ABSTRACT
Colorectal cancer (CRC) is a prevalent cancer worldwide, and its incidence has increased in recent years. 1,2-Dimethylhydrazine (DMH) is a carcinogenic alkylating agent that causes tumors in various organs, particularly the distal colon. Niclosamide (Nic) disrupts oxidative phosphorylation and lowers ATP levels in tumor cells. This study aimed to investigate the potential protective effects of Nic against DMH-induced CRC in male albino mice through histopathological, immunohistochemical, and biochemical analyses. The study involved subcutaneous injections of DMH at a dose of 15 μg/g administered twice weekly for six weeks, alone or in combination with Nic injections of 20 mg/kg twice weekly for six weeks. The colorectal paraffin slides were subjected to histopathology and apoptosis-inducing factor (AIF) immunostaining, and colorectal homogenate samples were used to measure the lipid peroxidation products malondialdehyde (MDA) and 8-hydroxy-2′-deoxyguanosine (8-OHdG).The results indicated that Nic injections reduced collagen deposition and AIF staining intensity in paraffin slides and decreased MDA content and 8-OHdG levels in tissue homogenates.These findings suggest that Nic injections may have an anti-CRC effect.
Introduction
Colorectal cancer (CRC) is a complex disease characterized by developing malignant tumors in the inner walls of the colon and rectum that resemble polyps [Citation1]. It is the third most common cancer worldwide and the second leading cause of cancer-related mortality [Citation2]. Modifiable risk factors for CRC include excess body weight, cigarette smoking, excessive alcohol consumption, high red or processed meat intake, and physical inactivity [Citation3]. Numerous studies have suggested that CRC survival rates are closely related to the diagnosis stage, with later-stage diagnoses resulting in worse survival rates [Citation4].
Over time, CRC progresses to malignancy as polyps grow and trigger metastasis to various organs, such as the liver, lungs, bones, brain, and spinal cord [Citation5]. Inflammation in the colon (colitis) is a crucial driver of oncogenic alterations that promote tumor growth by producing reactive oxygen species (ROS) [Citation6]. Dimethylhydrazine (DMH) induction causes an expansion of the proliferative zone in colonic crypts, increasing the number of marked cells in the crypts [Citation7].
DMH metabolism results in the formation of azoxymethane (AOM) and methylazoxymethanol (MAM), which migrate to the colon tissue through the bloodstream or bile to produce their ultimate carcinogenic metabolite (diazonium ion). This causes oxidative stress by methylating biomolecules in colonic epithelial cells, resulting in promutagenic events that promote tumor growth and inflammation [Citation8].
Colonic tumors can be induced by various doses of DMH, ranging from 2 to 200 mg/kg (single injection to 30 injections), and latency periods from 8 h to 78 weeks, which can produce CRC regardless of the mode of administration [Citation7]. Oxidative stress influences cancer formation by causing cancer cells to become resistant to anti-proliferative signals or apoptosis, anchorage-independent cell proliferation, and changes in cancer cell invasion and migration via epigenetic and metabolic pathways. Moreover, ROS contributes to tumor angiogenesis by releasing vascular endothelial growth factor (VEGF) and angiopoietin-1 [Citation9]. Elevated oxidative stress is particularly prevalent in several types of malignancies, as manifested by higher levels of the oxidized DNA base 8-hydroxy-2′-deoxyguanosine (8-OHdG) and the lipid peroxidation (LPO) product malondialdehyde (MDA) [Citation10,Citation11].
The inflammatory response is critical for tumor growth, and inflammation and oxidative stress contribute to CRC progression [Citation12,Citation13]. Evaluation of the changing patterns of tumor growth is essential for the development of anticancer treatments.
Niclosamide (Nic) is a drug listed on the World Health Organization’s Model List of Essential Medicines and has been used since the 1960s to treat tapeworm infections [Citation14]. Its mode of action is related to the uncoupling of oxidative phosphorylation in the mitochondria, which disrupts metabolism in these organisms [Citation15]. Recently, this molecule has been associated with new biological activities relevant to cancer, making it one of the most intriguing targets for repositioning in new therapeutic applications [Citation16]. Mounting evidence shows that Nic is a multifunctional drug that modulates various signaling pathways and biological processes [Citation17]. Given the Nic’s ability to disrupt many signaling pathways that regulate cancer initiation and development, it is not surprising that it can induce cell cycle arrest, growth inhibition, and apoptotic death in various cancer types [Citation18].
Aim of the work
This study aimed to investigate the potential protective effect of Nic against DMH-induced CRC in male albino mice through histopathological, immunohistochemical, and biochemical analyses.
Materials
Chemicals
Niclosamide, dimethylhydrazine, EDTA, DMSO, and Cremophor EL were purchased from Sigma-Aldrich (United States of America). Isoflurane, commercially known as Anahal 100% inhalation solution, was obtained from the Pharco Pharmaceuticals Company (Egypt).
Experimental animals
Fifty-four male Swiss albino mice weighing 20 ± 5 g were obtained from the Laboratory Animal Unit, Medical Technology Center, Medical Research Institute (MRI), Alexandria University. They were housed at 20–25°C, subjected to a 12-hour light-dark cycle, and provided free access to standard food and water.
