ABSTRACT
Acacia auriculiformis A. Cunn. Ex. Benth (family: Fabaceae) is traditionally used as a folk remedy for diabetes in Central Africa. The present study was intended to evaluate the antioxidant and enzyme inhibitory potential of hydroethanolic leaf extract (HEAA) and its fractions (n-hexane, chloroform and ethyl acetate) at different concentrations (100–500 μg/ml) to justify its role in diabetes. The leaf extract (HEAA) and its ethyl acetate fraction (EFAA) showed maximum scavenging effect (60.43 ± 0.11, 50.87 ± 0.31) at 500 µg/ml, respectively. However, in enzyme inhibitory assays, the HEAA extract and EFAA among the fractions exhibited maximum inhibition against α‑amylase (61.69 ± 0.05, 53.87 ± 0.33), α‑glucosidase (63.34 ± 0.12, 58.46 ± 0.90) and pancreatic lipase (51.77 ± 0.15, 49.87 ± 0.31), respectively, at 500 µg/ml when compared with standard. The IC50 values of HEAA and EFAA against α‑amylase (3.35 and 4.48, respectively), α‑glucosidase (3.36 and 4.04, respectively) and pancreatic lipase (4.47 and 5.07, respectively) are also significant. The results suggest that HEAA possesses good antioxidant and enzyme inhibitory potential, which in turn might be responsible for its antidiabetic effect.
Graphical Abstract
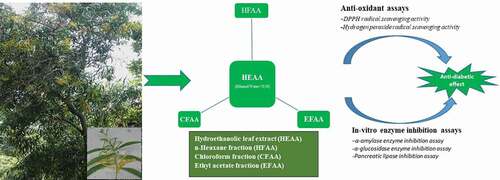
Introduction
Being a metabolic disorder, DM is characterized by hyperglycemia linked with some macro- and microvascular complications [Citation1]. It is reported to influence around 463 million adults globally in 2019 and is anticipated to increase to 700 million by 2045 [Citation2]. According to the WHO, diabetes has been spreading promptly in low- to middle-income countries and is majorly responsible for blindness, myocardial infarction and kidney failure. In April 2021, a global project launched by the WHO aimed at long-term advancement in the prevention and cure of diabetes mellitus, especially in low- and middle-income countries [Citation3]. DM is categorized into two classes: Type 1 and Type 2. In T1DM, there is insufficient production of insulin due to the devastation of pancreatic β-cells, while T2DM is manifested by malfunctioning insulin secretion in the pancreatic β-cells or by insulin resistance leading to hyperglycemia [Citation4]. The formation of free radicals or reactive oxygen species ordinarily takes place inside the cells when supplied with high blood glucose, which, in turn, can damage cellular macromolecules such as lipids, nucleic acids and proteins, ultimately advancing toward diabetes and the furtherance of its associated problems [Citation5]. Antioxidants prevent damage to β‑cells by preventing the peroxidation chain reaction and, thus, provide defense against the progression of diabetes [Citation6]. Plants have been widely acknowledged as natural antioxidative agents [Citation7,Citation8]. Thus, the free radical scavenging natural product therapy can prove a beneficial therapeutic tactic in diabetes control.
There are several therapeutic approaches in the management of DM for reducing the postprandial hyperglycemia by inhibiting carbohydrate-hydrolyzing enzymes, such as α-amylase and α-glucosidase [Citation9,Citation10]. α-Amylase, a digestive enzyme generally found in saliva and functional only in the presence of calcium, is also known as a carbohydrate digesting enzyme as it causes the conversion of polysaccharides into monosaccharides and increases glucose absorption in the blood vessels [Citation11]. Another enzyme, namely α-glucosidase, usually found in the brush borders of the small intestine, escalates blood glucose levels by breaking down starch into disaccharides. Inhibition of these carbohydrate hydrolyzing enzymes leads to a decrease in postprandial hyperglycemia by inhibiting glucose absorption. Obesity is also one of the main causes of diabetes mellitus as higher fatty acids and lipids lead to insulin resistance. Pancreatic lipase is the main lipid enzyme responsible for hydrolyzing dietary fat molecules, converting triglycerides into monoglycerides and free fatty acids [Citation12]. The inhibition of the lipase enzyme delays the lipolytic process and, thus, decreases the lipid absorption and ultimately leads to restoring insulin production from β-cells and protecting the pancreas [Citation13]. Acarbose and voglibose are commercially available enzyme inhibitors, but they have some serious side effects like gastrointestinal bloating, abdominal discomfort, diarrhea and flatulence [Citation14,Citation15]. On the other hand, plant-based remedies are economic, safe, and free from toxic or side effects. Thus, traditional medicines, especially medicinal plants, could have been a vital source of future drugs for controlling DM [Citation16]. Numerous plants have been reported in the literature for having enzyme inhibition mechanisms such as α-amylase, α-glucosidase, DPP-IV and pancreatic lipase inhibition [Citation17–19] and thus exhibit antidiabetic activity. Therefore, regulation of the action of the enzyme by plant-based constituents may be one of the most effective tools to control T2DM.
