ABSTRACT
This study is aimed to investigate the therapeutic potential of a strain of Lactobacillus brevis (SAD) isolated from an ethnic fermented food consumed regularly by the native people of Darjeeling Himalayan region. Effects of regular consumption of this lactic acid bacteria (LAB) in ameliorating the pathophysiological conditions associated with hyperglycemia, insulin resistance, and obesity is studied in mice having induced obesity by high-fat diet (HFD). Key objectives included evaluating the strain’s impact on glycemic parameters, lipid profiles, colonic oxidative stress, inflammation, and gut integrity while assessing the metagenomic composition. For this study, male Swiss albino mice were grouped into Normal Diet (ND), HFD, and L. brevis SAD supplemented (LB) groups. It was found that, L. brevis SAD supplementation significantly improved glycemic parameters, insulin sensitivity, at the same time reduced glycation. It also mitigated obesity-related effects, such as weight gain, organ enlargement, and adverse lipid profiles. Moreover, the HFD group exhibited pronounced deterioration in gut integrity, evidenced by alterations in colonic histomorphology, concurrent with the development of obesity and hyperglycemia. In LB group gut integrity appeared to be restored in the histological study. Metagenomic analysis demonstrated the strain’s potential to modulate the gut microbial composition towards health promoting groups. This study demonstrates the therapeutic potential of L. brevis SAD in mitigating the adverse metabolic effects induced by HFD.
Introduction
The industrial and digital revolutions have led to significant changes in our way of life, including dietary shifts that have challenged public health monitoring organizations for innovation. The World Health Organization (WHO) reports that worldwide obesity has nearly tripled since 1975, with over 1.9 billion adults being overweight [Citation1]. Obesity transitions into metabolic disorders through complex mechanisms, with excessive fat tissue promoting insulin resistance (IR), leading to elevated blood glucose levels [Citation2]. This disrupts the balance of metabolic processes, creating an environment conducive to diabetes. This metabolic disorder is a significant global health concern, with a multifactorial etiology. Type 2 Diabetes Mellitus (T2DM) is mainly affected by lifestyle changes, with several risk factors identified, such as age, diet, sedentary habit, and obesity [Citation3]. Existing treatments for T2DM include oral medications and insulin injections, each with distinct side effects. Insulin injections commonly lead to hypoglycemia and weight gain, with specific insulin variants promoting cell division leading to malignancy [Citation4]. On the other hand more commonly used drugs such as, glibenclamide usage is linked to hypoglycemic shock [Citation5], rosiglitazone has cardiovascular hazards [Citation6]. More evidences support the hypothesis that altered gut microbiota is crucial in the onset and development of IR and eventual T2DM [Citation7]. In the intestinal ecosystem, any imbalance in the gut microbiota can affect normal metabolic pathways, leading to immune dysfunctions and chronic inflammation [Citation8]. There is growing interest in exploring non-pharmaceutical alternatives like probiotics as potentially effective and safe normoglycemic agents.
The present research aims to investigate the therapeutic potential of Lactobacillus brevis SAD particularly to test its efficacy in ameliorating the pathophysiological processes that occur during the onset of hyperglycemia and insulin resistance. This LAB strain was isolated from ‘Sinki’ which is made from taproots of Raphanus sativus. The consumption of this ethnic fermented food is said to be benefitial. Hence, this investigation intends to assess metabolomic and metagenomic parameters to unravel the specific microbial changes and their associated physiological and metabolic outcomes in the context of obesity, hyperglycemia, and IR. These findings hold promise for developing innovative probiotic-based therapeutic strategies for hyperglycemia, with the ultimate goal of enhancing the quality of life.
Materials and methods
Bacterial strain and culture
L. brevis strain SAD is isolated from a traditional fermented food called ‘Sinki’ popular in the Darjeeling Himalayan Region. Identification of the strain was confirmed through partial sequencing of the 16S rDNA gene. The sequence is authenticated and submitted to the GenBank for public availability (Acc. No. OQ306549). Cell concentrations were adjusted to 2 × 108 Colony Forming Units/Millilitre (CFU/mL) to create bacterial suspensions for use in animal experimentation.
Animal subjects and experimental setup
Housing and grouping of animals
Swiss albino mice aged 6–8 weeks and weighing 20 ± 2 g were obtained from Chakraborty Enterprise, Kolkata, India, with proper authorization (Regd. No. 1443/PO/Bt/s/11/CPCSEA). These animals were housed at the animal facility, subjected to controlled environmental conditions, with formal ethical clearance (IAEC/NBU/2022/27). Following two-weeks acclimatization period with standard pellet based diet, animals were distributed into three cohorts: ND, HFD, and LB supplemented group (each group having six animals). The LB group received the same HFD with daily L. brevis SAD supplementation of 108 CFU in 500 μL phosphate buffer saline (PBS), while all groups received equivalent PBS volumes daily. The study spanned 8 weeks, concluding with euthanasia under isoflurane anesthesia. The dosage, HFD composition (Table S1), and study duration adhered to established research standards, incorporating specific adaptations and referencing prior works [Citation9–11].
Post-experimental procedure and sample harvesting
After the euthanasia of the animal subjects, a batch of blood samples was collected and preserved within vials coated with ethylenediaminetetraacetic acid (EDTA). An additional batch of collected blood was let to clot and then centrifuged at 3000 revolutions per minute (RPM) for 3 minutes to isolate the serum. Furthermore, the internal organs like the pancreas, colon, adipose tissues, etc., along with the fecal contents, were harvested employing aseptic techniques and were subsequently stored at −20 °C, while the EDTA-treated blood samples were kept at 4 °C until further use. Tissues intended for histopathological evaluations were washed using physiological saline and then promptly immersed in formalin solution (of appropriate concentration for each tissue).
