ABSTRACT
Colonization of pregnant women by Streptococcus agalactiae (S. agalactiae) is the main reason for intrauterine infection or transmission during parturition. This study investigated the prevalence, antimicrobial susceptibility, serotypes, virulence traits of S. agalactiae in pregnant women across three hospitals in Dakahlia Governorate, Egypt. Isolates were identified by culturing onto Hichrome Sterp B selective medium, latex agglutination and biochemical tests. The antimicrobial susceptibility was determined by Kirby-Bauer disc diffusion method. Capsular serotypes were determined by latex agglutination test. Hemolysin production and biofilm formation were assessed quantitively. Virulence genes were detected by polymerase chain reaction (PCR). Among 290 samples, 31.7% were S. agalactiae. All isolates were sensitive to penicillin G, ampicillin, cefotaxime, and vancomycin, but notably resistant to tetracycline (83.6%), erythromycin (68.4%), and clindamycin (54.3%). Serotype III (26.08%) was most common. Strong biofilm was produced by 72.8% of isolates. High hemolytic activity was seen (80.4%). Prevalent virulence genes included fsbA (97.8%), fbsB (96.7%), and scpB (96.7%). rib and alp4 were frequent surface protein genes (60.8% and 57.6%, respectively). Serotypes were significantly correlated to clindamycin resistance, biofilm production (Ia, III, VI serotypes), hemolytic activity (serotype V), and several virulence genes and surface proteins. Our study found a high prevalence of S. agalactiae isolates in pregnant women with diverse resistance patterns, high virulence indicating potential for increased pathogenicity.
Introduction
S. agalactiae, is a Gram-positive, β-hemolytic, facultative anaerobe and catalase-negative bacterium that has the group B antigen in the cell wall. The natural habitat of S. agalactiae is the urogenital tract and lower gastrointestinal tract as a part of normal flora [Citation1]. The rate of GBS colonization among pregnant women is relatively consistent worldwide, although there may be some variations as it may range between 10% and 30% in USA [Citation2] 6.5% − 36% in Europe [Citation3], and 11.9–31.6% in Africa [Citation4]. Maternal colonization with S. agalactiae during pregnancy has been linked to life-threatening invasive diseases in newborns including sepsis, meningitis, preterm birth and maternal infection. Neonatal infection usually occurs by vertical transmission of GBS from colonized mother either during labor or after membrane rupture. To prevent perinatal GBS infection, all pregnant women should have vaginal-rectal screening between weeks 35 and 37 of their pregnancy according to United States guidelines [Citation5]. For high-risk pregnant women, intrapartum antibiotic prophylaxis is recommended to prevent maternal colonization of GBS [Citation6].
Penicillin is the drug of choice for prevention and treatment of S. agalactiae infection. However, lower penicillin susceptibility which is associated with penicillin-binding protein mutation was noticed in some cases. Depending on the result of the antibiotics susceptibility test and the guidelines, erythromycin and clindamycin can be used for women who are penicillin-allergic and if bacterial resistance to these drugs occurs, vancomycin may be used for treatment [Citation7].
Capsular polysaccharide (CPS) is a crucial virulence factor of S. agalactiae that prevents the elimination of pathogens by the immune system mainly by preventing the deposition of complement and phagocytosis [Citation8]. Different forms of CPS, which underpin the serotyping system, allow for immune evasion because α2 → 3 linked sialic acid alterations exploit molecular mimicry with host sugar epitopes. Direct attachment to immunoglobulin-like lectins (Siglecs) on leukocytes prevents the activation of complement C3 on the bacterial surface. Complement inactivation is aided further by ScpB-mediated proteolytic C5a inactivation and reduced complement binding via the inhibitory factors BibA (GBS immunogenic bacterial adhesin) and factor H [Citation9]. Immunoglobulin binding, a necessary precursor for opsonophagocytosis, is impeded by the IgA-binding beta-antigen of the c protein complex, that targets the Fc region of human IgA [Citation10].
S. agalactiae CPS has been classified into 10 serotypes including Ia, Ib and II-IX [Citation11,Citation12]. It was reported that S. agalactiae with serotypes I, II and III were detected in less than 5% of mothers with S. agalactiae infections, while serotype V was the most frequently detected etiology for S. agalactiae colonization and invasive disease in mothers and neonates [Citation13]. In the United States, United Kingdom, and France, serotype V was identified as the third most common serotype associated with maternal disease [Citation14]. Compared to regions like North America, New Zealand, and Australia, West Africa (particularly Gabon) exhibits a higher frequency of maternal colonization by serotype V [Citation15]. However, the major cause of meningitis in late onset disease is serotype III, and subtype III-2 was more virulent in early onset disease. Also, S. agalactiae serotype Ia is commonly detected [Citation16]. The prevalence and dominance of specific serotypes can vary and undergo changes over time.
GBS possesses various virulence factors that enable its survival in the hostile vaginal environment and evade elimination. Several of these factors contribute to the attachment and invasion of host epithelial cells, facilitating colonization. The adherence and invasion processes are mediated through the interaction between GBS and components of the host extracellular matrix. These interactions not only aid in resisting mechanical clearance but also help GBS evade immune detection and cross epithelial barriers [Citation17]
S. agalactiae can form biofilm structures that could increase its ability of colonization and persistance in the host. Bacterial biofilm is widely recognized as a crucial virulence factor that contributes to persistent and chronic infections. For GBS, capsule and type IIa pili have been shown to play a vital role in the formation of biofilms. These structures enable GBS to establish and maintain biofilm communities, enabling them to evade the immune system and promote long-term infection [Citation18,Citation19]
Several virulence factors have been linked to the spread and severity of S. agalactiae infection, including hyaluronidase (HylB) that acts as an endoglycosidase that cleaves glycosaminoglycan chains and degrades hyaluronic acid. This leads to disruption of the maternal-fetal barrier, allowing the spread of GBS from the vagina to the fetus resulting in severe infection and damage [Citation20,Citation21]. Furthermore, the hemolytic pigment (ornithine rhamnolipid pigment) produced by genes of the cyl operon is responsible for the hemolytic activity of S. agalactiae. In addition to the hemolytic activity, it evades the elimination by cells of the immune system (mast cells, macrophages, and neutrophils) [Citation22].
The major adhesins which mediate the interaction of S. agalactiae with host cells are fibrinogen-binding proteins (FbsA, FbsB, FbsC, Srr1, and Srr2), laminin-binding protein (Lmb), group B streptococcal C5a peptidase (ScpB), streptococcal fibronectin-binding protein A (SfbA), and immunogenic bacterial adhesin (BibA). These adhesins mediate the adherence and/or invasion of S. agalactiae within the host. Moreover, GBS immunogenic bacterial adhesin (BibA) is a cell wall-anchored protein that facilitates colonization and adherence to host cells and provides resistance against phagocytic killing. Furthermore, another cell wall-anchored protein is hypervirulent adhesin (HvgA) that is specific for ST-17 clone. HvgA mediates GBS intestinal colonization and crossing both the intestinal and blood – brain barriers resulting in meningitis [Citation23]
Also, surface proteins of S. agalactiae including Alpha-C, Rib, Alp2, Alp3, Alp4, and Epsilon protein are virulence factors that are associated with epithelial cell adherence. The β-C protein which encoded by the bac gene is associated with epithelial cell invasion and resistance to phagocyte clearance [Citation16]. Furthermore, studying the genes encoding these surface proteins can help in epidemiological analysis of GBS infections across diverse geographical regions.