Experimental design
After one week of acclimatization, fifty-four mice were randomly assigned to six groups, with nine mice in each group (n = 9/group). The groups were as follows:
Control group: Mice were given a well-balanced diet and had ad libitum access to water.
EDTA group: mice were subcutaneously injected with 0.2 ml of 0.001 M EDTA.
DMSO group: mice were intraperitoneally injected with 0.1 ml of DMSO.
Nic group: Mice were intraperitoneally injected with Nic at 20 mg/kg [Citation19].
DMH group: Mice were subcutaneously injected with DMH at 15 μg/g [Citation20].
Nic-DMH group: Mice were injected with Nic as described in the Nic group and then, one hour later, injected with DMH at a dosage of 15 μg/g.
All treatments were administered twice a week for six weeks. At four- and six-weeks post-treatment, the mice were sacrificed under isoflurane anesthesia, and the colorectal area was excised. The excised tissue was washed with a saline solution (0.9%) and divided into two parts. One part was fixed in 10% neutral-buffered formalin for 72 h and used for histopathological and immunohistochemical studies. The other part was weighed and stored at − 80°C for biochemical studies.
Ethical statement
All experiments described in this study adhered to the standards outlined in the Guide for the Care and Use of Laboratory Animals of the National Institutes of Health (NIH, Bethesda, MD, USA, Publication No. 8023, revised 1978). This research was conducted with the approval of Alexandria University’s Institutional Animal Care and Use Committee (IACUC), with approval number AU0122051011.
To minimize animal stress, every attempt was made to follow the 3Rs guidelines (replacement, reduction, and refinement) throughout the experiments. The study also complied with the ARRIVE criteria and the National Research Council’s recommendations for treating and using laboratory animals.
Methods
Histopathological and immunohistochemical investigations
Sample preparation
The first part of the excised colorectal tissue was fixed in 10% neutral buffered formalin, dehydrated in an ascending series of ethyl alcohol solutions, and cleared with xylene. Subsequently, the tissue was infiltrated using molten wax in an oven. The samples were then blocked in wax using a suitable mold to prepare them for microtomy. Finally, 5 µm sections were cut and placed on normal glass slides for histopathological investigation and on positively charged glass slides for immunohistochemistry.
Hematoxylin and Eosin (H&E)
Tissue slides were stained with hematoxylin and eosin (H&E) and microscopically examined to observe any changes in the glandular crypts present in the mucosal layer. The slides were deparaffinized in xylene, rehydrated in descending alcohol grades, and immersed in distilled water. They were then stained with Mayer’s hematoxylin, counterstained with eosin, dehydrated in ascending grades of alcohol, and mounted with DPX.
Masson’s trichrome
Tissue slides were stained using Masson’s trichrome and microscopically examined to determine the presence of increased collagen fibers in diseased tissues. Deparaffinized sections were first processed in distilled water and then immersed in Bouin’s fixative. They were stained with Weigert’s hematoxylin, followed by Biebrich scarlet acid fuchsin, and differentiated in phosphomolybdic-phosphotungstic acid solutions. Finally, the sections were stained with Fast Green and processed for microscopic examination.
Immunohistochemical investigations
The expression of apoptosis-inducing factor (AIF) in fixed sections of colon tissue was detected using an indirect immunoperoxidase staining technique. The AIF primary antibody (‘NBP2–27177’, Novus Biologicals, United States) was used along with the Anti-Mouse/Rabbit Ultra Vision LP detection system with horseradish peroxidase (HRP) polymer and 3,3′-diaminobenzidine (DAB) plus chromogen (secondary antibody kit) (Thermo Fisher Scientific, United States) according to the manufacturer’s instructions.
The tissue slides were first placed in retrieval sodium citrate buffer, followed by incubation in a hydrogen peroxide block and washing in PBS. The primary antibody was then applied at a dilution of 1:150, and the slides were treated with HRP. After washing with PBS, DAB was applied to the sections.
Image analysis
Digital images of AIF immunoassayed sections were captured using a PC-driven digital camera (TOUPCAM, LCMOS05100KPA) mounted on a light microscope. A semi-quantitative scoring system was used to analyze the images using the ImageJ software (version 1.53k). Briefly, the stained sections were viewed under a microscope, and their glandular crypts were identified and captured. The images were then analyzed for AIF expression intensity, representing the percentage area of brown staining in the mucosal layer.
Biochemical parameters
Sample preparation
The second part of the colorectal tissue was divided into two parts for the assessment of malondialdehyde (MDA) and 8-hydroxy-2′-deoxyguanosine (8-OHdG) levels using a sandwich enzyme-linked immunosorbent assay (ELISA). Tissue samples were rinsed and homogenized in phosphate-buffered saline at a weight/volume ratio of 1:9. The resulting homogenates were centrifuged at 10,000 × g for 20 min at 4°C, and the supernatants were collected and divided into aliquots.
