ABSTRACT
Chronic use of sertraline (SRT) for depression treatment can elevate oxidative stress, potentially leading to neuropathy. Cyperus esculentus extract (CEE) has demonstrated neuroprotective properties against oxidative imbalance, memory impairment, and neural degeneration. Adult male albino rats (40 rats) were placed into four groups: control (GI), CEE (200 mg/kg/day, orally) (GII), SRT (20 mg/kg/day, intraperitoneal) (GIII), and SRT+CEE (GIV). The rats’ spatial learning was assessed using a multiple T-maze after four weeks. Subsequently, rats were euthanized, and brain tissue samples were collected to assess oxidative stress indicators, monoamine oxidase, and dopamine. Brain tissue samples were also examined histologically and immunohistochemically for synaptophysin. SRT significantly increased monoamine oxidase activity, leading to oxidative stress, reduced dopamine levels, and neuronal degeneration in the brain. Treatment with SRT resulted in a decrease in the expression of synaptophysin in the cerebral cortex, dentate gyrus of the hippocampus, and cornu ammonis. These changes, in turn, led to impaired spatial learning. Co-treatment with CEE ameliorated changes in the levels of monoamine oxidase, dopamine, and synaptophysin, restored the redox balance, and improved spatial learning. CEE demonstrated a protective effect against sertraline-induced oxidative damage, preserving neurons, synaptogenesis, and spatial learning. These findings suggest CEE’s potential positive impact on sertraline-related neurodegeneration.
Background
By 2030, depression, driven by lifestyle changes, genetics, and mental stress, is set to affect 350 million people globally, becoming the leading global health burden [Citation1]. This neuropsychiatric disorder, attributed to brain structural changes impacting emotion, mood, and cognition, poses a significant challenge by causing disability and contributing significantly to disease burden and suicide rates [Citation1–3].
Antidepressants treat depression in four classes: tricyclics, serotonin noradrenaline reuptake inhibitors, monoamine oxidase inhibitors, and serotonin reuptake inhibitors (SSRIs) regulating brain neurotransmitters for mood improvement [Citation1]. SSRIs are believed to cause genotoxic effects by elevating intracellular reactive oxygen species and oxidative stress, leading to DNA damage and cell toxicity [Citation4].
Sertraline (SRT), an SSRI, is used for conditions like major depression, social anxiety, obsessive-compulsive disorder, panic, and post-traumatic stress disorder [Citation1]. SRT boosts brain serotonin, enhancing mood and lowering mental health symptoms. It can help many people, but it may have negative effects and not work for everyone. Adverse effects of SSRIs include headaches, cognitive impairment, gastrointestinal discomfort, diarrhea, sexual dysfunction, anxiety, anorexia, weight loss, palpitations, and the rare but severe serotonin syndrome [Citation5,Citation6]. SRT administration damages mitochondrial membranes, generating ROS and oxidative stress, which disrupts antioxidant enzyme activity and causes cellular toxicity [Citation7,Citation8].
Recently, a case study highlighted the occurrence of neurological deficits, intraparenchymal hemorrhage, and seizures linked to an increase in SRT dosage, with symptom resolution upon discontinuation of the medication [Citation9]. Reports of Parkinsonian symptoms have emerged in patients using SRT for depressive symptoms [Citation10]. Studies in zebrafish [Citation11] and yellow catfish [Citation12] have highlighted the neurotoxic implications of prolonged SRT exposure. Lozano et al. [Citation13] have documented adverse impacts on the neurobehavioral development of rat offspring following prenatal exposure to sertraline. These findings suggest that SRT may have potentially neurotoxic effects, leading to various neurological symptoms and disorders. These cumulative findings underscore the potential risks of prolonged SRT usage, affecting both physical and neurological health. Consequently, further research is imperative to assess these risks and formulate effective mitigation strategies comprehensively.
Cyperus esculentus (Tiger nut) is a sweet, almond-like, nutritious tuber from North Africa valued for its health benefits [Citation14,Citation15]. In different regions and languages, it goes by names such as ‘Akiausa’ or ‘Offio’ in Igbo, ‘Aya’ in Hausa, ‘chufa’ in Spanish, ‘Imumu’ in Yoruba [Citation15], and “Hab El ‘Aziz’ in Egypt [Citation16].
Cyperus esculentus‘s edible part contains carbohydrates, protein, lipids (sterols, oleic acid, palmitic acid, and linoleic acid), flavonoids, quercetin, tannins, and saponins, as well as dietary minerals (calcium, zinc, potassium, iron, magnesium, and phosphorus) and vitamins (A, C, and E) [Citation16,Citation17] which offer protection against oxidative stress and inflammation.
Cyperus esculentus can treat mood disorders, migraine headaches, insomnia, cancer, cardiovascular issues, diabetes, bowel and liver disorders, urinary tract infections, atherosclerosis, and hypercholesterolemia, as well as as an anti-microbial [Citation15,Citation17,Citation18]. It contains neuroprotective antioxidant and anti-inflammatory compounds that can improve cognitive function and memory [Citation16].
Tiger nut milk, a refreshing beverage made from tiger nut tubers and water, is rich in carbs, fats, proteins, minerals, and vitamins (C and E) for digestion [Citation19]. It is loaded with oleic acid, which aids in preventing cardiac issues [Citation20]. The water extract yielded higher amounts of phenolic and flavonoid compounds compared to methanol, indicating a higher concentration of water-soluble phytochemicals in tiger nuts [Citation21]. Cyperus esculentus water extract is rich in alkaloids, tannins, saponins, glycosides, flavonoids, and steroids [Citation22]. Consuming 300 ml/day of raw tiger nuts milk has positive effects on gut microbiota [Citation23]. Tiger nuts contain medicinal compounds that provide anti-inflammatory, antibacterial, anti-tumor, and anti-cancer properties, making them a safe choice for individuals of all ages [Citation24].
The research article aims to investigate the potential neuroprotective properties of Cyperus esculentus water extract (CEE) concerning learning, memory, brain biochemistry, cellular morphology, and synaptogenesis in rats undergoing chronic sertraline (SRT) treatment.
Methods
Chemicals
Sertraline purchased from Besitran© Pfizer S.A. Madrid, Spain (25-mg tablet), was utilized along with MDA, SOD, and CAT assay kits (Biodiagnostic, Giza, Egypt; Catalog No. MD 25 29, SD 25 21, CA 25 17, respectively) for conducting biochemical analyses. Moreover, the Total Antioxidant Capacity Kit (Abcam; Catalog No. ab65329), Monoamine Oxidase ELISA Kit (CUSABIO; Catalog No. CSB-E15076r, Wuhan, China), and Dopamine ELISA Kit (DOU39-K01 Eagle Biosciences, Nashua, NH), were employed in the study’s procedures.