The present study aims to explore the prevalence of S. agalactiae among pregnant women in different hospitals. Phenotypic and genotypic characterization of virulence traits and antibiotic susceptibility were performed. In addition, serotyping of the isolates was investigated followed by assessment of their correlation with resistance to antimicrobial agents and virulence determinants.
Materials and methods
Sampling
During the period from October 2019 to May 2020, a total 290 specimens were recovered from vaginal smear of different pregnant women at 29–35 weeks whose age was 19–40 years from different hospitals in Mansoura University Hospitals (MUH), Balqas Hospitals and Sherbeen Hospitals, Egypt. Bacterial species were identified using standard methods.
After informed agreement, one vaginal swab was obtained from each patient and placed in Amies transport medium. (Lab M) and preserved at 4°C. In a selective enrichment broth medium (Todd-Hewitt broth, oxoid, supplemented with nalidixic acid 15 μg/ml and colistin 10 μg/ml), swabs were inoculated. After 18–24 h incubation at 37°C, broths were subcultured onto chromogenic Sterp B selective medium (Himedia) and were screened for blue pigmented colonies after 24–48 h. Furthermore, latex agglutination testing (AVIPATH STREP, Omega Diagnostics Ltd.) was done immediately on broth cultures. Positive GBS broth cultures (either by latex agglutination testing or group B selective medium) were verified by subculturing onto 5% blood agar and incubated for 18–24 h at 37°C. Gram stain, hemolysis, catalase, and the CAMP test were used to identify colonies [Citation7,Citation24,Citation25].
Demographic data collection
Demographic data including women age, parity, history of previous abortion and previous preterm labor were recorded.
Antimicrobial susceptibility testing
The antimicrobial susceptibility testing was carried out by disk diffusion method using Muller-Hinton agar(MHA) [Citation26]. The susceptibility of all isolates were tested for 12 different antimicrobial agents including ceftriaxone (CRO, 30 µg), cefepime (FEP, 30 µg), cefotaxim (CTX, 40 µg) penicillin (P, 10 µg), ampicillin (AMP, 10 µg), erythromycin (E 15 µg), clindamycin (DA 2 µg), levofloxacin(LEV, 5 µg), Meropenem(M, 10 µg), vancomycin (VA, 30,µg), tetracycline (TE, 30 µg), and chloramphenicol (C, 30 µg) (Oxoid, UK). Interpretation of the results was performed according to Clinical Laboratory Standards Institute (CLSI, 2019) [Citation27].
Serotyping reactions
Type specific antisera were used according to the method of Lancefield et al. and modification by Slotved et al. [Citation28]. Latex suspension was prepared by dilution of 1 µl of the antiserum with 0.5 ml of 1% latex suspension [1 ml of 10% latex solution was added to 9 ml of glycine buffered saline (pH 8.2)], followed by incubation at 55°C in a water bath for 20 min including four times vortexing at equal time intervals. After cooling, 20 µl of bovine serum albumin were added per ml to the suspension and stored at 5°C. Latex reaction was performed on a glass slide by adding equal volume of the previously prepared suspension to S. agalactiae MHB 24 h culture with mixing and observing the agglutination within 10 to 15 s [Citation11].
Phenotypic detection of some S. agalactiae virulence factors
Quantitative detection of biofilm production
Biofilm production was tested in 96-well sterile microtiter plate with flat bottom by adsorption method [Citation29]. One pure colony of each isolate was cultured in 5 ml Todd-Hewitt and incubated overnight at 37°C. A volume of 200 μl aliquots of adjusted fresh culture (OD to 0.23–0.27) was inoculated into four adjacent wells. A negative control containing Todd- Hewitt broth only was included in the same plate. After an overnight incubation at 37°C without shaking, the content of wells was aspirated followed by three time washing with 200 μl sterile physiological saline with shaking to remove adherent cells. Biofilm producing cells were fixed by the addition of 150 μl absolute methanol in each well followed by incubation for 20 min, then left to dry. The fixed biofilm was stained by 150 μl of 1% crystal violet for 15 min. The plate was washed to remove the excess dye. Then, 150 μl of 33% (v/v) glacial acetic acid was added to solubilize the bound dye with biofilm. Results were recorded by measuring the plate absorbance at 490 nm. Optical density of the tested isolate (ODT) was calculated from the mean OD of the three wells and compared with cut off optical density (ODC) which is calculated as three standard deviations above the mean of the negative control. Isolates were classified as non-adherent, weakly adherent, moderately adherent and strongly adherent as mentioned previously [Citation29].
Quantitative detection of hemolysin production
A pure colony was inoculated in 5 ml brain heart infusion (BHI) broth and incubated overnight at 37°C followed by adjustment of each bacterial suspension to OD600 of 0.23–0.27. After that, a volume of 100 µl of the suspension was added to 5 ml sterile BHI broth and incubated overnight at 37°C with shaking at 150 rpm followed by centrifugation at 10.000 rpm for 5 min. The prepared crude extract was used for testing hemolysin.
A volume of 500 µl of the prepared RBCs suspension was mixed with 500 µl of the bacterial extract of each isolate in a sterile eppendorf tube followed by incubation for 2 h at 37°C. A positive control was prepared by using 0.1% SDS instead of the bacterial extract, while BHI broth was used as a negative control. After incubation, the reaction mixture was centrifuged at 10,000 rpm for 10 min followed by measurement of the absorbance of hemoglobin released in the supernatant at 600 nm [Citation30].
Genotypic detection of virulence genes
All S. agalactiae isolates genomic DNA was extracted using extraction kit (Gene JET Genomic DNA Purification kit k0721) [Citation31]. Using the primers listed in , PCR was used to amplify virulence-associated genes: fbsA (Protein FbsA), fbsB (Protein FbsB), bac (components of protein C), lmb (Protein Lmb), hylB (hyaluronidate lyase), scpB (C5a peptidase), cylB (Hemolysin), and HvgA (hypervirulent adhesion protein). PCR amplifications were carried out in a Thermal Cycler T100TM (Bio-Rad, USA). The final volume (50 μL) contained 25 μL of Master mix, 15 μL of water, 2 μL of each primer with a concentration of 0.8 μM and 2 μL of template DNA. The initial denaturation temperature was set at 95°C for 30 s, extension at 72°C for 15 s, the annealing temperature specified for each gene is listed in for 30 s and a final extension at 72°C for 1 min. UV transillumination was used to visualize PCR products, using a 1.5% (w/v) agarose gel (Bio-Rad Chemi-Doc™MP System).
Table 1. Primer list used to amplify virulence-associated genes.
Surface protein genes genotyping
The detection of surface protein encoded genes was performed using a multiplex PCR reaction. Amplification reactions contained 12.5 µl master mix (Dream Taq mix, 1 µl of each primer, 3 µl of the template DNA and nuclease free water to 25 µl). A negative control was included by using nuclease free water instead of DNA. The PCR program was set by first denaturation at 94°C for 5 min, followed by 30 cycles of denaturation at 94°C for 45 s, annealing at 56°C for 30 s, and extension at 72°C for 35 s. The final extension was set at 72°C for 5 min. The amplified products were visualized by agarose gel electrophoresis, and their sizes were compared with GeneRuler 100 bp Plus DNA Ladder (ThermoFisher Scientific, UK) [Citation16].
Statistical analysis
Data were entered and analyzed using IBM-SPSS software (IBM Corp. Released 2017. IBM SPSS Statistics for Windows, Version 25.0. Armonk, NY: IBM Corp.). Qualitative data were expressed as frequency and percentage. The Chi-Square test was used for comparing data.