Assessment of oxidative stress markers (MDA)
The MDA content in colorectal homogenate samples was measured using a colorimetric kit (Biodiagnostic, Egypt) [Citation21]. To perform the assay, 200 µL of the sample and standard were transferred to separate tubes containing 1000 µL of chromagen. In a blank tube, 1000 µL of chromagen was added and incubated for 30 min in a boiling water bath. Following the incubation period, 200 µL of the sample was added to a blank tube containing only chromagen and mixed thoroughly. The resulting mixture was analyzed by measuring the absorbance of the sample (Sample A) against the blank and the absorbance of the standard (A Standard) against distilled water at a wavelength of 534 nm.
Determination of colorectal (8-OHdG) levels using the ELISA technique
The levels of 8-OHdG in the colorectal homogenate samples were quantitatively measured using a sandwich ELISA kit (Bioassay Technology Laboratory, China) following the manufacturer’s instructions. ELISA plate microwells were pre-coated with mouse 8-OHdG antibody to initiate the assay. Next, the sample containing 8-OHdG was transferred to the microwells and bound to antibodies adsorbed on the surface. Biotinylated mouse 8-OHdG was then added to the microwells to bind to the 8-OHdG in the sample. Subsequently, streptavidin-horseradish peroxidase (HRP) was added and bound to the biotinylated 8-OHdG antibody. Unbound streptavidin-HRP was removed through a washing step after a designated incubation period. Finally, a substrate solution was added, and the resulting HRP reaction produced color development that was directly proportional to the quantity of 8-OHdG present in the sample.
Statistical analysis
The IBM SPSS software package (version 20.0; Armonk, NY, IBM Corp.) was used for data analysis. The normality of the distribution was confirmed using the Shapiro-Wilk test. The results are presented as the mean ± standard deviation and percentage of change. One-way analysis of variance (ANOVA) was used to compare normally distributed quantitative variables among the different study groups. Pairwise comparisons were conducted using Tukey’s post hoc test (Tukey). Statistical significance was set at p < 0.05. The following acronyms were used in the presentation of the results: SD, standard deviation; p, p-value for one-way ANOVA test; *, statistical significance at p ≤ 0.05; a, significance with the control group; b, significance with the EDTA group; c, significance with the DMSO group; d, significance with the Nic group; e, significance with the DMH group.
Results
Hematoxylin and Eosin stain (H&E)
Normal control and EDTA-treated colorectal sections demonstrated a normal mucosal cellular structure characterized by an epithelium rich in goblet cells (, respectively). After four weeks, sections from the DMSO and Nic groups exhibited normal mucosal structures, with only a few inflammatory cells (, respectively). However, sections from the DMH group showed primitive signs of adenoma, presenting as aberrant crypt foci (ACF) and a reduction in goblet cells (). Sections from the Nic-DMH group showed almost normal crypt histology with scattered inflammatory cells ().
Figure 1. Photomicrographs of colorectal sections post-4 weeks through the mucosal layer showing the epithelium of (a) control and (b) EDTA groups rich with goblet cells (asterisks), (c) DMSO and (d) Nic groups with the normal structure of the mucosa within a few inflammatory cells (arrowheads), (e) DMH group with aberrant crypt foci (ACF) (dashed arrows) and goblet cell reduction (stars), and (f) Nic-DMH group with scattered inflammatory cells (arrowheads) (H&E stain, scale bar: 50 µm).
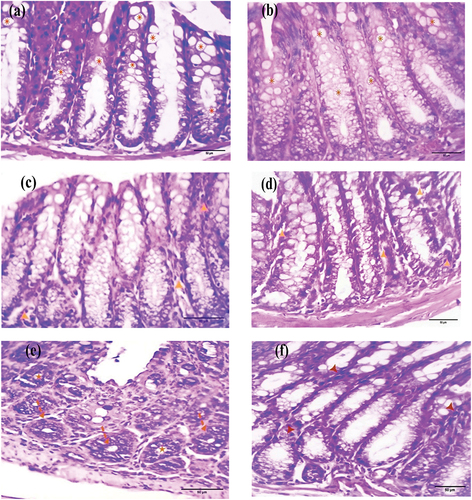
After six weeks, the colorectal sections from the EDTA, DMSO, and Nic groups exhibited a normal mucosal structure with scattered inflammatory cells (, respectively). Conversely, colorectal sections from the DMH group showed distinct signs of adenoma, such as dysplasia, hyperchromasia, and goblet cell depletion (). However, colorectal sections from the Nic-DMH group had nearly restored normal histoarchitecture ().
Figure 2. Photomicrograph of colorectal sections post-6 weeks through the mucosa layer showing the epithelium of (a) EDTA, (b) DMSO, and (c) Nic groups with scattered inflammatory cells (arrowheads), (d) DMH group with hyperchromasia (H), dysplastic cells (arrows), and goblet cell reduction (stars), and (e) Nic-DMH group with rich goblet cells (asterisks). (H&E stain, scale bar: 50 µm).