Assessment of glycemic parameters
Oral glucose tolerance test (OGTT)
To measure the OGTT, standard protocol was followed [Citation12]. In brief, the experimental procedure involved the administration of a single oral glucose dosage (2 g/kg) to mice, followed by the monitoring of blood glucose concentrations attained through a sterile puncture of the tail vein. Recordings were conducted promptly following glucose administration (0 minutes), as well as at intervals of 30, 60, 90, and 120 minutes thereafter, using a handheld glucometer (Dr. Morepen BG-03, Morpen Laboratories Ltd., India).
Fasting insulin, homeostatic model assessment for insulin resistance (HOMA-IR) and glycosylated haemoglobin (Ghb)
The quantification of glycosylated hemoglobin percentage (GHb%) and fasting insulin levels was carried out using established colorimetric and enzyme-linked immunosorbent assay (ELISA) methodologies, respectively, using standard kits (GHb Kit, Coral Clinical Systems, India; Mouse Insulin ELISA Kit, MyBiosource, USA) in accordance with the manufacturer’s instructions. The assessment of IR was conducted through the utilization of the Homeostatic Model Assessment for Insulin Resistance (HOMA-IR), a computational model that integrates fasting glucose and insulin levels [Citation13].
Histological evaluation of pancreas
Pancreas tissues were initially fixed using a 10% formalin solution followed by dehydration, hematoxylin and eosin (H&E) staining, and sectioning (5 µm). Observations were done using the Olympus Magnus CX21i Microscope (Olympus Magnus, Japan). To assess the islet hypertrophy, first, the diameter of the islet was taken from random islets using MagVision image analysis software using 10 X objective (with 100 μm scale bar) (Magnus Opto Systems, India) then the size of the islets was quantified in terms of μm2. For detailed visualization of the islets, images were also taken with a 40 X objective (With a 25 μm scale bar).
Assessment of obesity and lipidomic profiles
Percent body weight increase and relative organ weight
The body weights of each animal in each group were measured at the commencement and culmination of the experimental procedure to derive the percent increase. In evaluating relative organ weights, post-animal sacrifice, relevant organs were weighed, and their weight was calculated.
Serum biochemical analysis
To analyze the serum lipidomic status, standard colorimetric kits were used (Coral Clinical Systems, India) according to the manufacturer’s protocol. The tests involved the measurement of the levels of total cholesterol (TC), high-density lipoprotein cholesterol (HDL-C), triglycerides (TG), Aspertate transaminase (AST), Alanine Transaminase (ALT) and gamma-glutamyl transferase (GGT). These measurements were performed according to the manufacturer’s instructions. The values of the earlier measured lipids were used to derive additional lipid parameters, including low-density lipoprotein cholesterol (LDL-C), and very-low-density lipoprotein cholesterol (VLDL-C) using Friedewald’s formula [Citation14].
Histopathological assessment of adipose tissue
Perigonadal white adipose tissues (pgWAT) were collected from euthanized mice and fixed in 4% formalin, followed by paraffin embedding, sectioning (4 µm), dehydration and H&E staining. To determine the average cross-sectional area of adipocytes, the slides were examined using Olympus Magnus CX21i Microscope. The cross-sectional area of the adipocytes was measured by taking the diameter of random adipocytes from each group (at 40 X objective with 25 μm scale bar) using MagVision image analysis software and then calculating the area in terms of μm2. Overall visualization images were also taken with a 10 X objective (With a 100 μm scale bar).
Assessment of integrity, oxidative status, and inflammatory state of the gut
Macroscopic and microscopic (histological) scoring of colonic damage
To assess colonic damage, both macroscopic and microscopic methods were used. The macroscopic evaluation employed a standardized scoring system, including criteria such as diarrhea, adhesions, ulcerations, and the length of the large intestine [Citation15]. For microscopic assessment, colon samples were sectioned and stained with H&E stain and scored following a standard protocol with minor modifications [Citation16]. All microscopic examinations were conducted using an Olympus Magnus CX21i Microscope, and measurements were taken, particularly for muscle thickness, using MagVision image analysis software at 10 X objective (with 100 μm scale bar). Also, images of the basal side (B) and the luminal side (L) were taken at 40 X objective (With 25 μm scale bar) for detailed inspection of the integrity changes.
Assessment of colonic oxidative status
To assess the oxidative status of the gut tissues, samples were processed by homogenization, and double centrifugation. The superoxide dismutase (SOD) assay, Reduced glutathione (GSH) concentration, Malondialdehyde levels (MDA), and Catalase activity was determined by following standard protocols [Citation17–20].
Measurement of colonic inflammatory state
Serum levels of C- Reactive Protein (CRP) and Lipopolysaccharide-binding protein (LBP) were quantified using ELISA kits (MyBiosource, USA and Elabscience, USA, respectively).
Evaluation of fecal metagenomic composition
Biokart India Pvt. Ltd. conducted the fecal metagenomics analysis in Bengaluru, India. Bacterial DNA extraction from fecal contents was performed using the QIAamp DNA stool mini kit (Dusseldorf, Germany), according to the manufacturer’s guidelines. PCR amplification targeted the V3-V4 region of 16S rDNA using specific primers. Sequencing was performed on an Illumina Miseq system (Santiago, USA). Quality control of raw data involved FastQC (Ver. 0.11.9) and MultiQC (Ver. 1.14), followed by adapter trimming and removal of low-quality reads using TrimGalore (Ver 0.6.5). Further processing included merging paired-end reads, eliminating chimeras, and quantifying operational taxonomic unit (OTU) abundance using Quantitative Insights Into Microbial Ecology (QIIME, Version 2023.2) and Kraken Version 2 workflows. Databases such as SILVA, GREENGENES, and NCBI were utilized. The unprocessed sequencing data was deposited in the NCBI Sequence Read Archive (SRA) repository with the accession number PRJNA1026825.