Ethical considerations
The ethics committee of the Faculty of Pharmacy at EL-Mansoura University, Egypt, gave their clearance (2022–101). All the participants in this study gave their informed permission.
Results
Identification of isolates
In the present study, 92 out of 290 (31.7%) isolates were identified as S. agalactiae including 47 isolates from Balqas Central Hospital, 26 isolates from Sherbeen Central Hospital and 29 isolates from Mansoura Gynecological Hospital.
Demographics
The incidence of S. agalactiae across different age categories was studied. The mean age of the patients in the study was 29.06 ± 4.95. The minimum and maximum ages observed were 19 and 42 years, respectively. In terms of age categories, the highest proportion of isolates (58.7.1%, 54 isolates) fell within the 21–30 years range. This was followed by 33 isolates (35.9%) in the >30 years category. The age group below 20 years accounted for 5.4% (5 isolates) of the patients. In this study, 9 (9.8%), 23 (25%), 33 (35.9%), 12 (13%), and 15 (16.3%) pregnant women were experiencing their first, second, third, fourth, and fifth pregnancy. In addition, 19 (20.6%), 17(18.4%), 49(53.2%), and 7(7.6%) participants were in the 30th, 35th,36th, and 37th week of gestation. Moreover, out of pregnant women, 69(75%) had a history of abortion, while 23 (25%) pregnant women had a history of membrane rupture. shows the parity, gravidity, and abortion for all studied cases.
Table 2. Demographic characters of studied cases
Antimicrobial susceptibility
demonstrates the antimicrobial susceptibility of S. agalactiae isolates to 12 antimicrobials from different classes. It was shown that all the isolates were susceptible to penicillin G, ampicillin, cefotaxime, and vancomycin. The highest resistance was observed to tetracycline (83.6% of isolates) followed by erythromycin (68.4%) and clindamycin (64.1%). Moreover, resistance to chloramphenicol and levofloxacin was revealed by 50% and 45.6% of isolates, respectively. In contrast, the lowest resistance percentage was noticed for cefepime (11.9%).
Serotyping of S. agalactiae isolates
Different serotypes of S. agalactiae isolates are demonstrated in . Our analysis revealed that the presence of eight different serotypes among the tested isolates. Serotype III was detected in 24 (26.08%) isolates, serotype Ia in 17 (18.47%) isolates, serotype IV in 11 (11.95%) isolates, and serotype II in 8 (8.6%) isolates. Additionally, serotypes V and Ib were found in 7 (7.6%) isolates each. Serotypes VIII and VI were less common, 5 (5.4%) and one (1.08%) isolate(s), respectively. Twelve (13.04%) isolates were found to be non-typable.
Quantitative detection of the biofilm formation
The capacity of S. agalactiae isolates to form biofilm that was assessed using microtiter plate was scored as follows: ODT ≤ 0.24 is non -adherent (NA), 0.24< ODT ≤ 0.48 is weakly adherent (W), 0.48 < ODT ≤ 0.96 is moderately adherent (M) and 0.96< ODT is strongly adherent (S). Biofilm production was observed in all isolates. Among the tested isolates, 67 (72.8%) were classified as strong biofilm producers, 20 (21.7%) as moderate biofilm producers, and 5 (5.43%) as weak biofilm producers.
Quantitative detection hemolysin production
Hemolytic activity of S. agalactiae isolates was tested using 2% RBCs (O-type) suspension. Interestingly, 9 (9.7%) isolates showed 100% cell lysis. Sixty-five (70.6%) isolates exhibited an increased hemolytic activity (>50% and < 100% hemolysis) and 18 (19.56%) showed less than 50% hemolysis.
Correlation between antibiotics resistance, biofilm, hemolysis, and serotypes
Correlation between antibiotics resistance and serotypes
displays the distribution of antibiotic resistant S. agalactiae isolates among different serotypes. Serotype III was the highest detected serotype among the resistant strains of each antibiotic. While serotype II was not detected among isolates resistant to either ceftriaxone or meropenem, four serotypes (Ia, II, VI, and VIII) were not detected among cefepime resistant isolates. Clindamycin resistance was found to be significantly distributed among different serotypes (P = 0.025). Concerning the other antibiotics, all serotypes were insignificantly distributed with different percentages among the resistant isolates (P > 0.05).
Table 3. Distribution of antibiotics resistance among different serotypes of S. agalactiae isolates.
Correlation between biofilm forming capacity and serotypes
Among the strong biofilm producers, serotype III had the highest occurrence with 19 isolates, followed by serotype Ia with 11 isolates. Serotypes Ib, II, VI and VIII were also identified as strong biofilm producers, with 5 isolates each. A group of strong biofilm producers (8 isolates) was classified as untypable. Regarding moderate biofilm producers, serotype Ia was the most prevalent with 6 isolates, followed by serotypes III and II with 4 and 3 isolates, respectively. Other serotypes (Ib, IV, and V) were also identified as moderate biofilm producers. In the weak biofilm producer category, only three serotypes (III, IV, and V) were represented by one isolate each ().
Table 4. Correlation between biofilm forming capacity and different serotypes of S. agalactiae isolates.
The distribution of different biofilm categories shows significance difference within isolates of serotypes Ia, III, VI and untypable isolates (P = 0.003, P < 0.001, P = 0.002, and P = 0.008, respectively).
Correlation between biofilm forming capacity and antibiotics resistance
Statistical analysis has revealed that there was no significant correlation between different categories of biofilm formation capacity and resistance to different of antimicrobial agents (P > 0.05) ().
Table 5. Correlation between biofilm formation capacity and resistance to different antimicrobial agents among S. agalactiae isolates.
Correlation between hemolysis production and different serotypes
Complete (100%) hemolysis was produced only by Ib, II, III, IV and untypable serotypes. All detected serotypes were observed among isolates producing > 50% hemolysis, and serotype III had the highest incidence (17 isolates), followed by serotype Ia with (15 isolates). Similarly, serotype III was the highest detected one among isolates producing < 50% hemolysis (6 isolates), followed by serotype V (4 isolates) (). Distribution of different levels of hemolysis differs significantly within serotype V (P = 0.03).
Table 6. Correlation between hemolysis production and different serotype among S. agalactiae isolates.
Molecular detection of the tested virulence associated genes
shows the findings of PCR amplification of fsbA, fbsB and scpB genes. They were amplified in 97.8%, 96.7%and 96.7% of isolates, respectively. Likewise, lmb and cylB encoding genes were detected in 94.5%, and 93.5% of isolates, respectively. Also, bac, hylB and hvgA were common detected genes among our isolates where they were harbored by 71.7%, 68.4% and 57.6% of isolates, respectively.
Surface protein genes genotyping
The prevalence of surface anchored protein genes (alpha-C, rib, epsilon, alp2/3 and alp4) among the 92 S. agalactiae isolates was determined by multiplex PCR. The most predominant surface protein genes are rib and alp4 as they were detected in 56 (60.8%) and 53 (57.6%) isolates, respectively. Regarding Epsilon and alp2/3 genes, they were detected in 52 (56.5%) isolates, each. The least predominant gene was alpha-C (7.6%) ().
Figure 4. Electrophoretic graph of surface anchored protein genes in some represented isolates of S. agalactiae. Alpha-C: 398bp, rib: 295bp, epsilon:200bp, alp2/3: 334bp, alp4:130bp. M: 100bp DNA marker, C: negative control.