Acacia auriculiformis A. Cunn. Ex. Benth (family: Fabaceae) is commonly known as Australian babool or earpod wattle [Citation20]. It is enriched with carbohydrates like glucuronic acid, methyl glucuronic acid, galactose, L-rhamnose and arabinose, and seeds contain saponins, mainly, proacaciaside-I and proacaciaside-II [Citation21] and Acaciaside-A and Acaciaside-B and flavonoids, namely, (-)-teracacidin and (-)-isoteracacidin [Citation22]. This plant has been reported to possess several biological activities, namely, antimutagenic and chemopreventive [Citation23], antidiabetic [Citation24,Citation25], antimalarial [Citation26] and antiulcer [Citation27] activities. Traditionally, the leaves of plants have been used in the management of diabetes by local healers in the Gabon district of Central Africa [Citation28]. Till now, there is no in vitro antidiabetic activity performed on the leaf extract of the plant. Thus, the present study aimed to assess the in vitro enzyme inhibitory along with antioxidant assay of A. auriculiformis hydroethanolic leaf extract and its fractions to elucidate their role in diabetes.
Materials and methods
Chemicals and reagents
The chemicals and reagents used in this study, namely α-amylase, dimethylsulphoxide, 2,4-dinitrophenyl salicylic acid, disodium hydrogen phosphate, di-potassium hydrogen phosphate, p-nitrophenyl-α-D-glucopyranosyl, potassium dihydrogen phosphate, sodium dihydrogen phosphate, sodium potassium tartrate, starch and 96-well microplates were procured from Himedia, whereas, acarbose, α-glucosidase, orlistat, p-nitrophenyl butyrate and pancreatic lipase were purchased from Sigma Aldrich. All other chemicals used in the study were of standard quality.
Identification of plant material
The fresh leaves of Acacia auriculiformis were collected from the campus Botanical Garden of Kurukshetra University, Kurukshetra (29°57′28″N 76°48′57″E) in August 2018 and authenticated from NISCAIR, Delhi where a voucher specimen (No.: NISCAIR/RHMD/Consult/-2018/3259-60) is available for further reference.
Preparation of extract and fractions
After shade drying, the coarsely powdered leaves were extracted with hydroethanolic (water: ethanol: 30:70) in the soxhlet apparatus (approximately 72 h). The hydroethanolic leaf extract of Acacia auriculiformis (HEAA) solution was filtered and concentrated using Heidolph rotary evaporator (Model-4011, USA) to yield 82.45 g (8.2% w/w). About 50 g of HEAA extract was then fractionated with various solvents in the sequence of increasing polarity, e.g. n-hexane, chloroform and ethyl acetate, to ensure fractionation of active constituents, respectively. Each fraction was separately concentrated to dryness using a rotary evaporator to yield n-hexane (HFAA, 3.84% w/w), chloroform (CFAA, 22.12% w/w) and ethyl acetate (EFAA, 58.12% w/w) fractions, respectively. All the fractions were tightly packed and stored in the refrigerator at 4°C.
Preliminary phytochemical screening
An aliquot of the prepared leaf extract and its fractions were treated with different chemical reagents (namely Dragendorff/Mayer reagent test for alkaloids, Molisch test for carbohydrates, Borntrager/Keller Kilani/Legal’s test for glycosides, Shinoda test for flavonoids, foam test for saponins, Salkowski/Liebermann Burchard test for steroids and ferric chloride test for tannins) to ascertain the presence of main phytoconstituent classes according to the reported methods [Citation29].