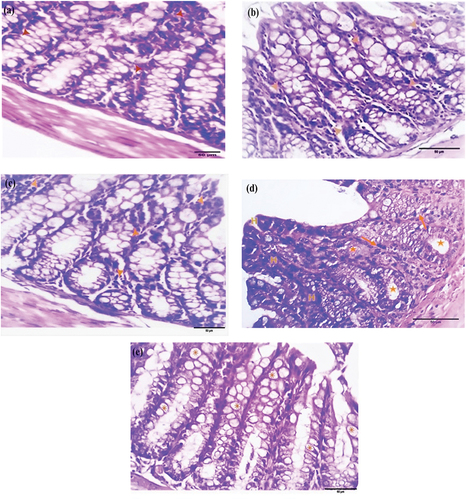
Masson’s trichrome stain for collagen
Masson’s trichrome stain assessed mouse colorectal sections for fibrosis to detect collagen deposition, which appears blue-green. Control sections exhibited mild collagen deposition (). After four weeks, sections from the EDTA, DMSO, and Nic groups showed moderate collagen deposition as a thin rim around the glandular crypts in the mucosal layer (, respectively). The DMH sections demonstrated moderate collagen deposition (), whereas the Nic-DMH sections showed moderate collagen deposition ().
Figure 3. Photomicrograph of colorectal section post-4 weeks through mucosa layer showing (a) control group with mild collagen deposition, (b) EDTA, (c) DMSO, (d) Nic, (e) DMH, and (f) Nic-DMH groups with moderate collagen deposition. (Masson’s trichrome staining, scale bar: 50 µm).
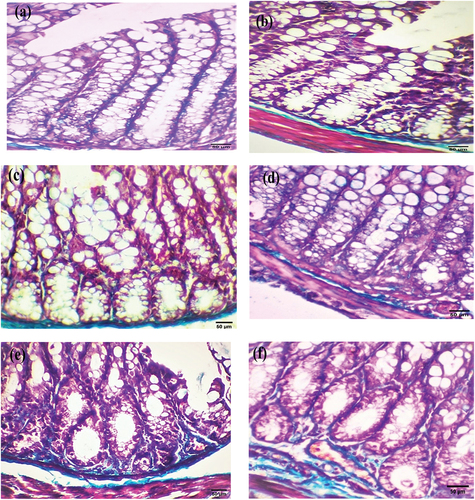
After six weeks, the colorectal sections of mice in the experimental groups that received EDTA, DMSO, and Nic showed a moderate increase in collagen deposition around glandular crypts in the mucosal layer (, respectively). However, sections from mice in the DMH group showed neoplastic cells with strong collagen deposition around the glandular crypts (). Colorectal sections from the Nic-DMH group revealed a nearly normal architecture with a mild staining reaction ().
Figure 4. Photomicrograph of colorectal section post-6 weeks through mucosa layer showing (a) EDTA group, (b) DMSO group, and (c) Nic group with moderate collagen deposition, (d) DMH group with strong collagen deposition, and (e) Nic-DMH group with mild collagen deposition (Masson’s trichrome staining, scale bar: 50 µm).
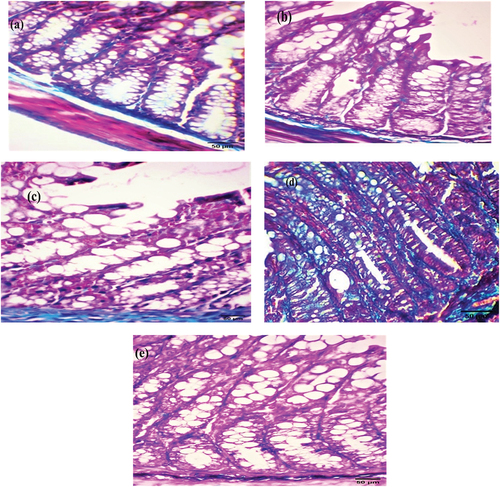
Immunohistochemical investigation
Immunohistochemistry of AIF was performed to detect apoptosis, and brown immunostaining was observed as a cytoplasmic reaction. The examined colorectal sections of control and EDTA mice showed negative cytoplasmic immunostaining ( respectively). After four weeks, the DMSO and Nic groups exhibited mild cytoplasmic immunostaining ( respectively). However, DMH sections demonstrated strong cytoplasmic immunostaining (), whereas Nic-DMH sections showed moderate cytoplasmic immunostaining ().
Figure 5. Photomicrograph of the colorectal section after four weeks through the mucosa layer showing (a) control and (b) EDTA groups with negative staining, (c) DMSO, and (d) Nic groups with cytoplasmic immunostaining reactions, (e) DMH group with strong immunostaining reaction, and (f) Nic-DMH group with moderate reaction. (AIF IHC staining; scale bar = 50 µm).