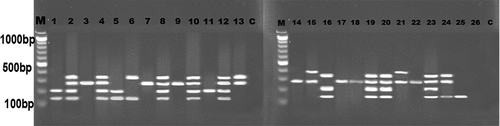
It was noticed that 30 (32.6%) isolates contained a single surface protein gene. Combinations of two genes were exhibited by 20 (21.7%) isolates. Moreover, 16 (17.4%) and 23 (25%) isolates contained combinations of three and four surface protein genes, respectively. Only one (1.1%) isolate harbored the five surface protein genes.
The profiles of surface protein tested among Streptococcus agalactiae isolates were diverse where a total of 17 different profiles were observed (). The most frequent profile associated with isolates was P15 (rib, epsilon, alp2/3 and alp4), it was exhibited by 22 (23.9%) isolates. Both P2 (rib) and P3 (epsilon) were observed in 13 (14.1%) isolates, each. Only one isolate harbored the five surface protein genes (P17).
Table 7. Profile patterns of Surface protein genes associated with S. agalactiae isolates.
Correlation between tested virulence encoding genes and different serotypes
The correlation between the detected virulence genes and serotypes of S. agalactiae isolates is shown in . All detected serotypes were observed among isolates harboring bac, cylB, lmb, and alp 4 genes. Serotype III was the most detected among isolates positive for fbsA, fbsB, hylB, cylB, lmb, scpB, hvgA, and rib genes. Ia was the most detected serotype among isolates harboring bac, epsilon, alp 2/3, and alp 4 genes. For alpha-C gene, serotype II was represented by the highest number of isolates.
Table 8. Correlation between the tested virulence genes and different serotypes among S. agalactiae isolates.
Concerning virulence associated genes, the distribution of fbsA, bac, hylB, scpB, hvgA genes among different serotypes showed significant difference (P = 0.006, P = 0.001, P ≤ 0.001, P = 0.018, and P = 0.006, respectively). Surface protein genes (rib, epsilon, alp2/3 and alp4) exhibited varying existence across serotypes, and their association with serotypes was statistically significant (P = 0.001, P ≤ 0.001, P = 0.009, and P = 0.028, respectively). alphaC gene had a low overall presence, with only serotypes II showing the highest percentage 37.5%. However, there was no significant association between alpha-C gene and serotypes.
Correlation between virulence genes and biofilm forming capacity
Comparing the prevalence of different virulence genes among different biofilm categories of the tested isolates revealed that there is no significant difference in respect to all virulence genes except for hylB gene (P = 0.037) ().
Table 9. Correlation between virulence genes and biofilm forming capacity among S. agalactiae isolates.
Discussion
S. agalactiae is a common colonizer of the urogenital tract in pregnant women all over the world, and it is a known primary risk factor for newborn sepsis caused by this organism. For the prevention and control of such infections, it is critical to monitor the epidemiology of S. agalactiae and their antibiotic resistance [Citation34]. In our study, the vaginal colonization rate by S. agalactiae was 31%, and the result was slightly higher than that reported by Shabayek in Ismailia, Egypt (27.9%) [Citation35] and higher than the result obtained by Elbaradie et al. at AlFayom, Egypt [Citation36]. Also, other studies informed lower prevalence rate of S. agalactiae isolated from vaginal samples in Ethiopia, Morocco, Nigeria, and Malawi (20.5%, 20.2%, 18% and 16.5%, respectively) [Citation37–40]. This disparity could be explained by the fact that the rates of S. agalactiae colonization vary widely throughout the world, and many factors affect the colonization rate of S. agalactiae in vaginal canal like food habits, climate, maternal hygiene and culture methods, including the number and type of sites cultured and type of medium used [Citation41].
Regarding age, the present study demonstrates that S. agalactiae coloization can occur across a wide age range (19–42 years). Results showed that pregnant women between the ages of 21–30 years had the highest incidence of S. agalactiae colonization (58.7%) which reinforces the increased vulnerability of women to S. agalactiae infections during their childbearing years. In agreement with our results, a previous study at Trinidad and Tobago reported that about 45.8% women of age 21–29 years were carriers of GBS [Citation42]. Similarly in Egypt, Shabayek reported that women with the same age had the highest colonization percent with GBS [Citation35]. Conversely, a study from the Netherlands found that there were no differences in GBS colonization with respect to age [Citation43]. This different in percentage can possibly underscore the fact that GBS colonization might be subjected to multiple factors which may vary from one geographical location to another.
There are variable results on the effect of parity on GBS colonization. Our results showed that the highest GBS colonization rates were associated with multiple parity (delivered 2 times, 39.1%). In various studies, no significant differences in colonization rates were noted based on age or parity, but increasing age or parity has also sometimes been associated with increased GBS colonization. In the latter study, they found higher GBS colonization (66.4%) in women who were multipara as delivered 2 or more than 2 times compared to fewer numbers of deliveries, but the difference was not statistically significant. Similarly, a study in Saudi Arabia found the higher GBS colonization in women with parity more than 5 times (50%) [Citation44]. Studies from Tanzania [Citation45] and the Netherlands [Citation43] showed that women with zero parity had a quite substantial rate of GBS colonization, (23.9% and 21%, respectively). Reasons for such variable colonization are unclear and need further study.
In our study, serotyping of the isolates revealed the presence of 8 serotypes. Serotype III was the most frequently recovered among the 92 S. agalactiae isolates as it was detected in 26.08% of isolates, followed by serotype Ia (18.47%), serotype II (9.7%), then serotypes V and Ib (7.6%). Serotypes VIII and VI were less frequently observed as they were represented by only 5.4% and 1%, respectively. Notably, 10.8% of the isolates could not be typed. These findings are consistent with previous studies by Wang et al. in China and Bobadilla et al. in Argentina where similar serotypes were reported [Citation8,Citation46]. Serotype III was the predominant serotype among our isolates, which is consistent with Yao et al. who reported that serotype III was the most frequently identified serotype (23.3%) [Citation47]. In contrast, Suhaimi et al. reported the prevalence of serotype Ia among 45% of the isolates, followed by III, V, VI, VIII and VII serotypes [Citation48]. Also, our findings align with previous studies that have reported a low prevalence of serotypes VI and VIII compared to other serotypes, and serotypes IX and VII were not commonly detected in human infections [Citation47]. Regional differences can explain this variation in the distribution of serotypes S. agalactiae.
The proportion of non-typable strains (10.8%) which was observed in this study aligns with findings reported by Bobadilla et al. who found a lower detection rate (5.5%) [Citation8]. The inability to detect these strains using the agglutination test could potentially be attributed to a limited expression of the capsule [Citation49].