Statistical analysis and graphical interpretations
Analysis of all the data except some parts of the metagenomics was done using GraphPad Prism 8 software package (Version 8.0.1). Data were expressed as mean ± standard deviation (SD). Statistical significance was calculated by comparing the ND and LB group with the HFD (as a negative control group) using one-way analysis of variance (ANOVA) followed by post hoc Dunnet’s test for multiple comparisons. Differences were considered significant at p < 0.05.
Results
The supplementation of L. brevis SAD led to a significant enhancement in glycemic parameters
Significant differences were observed in OGTT values among the experimental groups. Throughout the observation period, it had been observed that the ND and LB group showed a significantly lower trajectory (p < 0.0001) while compared with HFD group only at 30 mins after the administration of initial glucose load the LB group showed less significant (p < 0.0021) glucose-level change when compared with that of the HFD group. Moreover, it was observed that the glucose levels did not came back to normal baseline even after 120 mins in HFD group (). Fasting insulin levels also exhibited significant differences with the ND group (3.36 ± 0.05 mU/L; p < 0.0001) compared with the HFD group (8.15 ± 0.52 mU/L), LB group fasting insulin values (5.67 ± 0.52 mU/L; p < 0.0001) were although elevated but significantly lower than the HFD group, reinforcing normoglycemia due to L. brevis SAD supplementation (). GHb% was reduced significantly in case of the LB group (7.67 ± 0.82%; p < 0.0002) while comparing with HFD group (15.17 ± 4.50%), resembling that of the ND group (6.72 ± 1.41%; p < 0.0002) (). HOMA-IR values also reflected improved insulin sensitivity in the ND and LB groups compared to the HFD group (0.79 ± 0.09, 1.16 ± 0.25 vs. 3.34 ± 0.45; p < 0.0001) (). Histological analysis demonstrated hypertrophic islets in the HFD group (28711.74 ± 14422.32 µm2), while LB and ND groups displayed smaller, structurally normal islets (5472.15 ± 1199.34 µm2, 5679.70 ± 2172.48 µm2; p < 0.0002) () with no pathological damage ().
Figure 1. Effect of dietary supplementation of L. brevis SAD on glycemic parameters and pancreatic histomorphology among different experimental groups (each bar represents mean of 6 replicates ± SD; arrows in the histological images (10×) showing the position of the islets).
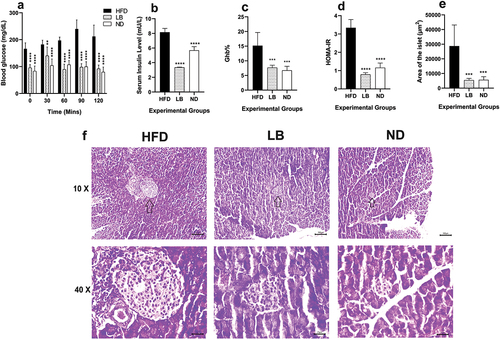
The administration of L. brevis SAD ameliorated the metabolic challenges linked to obesity, along with improvement in lipidomic profiles
Both the LB and ND groups exhibited significantly lower percent body weight increment compared to that of the HFD group, with LB showing 58.24 ± 13.41% (p < 0.0002) and ND showing 52.54 ± 12.80% (p < 0.0001) body weight gain, whereas in HFD a notable increase in percent body weight gain at 92.67 ± 2.45% was observed (). Similar patterns were observed in the relative organ weights, with pgWAT, liver, kidney, and pancreas all significantly enlarged in case of the HFD group, and the LB and ND groups having significantly lower relative organ weights ()). Serum lipidomic profiles showed that the LB and ND groups had significantly lower levels of detrimental lipids such as triglycerides and total cholesterol (Triglycerides in LB and ND: 98.36 ± 12.97 mg/dL; p < 0.0001 and 90.79 ± 17.67 mg/dL; p < 0.0001, Total Cholesterol in LB and ND: 99.11 ± 6.38 mg/dL; p < 0.0001 and 92.90 ± 11.78 mg/dL; p < 0.0001, respectively) compared to the HFD group (Triglycerides and Total Cholesterol: 212.72 ± 22.71 mg/dL and 219.18 ± 21.11 mg/dL, respectively) (). Furthermore, elevated levels of good cholesterol, HDL-C were significantly elevated in the LB and ND cohorts (36.75 ± 5.61 mg/dL; p < 0.0001 and 47.34 ± 4.02 mg/dL; p < 0.0001, respectively) when compared with the HFD group (14.96 ± 2.97 mg/dL) () but lower levels of LDL-C and VLDL-C was observed in LB and ND (LDL-C in LB and ND: 42.70 ± 5.04 mg/dL; p < 0.0001 and 27.40 ± 13.38 mg/dL; p < 0.0001, VLDL-C in LB and ND: 19.67 ± 2.59 mg/dL; p < 0.0001 and 18.16 ± 3.53 mg/dL; p < 0.0001, respectively) compared to the HFD group (LDL-C and VLDL-C: 161.68 ± 22.44 mg/dL and 42.54 ± 4.54 mg/dL, respectively) (). Furthermore, serum hepatic markers associated with obesity and metabolic dysregulation, including GGT, ALT, and AST, were significantly lower in the LB and ND groups compared to the HFD group (GGT of LB: 7.33 ± 1.45 U/L; p < 0.0002, ND: 4.92 ± 1.08 U/L; p < 0.0001, ALT of LB: 83.67 ± 8.18 U/L; p < 0.0021, ND: 65.32 ± 15.66 U/L; p < 0.0002, AST of LB: 107.36 ± 20.65 U/L; p < 0.0001, ND: 102.34 ± 24.88 U/L; p < 0.0001) ()). Histological examinations of pgWAT showed significantly smaller mean adipocyte area in the LB and ND groups (683.08 ± 243.83 µm2; p < 0.0001 and 771.52 ± 353.24 µm2; p < 0.0001, respectively) compared to the HFD group (3014.65 ± 1008.46 µm2) (), suggesting a reduction in lipid accumulation. Interestingly, animals from the HFD group exhibited histological characteristics indicative of increased cell proliferation or lipid accumulation, while the LB and ND groups displayed a more stable tissue architecture with tightly packed adipocytes ().