Preparation of Cyperus esculentus extract
The fresh tubers of Cyperus esculentus were sourced from a vendor at a local market in Alexandria, Egypt. After carefully removing any impurities from the tubers, they were allowed to air-dry in shaded conditions. A total of 300 g of air-dried tubers were finely powdered. This powder was immersed in 1000 ml of purified water at a temperature of 4°C. After 72 hours of soaking, the extract was separated through sieving and filtration using filter paper (Whatman No.1). The filtrate was dried in a 40°C water bath for 96 hours to obtain a concentrated extract, which was stored at 4°C and diluted as required [Citation15]. The yield was 26.6 ± 0.6 g/100 g sample.
Experimental design
Adult male albino rats (Rattus norvegicus), aged 12 weeks and weighing 154–166 grams, were procured from the Faculty of Medicine’s animal house at Alexandria University, Egypt. These rats were accommodated in plastic-wired cages and maintained under specified guidelines, including a temperature range of 21–24°C and a natural light-dark cycle. Throughout the study, the rats were given regular rodent food and unrestricted access to filtered water. Stringent care protocols were followed for the handling and management of the rats in fulfillment of the regulations of the Ethics Committee of Scientific Research (DMU-SCI-CSRE-23-06-04).
Seven days after acclimatization, the rats were randomly split into four groups (n = 10/group). For the next 4 weeks, rats were treated as follows: Group I was the control group, underwent no drug interventions, and only received 0.5 ml/rat/day of distilled water (i.p). Group II received 200 mg/kg b.w./day of Cyperus esculentus extract (CEE) orally administered diluted in distilled water [Citation25].
Group III (chronic SRT treatment model) rats were subjected to an intraperitoneal injection of 20 mg/kg b.w/day of sertraline (Besitran© Pfizer S.A. Madrid, Spain, 25-mg tablet), dissolved in distilled water [Citation26]. Group IV, labeled as SRT + CEE, was intraperitoneally injected with 20 mg/kg b.w./day SRT along with 200 mg/kg b.w./day CEE through gastric gavage.
At the culmination of the experiment, between 9 am and 11 am, rats fasting overnight underwent an evaluation of spatial learning using a multiple T-maze, followed by determining the weight change by calculating the difference between a rat’s final body weight and its initial body weight. Then, rats under anesthesia (60 mg/kg sodium pentobarbital) were euthanized via decapitation. Brains were dissected, washed, and allocated: one set for homogenized in chilled phosphate-buffered saline (10% w/v) with 0.16 mg/ml of heparin and then spun in a centrifuge at 4°C for 15 minutes at 4,000 rpm. For a neurochemical study, the supernatants were kept at −20°C. The other set is for histopathology and immunohistochemistry.
Investigated parameters
Behavior assessment of spatial learning by multiple T-mazes
Experimental setup and procedure
The Multiple T-maze (MTM) was used to test the rats’ spatial learning and memory by testing their ability to find a target box with food pellets by remembering which arms they had already explored [Citation27]. The MTM had a wooden platform with seven different choices. Each choice point was 12 cm long, 20 cm wide and 11 cm high. This design enabled different possible exits, allowing for left and right choices ().
Experimental protocol
After completing the designated study period, rats were subjected to an 18-hour food deprivation period to enhance their motivation for food exploration. The rats’ ability to learn new spatial locations was measured by how long it took them to navigate from a predetermined starting point inside the MTM to the reward chamber. Each trial ended with thoroughly cleaning the entire maze with a 70% ethanol solution to maintain standardized conditions for future tests.
Biochemical analysis of brain tissue
Assessment of malondialdehyde and antioxidant
As a marker of lipid peroxidation, the concentration of malondialdehyde (MDA) in brain tissue was measured colorimetrically using an MDA kit. The assessment was conducted using the thiobarbituric acid method, following the procedure outlined by Ohkawa et al. [Citation28]- using the formula: MDA level (nmol/g tissue) = (Sample Absorbance/Standard Absorbance) x 10/(grams of tissue used), absorbance measurements at 534 nm.
Brain tissue total antioxidant capacity (TAC) was assessed by a commercially available kit following the provided protocol based on Cu2+ to Cu+ ion conversion involving endogenous antioxidants [Citation29]. Using formal: TAC = (TAC amount determined from the standard curve in the sample well)/(volume of the sample added to the sample wells) × sample dilution, absorbance measurements at 570 nm.
Catalase (CAT) activity in brain homogenate was assessed following the Aebi [Citation30] method using a biodiagnostic kit, with CAT activity expressed as mmoles of H2O2 consumed per minute/milligram of protein using the formula: CAT Activity (U/g) = ((standard absorbance – sample absorbance)/(standard absorbance) x 1/(gram of tissue used)), absorbance measurements at 510 nm.
Using the method by Nishikimi et al. [Citation31], superoxide dismutase (SOD) activity was assessed using biodiagnostic kit, which relies on SOD’s ability to inhibit the reduction of nitroblue tetrazolium dye by phenazine methosulphate – using the formula: SOD Activity (U/g) = % Inhibition × 3.75 × 1/(gram of tissue used), absorbance measurements at 560 nm.
Quantifying monoamine oxidase
Monoamine oxidase (MAO) activity was quantified using the ELISA method, following the Jiang et al. [Citation32] protocol. The supernatant of brain tissue lysates was introduced onto ELISA plates that had been pre-coated with anti-MAO antibodies. Subsequently, biotin-conjugated horseradish peroxidase was added. After a washing step to eliminate any unbound biotin-enzyme reagent, a solution of 3,3′,5,5′-Tetramethylbenzidine (TMB) was introduced to the wells, leading to the development of color, the reaction of which was then halted. At 450 nm, color intensity was measured, and the data were calculated and expressed as mu/g.tissue.
Quantifying dopamine
The assessment of dopamine concentrations was carried out using a commercial ELISA kit designed for rat/mouse dopamine detection. The procedure followed the manufacturer’s instructions and protocol. Brain samples, which were pre-coated with primary antibodies specific to DA, were introduced into the wells of ELISA plates. Subsequently, anti-rabbit IgG-POD conjugate/peroxidase was added and allowed to interact for 60 minutes. Next, TMB solution was added to the plates, which were then gently agitated on an orbital shaker at room temperature for 35 to 45 minutes; then, a stop solution was added to halt the reaction. The measured absorbance at 450 nm was utilized to calculate the amount of antibody bound to the solid-phase DA. This value was then correlated inversely with the dopamine concentration within the samples. The DA levels were expressed as pg/g tissue.
