ABSTRACT
The common housefly (Musca domestica) is widely recognized as a significant pest and can transmit many pathogens. The habitual use of chemical insecticides against housefly has led to detrimental effects on humans, non-target species, and environment. Therefore, there is an urgent demand for new, sustainable alternatives of housefly control. This research investigates the effects of different extracts from common pear (Pyrus communis) leaves on M. domestica larvae, and lifecycle progression. Musca domestica was identified through DNA barcoding, and a sequernce for the COX1 gene was submitted to NCBI gene bank, with accession number of OQ363313. Results showed that extracts from P. communis leaves were lethal to the larvae at 650 and 150 ppm, respectively, with the n-hexane extract proving to be more efficacious. Also, tested extracts prolonged developmental stages of both larvae and pupae. Females emerged from treated larvae laid fewer eggs. A molecular docking was performed to examine the interactions between extract’s molecules and a crucial protein in M. domestica, Ls-AChBP (PDB ID: 2zju). Five compounds were identified as particularly effective: Tetratetracontane, Octacosyl acetate, Hexatriacontane, Eicosyl heptafluorobutyrate, and Bis (2-ethylhexyl) phthalate. The results indicate that these pear leaf extracts hold promise as alternative agents for managing M. domestica populations.
Introduction
Today, vector-borne diseases have increased worldwide via infection by insects, particularly houseflies [Citation1]. Musca domestica, a member of the family Muscidae, is a major mechanical vector (which carries the pathogen from one host to another, but the pathogen doesn’t replicate or develop inside the vector) for over a hundred different kinds of pathogens; it also annoys people and ruins food [Citation2]. They cause a wide variety of diseases, including those caused by bacteria, protozoa, helminths, and viruses. A dangerous strain of Escherichia coli was spread across Japan with the assistance of houseflies. They may spread cholera and typhoid in some areas of the globe [Citation3]. For many years, M. domestica was effectively controlled with chemical insecticides; however, continuous usage of commercial insecticides resulted in many severe issues, such as actual hazards to non-target organisms, the environment and developing resistance in houseflies to these insecticides, which is reported abundantly [Citation4–8]. Consequently, we urgently need to enhance eco-friendly and cheap novel alternatives for controlling houseflies to stay away from potentially harmful chemical pesticides [Citation9,Citation10]. On the contrary, several botanical extracts with their different phytoconstituents can act as conceivable alternatives [Citation11,Citation12].
Pyrus communis, commonly known as the European pear, has demonstrated promising insecticidal properties, making it an attractive candidate for developing bio-rational pest management approaches. Studies reveal that extracts and essential oils from various parts of P. communis, including the leaves, fruits, seeds, and stem bark, exhibit toxicity against a range of insect pests [Citation13–17]. The potent activity has been attributed to compounds such as flavonoids, tannins, terpenoids and polyphenols present in the plant [Citation14–17]. Specifically, the leaf and seed extracts containing flavonoids like quercetin have shown larvicidal effects against disease-vectoring mosquitoes of public health significance like Culex and Aedes species; also, fruit extracts have revealed toxicity against malaria and filariasis vectors. Fruit essential oils possess insecticidal activity against stored product pests attacking grains. Stem bark extracts containing polyphenols have been found effective against major lepidopteran pests. The extracts likely act through antifeedant effects, growth inhibition, reduced survival, and fecundity impairment [Citation18–25].
Although P. communis leaves extracts proved their activity counteracting the malarial parasite, Anopheles pharoensis [Citation13], This study aimed to evaluate the larvicidal efficacy of methanol and n-hexane extracts from P. communis leaves against the mechanical vector, M. domestica. Additionally, the research assessed the impact of these extracts on the reproductive capabilities of the female vectors that emerged post-treatment.
Materials and methods
Culturing of a housefly, Musca domestica
A stock of houseflies’ adult was collected by hunting from Cairo Governorate, Egypt (30°02’20’‘N, 31°16’28’’ E) using traps baited with wheat bran (200 g), milk (100 g), and powder yeast (5 g) mixed with 200 mL distilled water. Collected adults had been identified using DNA barcoding techniques targeting partial sequences of the COX1 Mitochondrial gene. The target species has been reared for several generations using standard specific metal frame cages (30 × 30 × 30 cm) blanketed with muslin on every side. A standard rearing procedure was adopted to provide 3rd instar larvae needed for the assay [Citation9] but with minor modifications. Wheat bran, milk, and powdered yeast (in a ratio of 200:100:5 g) were combined with 200 mL of distilled water to create an artificial diet for the larvae [Citation26].