Figure 2. Effect of dietary supplementation of L. brevis SAD on physical obesity parameters, serological lipidomic and hepatic profiling and histomorphology of pgWAT among different experimental groups (each bar represents mean of 6 replicates ± SD).
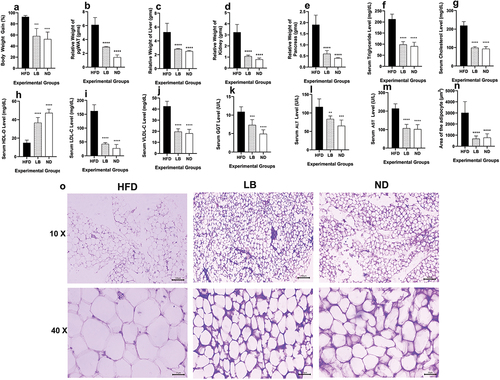
L. brevis SAD supplementation mitigated oxidative stress in the colon, alleviated the inflammatory milieu, and enhanced the colonic integrity associated with an HFD regimen
While measuring the colonic oxidative parameters, significant differences were observed. SOD levels were markedly higher in both the LB and ND groups compared to the HFD group (0.07 ± 0.01 Units/mg tissue, p < 0.0001 and 0.10 ± 0.01 Units/mg tissue, p < 0.0001, respectively, vs. 0.04 ± 0.01 Units/mg tissue in HFD (). Similarly, catalase activity levels were significantly elevated in the LB and ND groups (1.86 ± 0.40 Unit/mg of Colon, p < 0.0002 and 5.58 ± 0.92 Unit/mg of Colon, p < 0.0001, respectively) compared to the HFD group (0.37 ± 0.08 Unit/mg of Colon (). The GSH levels were also significantly higher in both the LB and ND groups (69.69 ± 5.47%, p < 0.0001 and 80.59 ± 5.06%, p < 0.0001, respectively), conversely the MDA equivalent levels were lower in the ND group (0.04 ± 0.01 nM/mg of tissue, p < 0.0001) than the HFD group (Relative GSH concentration and MDA equivalent: 25.14 ± 6.01% and 0.11 ± 0.02 nM/mg of tissue, respectively (). Serum levels of LBP remained within the normal range for both the LB and ND groups (278.50 ± 53.39 ng/mL, p < 0.0001 and 190.34 ± 42.67 ng/mL, p < 0.0001, respectively), contrasting with the HFD group’s markedly elevated LBP levels (1083.44 ± 51.67 ng/mL) (). On the other hand, CRP levels showed significant differences in the LB and ND groups (1.53 ± 0.03 mg/L, p < 0.0002 and 1.31 ± 0.19 mg/L, p < 0.0001, respectively) compared to the HFD group (1.94 ± 0.15 mg/L) (). Histological assessments further demonstrated the impact of L. brevis SAD on colonic architecture. Extensive immune cell infiltration was observed in HFD group along with colonic structural disruption, loss of muscular thickness, and goblet cell mass reduction, while the LB and ND groups showed significant improvements and resembled each other’s architecture (). Macroscopic evaluations demonstrated that the damage score values in the HFD group were significantly higher (5.66 ± 3.32) than those in the LB and ND groups (2.5 ± 1.37; p < 0.0332 and 1 ± 1.26; p < 0.0021, respectively) (). Likewise, microscopic damage scores exhibited a similar trend, with the HFD group scoring markedly higher at 7.16 ± 1.94 compared to both the LB and ND groups (3 ± 0.63; p < 0.0001 and 1.33 ± 0.51; p < 0.0001, respectively) () (Table S2).
Figure 3. Effect of dietary supplementation of L. brevis SAD on the colonic oxidative state, inflammatory status and colonic histomorphology among different experimental (each bar represents mean of 6 replicates ± SD; in case of the histological images at 40 X magnification, the arrows indicated the immune infiltration, circles denoted the muscular thickness, rectangular box indicated the apical structure of the villus; B indicates the basal side and L indicates the luminal side of the colon).