Results of antibiotic susceptibility testing demonstrated that all strains were sensitive to penicillin, ampicillin, vancomycin, and cefotaxime. However, the highest level of resistance was recorded in the tetracycline whereas 83.6% of strains were resistant followed by erythromycin and clindamycin (68.4% and 64.1%, respectively). On contrast, the lowest resistance percentage was noticed for cefepime (11.9%). Although erythromycin is commonly recommended in case of allergy to penicillin, resistance was detected in 68.4% of our isolates. Similar result was reported by Li et al. where GBS were sensitive to penicillin and vancomycin, 60.87% of strains were resistant to erythromycin and 52.17% were resistant to clindamycin [Citation50]. In addition, in Brazil Melo et al. reported similar result as tetracycline resistance was the highest one (82.3%) followed by erythromycin and clindamycin, and all isolates were sensitive to penicillin and vancomycin [Citation51]. Also, Mengist et al. in Ethiopia reported 100% susceptibility of their GBS isolates to penicillin G and reduced susceptibility (90%) to erythromycin [Citation52]. Regarding ampicillin, all isolates were found to be sensitive in previous studies conducted in Germany, India and China [Citation53–55]. In Egypt, Sadaka et al. reported 100% sensitivity to vancomycin [Citation56]. However, a study in Saudi Arabia by Khan et al. revealed reduced susceptibility to macrolides, with 16% and 5% of the isolates being resistant to erythromycin and clindamycin, respectively [Citation57]. Unlike our results, Motlová et al. and Suhaimi et al. reported higher sensitivity of tested isolates to tetracycline (83.9% and 90%, respectively) [Citation48,Citation58]. The observed rates of resistance, or even greater rates of resistance, to erythromycin, clindamycin, and chloramphenicol identified in our study support results reported by Lambiase et al., and Emaneini et al. [Citation59,Citation60]. Because of the high rates of GBS resistance to erythromycin and clindamycin, these antimicrobials should be used with caution for GBS prophylaxis. To guide the choice of antimicrobial medications used for prophylaxis in pregnant women and to assess the resistance profile of GBS to the most commonly used antibiotics, susceptibility tests must be done.
Studying the correlation between resistance antibiotics and serotypes revealed that serotype III was the highest detected serotype among the resistant strains of each antibiotic. While serotype II was not detected among isolates resistant to either ceftriaxone or meropenem, four serotypes (Ia, II, VI, and VIII) were not detected among cefepime resistant isolates. Association of antibiotic resistance serotypes was repoted by previous studies. Different associations were found by Genovese et al. [Citation61]. Unlike our results, they reported that the highest percentage of clindamycin-resistant and erythromycin sensible phenotype was found in serotype V followed by serotype Ia and also similar percentages of clindamycin- and erythromycin-resistant phenotype were observed in serotypes III [Citation61].
GBS pathogenesis involves various virulence factors, which includes adhesins, enzymes, carbohydrates, and various proteins. Biofilm is associated with antimicrobial resistance and medical devices mediated infections [Citation62]. It is considered as one of the important contributing factors in streptococcal infections. Many observations indicated that streptococcal infections of soft tissues may be a result of biofilm contribution. In these types of infections, streptococcal cells grow in close to each other in the infected sites to form the bacterial community [Citation63].
In the present study, all the 92 tested S. agalactiae isolates exhibited positive biofilm formation. Different biofilm production abilities were identified among the tested isolates including 72.8% strongly adherent, 21.7% moderately adherent and 5.43% weakly adherent. The result was higher than that reported by Kaczorek et al., who found that more than 70% of the tested S. agalactiae isolates were biofilm producers among which only 7% of isolates were strongly adherent [Citation64]. This variation in the two studies may be attributed to divergent sample sizes, different isolation sources and nutritional content of the medium [Citation65]
Overuse of antibiotics not only contributes to the emergence of biofilm-mediated antibiotic resistance strains but also helps microbes to adapt to many external stress conditions by forming biofilms. It has been suggested that biofilm formation plays an important role in antibiotic resistance by decreasing the antibiotic penetration rate and regulating bacterial gene expression, which makes it hard to treat bacterial infections [Citation66]. Bacteria found in biofilms may be immune-resistant to antibiotics and other therapies [Citation67]. In our study, most of the resistance strain to antibiotics showed high biofilm capacity formation, but statical analysis has revealed that there was no significant correlation between different categories of biofilm formation capacity to different antimicrobial agents.
Also, the correlation between the biofilm forming capacity and different serotypes of S. agalactiae isolates was investigated. The results revealed interesting associations between these two factors. Serotypes III and Ia were consistently associated with strong biofilm production, indicating a potential role of these serotypes in biofilm-associated infections. However, further research is needed to elucidate the mechanisms underlying this correlation.
Furthermore, it has been observed that virulence genes contribute to pathogenicity by promoting biofilm development, implying a link between the presence and expression of virulence genes and biofilm creation [Citation68]. In our study, high frequencies of the virulence genes FbsA,FbsB,lmb,Cylb and ScpB were detected in the GBS isolates and most isolates carried multiple virulence genes, these genes forming high biofilm which allowed the GBS strains to adhere, colonize, and invade host tissues. However, there is no significant difference with respect to all virulence genes except for hylB gene (P = 0.037).
Hemolysin is the virulence factor produced by hemolytic streptococci that is responsible for lysing the host blood components (erythrocytes, platelets and leukocytes) through pore formation in the cell membrane [Citation69]. As in our study, 65 (70.6%) isolates exhibited an increased hemolytic activity (>50% hemolysis) and 18 (19.56%) showed less than 50% hemolysis. Interestingly, 9 (9.7%) isolates showed 100% cell lysis. There is a difference in hemolysin production according to serotypes, as Complete (100%) hemolysis produced only by Ib, II, III, IV and untypable serotypes. Moreover, the distribution of different levels of hemolysis differ significantly within only serotype V (P = 0.03).
In the current study, molecular investigation of virulence associated genes was performed using PCR. fsbA and fbsB, scpB genes were the most predominant detected genes in our study whereas they were amplified in 97.8%, 96.7%, and 96.7%, respectively, followed by lmb, cylB, and bac genes that were amplified in 94.5%, 93.5%, and 71.7%, respectively. On the contrary, hylB and hvgA genes were the least common among our isolates whereas they were amplified in 68.4% and 57.6% of isolates, respectively. These results are similar to Oviedo et al. who detected lmb and hylB genes in 94.30% and 79.50% of the tested isolates, respectively [Citation70]. Also, similar results were found by to Hannoun et al. who reported scpB gene in 96.10% of their isolates, and scpB, fbsA and fbsB genes were detected in all isolates [Citation25]. Also, Dutra et al. and Bobadilla et al. found that all isolates carried the scpB gene [Citation8,Citation71]. Other studies reported lower frequencies for the scpB gene, which were 88.30% [Citation72], and 94.70% [Citation25]. In contrast to our results, Souza et al. and Dutra et al. reported lower percentages of bac gene (6.60% and 13.1%, respectively) [Citation71,Citation73]. Like serotypes, the corresponding variation in virulence of S. agalactiae may be attributed to regional differences.
In addition to capsular typing, the classification of S. agalactiae isolates can be achieved by typing surface protein antigens. The main types of surface localized proteins are Alpha-C, Rib, Alpha like protein 4, Epsilon and Bac protein [Citation74]. Our PCR results indicated that the most predominant surface protein genes are rib and alp 4 where they were detected in 58.6% and 60.8% of isolates, respectively, followed by epsilon and alp 2/3 by 54.3% and 53.2% of isolates, respectively. The least predominant gene was alpha-C that was harbored by only 8 isolates. By comparing our result with that of Sadah et al., rib gene was identified at the same percent of isolates while alpha- C gene was harbored by 3% and none of the tested isolates contained alp 4 gene [Citation16]. On the other hand, Shabayek et al. reported that epsilon gene was the most identified among their tested isolates followed by alpha C and rib genes (27%, 18% and 16%, respectively) and none of the tested isolates possessed alp4 gene [Citation74]. Coexistence of two or more surface protein genes was found in 65% of isolates. Simultaneous detection of rib, epsilon, alp2/3 and alp4 genes was detected in 23.9% of isolates.
