ABSTRACT
Chronic kidney disease (CKD) is a world-wide health concern, and thus curative approach becomes highly valuable. Herein, we examined the possible therapeutic effect of bee venom collected from Algeria (Apis mellifera intermissa) on adenine-induced chronic kidney damage. Mice received intraperitoneal injection of adenine at a dose of 50 mg/kg for four weeks to induce CKD. After that, bee venom was administered intraperitoneally (i.p) to mice at a dose of 0.76 mg/kg BW for a week. Adenine-treated mice decreased the body weight evolution, and significantly increased the kidney weight, water intake, and urine output compared with controls. In addition, serum and urine urea and creatinine levels, and serum albumin concentrations were significantly increased, while serum creatinine clearance level was significantly decreased in adenine-treated mice compared with controls. Adenine-induced oxidative stress was evidenced by increased MDA content, and decreased catalase and GST activity, and GSH content in renal tissue. Meanwhile, the administration of bee venom to adenine-treated mice markedly recovered kidney function via improvement of the altered physiological and biochemical parameters. These were confirmed by the morphological and histopathological results. In conclusion, bee venom is a prising therapeutic natural agent for improving kidney function in adenine-induced CKD.
Introduction
Kidneys are the crucial organ of the human urinary system, preserving the salt’s homeostasis and filtering the blood from metabolic waste into urine [Citation1]. Thus even small renal functional alteration may cause short-term and long-term functional impairments and diseases [Citation2]. Chronic kidney disease (CKD) or chronic kidney failure is the progression to end-stage renal dysfunction, evidenced overall by hyperuricemia, hyperalbuminemia, hyperproteinemia, and anemia, in addition to hypercreatinemia, and uremia, which lead to death [Citation3]. In fact, the air environmental contamination, the lack of medical resources, and the awareness of chronic diseases contribute to the rapid prevalence of CKD [Citation4]. The induction of CDK by adenine has received attention in clinical experiments using animal models. Adenine administrated orally results in the formation of crystals in the proximal tubular epithelia, and subsequently inflammation and tubulointerstitial fibrosis leading to kidney dysfunction and anemia [Citation5]. The CKD in animal models induced by adenine is still a benchmark for preclinical investigations [Citation6]. As a result, researchers and scientists have paid great attention to developing effective and high therapeutic potency medicines against kidney diseases with little side effects and hence avoiding complicated kidney surgery [Citation7]. Traditional oriental medicine has used natural products, including medicinal plants and venoms to treat illnesses and diseases [Citation8,Citation9]. In Algeria, two bee subspecies are found separately, in the north as Apis mellifera intermissa, and in the south as Apis mellifera sahariensis [Citation10] whose stings were commonly proven to have effective medical benefits [Citation11]. Bee venom has been reported as a main bee product having bioactive beneficial molecules, including melittin, apamin, phospholipase A2, hyaluronidase, and histidine decarboxylase making it an efficient therapeutic product [Citation12,Citation13]. In addition, bee venom has powerful biological activities, including antibacterial [Citation14], antioxidant, and anti-inflammatory activity, in addition to cytotoxic effects on cancer cells [Citation15]. Thus, it has been traditionally used as an effective folk medicine in the world to treat different diseases, such as Parkinson’s disease, inflammatory skin disease, multiple sclerosis, hepatic fibrosis, and atherosclerosis [Citation14]. Previous studies have reported the beneficial effect of bee venom in attenuating acute kidney injury induced by cisplatin [Citation16], and lipopolysaccharide in mice [Citation17]. However, the possible therapeutic effect of bee venom on adenine-induced chronic kidney disease has not been elucidated. The present study was, therefore, devoted to possibly suggesting bee venom from Apis mellifera intermissa as a promising natural alternative medicine against adenine-induced chronic kidney disease in mice.
Materials and methods
Chemicals
Adenine was purchased from Sigma Chemical Company (St Louis, Missouri. USA). All other chemicals used in this study were of Analytical Reagent grade.
Collection of bee venom
Bee venom (Apis mellifera intermissa) was provided by the National Nahl Beekeepers’ Company of M’Sila City (northeast Algeria), by using a low-level electrical shock (a safe method for bees). Collected bee venom was lyophilized and stored in a dry and cool place. For experimental uses, the lyophilized bee venom was dissolved in saline solution (0.9% NaCl), and the main stock was aliquoted and kept at −20°C. The proteins of the crude bee venom were analyzed by the SDS-PAGE technique as previously described [Citation18] ().
Animals
Adult male Albino mice (Swiss mice strain) weighing 25–28 g were housed in the animal house of the Faculty of Science, Annaba University of Algeria. Mice had food and tap water ad-libitum and were kept under laboratory conditions of temperature (20–25°C), humidity (60–65%), and a 12-h light/dark cycle. The Ethical Committee of Annaba University in accordance with the Algerian Ministry of Higher Education and Scientific Research approved the experimental protocol and animal handling (Approval number PRFU/2022; D01N01EN210120220001).