Brain histological examination by H&E
Following 24 hours of formalin fixation, the brain tissues underwent a series of preparation steps. The tissues were gradually dehydrated through an ascending sequence of ethanol concentrations and cleared in xylene before being embedded in paraffin. Brian paraffin blocks were sectioned and stained with hematoxylin and eosin (H&E) [Citation33] and inspected using an Olympus C × 31microscope (Japan). Photomicrographs (40X) were analyzed using ImageJ 1.47 V software.
Immunohistochemical labeling of synaptophysin
Paraffin sections (4 μm) on Fisher Thermo Scientific slides were processed: deparaffinized, rehydrated, and subjected to a 10-minute blocking step to inhibit endogenous peroxidase activity. At 4°C, brain tissue sections were incubated overnight with mouse/rat anti-synaptophysin antibody (1:50, M7315, Dako), followed by horseradish peroxidase-conjugated secondary antibody and 3,3-diaminobenzidine (DAB; Sigma, Germany) development. After counterstaining with hematoxylin, sections were dehydrated and mounted using Canada balsam. Synaptophysin expression was quantified in 40× magnification IHC-labeled sections using IHC Profiler, an ImageJ plugin following the Varghese et al. [Citation34] method.
Statistical analysis
Prism8 software (GraphPad Software, San Diego, CA, USA) was used to evaluate group differences using one-way ANOVA and Tukey’s test, with significance at p < 0.05. The data is expressed as the mean ± SD.
Results
Changes in spatial learning
In the MT-maze, the time rats took to locate rewards was calculated and analyzed. The results are represented in . Administration of CEE (GII) insignificantly raised the rat’s spatial learning (p > 0.05), as demonstrated by a decline in the period spent navigating the maze in comparison to the control group (GI). Conversely, SRT (GIII) significantly hindered the exploring speed of rats, resulting in impaired spatial learning. This effect was evidenced by prolonged passage times in comparison to both the control group (G I) (p < 0.01) and the CEE-treated group (GII) (p < 0.001). However, combined SRT and CEE treatment (IV) significantly suppressed SRT (p < 0.01), hindering the behavioral response of rats. This effect was marked by a non-significant increase in maze navigation time recorded (p > 0.05) compared to the control groups (GI & GII).
Figure 2. Impact of sertraline (SRT) and Cyperus esculentus extract (CEE) treatment on spatial learning. Sertraline slows down rat exploration to the MT-maze, while CEE speeds up rat exploration to the MT-maze in comparison to control and SRT – treated rats. Bars (mean ± SD) labeled with different letters indicate significant differences: asignificant variation with the control group, bwith the CEE group, cwith the SRT group, and dwith the SRT + CEE group.
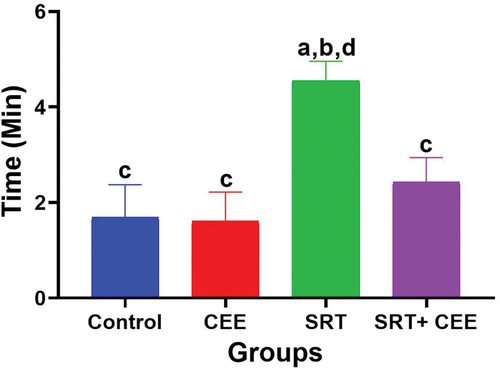
Changes in body weight
illustrates body weight gain percentages across the experimental groups. The administration of CEE (GII) alone is associated with a significant elevation in body weight gain percentage compared to other experimental groups (p < 0.001). The effect of SRT alone (GIII) on body weight gain was statistically significant (p < 0.05), indicating that SRT may have an impact on weight gain in this study. However, when CEE is combined with SRT (GIV), the effect on body weight gain % becomes less pronounced and is not statistically different from the control group (GI) or the SRT-treated group (GIII) (p > 0.05).
Figure 3. Impact of sertraline (SRT) and Cyperus esculentus extract (CEE) treatment on the percentage of body weight gain. Bars (mean ± SD) labeled with different letters indicate significant differences: asignificant variation with the control group, bwith the CEE group, cwith the SRT group, and dwith the SRT + CEE group.
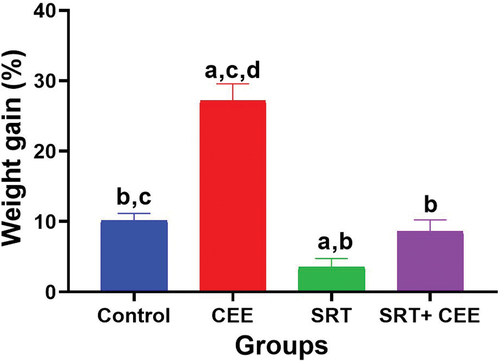
Changes in MDA and antioxidant parameters
The assessment of oxidative stress and antioxidant markers in brain tissue is presented in . Data revealed that the administration of CEE (GII) did not prompt any significant changes in TAC, MDA levels, and CAT activity; however, it caused a significant decline in SOD activity (p < 0.05). In contrast, SRT (GIII) significantly elevated MDA level and lowered TAC level, SOD, and CAT activity (p < 0.001) compared to controls (GI & GII), indicating heightened oxidative stress. The treatment with SRT and CEE (GIV) led to a significant increase in MDA level and a decrease in TAC level, SOD, and CAT activities (p < 0.001) compared to the control groups (GI&GII). In contrast, SRT and CEE (GIV) treatment significantly reduced MDA levels and elevated TAC level, SOD, and CAT activities (p < 0.001) compared to the SRT-treated group (GIII).
Figure 4. Impact of sertraline (SRT) and Cyperus esculentus extract (CEE) treatment on oxidative stress in rat’s brains: (a) malondialdehyde (MDA) level, (b) total antioxidant capacity (TAC) level, (c) catalase (CAT) and (d) superoxide dismutase (SOD) activity. Sertraline increased MDA and reduced TAC, CAT, and SOD, while CEE prevented the increase in MDA and decrease in TAC, CAT, and SOD in SRT-treated rats. Bars (mean ± SD) labeled with different letters indicate significant differences: asignificant variation with the control group, bwith the CEE group, cwith the SRT group, and dwith the SRT + CEE group.