Our results demonstrate a correlation between various virulence genes and serotypes of S. agalactiae isolates. The present study found that the fbsA and scpB genes showed a significant association with serotypes, and they were present in 100% of isolates for most serotypes. On the other hand, bac, hylB, and hvgA genes exhibited different percentages across serotypes, and their association with serotypes was statistically significant. Additionally, fbsB, cylB, and lmb genes were detected in a high percentage of isolates across most serotypes, ranging from 75% to 100%. However, no significant association was observed between these genes and serotypes (P > 0.05). Surface protein genes, including rib, epsilon, alp2/3, and alp4, also exhibited varying presence across serotypes, and their association with serotypes was statistically significant (P < 0.05). However, there was no significant association observed between alpha-C gene and serotypes. Several previous investigations have explored the relationship between virulence factors and serotypes of S. agalactiae. Bobadilla et al. conducted a similar analysis and reported a significant association between certain virulence genes and serotypes [Citation8], whereas they found an association between serotypes and bac, cylB, rib and lmb genes which is partly consistent with the current study’s findings. Additionally, Dore et al. and Manning et al. have reported statistically significant associations between serotypes and genes such as bac, bca, hylB, and rib [Citation75,Citation76]. Moreover, Martins et al. found associations between serotype Ib and bca, serotype II and bca, gene and serotype III and rib [Citation65]. The findings of Saleh et al. indicated a significant association between the surface protein genes and serotypes, which is consistent with the results obtained in the current study [Citation16]. These studies provide further evidence of the correlation between virulence factors and serotypes in S. agalactiae.
In conclusion
This study provides important insights into the prevalence, characteristics, and virulence profiles of S. agalactiae isolates in Egyptian hospitals. The observed prevalence of S. agalactiae indicates its significance as a pathogen in the studied population. The high resistance to certain antimicrobials underscores the need for judicious use of antibiotics and continuous surveillance of resistance patterns. The identified serotypes highlight the diversity of S. agalactiae strains circulating in the region. The widespread biofilm formation capacity emphasizes the potential role of biofilms in the persistence and virulence of these isolates. The detection of various virulence-associated genes suggests their contribution to the pathogenicity of S. agalactiae. The significant associations between certain genes and serotypes provide further insights into the genetic diversity and virulence potential of different strains. These findings contribute to our understanding of S. agalactiae epidemiology, aiding in the development of preventive strategies and potential therapeutic interventions. Continued surveillance and monitoring of S. agalactiae strains are essential for effective control and management of infections caused by this pathogen.
Disclosure statement
No potential conflict of interest was reported by the author(s).
References
- Delfani S, Bahmani M, Mohammadrezaei-Khorramabadi R, et al. Phytotherapy in streptococcusagalactiae: an overview of the medicinal plants effective against streptococcus agalactiae. J Clin Diagn Res. 2017;11(6):De01–de02. doi: 10.7860/JCDR/2017/25530.9988
- Schuchat A. Epidemiology of group B streptococcal disease in the United States: shifting paradigms. Clin Microbiol Rev. 1998;11(3):497–513. doi: 10.1128/CMR.11.3.497
- Barcaite EB, Tameliene A, Kliucinskas R, et al. Prevalence of maternal group B streptococcal colonisation in European countries. Acta Obstet Gynecol Scand. 2008;87(3):260–271. doi: 10.1080/00016340801908759
- Ippolito DL, James WA, Tinnemore D, et al. Group B streptococcus serotype prevalence in reproductive-age women at a tertiary care military medical center relative to global serotype distribution. BMC Infect Dis. 2010;10(1):336. doi: 10.1186/1471-2334-10-336
- Raabe VN, Shane AL. Group B Streptococcus (Streptococcus agalactiae). Microbiol Spectr. 2019;7(2):10.1128/microbiolspec.GPP3-0007-2018. doi: 10.1128/microbiolspec.GPP3-0007-2018
- Khademi F, Sahebkar A. Group B streptococcus drug resistance in pregnant women in Iran: a meta-analysis. Taiwanese J Obstetrics Gynecol. 2020;59(5):635–642. doi: 10.1016/j.tjog.2020.07.002
- Santana FAF, de Oliveira TVL, de Souza Filho MB, et al. Streptococcus agalactiae: identification methods, antimicrobial susceptibility, and resistance genes in pregnant women. World J Clin Cases. 2020;8(18):3988–3998. doi: 10.12998/wjcc.v8.i18.3988
- Bobadilla FJ, Novosak MG, Cortese IJ, et al. Prevalence, serotypes and virulence genes of streptococcus agalactiae isolated from pregnant women with 35–37 weeks of gestation. BMC Infect Dis. 2021;21(1):73. doi: 10.1186/s12879-020-05603-5
- Jarva H, Hellwage J, Jokiranta TS, et al. The group b streptococcal β and pneumococcal hic proteins are structurally related immune evasion molecules that bind the complement inhibitor factor h in an analogous fashion 1. J Immunol. 2004;172(5):3111–3118. doi: 10.4049/jimmunol.172.5.3111
- Jerlström PG, Chhatwal GS, Timmis KN. The IgA-binding beta antigen of the c protein complex of Group B streptococci: sequence determination of its gene and detection of two binding regions. Mol Microbiol. 1991;5(4):843–849. doi: 10.1111/j.1365-2958.1991.tb00757.x
- Slotved HC, Kong F, Lambertsen L, et al. Serotype IX, a proposed new streptococcus agalactiae serotype. J Clin Microbiol. 2007;45(9):2929–2936. doi: 10.1128/JCM.00117-07
- Johri AK, Paoletti LC, Glaser P, et al. Group B Streptococcus: global incidence and vaccine development. Nat Rev Microbiol. 2006;4(12):932–942. doi: 10.1038/nrmicro1552
- Matsubara K, Mikamo H, Numa M, et al. Three fatal cases of invasive serotype VI group B streptococcal infection. J Infect. 2006;53(3):139–142. doi: 10.1016/j.jinf.2005.11.023
- Hall J, Adams NH, Bartlett L, et al. Maternal disease with group B Streptococcus and serotype distribution worldwide: systematic review and meta-analyses. Clinl Infect Dis. 2017;65(suppl_2):S112–S124. doi: 10.1093/cid/cix660
- Russell NJ, Seale AC, O’Driscoll M, et al. Maternal colonization with group B Streptococcus and serotype distribution worldwide: systematic review and meta-analyses. Clinl Infect Dis. 2017;65(suppl_2):S100–S111. doi: 10.1093/cid/cix658
- Sadeh M, Firouzi R, Derakhshandeh A, et al. Molecular characterization of streptococcus agalactiae isolates from pregnant and non-pregnant women at yazd university hospital, Iran. Jundishapur J Microbiol. 2016;9(2):e30412. doi: 10.5812/jjm.30412
- Vornhagen J, Adams Waldorf KM, Rajagopal L. Perinatal group b streptococcal infections: virulence factors, immunity, and prevention strategies. Trends Microbiol. 2017;25(11):919–931. doi: 10.1016/j.tim.2017.05.013
- Konto-Ghiorghi YM, Mallet E, Duméni A, et al. Dual role for pilus in adherence to epithelial cells and biofilm formation in Streptococcus agalactiae. PLoS Pathog. 2009;5(5):e1000422. doi: 10.1371/journal.ppat.1000422
- Xia FD, Mallet A, Caliot E, et al. Capsular polysaccharide of Group B Streptococcus mediates biofilm formation in the presence of human plasma. Microbes Infect. 2015;17(1):71–76. doi: 10.1016/j.micinf.2014.10.007
- Vornhagen J, Quach PB, Merillat E, et al. Bacterial hyaluronidase promotes ascending GBS infection and preterm birth. MBio. 2016;7(3). doi: 10.1128/mBio.00781-16
- Liu Y, Liu J. Group B streptococcus: virulence factors and pathogenic mechanism. Microorganisms. 2022;10(12). doi: 10.3390/microorganisms10122483
- Whidbey C, Harrell MI, Burnside K, et al. A hemolytic pigment of group b streptococcus allows bacterial penetration of human placenta. J Exp Med. 2013;210(6):1265–1281. doi: 10.1084/jem.20122753
- Tazi A, Disson O, Bellais S, et al. The surface protein HvgA mediates group B streptococcus hypervirulence and meningeal tropism in neonates. J Exp Med. 2010;207(11):2313–2322. doi: 10.1084/jem.20092594
- Hensler ME, Quach D, Hsieh C-J, et al. CAMP factor is not essential for systemic virulence of group b streptococcus. Microb Pathog. 2008;44(1):84–88. doi: 10.1016/j.micpath.2007.08.005
- Hannoun A, Shehab M, Khairallah M-T, et al. Correlation between group b streptococcal genotypes, their antimicrobial resistance profiles, and virulence genes among pregnant women in lebanon. Int J Microbiol. 2009;2009:1–6. DOI:10.1155/2009/796512
- Bauer AW, Kirby WMM, Sherris JC, et al. Antibiotic susceptibility testing by a standardized single disk method. Am J Clin Pathol. 1966;45(4):493–496. doi: 10.1093/ajcp/45.4_ts.493
- CLSI. Clinical and Laboratory Standards Institute Methods for dilution of antimicrobial susceptibility for bacteria that grow aerobically. Approved Standard 10th ed. Wayne (PA): Clinical and Laboratory Standards Institute M100; 2019. (CLSI Document M07-A10).