Tested materials
Pyrus communis extract preparation
A private garden in Sadat City, Cairo-Alexandria desert route (30°21’38.7’ N, 30°29’58.3’ E, height 42 m), Menoufiya Governorate, Egypt, provided the fresh P. communis leaves which were used in this study, following the scientific ways of cutting and collecting the plant leaves without harming the tree itself. All plant material used in our experiments and field investigations on P. communis was collected legally and ethically in compliance with local, state, and federal regulations. We got the plant and verified its legitimacy. The leaves were rinsed in distilled water and air-dried at room temperature for 15°C under the cover of trees. Dried leaves were cut to a suitable size using an electrical blender (Philips, HR2058). The powdered leaves were extracted using methanol and n-hexane separately following a previously described standard procedure of extraction [Citation13]. Briefly, 100 g of leaf powder was extracted five times with 300 ml of methanol and n-hexane at room temperature. After 24 h, the supernatants were decanted, filtered through the Whatman filter and dried in a rotary evaporator at 40°C for 2 h. The dry extracts were kept in freezer (−4°C) until used for experiments.
Flufenoxuron
A positive control agent, flufenoxuron (Cascade®; 10% EC), was acquired in Cairo, Egypt, from Sidasa Com. for use in growing crops or combating pests.
Bioassay
Larvicidal activity
Different concentrations of each tested extract were utilized in 250 mL of distilled water. Methanol extract received 0.1 mL of methanol, while Two drops of Tween80 were added to the n-hexane extract to facilitate the dissolving of tested extract in water. An artificial larval diet was mixed with different concentrations of tested extracts or flufenoxuron instead of distilled water [Citation27]. Three replicates were used for each tested concentration (25 3rd instar larvae/replicate). Control was carried out using distilled water with 0.1 mL methanol or 2 drops of Tween80. All cups were incubated under controlled conditions at a temperature of 27 ± 2°C, relative humidity of 70–75% and 12–12 light-dark regime. Mortality was recorded daily; dead larvae and pupae were removed until adult emergence.
Reproductive potential activity
Five females which succeeded in emerging from 3rd instar larvae treated with lethal concentrations of tested extracts and flufenoxuron and transferred to a wooden cage with normal males (collected from the culture). The isolated adults were fed milk powder and a sucrose solution of 10% for 3 days. Females might lay eggs fed a synthetic diet. Eggs laid were counted, and the mean values were calculated [Citation28].
Molecular docking study
The binding mechanism and interactions between the most active complexes and the proteins 2zju were studied using molecular docking technique using MOE 2015 software. The results were compared to the 53 most mentioned bioactive molecules detected by GC-Mass. Crystal structures of Ls-AChBP were obtained from the protein data bank (http://www.rcsb.org.pdb) (PDB ID: 2zju). The most potent complexes’ 3D structures were built using Chem draws 18.0 and then exported as MDL mol files. The greatest score went to the molecule with the lowest binding affinity value.
Statistical analysis
SPSS V.22 was used for data encoding and entry. The normality and homogeneity of the data was examined using the Shapiro-Wilk and Kolmogorov-Smirnov tests, and the assumptions of parametric tests were verified. Chi-square x2 for comparing the observed and predicted frequencies of the outcomes of desired variables using MiniTab V. 14. Data were reported as mean, with standard deviation and P-value deemed significant at 0.05. With R-studio 4.1.3, data may be visualized.