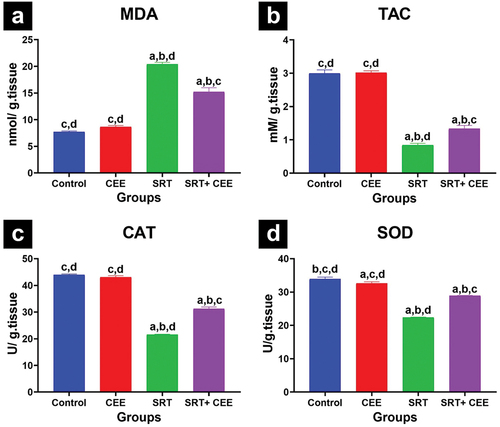
Changes in MAO activity and dopamine levels
illustrate the impact of the treatments on MAO activity and dopamine levels in the rat brain. Treatment with CEE alone (GII) led to a non-significant elevation in MAO activity and a significant elevation in dopamine levels (p < 0.05). On the contrary, SRT (GIII) caused significantly elevated MAO activity and reduced dopamine levels (p < 0.001) compared to controls (GI & GII). This effect indicates a disruption in neurotransmitter balance. The administration of SRT and CEE (GIV) resulted in a significant elevation in MAO activity, coupled with significant reductions in dopamine (p < 0.001) when compared to the control groups (GI & GII). Notably, SRT +CEE treatment (GIV) significantly counteracted SRT’s effects by preventing the elevation in MAO activity and the reduction in dopamine levels (p < 0.001) compared to the SRT-treated group (GIII).
Figure 5. Impact of sertraline (SRT) and Cyperus esculentus extract (CEE) treatment on neural markers in rat brains. (a) Monoamine oxidase (MAO) activity, and (b) dopamine levels in brain tissue. Sertraline increased MAO and reduced dopamine levels, while CEE prevented the increase in MAO and decrease in dopamine in SRT-treated rats. Bars (mean ± SD) labeled with different letters indicate significant differences: asignificant variation with the control group, bwith the CEE group, cwith the SRT group, and dwith the SRT + CEE group.
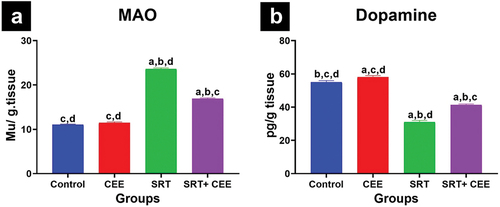
Histological and morphometric analysis of brain tissue
Cerebral cortical
Histological micrographs of the control group (GI) cortical tissues stained with H & E demonstrated normal proportions and architectures of neurons, glial cells, and blood capillaries scattering in the neuropil. A pink eosinophilic fibrillar homogenous neuropil, formed from neuronal and glial cell processes, was evident, with rounded non-pyramidal neuron cells displaying open-faced nuclei with prominent nucleoli. Oligodendrocytes and microglial cells were identified as two forms of glial cells. Oligodendrocytes exhibited dark nuclei and clear unstained cytoplasm, while microglial cells were smaller with small, deeply stained, or pale stained nuclei (). The CEE-treated group (GII) displayed a cortical tissue pattern similar to controls ().
Figure 6. Impact of sertraline (SRT) and Cyperus esculentus extract (CEE) treatment on cerebral cortex structure. (a & b) control and CEE-treated rats sections show healthy neurons (N), microglia cells (MG), oligodendrocytes (OL), and blood capillaries (BC) in a neuropil-rich environment (NP). (c) SRT-treated rats section shows degenerating neurons (DN) with pericellular halos (H), increased microglial cells (black circle), and neuropil spongiosis (black star). (d) SRT+CEE-treated rats section displays numerous normal neurons (N), some degenerating neurons (DN), and limited neuropil spongiosis (blue star). (H&E, A-D × 400). (e) mean number of viable neurons, degenerating neuron (DN), oligodendrocytes (OL), and microglial cells (MG) in the cerebral cortex of studied groups. Bars (mean ± SD) labeled with different letters indicate significant differences: asignificant variation with the control group, bwith the CEE group, cwith the SRT group, and dwith the SRT+CEE group.
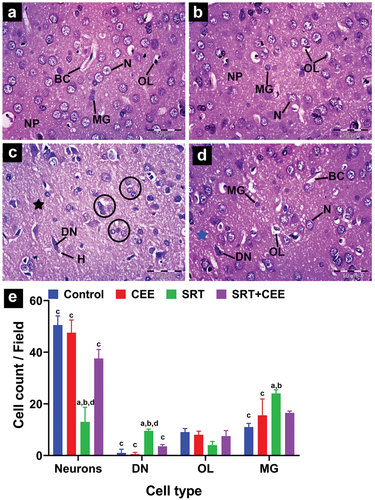
SRT-treated rats (GIII) exhibited disrupted proportions and architecture of cerebral cortical tissues, significantly increasing neuronal degeneration, loss of neuropil homogeneity, vacuolations, and hydropic changes (spongiosis). Degenerating neurons appeared as ghost masses with deeply stained nuclei and acidophilic cytoplasm surrounded by clear pericellular halos marking apoptosis surrounded by microglial cells (). This group showed an insignificant reduction in the number of oligodendrocytes alongside a significant elevation in the number of degenerating neurons and microglial cells (p < 0.05) in comparison to control groups (GI&GII) ().
The cortical architecture was mildly affected in the SRT+CEE-treated group (GIV), with a nearly regular cell arrangement and number. Neurons displayed vesicular nuclei, nucleoli, and a few dark-stained pyknotic nuclei (). The number of viable neurons significantly increased (p < 0.01), and the number of degenerating neurons insignificantly decreased compared to the SRT-treated group (). The SRT+CEE group showed minor changes in oligodendrocyte and microglial cell numbers (p > 0.05) compared to control groups (GI&GII), along with less pronounced vacuolation and hydropic changes in the neuropil ().
Hippocampus
In different experimental groups, hippocampus cells form Cornu amonus (CA) and dentate gyrus. Based on pyramidal cell density and size, the CA has four cornu amonus regions along its length. CA1, CA3, and dentate gyrus were chosen for histopathological studies ().
Figure 7. Impact of sertraline (SRT) and Cyperus esculentus extract (CEE) treatment on hippocampus CA1 and CA3 regions. (a-d) control and CEE-treated rats sections display organized CA1 and CA3 regions with normal pyramidal cell layer (PCL), microglia (MG), astrocytes (AST), and blood capillaries (BC) in polymorphic (POL) and inner molecular (ML) layers. (e-f) SRT-treated rats sections show disarranged PCL in both CA1 and CA3 regions with darkly stained degenerating neurons (DN) surrounded by pericellular holes (curved arrow), those neurons surrounded by an increased number of microglial cells (head arrows), few neurons have lightly stained dissoluted nucleus (arrow) and clumped processes (star) in ML. (g-h) sections from SRT+CEE-treated rats show a regular PCL layer in CA1 with a few degenerating neurons (DN), while CA3 exhibits mild neural degeneration. (H&E, A-H × 400). (i&j) mean CA1 and CA3 pyramidal layer thickness. Bars (mean ± SD) labeled with different letters indicate significant differences: asignificant variation with the control group, bwith the CEE group, cwith the SRT group, and dwith the SRT+CEE group.