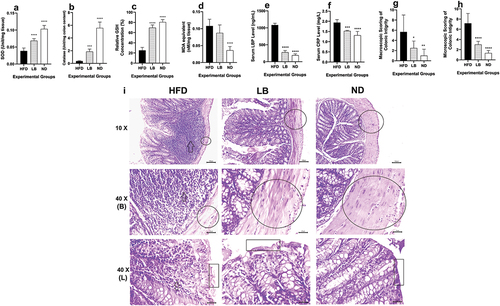
Administration of L. brevis SAD exerts a restorative influence in fecal metagenomic composition induced by HFD regimen
Metagenomic profiling revealed significant changes in microbiome diversity, with differences in phyla abundances among groups. In the HFD group, Bacteroidetes dominated (66.20%), followed by Firmicutes (20.46%), while the LB group displayed a relatively balanced Firmicute and Bacteroidetes percentage (39.10% and 44.37%, respectively). The ND group had a composition with Bacteroidetes at 51.61% and Firmicutes at 38.73% (Table S3). In case of the genus level it was observed that HFD group had enriched genera-like Bacteroides, Unclassified Bacteroidales, and Unclassified Clostridiales, reflecting diet-induced dysbiosis. The LB group showed increased Lactobacillus, Anaerostipes, and Blautia, suggesting positive microbial balance restoration. In the ND group, Bacteroides and Prevotella were dominant () (Table S4). Sequence abundance data demonstrated significant differences, with higher Bacteroidetes abundance in HFD (4919), higher Lactobacillus abundance in LB (1691), and higher Blautia abundance in LB. Species-level analysis showed similar trends, with Bacteroides dorei, Lactobacillus backii, and Prevotella denticola exhibiting differences among groups. Anaerostipes hadrus, Clostridium tyrobutyricum, and Treponema caldarium were virtually absent in the ND group but present in both HFD and LB groups (). Principal Coordinates Analysis (PCoA) based on Morisita Horn Dissimilarity Index revealed distinct microbial community patterns. In the HFD group significant separation from LB and ND along the first principal coordinate (PC1) was observed, indicating a distinct gut microbiota composition induced by the HFD. The LB group partially overlapped with the ND group along PC1, suggesting that L. brevis SAD supplementation may mitigate some dietary-induced changes. The ND group displayed the most minor variation along PC1, reflecting the stability of its gut microbiota composition under normal diet conditions ().
Discussion
The present study demonstrates that supplementation with L. brevis SAD can attenuate the metabolic syndrome induced by HFD. The LB group exhibited enhanced glucose homeostasis, improved insulin sensitivity, and reduced glycation compared to the HFD group. This improvement in metabolic parameters may be attributed to the influence of L. brevis SAD on gut microbiota and their metabolites, as suggested in previous research [Citation21]. Regarding pancreatic histomorphology, exposure to an HFD typically leads to hyperglycemia and islet hypertrophy due to increased β cell proliferation and subsequent hyperinsulinemia [Citation22]. Prolonged hyperglycemia induces islet hypertrophy as an adaptive response to insulin resistance [Citation23]. The histopathological analysis revealed that the HFD group experienced significant pancreatic islet enlargement and hyperinsulinemia, while the LB and ND group maintained stable histological structures and serum insulin levels. These findings highlight the effectiveness of L. brevis SAD in alleviating pancreatic islet hypertrophy and associated hyperglycemia induced by HFD consumption.
Moreover, the results of the lipidomic profiling indicated that supplementation with L. brevis SAD can mitigate the adverse effects of disruption of lipid metabolism by reducing percent body weight gain, fat accumulation, and organ enlargement. Serological lipid status was also positively influenced, with the LB group showing lower triglycerides, total cholesterol, LDL-C, and VLDL-C levels and increased HDL-C levels than the HFD group, indicating improved cardiovascular health. Histological analysis supported these findings, showing reduced lipid accumulation in pgWATs of the LB group. These results are consistent with prior research highlighting the potential of probiotics in mitigating inflammation, improving insulin resistance, and influencing gut microbiota [Citation24–26]. It demonstrates that L. brevis SAD supplementation can effectively prevent obesity, improve lipid metabolism, and counteract the adverse consequences of excessive dietary fat intake on body composition.
The assessment of colonic oxidative status in response to an HFD reveals heightened activity in intestinal epithelial cells (IEC) in the distal intestine, resulting in elevated reactive oxygen species (ROS), iron, copper, aldehydes, and lipid peroxidation products. This ROS production negatively impacts gut epithelial barrier integrity, ultimately leading to compromised gut integrity and disruption of the gut microbiome [Citation27]. Critical parameters such as MDA for lipid peroxidation, GSH concentration, catalase activity, and SOD inhibition are crucial in evaluating oxidative stress associated with gut integrity [Citation28]. In the present study it was observed that HFD regime decreased the catalase, SOD, GSH and catalase levels in HFD group, indicating reduced antioxidant capacity, likely due to increased ROS production in the colon. L. brevis SAD supplementation increased SOD and catalase levels, enhancing antioxidant capacity, possibly by reducing ROS production and activating the nuclear factor erythroid 2–related factor (Nrf2) pathway [Citation29]. Additionally, HFD elevated MDA levels, indicative of increased oxidative stress, potentially caused by overwhelming the antioxidant capacity and causing excessive lipid peroxidation in the colon [Citation30]. The study also assessed serological indicators of colonic inflammation, LBP and CRP. HFD has been observed to lead to immune infiltration, inflammation in colonic tissues, shifts in gut microbiota composition, and increased levels of inflammatory molecules and toxins. The results showed that L. brevis SAD mitigated systemic inflammation by reducing LBP and CRP levels, possibly by modulating nuclear factor-kappa B (NF-κB) expression and addressing gut barrier function disruption caused by HFD [Citation31]. These findings suggest that L. brevis SAD attenuated the systemic inflammation possibly by modulating the expression of NF-κB, which is a crucial transcription factor that regulates the production of inflammatory mediators [Citation27].
Interestingly macroscopic assessment of the colon of HFD group revealed compromised gut barrier function, characterized by diarrhea, adhesion of the colon to internal organs, reduced colon length, and inflammatory ulcerations. Conversely, the LB group exhibited significantly better scores, resembling the ND group. Microscopic examination unveiled immune cell infiltration and colonic tissue damage in the HFD group, both substantially improved in the LB group. Moreover, muscle thickening reduction and goblet cell depletion in the HFD group were countered by LB, resembling the ND group. L. brevis SAD also preserved colonic luminal surface integrity like the ND group. Scientific evidence corroborates that probiotics can enhance the intestinal barrier, maintain gut lining integrity, mitigate endotoxemia, reduce inflammation, and promote a healthier gut microbiota [Citation32,Citation33]. These findings suggest that supplementation with L. brevis SAD offers substantial benefits in ameliorating the deleterious impacts of a high-fat diet (HFD) on colonic gut barrier function.