- Lancefield RC, McCarty M, Everly WNJTJOEM. Multiple mouse-protective antibodies directed against group B streptococci. Special reference to antibodies effective against protein antigens. J Exp Med. 1975;142(1):165–179. doi: 10.1084/jem.142.1.165
- Stepanović S, Vuković D, Dakić I, et al. A modified microtiter-plate test for quantification of staphylococcal biofilm formation. J Microbiol Methods. 2000;40(2):175–179. doi: 10.1016/S0167-7012(00)00122-6
- Sato T, Kamaguchi A, Nakazawa F. Purification and characterization of hemolysin from Prevotella oris. J Oral Biosci. 2012;54(2):113–118. doi: 10.1016/j.job.2012.03.002
- Sambrook J, Russell D. Molecular Cloning: A Laboratory Manual (3-Volume Set). Vol. 1. New York: Spring Harbor Laboratory Press; 2001.
- Teatero S, McGeer A, Low DE, et al. Characterization of invasive group b streptococcus strains from the greater toronto area canada. J Clin Microbiol. 2014;52(5):1441–1447. doi: 10.1128/JCM.03554-13
- Sadeh M, Firouzi R, Derakhshandeh A, et al. Molecular characterization of streptococcus agalactiae isolates from pregnant and non-pregnant women at yazd university hospital, iran jundishapur. J Microbiol. 2016;9(2):e30412–e30412. doi: 10.5812/jjm.30412
- Rojo-Bezares B, AZCONA-GUTIérrez JM, MARTIN C, et al. Streptococcus agalactiae from pregnant women: antibiotic and heavy-metal resistance mechanisms and molecular typing. Epidemiol Infect. 2016;144(15):3205–3214. doi: 10.1017/S0950268816001692
- Shabayek S. Prevalence of group B streptococcus among pregnant and non-pregnant women in Ismailia. Egypt Records of Pharmaceutical and Biomedical Sciences. 2017;1(1):6–16.
- Elbaradie SM, Mahmoud M, Farid M. Maternal and neonatal screening for Group B streptococci by SCP B gene based PCR: a preliminary study. Indian J Med Microbiol. 2009;27(1):17–21. doi: 10.1016/S0255-0857(21)01746-1
- Mohammed MA, Daniel, W, Yimtubezinash, D. Prevalence of group B Streptococcus colonization among pregnant women attending antenatal clinic of Hawassa Health Center, Hawassa, Ethiopia. Ethiopian Journal of Health Development. 2012;26(1):36–42.
- Bassir A, Dhibou H, Farah M, et al. Portage vaginal du streptocoque du groupe B chez la femme enceinte au niveau de la région de Marrakech. Pan Afr Med J. 2016;23:23. DOI:10.11604/pamj.2016.23.107.9047
- Nkembe NMK, Baiye HG, Chafa WA, et al. Streptococcus agalactiae prevalence and antimicrobial susceptibility pattern in vaginal and anorectal swabs of pregnant women at a tertiary hospital in Cameroon. BMC Res Notes. 2018;11(1):480. doi: 10.1186/s13104-018-3589-x
- Dzowela T, Komolafe O, Igbigbi AJMMJ. Prevalence of group b streptococcus colonization in antenatal women at the queen elizabeth central hospital, blantyre–a preliminary study. Malawi Med J. 2005;17(3):97–99. doi: 10.4314/mmj.v17i3.10888
- Schuchat A, Wenger JD. Epidemiology of group B streptococcal disease. Risk factors, prevention strategies, and vaccine development. Epidemiol Rev. 1994;16(2):374–402. doi: 10.1093/oxfordjournals.epirev.a036159
- Akpaka PEH, Khamiya, T, Reinand, U, et al. Colonization of streptococcus agalactiae among pregnant patients in trinidad and Tobago. IJID Regions. 2022;3:96–100. doi: 10.1016/j.ijregi.2022.03.010
- Valkenburg-van den Berg AW, Sprij AJ, Oostvogel PM, et al. Prevalence of colonisation with group b streptococci in pregnant women of a multi-ethnic population in the Netherlands. Eur J Obstet Gynecol Reprod Biol. 2006;124(2):178–183. doi: 10.1016/j.ejogrb.2005.06.007
- Mubashir K, Faiz A, Ashshi A. Maternal colonization of group B streptococcus: Prevalence, associated factors and antimicrobial resistance. Ann Saudi Med. 2015;35(6):423–427. doi: 10.5144/0256-4947.2015.423
- Joachim A, Matee MI, Massawe FA, et al. Maternal and neonatal colonisation of group B streptococcus at muhimbili national hospital in dar es Salaam, Tanzania: prevalence, risk factors and antimicrobial resistance. BMC Public Health. 2009;9(1):437. doi: 10.1186/1471-2458-9-437
- Wang P, Tong J-J, Ma X-H, et al. Serotypes, antibiotic susceptibilities, and multi-locus sequence type profiles of Streptococcus agalactiae isolates circulating in Beijing, China. Plos One. 2015;10(3):e0120035. doi: 10.1371/journal.pone.0120035
- Yao K, Poulsen K, Maione D, et al. Capsular gene typing of Streptococcus agalactiae compared to serotyping by latex agglutination. J Clin Microbiol. 2013;51(2):503–507. doi: 10.1128/JCM.02417-12
- Suhaimi MES, Desa MNM, Eskandarian N, et al. Characterization of a group b streptococcus infection based on the demographics, serotypes, antimicrobial susceptibility and genotypes of selected isolates from sterile and non-sterile isolation sites in three major hospitals in Malaysia. J Infect Public Health. 2017;10(1):14–21. doi: 10.1016/j.jiph.2016.01.009
- Toniolo C, Balducci E, Romano MR, et al. Streptococcus agalactiae capsule polymer length and attachment is determined by the proteins CpsABCD. J Biol Chem. 2015;290(15):9521–9532. doi: 10.1074/jbc.M114.631499
- Li L, Wang R, Huang Y, et al. High incidence of pathogenic streptococcus agalactiae st485 strain in pregnant/puerperal women and isolation of hyper-virulent human cc67 strain. Front Microbiol. 2018;9:9. DOI:10.3389/fmicb.2018.00050
- Melo SC, SANTOS NCDS, OLIVEIRA MD, et al. ANTIMICROBIAL SUSCEPTIBILITY of Streptococcus agalactiae ISOLATED from PREGNANT WOMEN. Rev Inst Med Trop Sao Paulo. 2016;58 83. doi: 10.1590/S1678-9946201658083
- Mengist A, Kannan H, Abdissa A. Prevalence and antimicrobial susceptibility pattern of anorectal and vaginal group B streptococci isolates among pregnant women in Jimma, Ethiopia. BMC Res Notes. 2016;9(1):351. doi: 10.1186/s13104-016-2158-4
- Michael Ruess UMASRB. Antimicrobial susceptibility patterns of streptococcus agalactiae in a German University Hospital. Scand J Infect Dis. 2000;32(6):623–626. doi: 10.1080/003655400459522
- Jain B, Tewari A, Bhandari BB, et al. Antibiotic resistance and virulence genes in streptococcus agalactiae isolated from cases of bovine subclinical mastitis. Veterinary Archievs. 2012;82:423–432.