Treatments
After 1 week of acclimatization, thirty-two mice were divided equally into the control group receiving only saline solution, adenine group, bee venom (BV) group, and adenine-bee venom (Adenine + BV) group. In the adenine group, mice received a consecutive intraperitoneal injection (i.p) of adenine at 50 mg/kg BW for 4 weeks to induce CKD [Citation19]. Mice in the BV group received BV daily for one week at a dose of 0.76 mg/kg BW corresponding to 1/15th LD50 of BV in mice via intraperitoneal injection (LD50 of bee venom from Apis mellifera intermissa is 11.48 mg/kg BW, i.p), which was suggested as a safely promising therapeutic dose [Citation18]. In the Adenine + BV group, mice were treated for one week with 0.76 mg/kg BW after the CKD induction. The body weight of control and treated mice was determined three times per week throughout the treatment period. Also, 24-hour urine was collected according to a previously described method for mouse micturation over void spots on paper by applying careful transabdominal pressure to provide urine [Citation20] for determination of the urine output. After the induction of CKD (4 wk of adenine injection) followed by a daily injection of BV for a week, mice were killed and blood samples were collected in clean plastic centrifuge tubes. The samples were centrifuged at 2795 × g for 15 min at 4°C, and the resulting supernatant was collected and stored in a freezer at −70°C until use for the biochemical analysis. Further, the kidneys were removed, washed in saline solution, and then divided into two equal parts, one was kept for oxidative markers determination, and the other was fixed in 10% formalin for the histo-pathological evaluation.
Kidney biochemical markers
An automated analyzer (Siemens Healthcare Diagnostics, Marburg, Germany) with commercially available kits (SpinReact, Spain) was used to determine the serum and urine biochemical renal function markers, including serum and urine levels of creatinine and serum level of creatinine clearance [Citation21], urea [Citation22], and albumin [Citation23].
Assessment of oxidative stress
The kidney section was homogenized using an ultrasonic disruption machine (Ningbo. Xin-zhi Biotechnology Co. Ltd., China), centrifuged at 1008 × g for 15 min, and the supernatant was collected for determination of oxidative stress makers. The renal tissue homogenates were used to determine the major oxidative and antioxidant markers. The malondialdehyde (MDA) (a lipid peroxidation maker) level was estimated according to the method of Samadi-Noshahr [Citation24] based on the chemical reaction between the thiobarbituric acid (TBA) and the malondialdehyde, a lipids peroxidation product. The content of reduced glutathione was determined as previously described [Citation25] based on the reduction rate of the Ellman reagent (5,5′-dithiobis-2-nitrobenzoic acid (DTNB)) to 5-thio-2-nitrobenzoic acid (TNB) in presence of sulphosalicylic acid. The enzymatic activity of glutathione-S-transferase (GST) was estimated as reported elsewhere [Citation26] by measuring the reaction of 1-chloro-2,4-dinitrobenzene (CDNB) with – SH groups of glutathione catalyzed by samples GST (µmol/min/mg prot) in accordance with absorbance increase at 340 nm. Catalase (CAT) activity was determined by monitoring the absorbance decrease as a result of the degradation of hydrogen peroxide (H2O2) at 240 nm [Citation27].
Histopathological examination
The fixed kidney samples from each group were cut into 2 μm −2-thick slices using a microtome (CM1850, Leica Microsystems Nussloch GmbH, Germany), embedded in paraffin, rinsed with distilled water, and stained with hematoxylin and eosin (HE) for histopathological examination [Citation28]. The histological sections were examined by a Leica optical microscope equipped with a camera (Leica DFC300-FX), and hence the percentage of altered tissue by comparing the marked areas to the total area examined was estimated using ImageJ, (version 1.51 h) a free internet available software (http://imagej.nih.gov/ij/).
Statistical analysis
The data are displayed as mean ± S.E.M. Pairwise comparisons between groups were tested by one-way ANOVA, using GraphPad Prism 5.0 (Prism 5 for Windows, version 5) where p < 0.05 was considered significant.
Results
SDS-PAGE analysis of crude bee venom
In , SDS-PAGE analysis of crude bee venom at two concentrations 3 and 5 mg/ml showed bands of molecular weight of 5 kDa, 16 kDa, and approximately 41 kDa.
Physiological results
As indicated in , body weight evolution was markedly and gradually reduced from the first treatment day of adenine treatment compared with control and bee venom-treated mice, showing similar body weight evolution during the first week. Body weight evolution revealed a marked gradual increase from the 29th day corresponding to bee venom-injected CKD mice (adenine + BV). In , the relative kidney weight, water intake, and urine output were significantly increased in adenine mice (p < 0.01), and adenine + BV (p < 0.05) compared with those of control mice. Moreover, these physiological parameters were significantly (p < 0.05) reversed in adenine + BV mice as compared with the adenine group. No significant changes in these parameters were observed in BV-treated mice.
Figure 2. Evolution of body weight (g) of control mice, bee venom-treated mice (0.76mg/kg body weight, IP) for one week (7 days), mice treated with adenine (50mg/kg body weight, IP) for four weeks (28 days) to induce CKD, and mice treated with adenine (50mg/kg body weight, IP) for four weeks (28 days) followed by intraperitoneal injection (IP) of bee venom (0.76mg/kg body weight, IP) for a week (7 days).