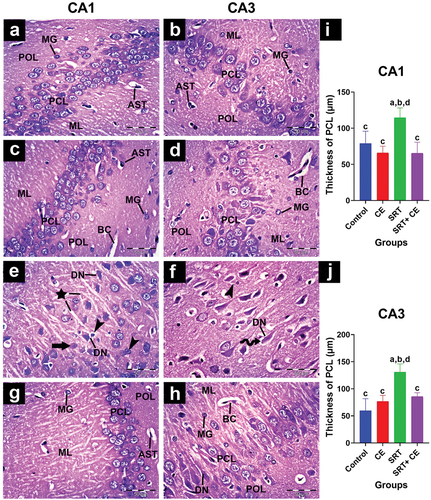
Control group: CA1 and CA3 cells organized into polymorphic, molecular, and pyramidal layers (). Neuropils in polymorphic and molecular layers surround capillaries, star-shaped astrocytes, and small oval microglial cells. In the pyramidal cell layer, cells have large, round nuclei with prominent nucleoli and limited cytoplasm. In CA1, smaller, packed cells are observed in three to six rows; in CA3, fewer, larger, loosely packed cells are observed in two to four rows of pyramidal cells. In the CEE-treated group (GII), the hippocampal tissue showed normal cytoarchitecture of Cornu amonus regions CA1 and CA3, similar to the control group ().
SRT injection (GIII) caused neural degeneration marked by cellular damage and layer disorganization. In the CA1 and CA3 regions, the pyramidal cells layer exhibited disorganized rows; most pyramidal cells appeared as dark, ghost-shaped cells with deeply eosinophilic cytoplasm surrounded by pericellular halos, marking neuron apoptosis ( and ). Numerous microglial cells and a few astrocytes were observed in the polymorphic and molecular layers. Clumping of neuronal processes was seen projecting into the molecular layer (). The thickness of the CA1 and CA3 pyramidal cell layers was significantly elevated (p < 0.001) () compared to the control groups (GI&GII).
Histological examination of SRT + CEE-treated group (GIV) sections revealed a mild attenuation of SRT neurodegeneration as evidenced by the regular cellular organization and thickness of the CA1 and CA3 pyramidal cell layers (p < 0.01) (). In the CA1 region, most pyramidal cells have large vesicular nuclei with dispersed chromatin, prominent nucleoli, and sparse basophilic cytoplasm (). Typical amounts of microglial cells and astrocytes are in the polymorphic and molecular layers. At the same time, the CA3 region showed a higher number of degenerating neurons than the CA1 region ().
The dentate gyrus of the control group appeared to be made up of a molecular, polymorphic layer in between the granular layer (). Both molecular and polymorphic layers mainly comprise neuropils that harbor a few microglial cells and astrocytes. The granular layer comprises four to ten rows of closely packed small granule cells with round, vesicle-shaped nuclei and thin cytoplasm. These cells are closely arranged to form the upper and lower limbs of the dentate gyrus, with the lower limb having a thicker layer than the upper limb. In addition, the sub-granular zone included spindle-shaped immature cells that were small, black, and varied in size. These cells were found in a relatively close space between the hilus and the granular layer. A similar cytoarchitecture of the dentate gyrus was observed in the CEE-treated group ().
Figure 8. Impact of sertraline (SRT) and Cyperus esculentus extract (CEE) on hippocampus dentate gyrus (DG) upper and lower limb. (a-d) control and CEE-treated rats sections display DG limbs with an organized granular cell layer (GCL) containing normal granule cells with small rounded nuclei separated from the hilus by a narrow sub-granular zone (SGZ). The dentate molecular layer (DML) and dentate pleomorphic layer (DOL) contain microglial cells (MG) and astrocytes (AST). (e-f) SRT-treated rats sections display a thin GCL with numerous degenerative neurons (DN) characterized by shrunken, darkly stained nuclei surrounded by clear pericellular holes (curved arrow) and clumped microglial cells (head arrow). SGZ is absent. (g-h) SRT-CEE treated rat’s sections show an apparently normal GCL with normal neurons and an evident SGZ area in the upper DG limb only. (H&E, A-H × 400) (i&j) mean granular cell layer of DG limbs thickness. Bars (mean ± SD) labeled with different letters indicate significant differences: asignificant variation with the control group, bwith the CEE group, cwith the SRT group, and dwith the SRT+CEE group.
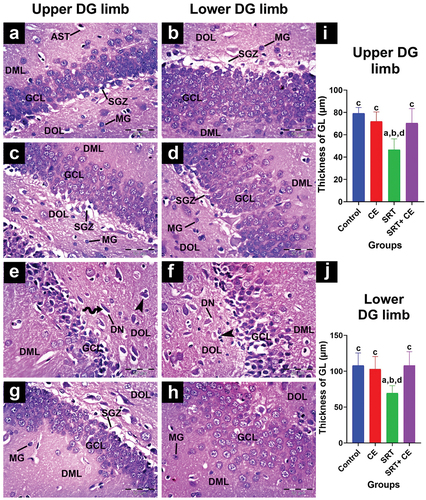
In SRT-treated rats, the dentate gyrus granular layer cells appeared as dark, shrunken ghost-shaped cells surrounded by pericellular halos surrounded by numerous microglial cells (). Sub-granular zones in the dentate gyrus were ill-defined. There was a significant decrease in the granular layer thickness in dentate gyrus limbs (p < 0.01) compared to the control group (GI) ().
Moreover, in the SRT+CEE-treated group (GIV), the upper limb of dentate gyrus limbs showed normal granular cell morphology and organization. Few cells appeared shrunken with irregular outlines interspersed among them, while the number of microglial cells within the sub-granular zone remained normal (). In contrast, the lower DG limb showed more degenerating neurons and a narrow sub-granular zone. The thickness of the granular layer was insignificantly decreased (p > 0.05) compared to the control groups (GI&GII) () and significantly increased (p < 0.01) compared to the SRT-treated group (GIII).