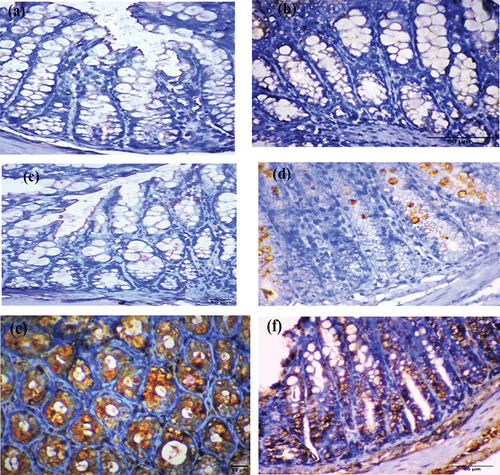
AIF immunohistochemical analysis was performed six weeks post-treatment. Colorectal sections of mice treated with EDTA showed negative cytoplasmic immunostaining (). Conversely, moderate cytoplasmic immunostaining was observed in sections from the DMSO and Nic groups ( respectively). Colorectal tumor sections from mice treated with DMH showed a strong cytoplasmic immunostaining reaction to AIF (). Colorectal sections from the Nic-DMH group showed mild cytoplasmic immunostaining for AIF ().
Figure 6. Photomicrograph of colorectal sections post-6 weeks showing AIF immunohistochemical staining through the mucosal layer, with (a) negative staining in the EDTA group, (b) moderate staining in the DMSO group, (c) moderate staining in the Nic group, (d) strong staining in the DMH group, and (e) mild staining in the Nic-DMH group. Scale bar: 50 µm.
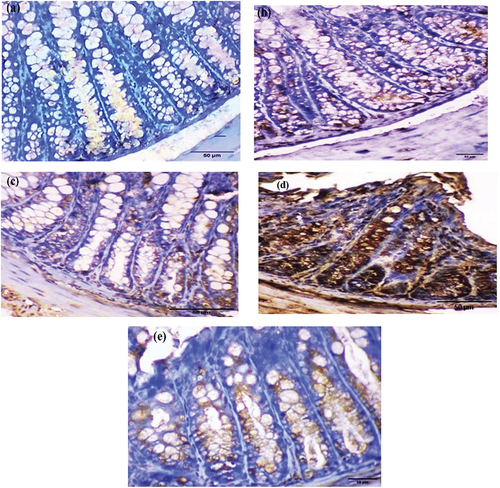
Image analysis of the AIF% area
summarize the AIF intensity represented as a percentage area for the control, EDTA, DMSO, Nic, DMH, and Nic-DMH groups.
Assessment of oxidative stress markers (MDA)
The MDA content was assessed in colorectal homogenate samples from 54 mice in control, EDTA, DMSO, Nic, DMH, and Nic-DMH groups and expressed as nmol/g. The results are summarized in .
Determination of colorectal (8-OHdG) levels using the ELISA technique
8-OHdG levels were analyzed in colorectal homogenate samples from 54 mice in different groups, and the concentrations were expressed in ng/mol. presents the results.
Discussion
Cancer is a term used to refer to disorders characterized by the uncontrollable development of aberrant cells that infiltrate other tissues [Citation22]. Geographically, CRC is more prevalent in developed countries, and in Egypt, it accounts for 6.48% of all cancers, according to the National Cancer Institute at Cairo University [Citation23]. CRC formation is a complex and diverse process that arises from various etiological factors [Citation24]. Depending on the degree, the condition can be classified as benign, noncancerous, or malignant [Citation25].
Animal models of CRC are valuable tools for studying the disease. Experimental induction of tumors in laboratory animals enables researchers to study tumor characteristics that are difficult to examine in patients. The DMH model, which exhibits morphological similarities to human CRC, is a widely used experimental model that has yielded significant insights into human CRC etiology and contributing factors of human CRC. The DMH model is beneficial for investigating colorectal carcinogens and chemopreventive agents [Citation26].
The organotypic colon carcinogen DMH is an alkylating chemical commonly used to induce benign and malignant neoplasms in rodent colon. DMH undergoes oxidation and transformation into AOM, which is subsequently hydroxylated to MAM in the liver and colonic mucosa by cytochrome P450. MAM is chemically unstable at body temperature, leading to its spontaneous dissociation from formaldehyde, water, and nitrogen. The breakdown of MAM generates the alkylating agent, methyl-diazonium ion, which ultimately forms a reactive carbonium ion capable of alkylating macromolecules (DNA, RNA, or protein) in the colon through enzymatic and non-enzymatic processes. Nitrogenous base oxygen atoms are the primary targets of alkylation, leading to an increased likelihood of DNA mispairing, which is essential in mutagenesis and carcinogenesis [Citation27]. ACFs were initially identified as the earliest visible lesions in the colons of mice exposed to carcinogens. DMH treatment resulted in the formation and proliferation of ACFs, which resemble the early stages of CRC in mice, accompanied by goblet cell reduction and nuclear alterations of the cells bordering the lumen of the crypt, reflecting its tumor-promoting capabilities. Carcinogen exposure causes significant adenoma abnormalities manifesting as dysplasia [Citation7].
Nic, a cell-permeable salicylanilide, has been approved by the Food and Drug Administration for its anthelmintic properties. Owing to its weak solubility in water, Nic was dissolved in a solvent mixture of 3/15/82 (v/v/v) dimethyl sulfoxide (DMSO)/Cremophor EL/water for animal administration [Citation28]. Several studies have demonstrated that Nic uncouples oxidative phosphorylation and reduces ATP levels in various tumor cells, including CRC cells [Citation29,Citation30]. Nic can indirectly inhibit DNA DSB (DNA double-strand break) repair [Citation31].