Results
Biological activity of the extracts
As shown in , the methanol and n-hexane extracts from the leaves of P. communis recorded complete larval mortality (100.0%) in the highest dosage of 650 and 150 ppm for methanol and n-hexane extracts, respectively; meanwhile, the lowest larval mortality recorded at 24.0 and 20.0% respectively for concentration low as 400 and 100 ppm, respectively. At the same time, positive control (flufenoxuron) recorded 100.0 and 20.0% mortality in M. domestica 3rd instar larvae at 1.4 and 4.0 ppm, respectively. On the other hand, pupal mortality was 44.44 and 22.22% by methanol extract at 600 and 550 ppm, while n-hexane extract recorded pupal mortality of 55.56 and 27.38% at 140 and 130 ppm, respectively, compared with 56.67 and 31.11% recorded for flufenoxuron at 1.4 and 1.2 ppm.
Figure 1. The bubble chart represents a. larval mortality; b. pupal mortality, and c. adult emergency.
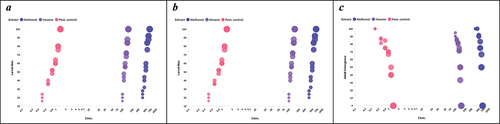
Larval mortality rates were also significantly different (P < 0.05) between the positive control, methanol, and n-hexane. In contrast, n-hexane showed no significant difference from the positive control (P > 0.05). Additionally, pupal mortality and adult emergency responses were significantly different in the methanol group vs the positive control and n-hexane groups (P < 0.05) ().
Based on calculated LC values in , it was obvious that n-hexane extract from leaves of P. communis was more effective against M. domestica 3rd instar larval than methanol extract.
Table 1. Lethal concentrations of methanol and n-hexane extracts from leaves of P. communis against M. domestica larvae.
Also, there was a clear difference (P < 0.05) between treated groups in their calculated larval time, pupal time, and total development times. The larval time recorded was 5.59 ± 0.16, 5.11 ± 0.08 at 600 and 140 ppm for methanol and n-hexane extracts, compared with 3.22 ± 0.12 recorded by flufenoxuron at 1.2 ppm ().
Figure 2. Box-Plot chart representing larval time, pupal time, and total development times for treated groups.
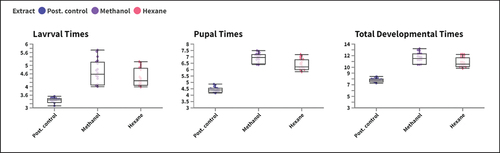
Regarding the M. domestica growth index, a reverse trend is represented in all treated and control groups, as concentration increases as the growth index follows a declining trend. On the same line, a statistically significant variation (P < 0.05) was presented between all treated groups in their observed growth index values ().
Figure 3. The fitted line chart directly compares the treated groups’ growth index and concentration.
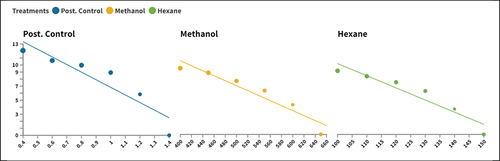
The fertility of females arising from treated larvae was significantly reduced by P. communis extracts evaluated in methanol and n-hexane, notably at the highest concentration levels. The number of eggs deposited by females after feeding varied significantly (P > 0.05) in comparison to the positive control. For both extracts, higher LC levels were associated with fewer eggs laid ().
Figure 4. The bar chart represents the number of eggs laid in relation to the calculated LC values (ppm) for all treated groups.
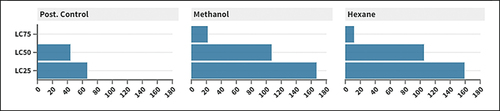
Both extracts showed a significant rise in the number of female eggs that failed to hatch after developing into treated larvae (). Also, there was a significant variation (P < 0.01) between hatched and non-hatched eggs for all treated groups at LC25, LC50, and LC75 ().
Figure 5. Pie chart representing hatched to non-hatched ratios for all treated groups at LC25, 50, and 75.
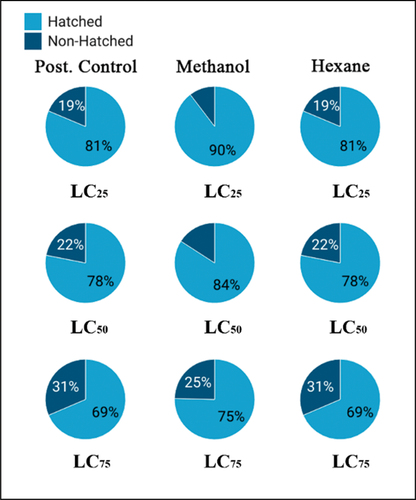
Table 2. Chi-square of hatched and non-hatched eggs laid by M. domestica females emerged from larvae treated with lethal concentrations of tested extracts.