Immunohistochemical observation of synaptophysin
Immunostaining of synaptophysin (SYP) is used as a marker for synaptogenesis. Data analysis, along with representative micrographs, is displayed in . The cerebral cortex and hippocampus regions CA1, CA3, and dentate gyrus limbs showed strong synaptophysin reactions in the control and CEE groups. The administration of SRT resulted in reduced synaptogenesis, as indicated by a significant decrease in SYP protein expression levels observed in the cortex (p < 0.001), hippocampal regions CA1 and CA3 (p < 0.01), as well as dentate gyrus limbs (p < 0.05) compared to the control groups (GI and GII).
Figure 9. Impact of sertraline (SRT) and Cyperus esculentus extract (CEE) treatment on synaptogenesis in rat’s brain cortex region. (a) Representative micrographs of SYP immunostained cerebral cortex (X 400). (b) Mean expressional levels of SYP in immunostained sections. Bars (mean ± SD) labeled with different letters indicate significant differences: asignificant variation with the control group, bwith the CEE group, cwith the SRT group, and dwith the SRT+CEE group.
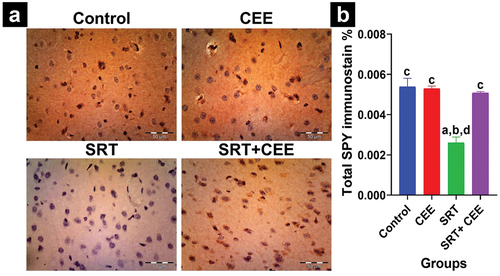
Figure 10. Impact of sertraline (SRT) and Cyperus esculentus extract (CEE) treatment on synaptogenesis in rat’s brain hippocampus. (a) Representative micrographs of SYP hippocampus regions (CA1, CA3) (X400). (b) Mean expressional levels of SYP in immunostained sections. Bars (mean ± SD) labeled with different letters indicate significant differences: asignificant variation with the control group, bwith the CEE group, cwith the SRT group, and dwith the SRT+CEE group.
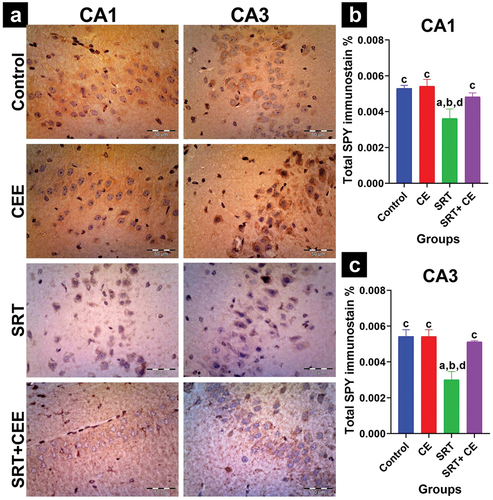
Figure 11. Impact of sertraline (SRT) and Cyperus esculentus extract (CEE) treatment on synaptogenesis in rat’s brain dentate gyrus. (a) Representative micrographs of SYP immunostained dentate gyrus upper limb and lower limb (X400). (b) Mean expressional levels of SYP in immunostained sections. Bars (mean ± SD) labeled with different letters indicate significant differences: asignificant variation with the control group, bwith the CEE group, cwith the SRT group, and dwith the SRT+CEE group.
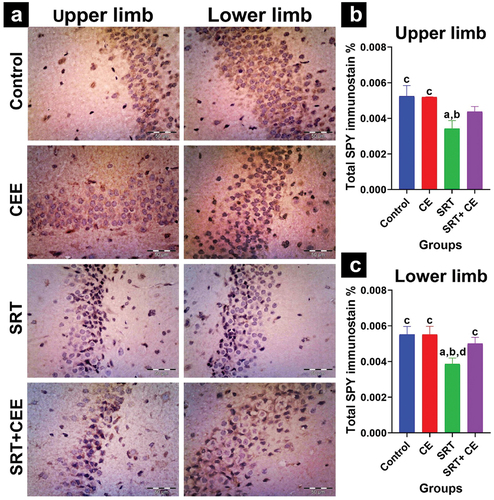
The study found that co-treatment with SRT and CEE significantly increased SYP protein expression levels in the cortex (p < 0.001), hippocampal CA1 (p < 0.05), and CA3 (p < 0.01) regions compared to the SRT- group (GIII). Notably, this co-treatment caused an insignificant reduction in expression levels compared to the control groups (GI and GII) for the same regions ( and ).
However, the SRT+CEE-treated group showed no significant increase in SYP expression in the upper DG limb and a significant increase in SYP expression in the lower DG limb (p < 0.05) compared to the SRT group. This group (GIV) also showed an insignificant reduction in SYP expression in both DG limbs compared to the control groups (GI&GII) ().
Discussion
Cyperus esculentus supplementation has garnered attention for its potential as a versatile intervention in addressing various health issues. Previous research has suggested its efficacy in managing conditions such as memory enhancement, age-related cognitive decline, atherosclerosis, migraines, insomnia, and mood disorders [Citation18]. Cyperus esculentus extract (CEE), known for its antioxidant, anti-inflammatory, and neuroprotective properties, is believed to hold promise in ameliorating memory-related disorders [Citation16]. Most of CEE’s neuroprotective properties come from its high antioxidant content, which is critical in minimizing the damaging impacts of free radicals in the brain environment [Citation15]. Prior studies have established the neurotoxic and neurobehavioral consequences of prolonged exposure to sertraline (SRT) in both fish and rat models [Citation11–13]. Given the potential neuroprotective effects of CEE, it is worth investigating whether it can mitigate the adverse effects of SRT on memory and cognitive function in animal models.
In this study, SRT administration led to significant weight loss. Previous studies have shown that SRT can reduce weight gain in animal models without affecting appetite group [Citation2]. This suggests that SRT’s impact on weight loss may be independent of appetite regulation.
Increased reactive oxygen species (ROS) in neurons and glial cells can cause macromolecular peroxidation, mitochondrial dysfunction, apoptosis, and neuronal damage [Citation35]. The normal metabolism of cells produces highly reactive ROS. When their levels become elevated, they can overwhelm the antioxidant defense systems of cells, thereby contributing to oxidative stress [Citation36]. This cascade of events has been linked to the development of neurodegenerative conditions and cognitive decline [Citation35]. Notably, chronic SRT consumption has been linked to heightened oxidative stress, potentially resulting in organ toxicity, including in the testes [Citation7], heart [Citation37], and liver [Citation38]. Antidepressants, such as sertraline, impact various neurotransmitters, including dopamine, by acting on monoamine receptors and associated proteins [Citation39].