Several studies have demonstrated that elevated levels of oxygen species (ROS) are associated with intrinsic apoptosis and G2/M phase cell cycle arrest triggered by anticancer drugs [Citation32,Citation33]. Nic has been found to arrest the cell cycle in the G2/M phase and induce cell death via the ROS-mitochondrial apoptotic pathway [Citation33]. G2/M arrest can prevent damaged cells from entering mitosis, leading to apoptosis [Citation34].
Histopathological analysis revealed that the glandular cells of the mucosal layer had a normal histological structure in the colorectal sections of mice in the control group. In colorectal sections of the induced cancer group, tumor cells with anaplasia, dysplasia, and hyperchromasia were observed in the lumen. The mucosal lining epithelium was significantly increased in mice treated with Nic-DMH, which is consistent with recent studies by Alkhuriji et al. [Citation35] and Wang et al. [Citation36]. These observations suggest that Nic treatment significantly reduced carcinogenesis by limiting the effectiveness of DMH in initiating and promoting neoplastic alterations.
Concerning Masson’s trichrome, colon sections from the control group showed normal collagenous fiber deposition in the periglandular cells of the mucosal layer and lamina propria. Leukocytic inflammatory cells aggregated with tumor cells in colon sections of mice with induced colon cancer. The structure of the Nic-DMH group was normal, with a slight increase in collagen content. This finding aligns with the results of Alkhuriji et al. [Citation35] and indicates colon cancer proliferation, migration, polarity, and differentiation. Furthermore, it illustrates that the basement membrane-like matrix shifts to an interstitial-type matrix containing fibril-forming collagen [Citation37].
Examination of AIF (% area) using light microscopy and ImageJ analysis showed a significant increase in the DMH group compared to the control group. However, in the Nic-DMH group, the AIF staining level was slightly higher than that in the control group, consistent with previous findings reported by Cobanoglu et al. [Citation38]. AIF expression in CRC may contribute to apoptosis induction, leading to selective pressure for apoptosis during cancer progression.
To evaluate the influence of Nic on CRC, MDA levels were compared. The colon homogenates of the DMH group showed a significant increase compared to those of the control group. However, this increase in LPO levels was prevented in the Nic-DMH group, which is consistent with the findings of Alkhuriji et al. [Citation35], and Sherif et al. [Citation39]. The increased oxidative stress shown by a higher MDA concentration corroborated the DMH buildup of free radicals.
Furthermore, increased ROS levels resulted in a significant increase in 8-OHdG levels in the colon of the DMH group compared to the control group. However, this increase in 8-OHdG levels was suppressed in the Nic-DMH group, which is consistent with the previous findings reported by Kitagawa et al. [Citation40]. Notably, Nic significantly decreased 8-OHdG levels prior to DMH treatment, confirming the antioxidant activity of Nic. Collagen deposition, AIF staining intensity, MDA concentration, and 8-OHdG levels were all reduced after Nic injection, demonstrating its potential anti-CRC impact.
Conclusion
This study demonstrated that Nic might positively affect CRC induced by DMH injections. The effect of Nic on oxidative stress was evidenced by a reduction in MDA levels, which were elevated in the DMH group. Nic’s reduction of oxidative stress was also reflected in the mucosal layer by a decrease in collagen deposition, AIF staining intensity, and 8-hydroxy-2’-deoxyguanosine (8-OHdG) levels. These findings suggest that Nic may be a potent therapeutic agent for CRC treatment. Further research is required to confirm these results and explore the mechanisms underlying the anti-CRC effects of Nic.
Authors’ contributions
Aya Sayed designed the study, conducted the experiments, and wrote the manuscript. Eman A. Youssef assisted with the data and image analysis using image analyzer software. Shimaa A. Mahmoud and Sara Youssry assisted with the practical biochemical analyses. Ashour A. Abdel-Mawla reviewed and revised the manuscript and made revisions. All authors have approved and agreed to submit the final version of the manuscript.
Consent for publication
The authors declare their agreement to publication.
Ethics approval
The guidelines for the ethical care and treatment of animals were followed in accordance with the ethical standards for scientific research established by the Institutional Animal Care and Use Committee (IACUC) of Alexandria University, Egypt. Ethical approval was obtained under reference number AU0122051011.
Limitations of the study
After analyzing tissue homogenates from mice, we found that Niclosamide has an antioxidant role in colorectal cancer. However, we recommend biochemical evaluation of serum tumor markers.
Additionally, we suggest including the crypt multiplicity as a way to confirm the presence of colorectal cancer. This will provide valuable insights and enhance the quality of the indicative outcomes. Therefore, experiments may be planned in the future to address these issues.
Acknowledgments
We thank Prof. Dr. Hossam Eldin M. Ghoneim (Dept. of Immunology and Allergy, Medical Research Institute, Egypt) and Dr. Fatema Elzahraa Ossama Badawy (Dept. of Pathology, Medical Research Institute, Egypt) for their valuable advice, expert technical assistance, and interpretation of results.
Disclosure statement
No potential conflict of interest was reported by the author(s).