Docking on the receptor of Ls-AChBP (PDB ID: 2zju)
The molecular docking was conducted on the 53 molecules reported in the GC-MS profile, which were investigated by applying the target molecules against Ls-AChBP (PDB ID: 2zju) through MOE 2015 apps. The five highest energy score molecules out of the fifty-three compounds () were determined to be −7.8314, −7.3263, −7.282, −7.2266 and −6.9511 kcal/mol for the tetratetracontane, octacosyl acetate, hexatriacontane, eicosyl heptafluorobutyrate, bis(2-ethylhexyl) phthalate complexes, respectively, as shown in . The stronger the contact, the greater the negative binding energy. So, the interaction followed the order of tetratetracontane > octacosyl acetate > hexatriacontane > eicosyl heptafluorobutyrate > bis(2-ethylhexyl) phthalate complexes. These outcomes are in line with insecticidal activity experiments. , against the best-scoring docked molecules, shows how the amino acid residues in 2zju form hydrogen bonds, polar contacts, and hydrophobic interactions.
Figure 6. The line chart represents the score of 53 molecules docked against Ls-AChBP (PDB ID: 2zju).
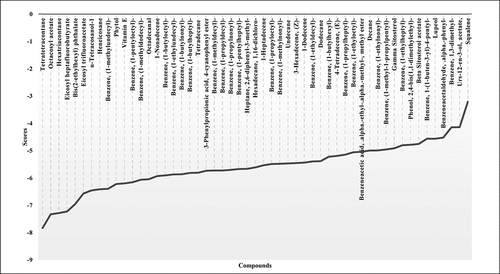
Figure 7. 2D & 3D interaction of tetratetracontane, octacosyl acetate, hexatriacontane, eicosyl heptafluorobutyrate, bis (2-ethylhexyl) phthalate complexes against the active site of Ls-AChBP (PDB ID: 2zju). Hydrogen bonds are displayed in cyan & H-pi-bonds in dark magenta.
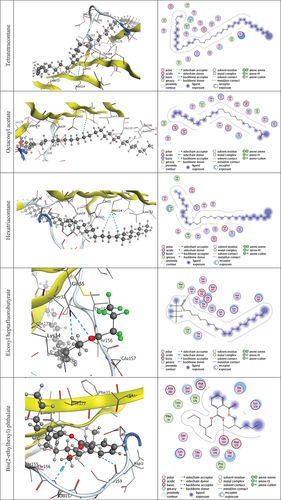
Table 3. Docking results inside Ls-AChBP (PDB ID: 2zju) active spots, arranged from the negatively highest to the lowest score.
Discussion
The findings from the current study emphasize the substantial larvicidal properties of n-hexane and methanol extracts derived from Pyrus communis leaves against the housefly Musca domestica. Interestingly, the efficacy of these extracts is influenced by both the type of extraction solvent and the concentration of the extract. When compared based on lethal concentrations (LC), the n-hexane extract demonstrated superior effectiveness against the larvae than its methanol counterpart. This observation mirrors findings from other studies: for instance, ethanol extracts from Calotropis procera seeds induced 100.0% larval mortality at a concentration of 500 ppm against the M. domestica 3rd larval instar [Citation29]. Similarly, n-hexane extracts from Jatropha curcas leaves indicated a promising LC50 value of 0.27 mg/cm2 against M. domestica larvae [Citation30]. An Ocimum basilicum crude extract also presented an LC50 value of 110 ppm against the same larvae [Citation31]. Such comparative data underscores the importance of solvent selection when assessing plant-based larvicidal activities.
Beyond mortality rates, the behavior and development of the M. domestica larvae were also affected by the studied extracts. Specifically, there was a significant prolongation in the larval and pupal development times (P < 0.05). This phenomenon aligns with previous observations made with P. harmala extract at a concentration of 25% [Citation32]. Other studies have documented similar effects on larval duration upon treatment with extracts from Allium sativum, Piper nigrum, and Moringa oleifera [Citation2].