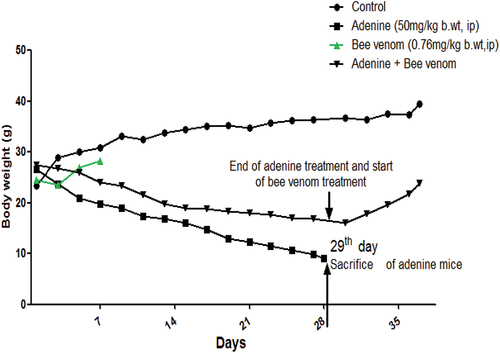
Table 1. Changes in some physiological parameters in control and treated mice.
Kidney gross morphology
As shown in , the control (A) and bee venom-treated mice (C) appeared normal bean- bean-shaped kidney morphology with a reddish-brown color, while in adenine-treated mice, kidneys were hypertrophy, and looked white-pale (B). The kidneys from bee venom-treated CKD mice (D) appeared improved kidney morphology compared with that of the group treated with adenine alone.
Biochemical parameters of kidney function
shows a significant increase in serum (p < 0.01) and urine (p < 0.001) creatinine, urea, and serum albumin concentrations, along with a significant decrease (p < 0.01) in creatinine-clearance-concentration noticed in adenine induced CKD and bee venom treated CKD mice (p < 0.05). These biochemical changes were significantly reversed in serum (p < 0.05) and urine (p < 0.01) of bee venom-treated CKD mice. Of note, bee venom-treated mice revealed no significant changes (ns) in the above-mentioned parameters compared with control mice.
Figure 3. Gross (Macroscopic) observations of kidneys from control and treated mice: Control (a) and bee venom mice (c) showed normal kidneys as bean-shaped with a reddish-brown color, adenine mice (b) appeared hypertrophic and white-pale colored kidneys, and kidneys from bee venom treated adenine mice (bee venom + adenine) (d) showing less morphological changes (kidneys with a close to normal color, and less hypertrophy) compared with adenine kidney mice.
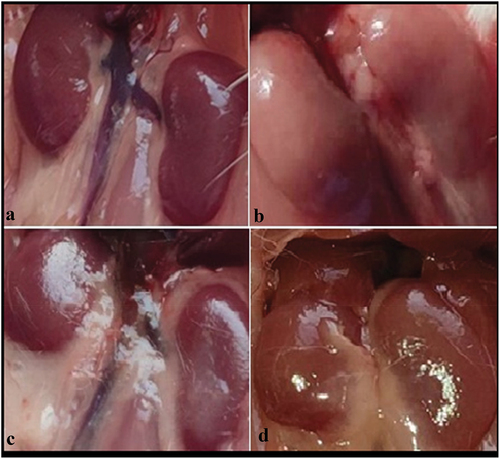
Oxidative stress effects
In , the GSH content, and the enzymatic activity of CAT and glutathione S-transferase (GST) were significantly decreased (p < 0.001) in CKD mice, and bee venom-treated CKD mice (p < 0.05) as compared with control mice, but they significantly increased (p < 0.05) when compared with adenine group. In contrast, results showed a significant increase in the tissue content of MDA in the adenine group (p < 0.001) and bee venom-treated adenine mice (p < 0.05) group compared to the control group, and a significant decrease (p < 0.05) when compared with adenine treated mice.
Figure 4. Concentrations of serum and urine urea and creatinine, serum concentration of albumin, and creatinine clearance in control and treated mice with adenine alone (50mg/kg body weight, IP) for four weeks, Bee venom (BV) alone (0.7mg/kg body weight, IP) for seven, and Adenine + Bee venom, BV was injected for 7 days after CKD induction. Error bars represent the error of the means. *p<0.05, **p< 0.01 and ***p<0.001 versus control group. #p< 0.05, ##p< 0.01 versus adenine group.
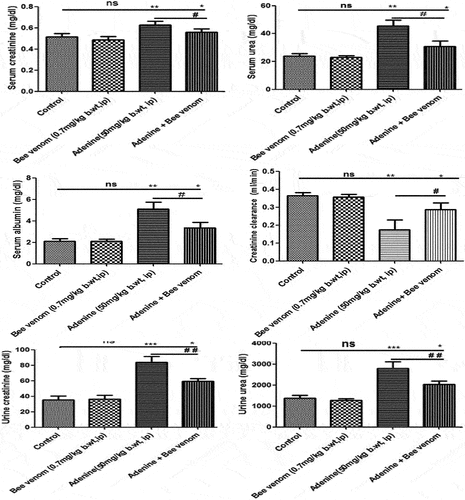
Histopathological changes
As shown in , Kidney sections from control and bee venom-treated mice appeared a comparable normal architecture of glomeruli and renal tubules, while adenine-treated mice showed marked kidney histological abnormalities characterized by degeneration of glomeruli with enlarged capsular space, and tubular necrosis in approximately 80% of the examined tissue areas, and presence of inflammatory cells. These histological changes were reduced in kidney histology of adenine + bee venom showing less glomeruli atrophy and tubular necrosis, and absence of inflammation involving about 45% of examined areas ().