References
- Fanelli GN, Dal Pozzo CA, Depetris I, et al. The heterogeneous clinical and pathological landscapes of metastatic Braf-mutated colorectal cancer. Cancer Cell Int. 2020;20(1):30. doi: 10.1186/s12935-020-1117-2
- Beena TB, Jesil MA, Harikumar KB. Cross-talk between AMP-activated protein kinase and the sonic hedgehog pathway in the high-fat diet triggered colorectal cancer. Arch Biochem Biophys. 2023;735:109500. doi: 10.1016/j.abb.2022.109500
- Siegel RL, Jakubowski CD, Fedewa SA, et al. Colorectal cancer in the Young: epidemiology, prevention, management. Am Soc Clin Oncol Edu Book. 2020;2020(40):e75–e88. doi: 10.1200/edbk_279901
- Wong MCS, Huang J, Lok V, et al. Differences in incidence and mortality trends of colorectal cancer worldwide based on sex, age, and anatomic location. Clin Gastroenterol Hepatol. 2021;19(5):955–966.e961. doi: 10.1016/j.cgh.2020.02.026
- Guo J, Yu Z, Das M, et al. Nano codelivery of oxaliplatin and folinic acid achieves synergistic chemo-immunotherapy with 5-fluorouracil for colorectal cancer and liver metastasis. ACS Nano. 2020;14(4):5075–5089. doi: 10.1021/acsnano.0c01676
- Grivennikov SI. Inflammation and colorectal cancer: colitis-associated neoplasia. Semin Immunopathol. 2013;35(2):229–244. doi: 10.1007/s00281-012-0352-6
- Venkatachalam K, Vinayagam R, Arokia Vijaya Anand M, et al. Biochemical and molecular aspects of 1,2-dimethylhydrazine (DMH)-induced colon carcinogenesis: a review. Toxicol Res. 2020;9(1):2–18. doi: 10.1093/toxres/tfaa004
- Hassan HFH, Mansour AM, Salama SA, et al. The chemopreventive effect of thymol against dimethylhydrazine and/or high fat diet-induced colon cancer in rats: relevance to NF-κB. Life Sci. 2021;274:119335. doi: 10.1016/j.lfs.2021.119335
- Kim Y-W, Byzova TV. Oxidative stress in angiogenesis and vascular disease. Blood. 2014;123(5):625–631. doi: 10.1182/blood-2013-09-512749
- Kumar B, Koul S, Khandrika L, et al. Oxidative stress is inherent in prostate cancer cells and is required for aggressive phenotype. Cancer Res. 2008;68(6):1777–1785. doi: 10.1158/0008-5472.Can-07-5259
- Zińczuk J, Maciejczyk M, Zaręba K, et al. Antioxidant barrier, redox status, and oxidative damage to biomolecules in patients with colorectal cancer. Can malondialdehyde and catalase be markers of colorectal cancer advancement? Biomolecules. 2019;9(10):637. doi: 10.3390/biom9100637
- Greten FR, Grivennikov SI. Inflammation and cancer: triggers, mechanisms, and consequences. Immunity. 2019;51(1):27–41. doi: 10.1016/j.immuni.2019.06.025
- Zhao H, Wu L, Yan G, et al. Inflammation and tumor progression: signaling pathways and targeted intervention. Signal Transduct Target Ther. 2021;6(1):263. doi: 10.1038/s41392-021-00658-5
- Panahi Y, Dadkhah M, Talei S, et al. Can anti-parasitic drugs help control COVID-19? Future Virol. 2022;17(5):315–339. doi: 10.2217/fvl-2021-0160
- Frayha GJ, Smyth JD, Gobert JG, et al. The mechanisms of action of antiprotozoal and anthelmintic drugs in man. Gen Pharmacol. 1997;28(2):273–299. doi: 10.1016/S0306-3623(96)00149-8
- Wang Z, Ren J, Du J, et al. Niclosamide as a promising therapeutic player in human cancer and other diseases. Int J Mol Sci. 2022;23(24):16116. doi: 10.3390/ijms232416116
- Chen W, Mook RA, Premont RT, et al. Niclosamide: Beyond an antihelminthic drug. Cell Signall. 2018;41:89–96. doi: 10.1016/j.cellsig.2017.04.001
- Pan JX, Ding K, Wang CY. Niclosamide, an old antihelminthic agent, demonstrates antitumor activity by blocking multiple signaling pathways of cancer stem cells. Chin J Cancer. 2012;31(4):178–184. doi: 10.5732/cjc.011.10290
- Luo F, Luo M, Rong Q-X, et al. Niclosamide, an antihelmintic drug, enhances efficacy of PD-1/PD-L1 immune checkpoint blockade in non-small cell lung cancer. J Immunther Cancer. 2019;7(1):245. doi: 10.1186/s40425-019-0733-7
- Gurley KE, Moser RD, Kemp CJ. Induction of colon cancer in mice with 1,2-dimethylhydrazine. Cold Spring Harb Protoc. 2015;2015(9):db.prot077453. doi: 10.1101/pdb.prot077453