The reproductive capabilities of female M. domestica arising from treated larvae also came under the influence of the tested extracts. We observed a noticeable reduction in both fertility and fecundity. Such reductions can be attributed to multiple factors: the physical state of the treated females, inhibition exerted by the extracts on factors crucial for mating, disruption in the ovarian activity, which can result in irreversible sterility, and a slowing down or halt of ova production, leading to the re-absorption of eggs within the ovaries. These factors might result from a diminished metabolic rate instigated by the extracts’ presence [Citation33–36]. Our observations resonate with earlier studies that demonstrated similar declines in fecundity and fertility after treatment with various plant extracts and powders [Citation2,Citation34,Citation35].
The final segment of our study journeyed into the molecular realm. Molecular docking allowed us to dissect the bioactivity of the constituents present in the extract and to ascertain the potential reasons behind the recorded larvicidal activity. Within the GC-MS profile, a total of 53 compounds were identified. Their potential interactions with the Ls-AChBP protein (PDB ID: 2zju) were assessed through molecular docking. Among these, the tetratetracontane, octacosyl acetate, hexatriacontane, eicosyl heptafluorobutyrate, and bis (2-ethylhexyl) phthalate complexes recorded the highest energy scores. This signifies their dominant role in the extract’s bioactivity.
In conclusion, our research builds a compelling case for the larvicidal properties of Pyrus communis extracts against M. domestica, setting the stage for further exploration and potential commercial application.
Conclusion
In our recent research, we delved into the potential of Pyrus communis extracts as sustainable alternatives to traditional chemical insecticides, with a particular focus on managing the housefly Musca domestica. Our study has revealed that these extracts, especially when derived using methanol and n-hexane as solvents, offer a promising eco-friendly approach to pest control in an age where environmental conservation is paramount. Interestingly, among the two tested extracts, the n-hexane variant showcased superior efficacy in targeting housefly isolates, pointing researchers and industries in a clear direction for harnessing the insecticidal properties of Pyrus communis. Further reinforcing our findings, molecular docking studies provided invaluable insights into the molecular interactions underpinning the insecticidal properties, ensuring a deeper understanding of the extracts’ mechanism of action. Beyond the immediate scientific implications, our findings have a broader commercial potential. Given the ubiquity of pear trees cultivated primarily for fruit, their leaves could be an overlooked resource. These leaves can be processed to produce the extracts of our study, which were found to be potent. With the right development, such extracts can pave the way for commercial natural insecticides, offering society a twofold benefit: a natural deterrent against pests like M. domestica and, consequently, a reduction in the diseases they spread.
Disclosure statement
No potential conflict of interest was reported by the author(s).
References
- Semiatizki A, Weiss B, Bagim S, et al. Effects, interactions, and localization of Rickettsia and Wolbachia in the house fly parasitoid, spalangia endius. Microb Ecol. 2020;80(3):718–728. doi: 10.1007/s00248-020-01520-x
- Nisar MS, Ismail MA, Ramzan H, et al. The impact of different plant extracts on biological parameters of housefly [Musca domestica (Diptera: muscidae)]: implications for management. Saudi J Biol Sci. 2021;28(7):3880–3885. doi: 10.1016/j.sjbs.2021.03.070