Figure 5. Changes in the oxidative stress markers (GSH and MDA contents, and CAT and GST activity) in kidney tissue of control and treated mice with adenine alone (50mg/kg body weight, IP) for four weeks, Bee venom (BV) alone (0.7mg/kg body weight, IP) for seven, and Adenine + Bee venom, where BV was injected for 7 days after CKD induction. Error bars represent the error of the means. *p<0.05, **p< 0.01 and ***p<0.001 versus control group. #p< 0.05 versus adenine group.
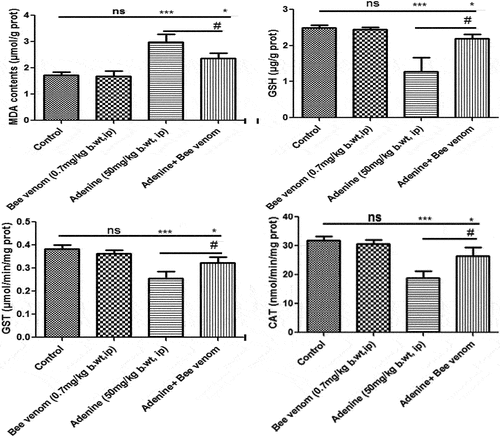
Figure 6. Hematoxylin and eosin staining of renal tissue (400x). Control mice and bee venom (0.7mg/kg bwt, ip) treated mice, showing normal renal histological structure and architecture. Adenine (50mg/kg bwt, ip) treated mice showing tubular necrosis (small arrow), glomeruli inflammation (Star), enlarged capsular space (thin arrow), and inflammatory cells (head arrow). Adenine + bee venom treated mice showing slight glomeruli tubular necrosis alterations. Scale bar: 50 µm.
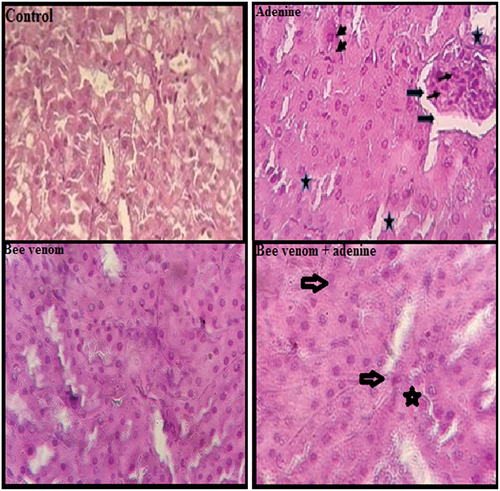
Discussion
Using natural products of bees, in particular, bee venom may be officious with less cost and side effects to avoid the progression of renal dysfunction to kidney failure, where kidney dialysis or transplantation are the only selected treatment options, which are quite expensive or unavailable in several developing countries. To our knowledge, the present study is the first to demonstrate that treatment with bee venom (Apis mellifera intermissa) collected from Algeria after the end of the CKD induction by adenine treatment effectively recovered kidney function. The SDS-PAGE analysis of the used crude bee venom revealed bands of molecular weights of 5 kDa, 16 kDa, and 41 kDa, which are previously reported to correspond respectively to melittin [Citation18], phospholipase A2 [Citation29], and hyaluronidase [Citation30]. Further, adenine has been largely used via ingestion in feed to induce CKD by adenine in animal models [Citation31–33]. As previously reported [Citation4,Citation5,Citation34–36], the high level of adenine leads to an increase in xanthine dehydrogenase that can be metabolized into 2,8-dihydroxyadenine, and consequently can deposit in renal tubules and induce oxidative chronic kidney damage. In this study, adenine injection decreased body weight, and increased kidney weights, water intake, and urine volume compared with control is likely due to interference of hepatic enzymes like cytochrome P450 enzyme system (CYP450) and metabolic process [Citation37]. Further, serum and urine concentrations of urea, creatinine, and serum albumin significantly increased, but creatinine clearance significantly decreased, in addition to morphological and histopathological alterations in the kidneys of the adenine-injected group. These adverse effects confirm the development of CKD, according to several previously reported results [Citation5,Citation6,Citation35,Citation38]. However, the mechanism by which adenine induces CKD is poorly investigated. Adenine and its main metabolite, 2,8-dihydroxyadenine (DHA), were reported to be low soluble compounds, and thus they can accumulate in the renal tubules and lead consequently to the development of kidney uremia [Citation39,Citation40]. Increment inflammation and oxidative stress states are common clinical and experimental complications in CKD patients [Citation4]. Additionally, increased oxidative stress-mediated overproduction of reactive oxygen species (ROS) and reactive nitrogen species (RNS) initiates kidney inflammatory cascade and cellular damage, in association with oxidation of cell macromolecules including lipids, proteins, and DNA, resulting in kidney injury [Citation41–43]. Accordingly, ROS was reported to be the plausible mechanism underlying adenine-induced chronic kidney damage [Citation44]. As a result, cells can prevent themselves from oxidative stress damage by improving the antioxidant enzymatic activities including CAT, and GST in addition to reduced glutathione content (GSH) which could reinforce the antioxidant defense system [Citation45]. In this study, adenine had a tendency to cause severe kidney oxidative injury evidenced by a marked decrease in kidney glutathione (GSH) content, and the enzymatic activity of catalase and glutathione S-transferase (GST), along with an increase in malondialdehyde (MDA) content. The results of catalase and MDA concord with those previously reported in mice administered intragastrically with 250 mg/kg bw of adenine daily for 7 days and then 135 mg/kg bw of adenine for 21 days every other day [Citation46]. Here, the authors referred that, under CKD development, the increased MDA level to the increased lipoperoxidation, and the decreased