The metagenomic investigation indicates an elevation in Bacteroidetes content as a consequence of eating HFD. While the Firmicutes/Bacteroidetes (F/B) ratio is a focal point, it is imperative to acknowledge that alterations in this ratio can result from increases in other phyla, and such dysbiotic changes may not necessarily influence the F/B ratio. Notably, Proteobacteria emerged as the most variable phylum, contributing to dysbiosis [Citation34] and exhibiting correlations with reduced Firmicutes and overall microbial diversity in inflammatory bowel disease (IBD). In an earlier study augmented Firmicutes and diminished Bacteroidetes were observed, with regulatory T cell trafficking from lymph nodes to the intestine in response to probiotic treatment [Citation35]. Several human studies have reported a higher abundance of Bacteroidetes and a decreased abundance of Firmicutes in obese individuals [Citation36]. Given the HFD’s association with increased percent body weight gain and related obesity parameters, it is plausible that these pathophysiological changes underlie the observed reduction in the gut F/B ratio in the present study. Heatmap results indicated elevated levels of detrimental genera in the microbiome of the HFD group like Alistipes, Bacteroides, Clostridium, Desulfovibrio, Muribaculum, and Staphylococcus, but the same remained relatively low or absent in LB and ND. These genera had been linked to metabolic abnormalities and gut dysbiosis. These findings align with previous studies, emphasizing the intricate relationship between diet, supplementation, and microbial communities [Citation37–41].
These metagenomic findings establish a correlation with the observed biochemical, histological, and physiological outcomes, providing mechanistic insights into the beneficial effects of L. brevis SAD supplementation in ameliorating HFD-induced metabolic syndrome [Citation42]. The significant increase in Lactobacillus in the LB group, alongside the positive alteration of the F/B ratio, reflects the potential of L. brevis SAD in modulating gut microbiota composition [Citation42]. This microbial modulation corresponds well with the observed improvements in glucose homeostasis, insulin sensitivity, and reduced glycation, suggesting a direct influence on metabolic parameters. Furthermore, the improved lipid profile in the LB group coincides with the metagenomic findings, emphasizing the interplay between gut microbiota modulation and lipid metabolism [Citation43]. The enhanced antioxidant capacity observed in the colonic tissues of the LB group, coupled with the alleviation of systemic inflammation, supports the link between microbial composition, gut barrier function, and metabolic health [Citation43,Citation44]. The metagenomic analysis therefore elucidates the potential role of L. brevis SAD in countering HFD-induced dysbiosis, offering a comprehensive understanding of the intricate relationships among dietary supplementation, gut microbiota, and metabolic outcomes.
Conclusion
This study presents robust evidence demonstrating the efficacy of L. brevis SAD supplementation in ameliorating the metabolic syndrome induced by HFD. The positive impacts of L. brevis SAD are multifaceted, encompassing enhancements in glucose regulation, improved insulin sensitivity, and reduced glycation of molecules. Histological examinations aligned with previous researches and indicated the connection between hyperglycemia and islet hypertrophy in response to HFD. L. brevis SAD supplementation effectively mitigated islet hypertrophy, maintaining stable pancreatic structure and insulin levels. Lipidomic profiling further illustrates the strain’s ability to counteract the detrimental effects of obesity, including weight gain, fat deposition in adipose tissues, and organ enlargement. Notably, L. brevis SAD supplementation results in more favorable serum lipid profiles, lowering triglycerides, total cholesterol, LDL-C, and VLDL-C while increasing HDL-C, potentially reducing the risk of cardiovascular disease. In colonic tissues, L. brevis SAD effectively modulates oxidative stress and reduces inflammation. The gut barrier function is significantly improved, enhancing gut lining integrity. Finally, alterations in gut microbiota composition revealed L. brevis SAD’s capacity to influence metabolic health positively. In summary, this study comprehensively demonstrates the potential of L. brevis SAD as a valuable dietary supplement suggesting its future therapeutic promises.
Language help
The language of the original manuscript was checked and verified by Grammarly (v1.0.42.877; subscription EDU) software package.
CRediT author statement
Amlan Jyoti Ghosh: Methodology, Investigation, Writing – original draft, Writing – review & editing. Supriyo Ghosh: Methodology, Investigation, Formal analysis, Writing – review & editing. Rejuan Islam: Investigation, Visualization, Formal analysis. Sagar Sarkar: Investigation, Data Curation. Tilak Saha: Conceptualization, Writing – review & editing, Supervision.
Ethics approval
The in vivo animal experimentation received approval from the Institutional Animal Ethical Committee (IAEC) at the University of North Bengal, West Bengal, India. The Committee granted this approval for the Purpose of Control and Supervision of Experiments on Animals (CPCSEA), and it is documented under reference number IAEC/NBU/2022/27, dated 23 September 2022.
Supplementary Information.doc
Download MS Word (197 KB)Author Bio.doc
Download MS Word (31 KB)Acknowledgments
Fellowship support
Fellowship support from the Council of Scientific and Industrial Research (CSIR), Government of India for Amlan Jyoti Ghosh (Ref. No. 09/285(0089)/2019-EMR-I Dated 07.10.2019), University Grants Commission (UGC), Government of India for Supriyo Ghosh (NTA Ref. No. 201610130713 Dated 01.04.2021), and University Grants Commission (UGC), Government of India for Rejuan Islam (UGC Ref. No. 711/(CSIR-UGC NET DEC.2018) dated 11.07.2019), are duly acknowledged.
