219.
- Bobulescu IA, Di Sole F, Moe OW. Na+/H+ exchangers: physiology and link to hypertension and organ ischemia. Curr Opin Nephrol Hypertens. 2005;14(5):485–494.
- Aoi W, Marunaka Y. Importance of pH homeostasis in metabolic health and diseases: crucial role of membrane proton transport. Biomed Res Int. 2014;2014:1–8.
- Sun Y-M, Su Y, Li J, et al. Role of the Na+/H+ exchanger on the development of diabetes mellitus and its chronic complications. Biochem Biophys Res Commun. 2012;427(2):229–231.
- Kalogeris T, et al. Cell biology of ischemia/reperfusion injury. In: International review of cell and molecular biology. Elsevier; 2012. p 229–317. DOI: 10.1016/B978-0-12-394309-5.00006-7
- Dalirfardouei R, Karimi G, Jamialahmadi K. Molecular mechanisms and biomedical applications of glucosamine as a potential multifunctional therapeutic agent. Life Sci. 2016;152:21–29.
- Fang C, Peng M, Li G, et al. New functions of glucosamine as a scavenger of the lipid peroxidation product malondialdehyde. Chem Res Toxicol. 2007;20(6):947–953.
- Balcerczyk A, Bartosz G. Thiols are main determinants of total antioxidant capacity of cellular Homogenates. Free Radic Res. 2003;37(5):537–541.
- Zhu Y, Carvey PM, Ling Z. Age-related changes in glutathione and glutathione-related enzymes in rat brain. Brain Res. 2006;1090(1):35–44.
- Kapoor M, Mineau F, Fahmi H, et al. ‘Glucosamine sulfate reduces prostaglandin E2Production in osteoarthritic chondrocytes through inhibition of microsomal PGE synthase-1’. J Rheumatol. 2012;39(3):635–644.
- Mazzanti L, Rabini RA, Salvolini E, et al. Sialic acid, diabetes, and aging: a study on the erythrocyte membrane. Metabolism. 1997;46(1):59–61.
- Kumar D, Rizvi SI. Erythrocyte membrane bound and plasma sialic acid during aging. Biologia. 2013;68(4):762–765.
- Gordon MB, Kloxin CJ, Wagner NJ. Structural and rheological aging in model attraction-driven glasses by Rheo-SANS. Soft Matter. 2021;17(4):924–935.