catalase activity to its vital role in catalyzing hydrogen peroxide into oxygen and water. In accordance with our results, the decreased activity of GST and GSH content in kidney tissue was reported in the work of Ali et al. [Citation47], investigating the induction of chronic kidney failure by adenine administered to mice at 0.75%, w/w in feed four weeks. Furthermore, our data revealed that adenine-induced CKD caused severe kidney histopathological alterations evidenced mainly by increased tubular necrosis, and glomeruli inflammation, and these results are in line with those previously reported [Citation48,Citation49]. On the other hand, the efficacy of several potential drugs and natural products was utilized to prevent and reverse adenine-induced kidney damage [Citation50,Citation51]. In this context, the study was performed on using bee venom as a promising therapeutic natural product of Apis mellifera intermissa subspecies bees of northeast Algeria. The BV was injected intraperitoneally into mice at the dose of 1/15th LD50 (0,76 mg/kg bw) on the basis of the previously determined LD50 value (11,48 mg/kg, i.p) [Citation18] to test the possible therapeutic effect in CKD mice. Alike to our experimental design, some studies adopted almost similar BV dosage treatments (dose and duration) to treat some health conditions, including Parkinson’s, arthritis, and cancer diseases [Citation52,Citation53]. Accordingly, bee venom and/or its main components were found to be therapeutically beneficial against some infections and diseases in animal models, including Streptococcus pyogenes-induced skin infection in mice [Citation54], Parkinson’s disease [Citation55], cholestatic liver disease in mice [Citation56] and cardiac damage in diabetic hyperlipidemic rats [Citation57]. Our results revealed no statistically significant differences in the kidney biochemical parameters, oxidative markers, and histological alterations compared with the control. Therefore, it is likely that bee venom-treated adenine mice showed noteworthy improvement in functional biochemical markers (serum and uric levels of urea and creatinine, serum level of albumin, and creatinine clearance), and the histological structure of the kidney. Consistent with these results, bee venom recovered kidney damage in cisplatin [Citation16] and lipopolysaccharide intraperitoneally injected mice [Citation17], and unilateral ureteral obstruction [Citation58]. The beneficial therapeutic effect of bee venom was related to the alleviation of tissue inflammation, oxidative stress, and tubular cell necrosis, and consequently, proved the novel recovery effects of bee venom against adenine-related CKD. As the study was performed on the whole bee venom, a complex mixture of bioactive molecules, further studies would be required to indicate the specific bee venom component responsible for the therapeutic effect. As reported, bee venom is composed mainly of pharmacological molecules, including melittin, apamin, ado-lapin, phospholipase A2, mast cell degranulation peptide, and hyaluronidase [Citation17,Citation59]. Moreover, bee venom attenuated adenine-induced oxidative stress in the kidney, as evidenced by an increased level of reduced glutathione (GSH), and the enzymatic activity of catalase and glutathione S- transferase (GST), along with a decreased level of MDA. These findings are in line with some previous studies, reporting the potential antioxidant activity of whole bee venom, and/or its main components through improvement of the cell antioxidant defense systems and reduction of ROS production in adverse health conditions in human and animal models [Citation60,Citation61]. Bee venom has been found to possess antioxidant activity and free radical scavenging properties. These properties contribute to the prevention of certain illnesses and diseases and the protection of cells against damage caused by oxidative agents such as free radicals [Citation62].
Conclusion
Bee venom collected in Algeria has effectively attenuated adenine-induced- worsened kidney damage. Further research and physicochemical analyses of bee venom are required to understand the molecular action mechanisms and therapeutic strategies.
Acknowledgments
The authors thank gratefully Pr. Aouadi S and Pr. Berrdjam H from the Department of Biochemistry, and Dr. Belhamzaoui Khaled from the Department of Biology of Annaba University of Algeria for their valuable assistance. This work is part of a national research project belonging to the University Training and Research (UTR) program (D01N01EN210120220001) and funded by the Ministry of Higher Education of Algeria.
Disclosure statement
No potential conflict of interest was reported by the author(s).