Disclosure statement
No potential conflict of interest was reported by the author(s).
Data availability statement
The information created and analyzed during the current investigation is available upon reasonable request from the corresponding author.
Supplementary material
Supplemental data for this article can be accessed online at https://doi.org/10.1080/2314808X.2024.2324409.
Additional information
Funding
References
- World Health Organization, Obesity and overweight. [cited 2023 Sep 26]. Available from: https://www.who.int/news-room/fact-sheets/detail/obesity-and-overweight;2023
- Singh P, Rai SN. Factors affecting obesity and its treatment. Obes Med. 2019;16:100140. doi: 10.1016/j.obmed.2019.100140
- Qin J, Li Y, Cai Z, et al. A metagenome-wide association study of gut microbiota in type 2 diabetes. Nature. 2012;490(7418):55–60. doi: 10.1038/nature11450
- Donnor T, Sarkar S. Insulin- pharmacology, therapeutic regimens and principles of intensive insulin therapy. In: Feingold KR, Anawalt B, Blackman MR, et al., editors. Endotext [Internet]. South Dartmouth (MA): MDText.com, Inc; 2000.
- Gangji AS, Cukierman T, Gerstein HC, et al. A systematic review and meta-analysis of hypoglycemia and cardiovascular events: a comparison of glyburide with other secretagogues and with insulin. Diabetes Care. 2007;30(2):389–94. doi: 10.2337/dc06-1789
- Liu Y, Zheng S, Cui J, et al. Lactiplantibacillus plantarum Y15 alleviate type 2 diabetes in mice via modulating gut microbiota and regulating NF-κB and insulin signaling pathway. Braz J Microbiol. 2022;53(2):935–945. doi: 10.1007/s42770-022-00686-5
- Iatcu CO, Steen A, Covasa M. Gut microbiota and complications of type-2 diabetes. Nutrients. 2021;14(1):166. doi: 10.3390/nu14010166
- Zhong H, Wang J, Abdullah HM, et al. Lactobacillus plantarum ZJUFB2 prevents high fat diet-induced insulin resistance in association with modulation of the gut microbiota. Front Nutr. 2021;8:754222. doi: 10.3389/fnut.2021.754222
- Ondee T, Pongpirul K, Visitchanakun P, et al. Lactobacillus acidophilus LA5 improves saturated fat-induced obesity mouse model through the enhanced intestinal akkermansia muciniphila. Sci Rep. 2021;11(1):6367. doi: 10.1038/s41598-021-85449-2
- Pratchayasakul W, Kerdphoo S, Petsophonsakul P, et al. Effects of high-fat diet on insulin receptor function in rat hippocampus and the level of neuronal corticosterone. Life Sci. 2011;88(13–14):619–27. doi: 10.1016/j.lfs.2011.02.003
- Le Barz M, Anhê FF, Varin TV, et al. Probiotics as complementary treatment for metabolic disorders. Diabetes Metab J. 2015;39(4):291–303. doi: 10.4093/dmj.2015.39.4.291
- Sermet S, Cam ME, Hazar-Yavuz AN, et al. The methanolic extracts of teucrium polium L. and micromeria fruticosa (L.) Druce subsp. brachycalyx PH Davis improve diabetes in streptozotocin/nicotinamide-induced type 2 diabetic female Sprague Dawley rats. Obes Med. 2023;100517:100517. doi: 10.1016/j.obmed.2023.100517
- Ul Haq Shah MZ, Shrivastava VK, Mir MA. Ginger extract ameliorates endocrine-metabolic disturbances in letrozole-induced PCOS mice model by altering androgen-adiponectin status. Obes Med. 2023;39:100485. doi: 10.1016/j.obmed.2023.100485
- Martin SS, Blaha MJ, Elshazly MB, et al. Comparison of a novel method vs the Friedewald equation for estimating low-density lipoprotein cholesterol levels from the standard lipid profile. JAMA. 2013;310(19):2061–8. doi: 10.1001/jama.2013.280532
- Appleyard CB, Wallace JL. Reactivation of hapten-induced colitis and its prevention by anti-inflammatory drugs. Am J Physiol. 1995;269(1 Pt 1):G119–25. doi: 10.1152/ajpgi.1995.269.1.G119
- Chompre G, Sambolin L, Cruz ML, et al. A one month high fat diet disrupts the gut microbiome and integrity of the colon inducing adiposity and behavioral despair in male Sprague Dawley rats. Heliyon. 2022;8(11):e11194. doi: 10.1016/j.heliyon.2022.e11194
- Misra HP, Fridovich I. The role of superoxide anion in the autoxidation of epinephrine and a simple assay for superoxide dismutase. J Biol Chem. 1972;247(10):3170–5. doi: 10.1016/S0021-9258(19)45228-9
- Rahman I, Kode A, Biswas SK. Assay for quantitative determination of glutathione and glutathione disulfide levels using enzymatic recycling method. Nat Protoc. 2006;1(6):3159–65. doi: 10.1038/nprot.2006.378
- Heath RL, Packer L. Photoperoxidation in isolated chloroplasts. I. Kinetics and stoichiometry of fatty acid peroxidation. Arch Biochem Biophys. 1968;125(1):189–98. doi: 10.1016/0003-9861(68)90654-1
- Luck H. Catalase. In: Bergmeyer H, editor. Method of enzymatic analysis. New York and London: Academic Press; 1965. p. 885–894.