Monoamine oxidase (MAO), a brain enzyme, is vital in making ROS and catalyzing dopamine’s oxidative deamination [Citation40]. Louei Monfared and Yousefizadeh [Citation7] stated that increased ROS generation mediates SRT-induced testicular toxicity. The data analysis revealed that SRT-induced MAO and ROS represent a state of oxidative damage within the brain. The significant increase in MAO and MDA levels and a significant decrease in the antioxidative defense markers SOD, CAT, and TAC support this conclusion. These changes in antioxidant enzymes can render the brain susceptible to further oxidative damage [Citation10]. This finding aligns with earlier research reporting that elevated MAO activity can increase neuronal ROS levels in Alzheimer rats model [Citation35].
Similarly, Arihan et al. [Citation41] reported that the administration of SRT (20 mg/kg) for 21 days triggered a significant increase in the levels of MDA. In a previous study, SRT treatment increased MDA and H2O2 production and decreased antioxidants like SOD, which may affect serotonin receptors, neuronal membranes, and serotonergic function [Citation42]. Disturbances in antioxidant enzyme activities are correlated with the peroxidation reactions of phospholipids in brain cells, contributing to brain damage and neurode-generation [Citation43]. These findings suggest that the administration of SRT may lead to a redox imbalance and oxidative damage in the brain tissue.
Neurodegeneration is the gradual impairment of the structure or function of neurons, which can contribute to a decline in cognition and memory skills [Citation16]. Along with the hippocampus, the cerebral cortex is an essential part of the brain that is needed for learning and remembering [Citation35]. Histopathological analysis of the SRT-treated group’s cerebral cortex and hippocampus sections demonstrated signs of neurodegeneration. The signs of neural degeneration identified included ghost-like neurons that were stained darkly and surrounded by perineuronal spaces, an elevated number of degenerated neurons, and microglial cells that were surrounded by vacuolated neuropils. These traits were present in both the cortical and hippocampal regions. Additionally, signs of disorganized pyramidal cell layers of CA1 and CA3 and a reduction in the granular layer’s thickness in the hippocampus’s DG limb regions were observed.
Several studies found that increased lipid peroxidation (MDA) and lower antioxidants (CAT, SOD) provoked oxidative stress, causing cortical and hippocampus neural degeneration, nuclear condensation and pyknosis (neuron apoptosis), neuron necrosis, neuropils vacuolation, increased glial cells to engulf apoptotic neurons, and impaired brain function [Citation44–47].
In the Alzheimer rat model, decreased synaptic dysfunction has been linked to redox imbalance (↑MDA, ↓GSH) [Citation48]. In synapses, many receptors, channels, and functioning proteins like synaptophysin help form synapses, control synaptic plasticity, and discharge neurotransmitters [Citation49]. Synaptophysin (SYP), a major synapse protein, plays a crucial role in neurotransmission, serving as a marker for synaptic plasticity, vesicle production, and the release of neurotransmitters [Citation50,Citation51]. The current study’s immunostain data analysis revealed low SYP expression levels in SRT-treated rats, marking decreased synaptogenesis. Previous studies suggested that oxidative damage and membrane disruption contribute to the neurodegenerative processes in the cortex and hippocampus (neuron apoptosis and increased number of glial cells), leading to synaptic loss by reduced SYP expression [Citation35,Citation50,Citation52]. These findings point to an SRT-induced redox imbalance, leading to neurodegeneration and a reduction in synaptogenesis.
Abdel-Salam et al. [Citation10] reported that SRT treatment induced oxidative stress, decreased acetylcholinesterase, and alternated cholinergic neurotransmission. Oxidative damage can potentially disrupt essential neurotransmitter systems, such as dopamine, and impact overall neuronal structure and function. In the current study, SRT treatment resulted in a decline in dopamine levels, which can be attributed to two possible mechanisms. The first mechanism related to the increase in MAO activity could potentially lead to higher levels of dopamine breakdown, resulting in lower dopamine levels. This finding aligns with earlier research reporting that elevated MAO activity can reduce dopamine levels in the scopolamine Alzheimer rats model [Citation35]. The increased ROS production can lead to impaired dopamine metabolism and ultimately lead to the degeneration of dopaminergic neurons [Citation53]. The second possible mechanism is reduced synaptogenesis, leading to decreased dopamine levels. Altered SYP protein levels can impact dopamine release, possibly contributing to neurological disorders [Citation54], suggesting that there may be a link between MAO, synaptogenesis, and imbalances in neurotransmitters in the brain. The data reported aligns with Kumar et al. [Citation55], who suggested that elevated MAO activity reduces acetylcholine, induces oxidative stress, and causes synaptic and neuronal dysfunction in Alzheimer’s disease.
According to a previous study, selective serotonin reuptake inhibitors like SRT may impair spatial learning [Citation56]. Changes in the levels of neurotransmitters, particularly dopamine, can cause changes in behavior and disrupt motor coordination [Citation36]. The MT-maze behavior test is used as a spatial learning task. Animals have to learn a complicated path to the reward, which shows how well they can remember things in the short term [Citation57]. The MT-maze test data analysis showed that the SRT-treated rats significantly dropped their short-term memory abilities, as shown by increased exploration time in a maze task. Learning deficits on complex spatial tasks like the Morris water maze have also been linked to selective serotonin reuptake inhibitors, as reported by Rad et al. [Citation56].
Earlier studies reported that oxidative stress induces cortical and hippocampal neuron death, leading to a decline in dopamine levels, impaired behavior, cognitive dysfunction, and memory deficits [Citation43,Citation58,Citation59]. Heightened MAO activity can significantly contribute to oxidative stress, neurodegeneration, apoptosis, and diminished neuroplasticity, resulting in mental and neurodegenerative disorders [Citation5].
Elevated MAO activity can deactivate dopamine and impair emotion and specific memory signaling pathways. In contrast, reduced AChE activity can lead to prolonged cholinergic signal stimulation, impacting attention, memory, and motivation [Citation44]. Olajide et al. [Citation60] study linked cognitive decline to the hippocampus and cortical synaptophysin loss. The impairment in short-term memory could potentially be associated with the heightened MAO activity induced by SRT, consequently leading to increased oxidative damage within the brain. This oxidative stress might contribute to neurodegeneration, alterations in dopamine levels, and a decrease in synaptogenesis.
Depression is usually associated with changes in weight and appetite. The study found that CEE administration significantly increased body weight gain and aids in weight preservation in rats treated with SRT and CEE. Previous studies reported that the rate of weight gain for the CEE-treated groups was significantly higher than that for the control [Citation61,Citation62]. This increase was attributed to improved appetite, suggesting that CEE’s bioactive components may neutralize oxidative stress [Citation63].