In vitro antioxidant assay
DPPH radical scavenging activity
Being a stable free radical, DPPH forms 1,1-diphenyl-2-picryl hydrazine due to which it turns yellow. In the present study, the procedure of the DPPH radical scavenging assay was followed as per the reported method [Citation30]. The percentage scavenging of the hydroethanolic leaf extract, its fractions, and standard (ascorbic acid) at concentrations of 100, 200, 300, 400 and 500 µg/ml was measured at 517 nm on UV–visible spectrophotometer. The results obtained were expressed as the percentage inhibition and calculated by using the below formula:
Percent inhibition of DPPH = [(Acontrol – Asample)/Acontrol] × 100,
Acontrol – absorbance of the DPPH solution without the sample.
Asample – absorbance of the DPPH solution with the sample.
Hydrogen peroxide radical scavenging activity
Hydrogen peroxide produced by several oxidase enzymes in the body scavenges through its reduction product hydroxyl radical (OH). In this procedure, when the sample was incubated with hydrogen peroxide, the decay or loss of hydrogen peroxide was estimated. All the samples (hydroethanolic leaf extract, fractions and ascorbic acid) at different concentrations (100–500 µg/ml) were measured spectrophotometrically at 230 nm as per the reported method [Citation31]. The percentage inhibition of hydrogen peroxide free radical was estimated by the following formula:
Percent inhibition (%) = [(A control – A sample)/(A control)] × 100,
where Acontrol and Asample denote the absorbance of control and sample, respectively.
In vitro enzyme inhibition assays
α-Amylase enzyme inhibition assay
The inhibitory effects of extract (HEAA) and different fractions (HFAA, CFAA and EFAA) were investigated at varying concentrations (100–500 μg/ml) and compared with standard, i.e. acarbose following the reported method [Citation32]. All the preparation was made in 0.02 M sodium phosphate buffer solution containing 0.006 mol/L of sodium chloride (pH 6.9). Various dilutions of tests were mixed with 100 µl of α-amylase and placed in an incubator at 25°C for 15 min. At that point, 1% starch of pH 6.8 was added (200 µl to each tube and kept in an incubator for 30 min at 37°C). Then, the reaction was stopped by adding 100 µl of 3,5-dinitrosalicylic color reagent and boiled for 20 min. 200 μl of each sample, standard and control was then moved to 96-well microplates and noticed the absorbance at 595 nm on iMark Microplate Reader (BioRad). The absorbance was estimated in triplicate, and the inhibitory action was determined as given below:
Percent inhibition (%) = Ac-[As – A0]/Ac × 100.
where Ac, As and A0 signify the absorbance of the control, test with enzyme and test without enzyme, respectively.
α-Glucosidase inhibition assay
The impact of the plant extracts and its fractions on α ‑ glucosidase action was performed as per previously reported method [Citation33]. Phosphate buffer saline (100 mM, pH 6.9) was utilized to set up all arrangements. 95 μl of buffer saline and 25 μl of the enzyme (0.5 U/ml) were added to 96-well microplates. 30 μl of test or standard (acarbose) at different concentrations (100–500 μg/ml) was added and incubated at 37°C for 20 min. Thereafter, 50 μl of 5 mM substrate (p-nitrophenyl-α-D glucopyranoside) was added and again incubated at 37°C for 45 min. Absorbance was estimated at 415 nm on iMark Microplate Reader (BioRad) and taken in a triplicate manner. The results were expressed as mean ± standard error of the mean (SEM). The percentage inhibition was determined as given below:
Percent inhibition (%) = Ac – [As – A0]/Ac × 100,
where Ac, As and A0 signify the absorbance of the control, test with enzyme and test without enzyme, respectively.
Pancreatic lipase inhibition
Pancreatic lipase prevention activity was performed as per the reported method [Citation34]. 0.1 M potassium phosphate buffer with 0.1% tween 80 (pH 7.2) was prepared and loaded into microplate wells followed by the addition of 25 μl of porcine pancreatic lipase (1 mg/ml). Thereafter, 30 μl of samples or orlistat in different concentrations were added and preincubated at 37°C for 30 min. After the pre-incubation, p-nitrophenyl butyrate (10 mM, 50 μl) was incorporated, further incubated at 37°C for 40 min and assessed spectrophotometrically at 415 nm using iMark Microplate Reader (BioRad). The observations were noted in triplicate and expressed as mean ± standard error of the mean (SEM). The inhibition was estimated as given below:
Percent inhibition (%) = Ac – [As – A0]/Ac × 100,
where Ac, As and A0 demonstrate the absorbance of the control, test with enzyme and test without enzyme, respectively.