- Geng Y, Wang KY, Huang XL, et al. Streptococcus agalactiae, an emerging pathogen for cultured ya-fish, Schizothorax prenanti, in China. transbound emerg dis. Transbound Emerg Dis. 2012;59(4):369–375. doi: 10.1111/j.1865-1682.2011.01280.x
- Mohamed Sadaka S, Abdelsalam Aly H, Ahmed Meheissen M, et al. Group B streptococcal carriage, antimicrobial susceptibility, and virulence related genes among pregnant women in Alexandria, Egypt. Alexandria J Med. 2018;54(1):69–76. doi: 10.1016/j.ajme.2017.01.003
- Khan MA, Faiz A, Ashshi AMJAOSM. Maternal colonization of group B streptococcus: prevalence, associated factors and antimicrobial resistance. Ann Saudi Med. 2015;35(6):423–427. doi: 10.5144/0256-4947.2015.423
- Motlová J, Straková L, Urbásková P, et al. Vaginal & rectal carriage of streptococcus agalactiae in the czech republic: incidence, serotypes distribution & susceptibility to antibiotics. Indian J Med Res. 2004;119:84–87.
- Lambiase A, Agangi A, Del Pezzo M, et al. In vitro resistance to macrolides and clindamycin by group b streptococcus isolated from pregnant and nonpregnant women. Infect Dis Obstet Gynecol. 2012;2012:1–5. DOI:10.1155/2012/913603
- Emaneini M. High incidence of macrolide and tetracycline resistance among Streptococcus agalactiae strains isolated from clinical samples in Tehran, Iran. Maedica. 2014;9(2):157.
- Genovese C, D’Angeli F, Di Salvatore V, et al. Streptococcus agalactiae in pregnant women: serotype and antimicrobial susceptibility patterns over five years in Eastern Sicily (Italy). Eur J Clin Microbiol Infect Dis. 2020;39(12):2387–2396. doi: 10.1007/s10096-020-03992-8
- Khan F, Shukla I, Rizvi M, et al. Detection of biofilm formation in staphylococcus aureus. does it have a role in treatment of mrsa infections. Trends In Medical Research. 2011;6(2):116–123. doi: 10.3923/tmr.2011.116.123
- Cho KH, Caparon MG. Patterns of virulence gene expression differ between biofilm and tissue communities of Streptococcus pyogenes. Mol Microbiol. 2005;57(6):1545–1556. doi: 10.1111/j.1365-2958.2005.04786.x
- Kaczorek-Łukowska E, Małaczewska J, Wójcik R, et al. Biofilm production and other virulence factors in Streptococcus spp. isolated from clinical cases of bovine mastitis in Poland. Access Microbiology. 2019;1(1A):1. doi: 10.1099/acmi.ac2019.po0442
- Martins ER, Andreu A, Correia P, et al. Group B streptococci causing neonatal infections in barcelona are a stable clonal population: 18-year surveillance. J Clin Microbiol. 2011;49(8):2911–2918. doi: 10.1128/JCM.00271-11
- Sharma D, Misba L, Khan AU, et al. Antibiotics versus biofilm: an emerging battleground in microbial communities. Antimicrob Resist Infect Control. 2019;8(1):1–10. doi: 10.1186/s13756-019-0533-3
- Vestby LK, Grønseth T, Simm R, et al. Bacterial biofilm and its role in the pathogenesis of disease. Antibiotics. 2020;9(2):59. doi: 10.3390/antibiotics9020059
- Amin M, Navidifar T, Saleh Shooshtari F, et al. Association between biofilm formation, structure, and the expression levels of genes related to biofilm formation and biofilm-specific resistance of acinetobacter baumannii strains isolated from burn infection in Ahvaz, Iran. Infection And Drug Resistance 2019: p. 3867–3881. Volume 12 10.2147/IDR.S228981.
- Rosa-Fraile M, Dramsi S, Spellerberg B. Group B streptococcal haemolysin and pigment, a tale of twins. FEMS Microbiol Rev. 2014;38(5):932–946. doi: 10.1111/1574-6976.12071
- Oviedo P, Pegels E, Laczeski M, et al. Phenotypic and genotypic characterization of streptococcus agalactiae in pregnant women. first study in a province of Argentina. Braz J Microbiol. 2013;44(1):253–258. doi: 10.1590/S1517-83822013005000030
- Dutra VG, Alves VM, Olendzki AN, et al. Streptococcus agalactiae in Brazil: serotype distribution, virulence determinants and antimicrobial susceptibility. BMC Infect Dis. 2014;14(1):323. doi: 10.1186/1471-2334-14-323
- Udo EE, Boswihi SS, Al-Sweih N. Genotypes and virulence genes in group b streptococcus isolated in the Maternity Hospital, Kuwait. Med Princ Pract. 2013;22(5):453–457. doi: 10.1159/000349932
- Souza VC, Kegele FCO, Souza SR, et al. Antimicrobial susceptibility and genetic diversity of Streptococcus agalactiae recovered from newborns and pregnant women in Brazil. Scand J Infect Dis. 2013;45(10):780–785. doi: 10.3109/00365548.2013.810814
- Shabayek S, Spellerberg B. Group bstreptococcal colonization, molecular characteristics, and epidemiology. Front Microbiol. 2018;9:9. doi: 10.3389/fmicb.2018.00437
- Dore N, BENNETT D, KALISZER M, et al. Molecular epidemiology of group B streptococci in Ireland: associations between serotype, invasive status and presence of genes encoding putative virulence factors. Epidemiol Infect. 2003;131(2):823–833. doi: 10.1017/S0950268803008847
- Manning SD, Ki M, Marrs CF, et al. The frequency of genes encoding three putative group B streptococcal virulence factors among invasive and colonizing isolates. BMC Infect Dis. 2006;6(1):116. doi: 10.1186/1471-2334-6-116