- Morey RA, Khandagle AJ. Bioefficacy of essential oils of medicinal plants against housefly, Musca domestica L. Parasitol Res. 2012;111(4):1799–1805. doi: 10.1007/s00436-012-3027-2
- Scott JG, Alefantis TG, Kaufman PE, et al. Insecticide resistance in house flies from caged-layer poultry facilities. Pest Manag Sci. 2000;56(2):147–153. doi: 10.1002/(SICI)1526-4998(200002)56:2<147:AID-PS106>3.0.CO;2-7
- Kaufman PE, Scott JG, Rutz DA. Monitoring insecticide resistance in house flies (Diptera: muscidae) from New York dairies. Pest Manag Sci. 2001;57(6):514–521. doi: 10.1002/ps.319
- Shono T, Zhang L, Scott JG. Indoxacarb resistance in the house fly, Musca domestica. Pestic Biochem Physiol. 2004;80(2):106–112. doi: 10.1016/j.pestbp.2004.06.004
- Jesikha M. Mixed flower waste extract as larvicides against Musca Domestica. Sci Rep. 2012;1:558. doi: 10.4172/scientificreports.558
- Elhawary EA, Mostafa NM, Shehata AZI, et al. Comparative study of selected Rosa varieties’ metabolites through UPLC-ESI-MS/MS, chemometrics and investigation of their insecticidal activity against Culex pipiens L. Jordan J Pharm Sci. 2021;14:417–443. https://journals.ju.edu.jo/JJPS/article/view/107758
- Bisseleua HBD, Gbewonyo SWK, Obeng-Ofori D. Toxicity, growth regulatory and repellent activities of medicinal plant extracts on Musca domestica L. (Diptera: muscidae). Afr J Biot. 2008;7:4635–4642. doi: 10.5897/AJB08.573
- Pavela R. Insecticidal properties of several essential oils on the house fly (Musca domestica L.). Phyther Res. 2008;22(2):274–278. doi: 10.1002/ptr.2300
- Kestenholz C, Stevenson PC, Belmain SR. Comparative study of field and laboratory evaluations of the ethnobotanical Cassia sophera L. (Leguminosae) for bioactivity against the storage pests Callosobruchus maculatus (F.) (Coleoptera: Bruchidae) and Sitophilus oryzae (L.) (Coleoptera: Curculionidae). J Stored Prod Res. 2007;43:79–86. doi: 10.1016/j.jspr.2005.11.003
- de Oliveira APS, Agra-Neto AC, Pontual EV, et al. Evaluation of the insecticidal activity of moringa oleifera seed extract and lectin (WSMoL) against sitophilus zeamais. J Stored Prod Res. 2020;87:101615. doi: 10.1016/j.jspr.2020.101615
- Shehata A, Labib RM, Abdel-Samad MRK. Insecticidal activity and phytochemical analysis of Pyrus communis L. extracts against malarial vector, Anopheles pharoensis Theobald, 1901 (Diptera: Culicidae). Polish J Entomol. 2021;90:209–222. doi: 10.5604/01.3001.0015.6329
- Vivekanandhan P, Venkatesan R, Ramkumar G, et al. Comparative analysis of major mosquito vectors response to seed-derived essential oil and seed pod-derived extract from acacia nilotica. Int J Environ Res Public Health. 2018;15(2):388.. doi: 10.3390/2Fijerph15020388
- Vivekanandhan P, Senthil-Nathan S, Shivakumar MS. Larvicidal, pupicidal and adult smoke toxic effects of Acanthospermum hispidum (DC) leaf crude extracts against mosquito vectors. Physiol Mol Plant Pathol. 2018;101:156–162. doi: 10.1016/j.pmpp.2017.05.005
- Vivekanandhan P, Usha-Raja-Nanthini A, Valli G, et al. Comparative efficacy of Eucalyptus globulus (labill) hydrodistilled essential oil and temephos as mosquito larvicide. Nat Prod Res. 2020;34(18):2626–2629. doi: 10.1080/14786419.2018.1547290
- Pratheeba T, Vivekanandhan P, Faeza AKN, et al. Chemical constituents and larvicidal efficacy of Naringi crenulata (Rutaceae) plant extracts and bioassay guided fractions against Culex quinquefasciatus mosquito (Diptera: Culicidae). Biocatal Agric Biotechnol. 2019;19:101137. doi: 10.1016/j.bcab.2019.101137
- Hussain SZ, Naseer B, Qadri T, et al. Pear (Pyrus Communis)-morphology, taxonomy, composition and health benefits. In: Fruits grown in Highland regions of the Himalayas: nutritional and health benefits. Bhat, TA, editor. Cham: Springer International Publishing; 2021. pp. 35–48.
- Ibrahim RM, Hammoudi ZM. Phytochemistry and pharmacological activity of pear (Pyrus communis Linn): a review. Plant Arch. 2020;20(2):7820–7828.