Statistical analysis
All observations were done in triplicate, and the results were expressed as mean ± standard error of the mean (S.E.M.). The IC50 values were also calculated by using linear regression analysis equation, i.e. y = mx + c, where y is the percentage activity (equals to 50), m is the slope, x is the concentration and c is the intercept.
Results
Phytochemical screening
Phytochemical screening confirmed the presence of flavonoids, saponins and steroids as main constituents in HFAA and EFAA, whereas flavonoids were absent in CFAA.
Antioxidant activity
DPPH and H2O2 radical scavenging activity
Determining the free radical scavenging activity of sample depends on the general searching impacts of DPPH and H2O2 radicals and is one of the routinely followed antioxidant assays as these techniques are considered rapid, simple and most convenient. Results of the DPPH assay indicate a concentration-dependent rise in the free radical scavenging effect of the extract (HEAA) and all its fractions when compared with ascorbic acid taken as standard (). HEAA shows maximum scavenging activity, i.e. 60.43 ± 0.11 at 500 µg/ml concentration with IC50 value of 4.06 µg/ml, whereas the fractions possess scavenging activities in the order, i.e. EFAA (50.87 ± 0.31) > CFAA (21.68 ± 0.27) > HFAA (14.74 ± 0.24), respectively, at the same concentration. The HEAA represents an IC50 value of 4.91 µg/ml. Similarly, in the H2O2 free radical scavenging assay, HEAA exhibited maximum (64.89 ± 0.12) hydrogen peroxide radical scavenging activity with an IC50 value of 3.63 µg/ml as compared to other fractions. The fractions showed lesser scavenging activity than extract in the order: EFAA (59.87 ± 0.31) > CFAA (24.58 ± 0.27) > HFAA (19.74 ± 0.24). Here also, EFAA represents a significant IC50 value of 4.26 µg/ml. Results of the in-vitro antioxidant assay show that HEAA and EFAA represent higher radical scavenging activity as compared to other fractions and may possess good antioxidant properties.
Table 1. Free radical scavenging activities of A. auriculiformis leaf extract/fractions.
Antidiabetic activity
Inhibition of α-amylase, α-glucosidase and pancreatic lipase
The results of the inhibitory effect of the extract and its fractions on α‑amylase and α‑glucosidase enzymes were compared with acarbose (; ) and revealed a dose‑dependent inhibitory effect on both enzymes. The IC50 values of all the fractions, as well as the extract of AA, are given in . The hydroethanolic extract (HEAA) exhibited maximum inhibition against α‑amylase, i.e. 61.69 ± 0.05, whereas among fractions, ethyl acetate fraction (EFAA) showed maximum α‑amylase inhibition (53.87 ± 0.33) at 500 µg/ml compared with standard. In the α-glucosidase inhibition method, HEAA showed a significant inhibitory effect (63.34 ± 0.12) in a dose-dependent manner (; ). Among the fractions, EFAA (58.46 ± 0.90) produced more inhibition than CFAA (40.87 ± 0.42) at all concentrations followed by HFAA (36.24 ± 0.18).
Figure 1. Percentage inhibition of hydroethanolic extract of A. auriculiformis (HEAA) and its fractions against α-amylase.
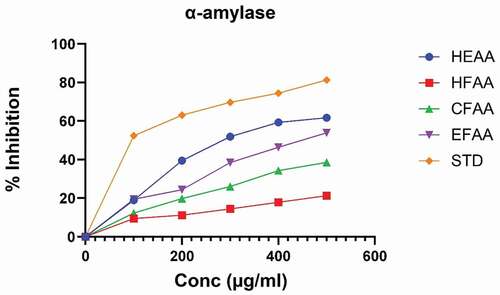
Figure 2. Percentage inhibition of hydroethanolic extract of A. auriculiformis (HEAA) and its fractions against α-glucosidase.