References
- Oroojalian F, Charbgoo F, Hashemi M, et al. Recent advances in nanotechnology-based drug delivery systems for the kidney. J Control Release. 2020;321:442–462. doi: 10.1016/j.jconrel.2020.02.027
- Lameire NH, Bagga A, Cruz D, et al. Acute kidney injury: an increasing global concern. Lancet. 2013;382(9887):170–179. doi: 10.1016/S0140-6736(13)60647-9
- Webster AC, Nagler EV, Morton RL, et al. Chronic kidney disease. Lancet. 2017;389(10075):1238–1252. doi: 10.1016/S0140-6736(16)32064-5
- Ali BH, Al-Husseni I, Beegam S, et al. Effect of gum Arabic on oxidative stress and inflammation in adenine–induced chronic renal failure in rats. PLoS One. 2013;8(2):e55242. doi: 10.1371/journal.pone.0055242
- Ashour WMR, Zamzam MSA, El Sayed HEED, et al. Effect of fetuin-A on adenine-induced chronic kidney disease model in male rats. Iran J Basic Med Sci. 2023;26(5):511. doi: 10.22038/IJBMS.2023.66346.14584
- Claramunt D, Gil-Peña H, Fuente R, et al. Animal models of pediatric chronic kidney disease. Is adenine intake an appropriate model? Nefrologia. Nefrología. 2015;35(6):517–522. doi: 10.1016/j.nefro.2015.08.004
- Bukutu C, Vohra S. Complementary Therapies for Renal Diseases. In: Pediatric Kidney Disease. 3rd ed. USA: Springer Link; 2023. p. 1987–2018. doi: 10.1007/978-3-031-11665-0
- Yuan H, Ma Q, Ye L, et al. The traditional medicine and modern medicine from natural products. Molecules. 2016;21(5):559. doi: 10.3390/molecules21050559
- Puzari U, Fernandes PA, Mukherjee AK. Pharmacological re-assessment of traditional medicinal plants-derived inhibitors as antidotes against snakebite envenoming: a critical review. J Ethnopharmacol. 2022;292:115208. doi: 10.1016/j.jep.2022.115208
- Adjlane N, Dainat B, Gauthier L, et al. Atypical viral and parasitic pattern in Algerian honey bee subspecies Apis mellifera intermissa and am sahariensis. Apidologie. 2016;47(5):631–641. doi: 10.1007/s13592-015-0410-x
- Wesselius T, Heersema DJ, Mostert JP, et al. A randomized crossover study of bee sting therapy for multiple sclerosis. Neurology. 2005;65(11):1764–1768. doi: 10.1212/01.wnl.0000184442.02551.4b
- Shi P, Xie SH, Yang JL. Pharmacological effects and mechanisms of bee venom and its main components: recent progress and perspective. Front Pharmacol. 2022;13:1001553. doi: 10.3389/fphar.2022.1001553
- Ullah A, Aldakheel FM, Anjum SI, et al. Pharmacological properties and therapeutic potential of honey bee venom. Saudi Pharm J. 2023;31(1):96–109. doi: 10.1016/j.jsps.2022.11.008
- Kong R, Lee YS, Kang DH, et al. The antibacterial activity and toxin production control of bee venom in mouse MRSA pneumonia model. BMC Complement Med Ther. 2020;20(1):1–12. doi: 10.1186/s12906-020-02991-8
- Sobral F, Sampaio A, Falcão S, et al. Chemical characterization, antioxidant, anti-inflammatory and cytotoxic properties of bee venom collected in Northeast Portugal. Food Chem Toxicol. 2016;94:172–177. doi: 10.1016/j.fct.2016.06.008
- Kim H, Lee G, Park S, et al. Bee venom mitigates cisplatin-induced nephrotoxicity by regulating CD4+ CD25+ Foxp3+ regulatory T cells in mice. Evid Based Complement Altern Med. 2013;2013:1–10. doi: 10.1155/2013/879845
- Kim JY, Lee SJ, Maeng YI, et al. Protective effects of bee venom against endotoxemia-related acute kidney injury in mice. Biology. 2020;9(7):154. doi: 10.3390/biology9070154
- Dahdouh F, Belhamzaoui K, Aouadi L, et al. Bee venom causes oxidative stress, biochemical and histopathological changes in the kidney of mice. Physiol Res. 2023;72(4):455–463. doi: 10.33549/physiolres.935125
- Abdolmaleky HM, Sheng Y, Zhou JR. Bioactive nutraceuticals oligo-lactic acid and fermented soy extract alleviate cognitive decline in mice in part via anti-neuroinflammation and modulation of gut microbiota. Front Nutr. 2023;10:278. doi: 10.3389/fnut.2023.1116278
- Kurien BT, Everds NE, Scofield RH. Experimental animal urine collection: a review. Lab Anim. 2004;38(4):333–361. doi: 10.1258/0023677041958945
- Young DS. Effects of drugs on clinical laboratory tests. Ann Clin Biochem. 1997;34(6): 579–81. doi: 10.1177/000456329703400601
- LA K, McNeely MDD. Folic acid assay. In: Kaplan L, and Pesce A, editors Clinical chemisty st louis CV Mosby. Toronto Canada; 1984. p. 1402–1406.