- Rastogi S, Singh A. Gut microbiome and human health: exploring how the probiotic genus Lactobacillus modulate immune responses. Front Pharmacol. 2022;13:1042189. doi: 10.3389/fphar.2022.1042189
- Mosser RE, Maulis MF, Moullé VS, et al. High-fat diet-induced β-cell proliferation occurs prior to insulin resistance in C57Bl/6J male mice. Am J Physiol Endocrinol Metab. 2015;308(7):E573–82. doi: 10.1152/ajpendo.00460.2014
- Ahrén J, Ahrén B, Wierup N. Increased β-cell volume in mice fed a high-fat diet: a dynamic study over 12 months. Islets. 2010;2(6):353–6. doi: 10.4161/isl.2.6.13619
- Wang X, Ba T, Cheng Y, et al. Probiotics alleviate adipose inflammation in high-fat diet-induced obesity by restoring adipose invariant natural killer T cells. Nutrition. 2021;89:111285. doi: 10.1016/j.nut.2021.111285
- Kobyliak N, Falalyeyeva T, Tsyryuk O, et al. New insights on strain-specific impacts of probiotics on insulin resistance: evidence from animal study. J Diabetes Metab Disord. 2020;19(1):289–296. doi: 10.1007/s40200-020-00506-3
- Kobyliak N, Falalyeyeva T, Mykhalchyshyn G, et al. Probiotic and omega-3 polyunsaturated fatty acids supplementation reduces insulin resistance, improves glycemia and obesity parameters in individuals with type 2 diabetes: a randomised controlled trial. Obes Med. 2020;19:100248. doi: 10.1016/j.obmed.2020.100248
- Ballard JWO, Towarnicki SG. Mitochondria, the gut microbiome and ROS. Cell Signal. 2020;75:109737. doi: 10.1016/j.cellsig.2020.109737
- Fan S, Chen S, Lin L. Research progress of gut microbiota and obesity caused by high-fat diet. Front Cell Infect Microbiol. 2023;13:1139800. doi: 10.3389/fcimb.2023.1139800
- Wu RY, Abdullah M, Määttänen P, et al. Protein kinase C δ signaling is required for dietary prebiotic-induced strengthening of intestinal epithelial barrier function. Sci Rep. 2017;7(1):40820. doi: 10.1038/srep40820
- Rahman I, MacNee W. Role of oxidants/antioxidants in smoking-induced lung diseases. Free Radic Biol Med. 1996;21(5):669–81. doi: 10.1016/0891-5849(96)00155-4
- Kim KA, Gu W, Lee IA, et al. High fat diet-induced gut microbiota exacerbates inflammation and obesity in mice via the TLR4 signaling pathway. PLoS One. 2012;7(10):e47713. doi: 10.1371/journal.pone.0047713
- Hemarajata P, Versalovic J. Effects of probiotics on gut microbiota: mechanisms of intestinal immunomodulation and neuromodulation. Therap Adv Gastroenterol. 2013;6(1):39–51. doi: 10.1177/1756283X12459294
- Gou HZ, Zhang YL, Ren LF, et al. How do intestinal probiotics restore the intestinal barrier? Front Microbiol. 2022;13:929346. doi: 10.3389/fmicb.2022.929346
- Shin NR, Whon TW, Bae JW. Proteobacteria: microbial signature of dysbiosis in gut microbiota. Trends Biotechnol. 2015;33(9):496–503. doi: 10.1016/j.tibtech.2015.06.011
- Liu Y, Tian X, He B, et al. Lactobacillus reuteri DSM 17938 feeding of healthy newborn mice regulates immune responses while modulating gut microbiota and boosting beneficial metabolites. Am J Physiol Gastrointest Liver Physiol. 2019;317(6):G824–G838. doi: 10.1152/ajpgi.00107.2019
- Duan M, Wang Y, Zhang Q, et al. Characteristics of gut microbiota in people with obesity. PLoS One. 2021;16(8):e0255446. doi: 10.1371/journal.pone.0255446
- Parker BJ, Wearsch PA, Veloo ACM, et al. The genus alistipes: gut bacteria with emerging implications to inflammation, cancer, and mental health. Front Immunol. 2020;11:906. doi: 10.3389/fimmu.2020.00906
- Wang J, Li W, Wang C, et al. Enterotype bacteroides is associated with a high risk in patients with diabetes: a Pilot study. J Diabetes Res. 2020;2020:6047145. doi: 10.1155/2020/6047145
- Hassan SA, Rahman RA, Huda N, et al. Hospital-acquired clostridium difficile infection among patients with type 2 diabetes mellitus in acute medical wards. J R Coll Physicians Edinb. 2013;43(2):103–7. doi: 10.4997/JRCPE.2013.203
- Nie Q, Hu J, Chen H, et al. Arabinoxylan ameliorates type 2 diabetes by regulating the gut microbiota and metabolites. Food Chem. 2022;371:131106. doi: 10.1016/j.foodchem.2021.131106
- Qin Q, Yan S, Yang Y, et al. A metagenome-wide association study of the gut microbiome and metabolic syndrome. Front Microbiol. 2021;12:682721. doi: 10.3389/fmicb.2021.682721
- Watanabe J, Hashimoto N, Yin T, et al. Heat-killed lactobacillus brevis KB290 attenuates visceral fat accumulation induced by high-fat diet in mice. J Appl Microbiol. 2021;131(4):1998–2009. doi: 10.1111/jam.15079
- Antony MA, Chowdhury A, Edem D, et al. Gut microbiome supplementation as therapy for metabolic syndrome. World J Diabetes. 2023;14(10):1502–1513. doi: 10.4239/wjd.v14.i10.1502
- Araujo R, Borges-Canha M, Pimentel-Nunes P. Microbiota modulation in patients with metabolic syndrome. Nutrients. 2022;14(21):4490. doi: 10.3390/nu14214490