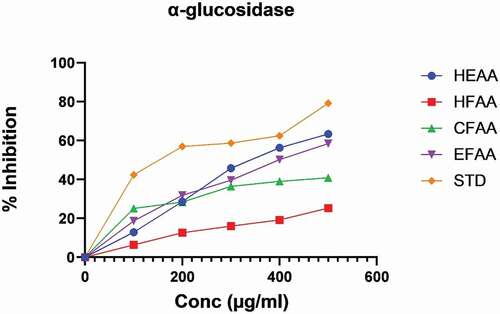
Table 2. Enzyme inhibition by A. auriculiformis leaf extract and its fractions.
Table 3. IC50 value of extract and fractions of A. auriculiformis.
In the pancreatic lipase method, the inhibitory effect was observed to be increased with an increase in concentration () with maximum inhibition (51.77 ± 0.15) exhibited by HEAA followed by EFAA (49.87 ± 0.31), respectively, at 500 µg/ml and compared with orlistat taken as standard.
Discussion
Diabetes is a major metabolic disorder in the developing countries of the world. The management of this disease and its complications are displaying a great challenge to the health-care system worldwide. Oxidative stress has been found among one of the factors causing diabetes as it is responsible for increasing the apoptotic processes and activating TLRs. The reduction in both the steps including β-cell neogenesis and metabolic pathways in β-cells causes dysfunction of β-cells, which eventually leads to insulin resistance [Citation35–37]. Free radicals in high concentration also interfere with multiple metabolic pathways like NF-κB, JNK/SAPK, p38 MAPK and hexosamine. These signaling pathways have a very important role in the dysfunction of β-cell [Citation38]. Therefore, the agents with antioxidative potential could be considered an effective tool in the management of diabetes [Citation39]. Plants that are rich sources of antioxidants like flavonoids, tannins, tocopherol and ascorbic acid are used to control diabetes and its complications [Citation40]. Furthermore, an effective approach to regulate diabetes is to inhibit carbohydrate digestive enzymes, which in turn significantly reduces the postprandial hyperglycemia and also minimizes the complications related to diabetes [Citation41]. Several previous investigations have reported the ameliorating effect of medicinal plants such as α‑glucosidase, α‑amylase and pancreatic lipase enzyme inhibitors that can lower the blood glucose levels by decreasing the intestinal assimilation of starches and fats, individually [Citation34,Citation42]. Moreover, it has been reported in several studies that antioxidants derived from plants act as potential α‑amylase and α‑glucosidase inhibitors, thus indicating their great capabilities in the management of diabetes [Citation43,Citation44]. The α-amylase inhibition prevents the metabolism of carbohydrates, thus lowering the blood sugar level [Citation11]. Similarly, α-glucosidase minimizes the glucose absorption rate by delaying carbohydrate metabolism [Citation12]. Increased fat/lipid deposition in obesity causes β-cell apoptosis due to increased level of free fatty acid (FFA) and c-Jun N-terminal kinase (JNK). This leads to insulin resistance in diabetic patients [Citation45]. Pancreatic lipase inhibitors reduce the levels of free fatty acid by inhibiting the lipase enzyme [Citation12]. Hence, plants with inhibitory effects on these enzymes are an attractive pharmacotherapy in the treatment of diabetes. In the present study, free radical scavenging methods (namely, DPPH and H2O2 radical scavenging) and in-vitro enzyme inhibitory assays (α-amylase, α-glucosidase and pancreatic lipase) were performed to investigate the hydroethanolic leaf extract of A. auriculiformis and its fractions for their antioxidant potential and enzyme inhibitory effect to justify the role of this plant in diabetes.
In previous studies, A. auriculiformis leaf extract is found to contain flavonoids, tannins, terpenoids and polyphenols as the main phytoconstituent classes along with free radical scavenging potential and aligns with our results [Citation24,Citation44]. Furthermore, it is reported that the phenolics and flavonoids present in other plants possess free radical scavenging effects by several mechanisms [Citation6,Citation24]. Preliminary phytochemical screening indicates the presence of phenolic groups in HEAA and EFAA. HEAA showed maximum percentage scavenging activity in comparison to its fractions. Similarly, the ethyl acetate fraction (EFAA) shows a maximum free radical scavenging effect in comparison to other fractions. The IC50 value of HEAA and EFAA is also significant as compared to the other fractions. Since the extract and fractions contain phenolics and flavonoids along with free radical scavenging effects, it can be speculated that the antioxidant potential of this plant may be considered an important property associated with diabetes [Citation46].