- Salta J, Martins A, Santos RG, et al. Phenolic composition and antioxidant activity of rocha pear and other pear cultivars – a comparative study. J Funct Foods. 2010;2(2):153–157. doi: 10.1016/2Fj.jff.2010.02.002
- Li X, Wang T, Zhou B, et al. Chemical composition and antioxidant and anti-inflammatory potential of peels and flesh from 10 different pear varieties (Pyrus spp.). Food Chem. 2014;152:531–538. doi: 10.1016/j.foodchem.2013.12.010
- Sarkar D, Ankolekar C, Pinto M, et al. Dietary functional benefits of Bartlett and starkrimson pears for potential management of hyperglycemia, hypertension and ulcer bacteria helicobacter pylori while supporting beneficial probiotic bacterial response. Food Res Int. 2015;69:80–90. doi: 10.1016/j.foodres.2014.12.014
- Jiang G, Yim S, Eun J. Physicochemical characteristics and antioxidant activities of new Asian pear cultivars. J Appl Biol Chem. 2016;59(4):337–343. doi: 10.3839/jabc.2016.057
- Clementino M, Shi X, Zhang Z. Prevention of polyphenols against carcinogenesis induced by environmental carcinogens. J Environ Pathol Toxicol Oncol. 2017;36(1):87–98. doi: 10.1615/JEnvironPatholToxicolOncol.2017019057
- Hong SY, Lansky E, Kang SS, et al. A review of pears (Pyrus spp.), ancient functional food for modern times. BMC Complement Med Ther. 2021;21(1):219. doi: 10.1186/s12906-021-03392-1
- Ahmad M, Ghaffar A, Rafiq M. Host plants of leaf worm, Spodoptera litura (Fabricius) (Lepidoptera: Noctuidae) in Pakistan. Asian J Agric Biol. 2013;1:23–28.
- Hasaballah AI, Shehata AZ, Fouda MA, et al. The biological activity of Cupressus sempervirens extracts against Musca domestica. Asian J Biol. 2018;5(1):1–12. doi: 10.9734/AJOB/2018/38023
- Hassan MI, Shehata AZI, Gabarty A, et al. Effect of gamma radiation on some biological aspects and ultrastructure of female ovaries of the house fly, Musca domestica L. (Diptera: muscidae). J Radiat Res Appl Sci. 2019;12(1):343–351. doi: 10.1080/16878507.2019.1654658
- Begum N, Sharma B, Pandey RS. Toxicity potential and anti- activity of some plant extracts on Musca domestica. J Biofer Biopesti. 2011;2:1–6.
- Chauhan N, Kumar P, Mishra S, et al. Insecticidal activity of jatropha curcas extracts against housefly, Musca domestica. Environ Sci Pollut Res. 2015;22(19):14793–14800. doi: https://doi.org/10.1007/s11356-015-4686-1
- Morey RA Evaluation of crude extracts of Citrus limon And Ocimum Basilicum against Musca Domestica L. 2016. Available from: http://rutpp.com,1-4
- Attaullah ZM, Zahoor MA, Zahoor MA, et al. Insecticidal, biological and biochemical response of Musca domestica (Diptera: Muscidae) to some indigenous weed plant extracts. Saudi J Biol Sci. 2020;27(1):106–116. doi: 10.1016/j.sjbs.2019.05.009
- Tripathi AK, Prajapati V, Khanuja SPS, et al. Effect of d-limonene on three stored-product beetles. J Econ Entomol. 2003;96:990–995. doi: 10.1603/0022-0493-96.3.990
- Shaapan RM, Shehata AZ. Efficacy of Trigonella stellata (Fabaceae) and eucalyptus citriodora (Verbenaceae) extracts against the housefly , Musca domestica (Diptera: muscidae). J Entomol. 2019;16(1):1–8. doi: 10.3923/je.2019.1.8
- Elkattan NA, Ahmed KS, Elbermawy SM, et al. Effect of some botanical materials on certain biological aspects of the house fly, Musca domestica L. Egypt J Hosp Med. 2011;42(1):33–48.. doi: 10.21608/ejhm.2011.16794
- Lucantoni L, Giusti F, Cristofaro M, et al. Effects of a neem extract on blood feeding, oviposition and oocyte ultrastructure in anopheles stephensi Liston (Diptera: Culicidae). Tissue Cell. 2006;38(6):361–371. doi: 10.1016/j.tice.2006.08.005