- Rodkey FL. Direct spectrophotometric determination of albumin in human serum. Clin Chem. 1965;11(4):478–487. doi: 10.1093/clinchem/11.4.478
- Samadi-Noshahr Z, Hadjzadeh M, Moradi-Marjaneh R, et al. The hepatoprotective effects of fennel seeds extract and trans-Anethole in streptozotocin-induced liver injury in rats. Food Sci Nutr. 2021;9(2):1121–1131. doi: 10.1002/fsn3.2090
- Ellman GL, Courtney KD, Andres JV, et al. A new and rapid colorimetric determination of acetylcholinesterase activity. Biochem Pharmacol. 1961;7(2):88–95. doi: 10.1016/0006-2952(61)90145-9
- Habig WH, Pabst MJ, Jakoby WB. Glutathione S-transferases the first enzymatic step in mercapturic acid formation. J Biol Chem. 1974;249(22):7130–7139. doi: 10.1016/S0021-9258(19)42083-8
- Aebi H. Catalase in vitro. Methods in enzymology. 1984;105:121–126. doi: 10.1016/s0076-6879(84)05016-3
- Bancroft JD MG, Churchill Livingstone, ed. Theory and practice of histological techniques. 6th ed. China: Elsevier health Sciences; 2008.
- Mammadova FZ, Topchiyeva A. Isolation and identification of biologically active components from the honey bee venom Apis mellifera L. caucasica. Moj Texicol. 2017;3(7):178–181. doi: 10.15406/mojt.2017.03.00078
- Zolfagharian H, Mohajeri M, Babaie M. Honey bee venom (Apis mellifera) contains anticoagulation factors and increases the blood-clotting time. J Pharmacopunct. 2015;18(4):7–11. doi: 10.3831/KPI.2015.18.031
- Metzger CE, Swallow EA, Stacy AJ, et al. Adenine-induced chronic kidney disease induces a similar skeletal phenotype in male and female C57BL/6 mice with more severe deficits in cortical bone properties of male mice. PloS One. 2021;16(4):e0250438. doi: 10.1371/journal.pone.0250438
- Aguiar CF, Naffah-de-Souza C, Castoldi A, et al. Administration of α-galactosylceramide improves adenine-induced renal injury. Mol Med. 2015;21(1):553–562. doi: 10.2119/molmed.2014.00090
- Morishita Y, Ohnishi A, Watanabe M, et al. Establishment of acute kidney injury mouse model by 0.75% adenine ingestion. Ren Fail. 2011;33(10):1013–1018. doi: 10.3109/0886022X.2011.618906
- Lee SC, Kim J, La IJ, et al. Characterization of recombinant FAD-independent catabolic acetolactate synthase from Enterococcus faecalis V583. Enzyme Microb Technol. 2013;52(1):54–59. doi: 10.1016/j.enzmictec.2012.10.006
- Xia CH, Han XT, Zhang X, et al. Yiqihuoxue formula activates autophagy and offers renoprotection in a rat model of adenine-induced kidney disease. Evid Based Complement Altern Med. 2019;2019:1–12. doi: 10.1155/2019/3423981
- Wang J, Zhang Q, Jin W, et al. Effects and mechanism of low molecular weight fucoidan in mitigating the peroxidative and renal damage induced by adenine. Carbohydr Polym. 2011;84(1):417–423. doi: 10.1016/j.carbpol.2010.11.055
- Yokozawa T, Nakagawa T, Oya T, et al. Green tea polyphenols and dietary fibre protect against kidney damage in rats with diabetic nephropathy. J Pharm Pharmacol. 2005;57(6):773–780. doi: 10.1211/0022357056154
- Ali BH, Adham SA, Za’abi M A, et al. Ameliorative effect of chrysin on adenine-induced chronic kidney disease in rats. PLoS One. 2015;10(4):e0125285. doi: 10.1371/journal.pone.0125285
- Choi J, Choi MS, Jeon J, et al. In vivo longitudinal 920 nm two-photon intravital kidney imaging of a dynamic 2, 8-DHA crystal formation and tubular deterioration in the adenine-induced chronic kidney disease mouse model. Biomed Opt Express. 2023;14(4):1647–1658. doi: 10.1364/BOE.485187
- Wyngaarden JB, Dunn JT. 8-hydroxyadenine as the intermediate in the oxidation of adenine to 2, 8-dihydroxyadenine by xanthine oxidase. Arch Biochem Biophys. 1957;70(1):150–156. doi: 10.1016/0003-9861(57)90088-7
- Donate-Correa J, Martín-Carro B, Cannata-Andía JB, et al. Klotho, oxidative stress, and mitochondrial damage in kidney disease. Antioxidants. 2023;12(2):239. doi: 10.3390/antiox12020239
- Zaaba NE, Al-Salam S, Beegam S, et al. Catalpol attenuates oxidative stress and inflammation via mechanisms involving sirtuin-1 activation and NF-κB inhibition in experimentally-induced chronic kidney disease. Nutrients. 2023;15(1):237. doi: 10.3390/nu15010237