α‑Glucosidase, α‑amylase and pancreatic lipase inhibition assays revealed a dose‑dependent inhibition by hydroethanolic leaf extract (HEAA) as well as the fractions of A. auriculiformis, with maximum inhibition observed at higher concentrations, while EFAA showed higher inhibition among all fractions against α-amylase, α-glucosidase and pancreatic lipase enzyme. Phenolics have shown α-amylase, α-glucosidase and pancreatic lipase enzyme inhibition in previous studies [Citation10,Citation12,Citation24]. Preliminary phytochemical screening confirmed the presence of phenolic constituents like flavonoids in the ethyl acetate fraction of the extract, which could be partially responsible for increased enzyme inhibition. The maximum inhibition of α-amylase inhibitory activity is shown by the extract (HEAA) and EFAA among the fractions. The HEAA and EFAA show 61.69 ± 0.05 and 53.87 ± 0.33 percentage inhibition at 500 µg/ml, respectively, with IC50 value of 3.35 and 4.48 µg/ml. Similarly, the pancreatic lipase inhibition model shows that HEAA possesses maximum lipolytic activity in comparison to other fractions. The potency of postprandial antihyperglycemia of the extract might be derived from the inhibition of α-amylase and α-glucosidase enzymes, which in turn could contribute to the antihyperglycemic effect. Other species of this plant have also contributed to treating postprandial hyperglycemia by inhibiting lipase and glucosidase enzymes. In one study, polyphenols obtained from the bark of Acacia mearnsii have been reported to possess antidiabetic activity [Citation47]. Hence, the lipase inhibitory effect leading to antidiabetic potentials shown by extract or fractions of A. auriculiformis might be because of the presence of phenolics, tannins, saponins and flavonoids in the extract or fraction of the plant.
Conclusion
In conclusion, hydroethanolic leaf extract of A. auriculiformis and its ethyl acetate fraction show maximum antioxidant as well as enzyme inhibitory activities, which could be responsible for their antidiabetic effect. The consequences of the present study have given a hint that the plant leaf contains such chemical constituent(s) that could serve as antioxidative agents as well as inhibitors of α‑glucosidase, α‑amylase and pancreatic lipase enzymes, indicating its therapeutic potential in the management of T2DM and its complications.
Disclosure statement
No potential conflict of interest was reported by the authors.
Correction Statement
This article has been corrected with minor changes. These changes do not impact the academic content of the article.
References
- Baynes HWJJDM. Classification, pathophysiology, diagnosis and management of diabetes mellitus. J Diabetes Metab. 2015;6(5):1–9.
- Atanasov AG, Zotchev SB, Dirsch VM, et al. Natural products in drug discovery: advances and opportunities. Nat Rev Drug Discov. 2021;20(3):200–216.
- Yan K-H, Lee L-M, Yan S-H, et al. Tomatidine inhibits invasion of human lung adenocarcinoma cell A549 by reducing matrix metalloproteinases expression. Chem Biol Interact. 2013;203(3):580–587.
- Bharti SK, Krishnan S, Kumar A, Kumar A. Antidiabetic phytoconstituents and their mode of action on metabolic pathways. Ther Adv Endocrinol Metab. 2018;9(3):81–100.
- Johansen JS, Harris AK, Richly DJ, Ergul A. Oxidative stress and the use of antioxidants in diabetes: linking basic science to clinical practice. Cardiovasc Diabetology. 2005;4(1):1–11.
- Chaudhuri A, Ray -SJJOK-SU-S. In vitro free radical scavenging activities of aerial parts’ aqueous extract and extract fractions of Ampelocissus latifolia (Roxb.) Planch. in relation to total phenolics and flavonoid contents. J King Saud Univ Sci. 2020;32(1):732–739.
- Pietta P, Simonetti P, Mauri P. Antioxidant activity of selected medicinal plants. J Agric Food Chem. 1998;46(11):4487–4490.
- Krishnaiah D, Sarbatly R, Nithyanandam R. A review of the antioxidant potential of medicinal plant species. Food Bioprod Process. 2011;89(3):217–233.