- Liu H, Weisman D, Y-B Y, et al. An oxidative stress response to polycyclic aromatic hydrocarbon exposure is rapid and complex in Arabidopsis thaliana. Plant Sci. 2009;176(3):375–382. doi: 10.1016/j.plantsci.2008.12.002
- Berg DJ, Davidson N, Kühn R, et al. Enterocolitis and colon cancer in interleukin-10-deficient mice are associated with aberrant cytokine production and CD4(+) TH1-like responses. J Clin Invest. 1996;98(4):1010–1020. doi: 10.1172/JCI118861
- Ayad E, Eesa AN, Radi R, et al. Expression of “connexin 43” in colorectal carcinomas: histopathological and immunohistochemical study. Open Access Maced J Med Sci. 2020;8(A):354–359. doi: 10.3889/oamjms.2020.4676
- Pancione M, Remo A, Colantuoni V. Genetic and epigenetic events generate multiple pathways in colorectal cancer progression. Pathol Res Int. 2012;2012:509348. doi: 10.1155/2012/509348
- Angell HK, Bruni D, Barrett JC, et al. The immunoscore: colon cancer and beyond. Clin Cancer Res. 2020;26(2):332–339. doi: 10.1158/1078-0432.Ccr-18-1851
- Perše M, Cerar A, Cerar A. The dimethylhydrazine induced colorectal tumours in rat - experimental colorectal carcinogenesis. Radiol Oncol. 2005;39(1):61–70. doi: 10.1080/00365520410009519
- Fu D, Calvo JA, Samson LD. Balancing repair and tolerance of DNA damage caused by alkylating agents. Nat Rev Cancer. 2012;12(2):104–120. doi: 10.1038/nrc3185
- Moskaleva EY, Perevozchikova VG, Zhirnik AS, et al. Molecular mechanisms of antitumor activity of niclosamide. Biochem (Mosc) Suppl B. 2015;9(4):312–324. doi: 10.1134/S1990750815040022
- Xiang M, Chen Z, Yang D, et al. Niclosamide enhances the antitumor effects of radiation by inhibiting the hypoxia-inducible factor-1α/vascular endothelial growth factor signal. Oncol Lett. 2017;14(2):1933–1938. doi: 10.3892/ol.2017.6372
- Zhirnik AS, Semochkina YP, Moskaleva EY, et al. Molecular mechanisms of antitumor activity of the polymeric form of niclosamide with respect to human colorectal cancer cells. Biochem (Mosc) Suppl B. 2017;11(3):301–307. doi: 10.1134/S1990750817030131
- Mook RA, Ren X-R, Wang J, et al. Benzimidazole inhibitors from the Niclosamide chemotype inhibit Wnt/β-catenin signaling with selectivity over effects on ATP homeostasis. Bioorg Med Chem. 2017;25(6):1804–1816. doi: 10.1016/j.bmc.2017.01.046
- Lei Q, Zhang L, Xia Y, et al. A novel benzothiazole derivative SKLB826 inhibits human hepatocellular carcinoma growth via inducing G2/M phase arrest and apoptosis. RSC Adv. 2015;5(52):41341–41351. doi: 10.1039/C5RA05387K
- Yang F, Ye T, Liu Z, et al. Niclosamide induces colorectal cancer apoptosis, impairs metastasis and reduces immunosuppressive cells in vivo. RSC Adv. 2016;6(107):106019–106030. doi: 10.1039/C6RA21533E
- Gao F, Ma N, Zhou H, et al. Zinc oxide nanoparticles-induced epigenetic change and G2/M arrest are associated with apoptosis in human epidermal keratinocytes. Int J Nanomedicine. 2016;11:3859–3874. doi: 10.2147/ijn.S107021
- Alkhuriji AF, Alsaiari SG, Alomar SY, et al. Effect of mesenchymal stem cells on cytochrome-c release and inflammation in colon cancer induced by 1,2-dimethylhydrazine in Wistar albino rats. Biosci Rep. 2021;41(3):BSR20204356. doi: 10.1042/bsr20204356
- Wang C, Qiao X, Wang J, et al. Amelioration of DMH-induced colon cancer by eupafolin through the reprogramming of apoptosis-associated p53/Bcl2/Bax signaling in rats. Eur J Inflammat. 2022;20:20587392211069771. doi: 10.1177/20587392211069771
- Grigorescu M. Noninvasive biochemical markers of liver fibrosis. J Gastrointestin Liver Dis. 2006;15(2):149–159.
- Cobanoglu B, Ceyran AB, Simsek M, et al. Immunohistochemical analysis of Bax and AIF in colorectal tumors. Int J Clin Exp Med. 2015;8(9):16071–16076.
- Sherif DA, Makled MN, Suddek GM. The HIV reverse transcriptase inhibitor tenofovir suppressed DMH/HFD-induced colorectal cancer in Wistar rats. Fundam Clin Pharmacol. 2021;35(6):940–954. doi: 10.1111/fcp.12679
- Kitagawa H, Kitajima Y, Kai K, et al. Predictive value of the ratio of 8‑hydroxydeoxyguanosine levels between cancerous and normal tissues in patients with stage II/III colorectal cancer. Oncol Rep. 2019;41(5):3041–3050. doi: 10.3892/or.2019.7086