- Gyurászová M, Gurecká R, Bábíčková J, et al. Oxidative stress in the pathophysiology of kidney disease: implications for noninvasive monitoring and identification of biomarkers. Oxid Med Cell Longev. 2020;2020:1–11. doi: 10.1155/2020/5478708
- Cai H, Su S, Li Y, et al. Protective effects of Salvia miltiorrhiza on adenine-induced chronic renal failure by regulating the metabolic profiling and modulating the NADPH oxidase/ROS/ERK and TGF-β/Smad signaling pathways. J Ethnopharmacol. 2018;212:153–165. doi: 10.1016/j.jep.2017.09.021
- Wang D, Jiang X, Teng S, et al. The antidiabetic and antinephritic activities of auricularia cornea (an albino mutant strain) via modulation of oxidative stress in the db/db mice. Front Immunol. 2019;10:1039. doi: 10.3389/fimmu.2019.01039
- Song X, Pang H, Cui W, et al. Renoprotective effects of enzyme-hydrolyzed polysaccharides from Auricularia polytricha on adenine-induced chronic kidney diseases in mice. Biomed Pharmacother. 2021;135:111004. doi: 10.1016/j.biopha.2020.111004
- Ali BH, Beegam S, Al-Lawati I, et al. Comparative efficacy of three brands of gum acacia on adenine-induced chronic renal failure in rats. Physiol Res. 2013;62(1):47–56. doi: 10.33549/physiolres.932383
- Diwan V, Small D, Kauter K, et al. Gender differences in adenine-induced chronic kidney disease and cardiovascular complications in rats. Am J Physiol Physiol. 2014;307(11):F1169–F1178. doi: 10.1152/ajprenal.00676.2013
- Ali BH, Al-Salam S, Za’abi M A, et al. New model for adenine-induced chronic renal failure in mice, and the effect of gum acacia treatment thereon: comparison with rats. J Pharmacol Toxicol Methods. 2013;68(3):384–393. doi: 10.1016/j.vascn.2013.05.001
- Hamdy MM, Abdel-Rahman MS, Badary DM, et al. Effects of furosemide and tadalafil in both conventional and nanoforms against adenine-induced chronic renal failure in rats. Eur J Med Res. 2022;27(1):1–17. doi: 10.1186/s40001-022-00747-3
- Zhang X, Yang Z, Li L, et al. Prevention of injury by resveratrol in a rat model of adenine-induced chronic kidney disease. Trop J Pharm Res. 2017;16(8):2027–2032. doi: 10.4314/tjpr.v16i8.37
- Rakha MK, Tawfiq RA, Sadek MM, et al. Neurotherapeutic effects of bee venom in a rotenone-induced mouse model of Parkinson’s disease. Neurophysiol. 2018;50(6):445–455. doi: 10.1007/s11062-019-09777-w
- Son DJ, Lee JW, Lee YH, et al. Therapeutic application of anti-arthritis, pain-releasing, and anti-cancer effects of bee venom and its constituent compounds. Pharmacol Ther. 2007;115(2):246–270. doi: 10.1016/j.pharmthera.2007.04.004
- Bae S, Gu H, Gwon MG, et al. Therapeutic effect of bee venom and Melittin on skin infection caused by Streptococcus pyogenes. Toxins (Basel). 2022;14(10):663. doi: 10.3390/toxins14100663
- Dantas CG, da Paixão AO, Nunes TLGM, et al. Africanized bee venom (Apis mellifera Linnaeus): neuroprotective effects in a Parkinson’s disease mouse model induced by 6-hydroxydopamine. Toxics. 2022;10(10):583. doi: 10.3390/toxics10100583
- Kim JY, Jang HJ, Leem J, et al. Protective effects of bee venom-derived phospholipase A2 against cholestatic liver disease in mice. Biomedicines. 2021;9(8):992. doi: 10.3390/biomedicines9080992
- Zahran F, Mohamad A, Zein N. Bee venom ameliorates cardiac dysfunction in diabetic hyperlipidemic rats. Exp Biol Med (Maywood). 2021;246(24):2630–2644. doi: 10.1177/15353702211045924
- An HJ, Kim KH, Lee WR, et al. Anti-fibrotic effect of natural toxin bee venom on animal model of unilateral ureteral obstruction. Toxins (Basel). 2015;7(6):1917–1928. doi: 10.3390/toxins7061917
- Abd El-Wahed AA, Khalifa SA, Sheikh BY, et al. Bee venom composition: from chemistry to biological activity. Stud Nat Prod Chem. 2019;60:459–484.
- Lee WR, Pak SC, Park KK. The protective effect of bee venom on fibrosis causing inflammatory diseases. Toxins (Basel). 2015;7(11):4758–4772. doi: 10.3390/toxins7114758
- Hanafi MY, Zaher EL, ElAdely SE, et al. The therapeutic effects of bee venom on some metabolic and antioxidant parameters associated with HFD‑induced non‑alcoholic fatty liver in rats. Exp Ther Med. 2018;15(6):5091–5099. doi: 10.3892/etm.2018.6028
- El Adham EK, Hassan AI A, Dawoud MM. Evaluating the role of propolis and bee venom on the oxidative stress induced by gamma rays in rats. Sci Rep. 2022;12(1):1–22. doi: 10.1038/s41598-022-05979-1