- Shori ABJJOIM. Screening of antidiabetic and antioxidant activities of medicinal plants. J Integr Med. 2015;13(5):297–305.
- Chokki M, Cudălbeanu M, Zongo C. Exploring antioxidant and enzymes (A-amylase and B-glucosidase) inhibitory activity of Morinda lucida and Momordica charantia leaves from Benin. Foods. 2020;9(4):434.
- Agarwal P, Gupta RJRRJMHS. Alpha-amylase inhibition can treat diabetes mellitus. Res Rev J Med Health Sci. 2016;5(4):1–8.
- Inthongkaew P, Chatsumpun N, Supasuteekul C. α-Glucosidase and pancreatic lipase inhibitory activities and glucose uptake stimulatory effect of phenolic compounds from Dendrobium formosum. Revista brasileira de farmacognosia. 2017;27:480–487.
- Mulder H, Yang S, Winzell MS, et al. Inhibition of lipase activity and lipolysis in rat islets reduces insulin secretion. Diabetes. 2004;53(1):122–128.
- Kalita D, Holm DG, LaBarbera DV, et al. Inhibition of α-glucosidase, α-amylase, and aldose reductase by potato polyphenolic compounds. PloS one. 2018;13(1):e0191025.
- Cheng AYY, Fantus IGJC. Oral antihyperglycemic therapy for type 2 diabetes mellitus. CMAJ: Canadian Medical Association Journal = Journal de l’Association Medicale Canadienne. 2005;172(2):213–226.
- Kumar S, Saini M, Kumar V. Traditional medicinal plants curing diabetes: a promise for today and tomorrow. Asian J Tradit Med. 2012;7(4):178–188.
- Alagesan K, Raghupathi PK, Sankarnarayanan SJIJPLS. Amylase inhibitors: potential source of anti-diabetic drug discovery from medicinal plants. Int J Pharm Life Sci. 2012;3(2):1407–1412.
- Seyedan A, Alshawsh MA, Alshagga MA. Medicinal plants and their inhibitory activities against pancreatic lipase: a review. Evidence Complementary Altern Med. 2015;2015.
- Sharma D, Kumar S, Kumar S, Kumar, D, et al., DPP-IV Inhibitors from natural sources: an alternative approach for treatment and management of diabetes. Indian Journal of Natural Products and Resources . 2019;10(4): 227–237.
- Doran JCJAM. Australian trees and shrubs: species for land rehabilitation and farm planting in the tropics. ACIAR Monogr. 1997;24:314–319.
- Mahato SB Saponins with antifilarial activity from Acacia auriculiformis. In: Waller GR, Yamasaki K, editors. Saponins Used in Traditional and Modern Medicine. New York: Springer; 1996. p. 173–184.
- Pakrashi A, Ray H, Pal BC, et al. Sperm immobilizing effect of triterpene saponins from Acacia auriculiformis. Contraception. 1991;43(5):475–483.
- Kaur K, Arora S, Hawthorne ME, et al. A correlative study on antimutagenic and chemopreventive activity of Acacia auriculiformis A. Cunn. and Acacia nilotica (L.) Willd. Ex Del. Drug and Chemical Toxicology. 2002;25(1):39–64.
- Sathya A, Siddhuraju PJAPJOTM. Role of phenolics as antioxidants, biomolecule protectors and as anti-diabetic factors – evaluation on bark and empty pods of Acacia auriculiformis. Asian Pacific Journal of Tropical Medicine. 2012;5(10):757–765.
- Buavaroon, Srichaikul GB. Antioxidant and antidiabetic activities of leaf and flower extracts from Acacia auriculiformis A. Cunn. ex Benth. Global J Res Anal. 2017;6(1):660–662. doi:10.15373/2249555X.
- Okokon JE, Kackson O, Opara KN, Emmanuel E, et. al. In vivo antimalarial activity of ethanolic leaf extract of Acacia auriculiformis. Int J Drug Dev Res. 2010;2:482–487.
- Kaur R, Singh A. Anti-ulcer effect of ethanolic extract of Acacia auriculiformis. 2017.
- Tjeck OP, Souza A, Mickala P, Lepengue AN,M'Batchi B, et. al. Bio-efficacy of medicinal plants used for the management of diabetes mellitus in Gabon: an ethnopharmacological approach. J Intercult Ethnopharmacol. 2017;6(2):206.
- Khandelwal KR. Practical pharmacognosy: Techniques & Experiments. Vol. 18. Pune: Nirali Prakashan; 2007.
- Doan HV. Antidiabetic activity, glucose uptake stimulation and α-glucosidase inhibitory effect of Chrysophyllum cainito L. stem bark extract. BMC Complementary Altern Med. 2018;18(1):1–10.
- Kumar D, Kumar SJIJOPS. Phytochemical investigation and antioxidant activity of Chenopodium album. Int J Plant Sci. 2008;3(1):166–167.
- Fatima S, Akhtar MF, Ashraf KM, et al. Antioxidant and alpha amylase inhibitory activities of Fumaria officinalis and its antidiabetic potential against alloxan induced diabetes. Cellular and Molecular Biology. 2019;65(2):50–57.
- Sharma H, Kumar SJJOP. Management of metabolic syndrome by some herbs ethnic to western Himalayan region of Himachal Pradesh. J Pharmacogn Phytochem. 2016;5(3):192.
- Roh C, Jung UJIJOMS. Screening of crude plant extracts with anti-obesity activity. International Journal of Molecular Sciences. 2012;13(2):1710–1719.
- Drews G, Krippeit-Drews P, Düfer M. Oxidative stress and beta-cell dysfunction. Pflugers Arch. 2010;460(4):703–718.
- Gerber PA, Rutter GA. The role of oxidative stress and hypoxia in pancreatic beta-cell dysfunction in diabetes mellitus. Antioxid Redox Signal. 2017;26(10):501–518.
- Robertson RP, Harmon JS. Pancreatic islet β-cell and oxidative stress: the importance of glutathione peroxidase. FEBS Lett. 2007;581(19):3743–3748.
- Wang J, and Wang H. Oxidative stress in pancreatic beta cell regeneration. Oxid Med Cell Longev. 2017;2017:1930261. doi:10.1155/2017/1930261.
- Jordan SA, Cunningham DG, Marles RJ, et al. Assessment of herbal medicinal products: challenges, and opportunities to increase the knowledge base for safety assessment. Toxicology and Applied Pharmacology. 2010;243(2):198–216.
- Pratt DE. Natural antioxidants from plant material. In: Houang MT, Ho CT, Lee CY editors. Phenolic compounds in food and their effects on health II . Washington, DC: American Chemical Society; 1992. p. 54–71. ACS Symposium Series. 9780841224766. doi:10.1021/bk-1992-0507.ch005.
- Duarte AM, Guarino MP, Barroso S,Gil MM, et. al. Phytopharmacological strategies in the management of type 2 diabetes mellitus. Foods. 2020;9(3):271 doi:10.3390/foods9030271.
- Buchholz T, Melzig MFJPR. Medicinal plants traditionally used for treatment of obesity and diabetes mellitus – screening for pancreatic lipase and α-amylase inhibition. Phytotherapy Research: PTR. 2016;30(2):260–266.
- Khan SA, Al Kiyumi AR, Al Sheidi MS, Al Khusaibi TS, Al Shehhi NMAlam T, et al. In vitro inhibitory effects on α-glucosidase and α-amylase level and antioxidant potential of seeds of Phoenix dactylifera L. Asian Pac J Trop Biomed. 2016;6(4):322–329.
- Quan NV, Xuan TD, Tran HD, Thuy NTD, Trang LT, Huong CT, Andriana Y, Tuyen Y, et al. Antioxidant, α-amylase and α-glucosidase inhibitory activities and potential constituents of Canarium tramdenum bark. Molecules. 2019;24(3):605.
- Kawser Hossain M, Abdal Dayem A, Han J, et al. Molecular mechanisms of the anti-obesity and anti-diabetic properties of flavonoids. Int J Mol Sci. 2016;17(4):569.
- Chaki S, Ghosh B, Bandyopadhyay S. Detection of various phytochemical compounds from seeds of A. auriculiformis for possibilities of obtaining potent antimicrobial agents. Int J Biol Pharm Res. 2015;6(2):120–128.
- Ikarashi N, Takeda R, Ito K. The inhibition of lipase and glucosidase activities by acacia polyphenol. Evidence Complementary Altern Med. 2011;2011:272075. doi:10.1093/ecam/neq043.