Previous research found that CEE’s antioxidants, flavonoids, and E and C vitamins protect neural tissue from oxidative damage [Citation18]. In the current study, co-treatment with SRT and CEE hindered MAO activity and boosted dopamine levels compared to SRT – treated rats. It also reduced redox imbalance by hindering the elevation in MDA level and increasing the antioxidant markers such as SOD, CAT, and TAC. Additionally, it countered the neurodegenerative impact of SRT, mitigating synaptic damage, loss, and memory impairment. This neuroprotective effect was evident through a higher count of viable neurons, reduced numbers of glial cells, and elevated expression levels of SYP.
Furthermore, it maintained an average low exploration time in the MT maze compared to the SRT-treated group. These findings suggest that the combination of SRT and CEE may synergistically reduce oxidative stress and enhance antioxidant capacity. These findings signify the strengthening of the brain’s antioxidant defenses due to CEE administration.
CEE flavonoids and vitamins exhibit antioxidant and neuroprotective properties against scopolamine-induced Alzheimer’s disease by reducing lipid peroxidation (↓MDA), increasing antioxidant capacity (↑SOD, ↑CAT), reducing neural degeneration and necrosis, and enhancing cognitive function [Citation16,Citation18]. Previously, Arogundade et al. [Citation15] reported that neurotherapeutic and antioxidant properties of CEE could augment the production of SOD, CAT, and TAC in the different brain regions. Previous research has highlighted the neuroprotective potential of flavonoids, emphasizing their ability to reduce MAO activity [Citation64]. Plant-derived flavonoids improved Alzheimer’s disease rats’ spatial cognition, redox imbalances, cortical and hippocampus architecture, and SYP immunoreactivity [Citation48]. In rats, epimedium flavonoids inhibit neuron apoptosis, minimize synaptic destruction, and improve cognitive impairment caused by chronic hypoperfusion by increasing SYP expression in the cortex and hippocampus [Citation49]. In arsenic-induced neuropathy, the flavonoid-rich extracts decreased the activity of MAO and stopped neuron death and vacuolation [Citation44]. This data signify that CEE exhibits neuroprotective qualities by virtue of its vitamins and flavonoids, which reduce oxidative stress, improve cognitive function, safeguard neurons, and prevent neurodegeneration.
Limitation
Neurological conditions and cognitive impairment may result from an increased vulnerability to oxidative damage caused by SRT. It is crucial to develop therapies to counteract oxidative stress caused by SRT and restore the balance of antioxidants. Therefore, it is necessary to conduct more studies on long-term SRT use and ways to lessen its side effects.
Animal studies provide valuable insights, but clinical investigations are essential for validating CEE’s neuroprotective effects in humans. The study suggests that consuming Cyperus esculentus within the typical daily range may provide neuroprotective effects in humans. The equivalent human dose for a 200 mg/kg rat dose would be around 53.2 grams. This dosage is less than the typical daily intake of dried tiger nuts by humans (100–200 grams) [Citation65]. Further research is needed to determine the optimal dosage and application of Cyperus esculentus to fully understand its potential to promote brain health and mitigate medication-induced side effects.
Conclusion
CEE administration protected against SRT neurotoxicity and maintained cognitive function by lowering oxidative stress in the brain.
Disclosure statement
We express our gratitude to Prof. Mohamed Saad Allah El-Gerbed and Prof. Abd El-Fattah El-Beltagy from the Zoology Department, Faculty of Science, Damanhour University. Their helpful contributions involved providing insightful comments and assistance during the drafting of this manuscript, significantly enhancing the quality of our study.
References
- Qiao Y, Li C, Zhang M, et al. Effects of Tibetan medicine metacinnabar (β-HgS) combined with imipramine or sertraline on depression-like symptoms in mice. Front Pharmacol. 2022;13:971243.
- Onaolapo AY, Sulaiman H, Olofinnade AT, et al. Antidepressant-like potential of silymarin and silymarin-sertraline combination in mice: highlighting effects on behaviour, oxidative stress, and neuroinflammation. World J Pharm. 2022;11(3):27–47. doi: 10.5497/wjp.v11.i3.27
- Kafetzopoulos V, Kokras N, Sousa N, et al. Nucleus reuniens lesion and antidepressant treatment prevent hippocampal neurostructural alterations induced by chronic mild stress in male rats. Neuroscience. 2021;454:85–93. doi: 10.1016/j.neuroscience.2020.08.017
- Istifli ES, Çelik R, Hüsunet MT, et al. In vitro cytogenotoxic evaluation of sertraline. Interdiscip Toxicol. 2018;11(3):181–188. doi: 10.2478/intox-2018-0015
- Ľupták M, Fišar Z, Hroudová J. Different effects of SSRIs, Bupropion, and Trazodone on Mitochondrial Functions and Monoamine oxidase isoform activity. Antioxidants. 2023;12(6):1208. doi: 10.3390/antiox12061208
- Sayed DA. The protective effect of Nigella sativa oil against reproductive toxicity, hormonal alterations, and oxidative damage induced by Sertraline in male rats. Egypt J Exp Biol (Zoo). 2019;15(1):1–77. doi: 10.5455/egysebz.20190325113108
- Louei Monfared A, Yousefizadeh S. Curcumin Ameliorates Sertraline-mediated testicular toxicity in rats via inhibition of oxidative stress. Iran J Toxicol. 2023;17(1):25–34. doi: 10.32598/IJT.17.1.977.1
- Elkelany MM, Kashef SM. Role of spirulina extract in ameliorating histological and immunohistochemical changes induced by sertraline in the testis of adult albino rat. Egypt J Histol. 2021. doi: 10.21608/ejh.2021.91359.1545
- Prajapati NB, Gupta SD, Sood SV, et al. Antidepressants and seizure threshold: a case report on sertraline-induced seizure. Apollo Medicine. 2023;20(Suppl 2):S70–S72. doi: 10.4103/am.am_79_23
- Abdel-Salam OM, Youness ER, Khadrawy YA, et al. Brain and liver oxidative stress after sertraline and haloperidol treatment in mice. J Basic Clin Physiol Pharmacol. 2013;24(2):115–123. doi: 10.1515/jbcpp-2012-0022
- Yang H, Gu X, Chen H, et al. Transcriptome profiling reveals toxicity mechanisms following sertraline exposure in the brain of juvenile zebrafish (danio rerio). Ecotoxicol Environ Saf. 2022;242:113936. doi: 10.1016/j.ecoenv.2022.113936
- Chen H, Liang X, Gu X, et al. Environmentally relevant concentrations of sertraline disrupts behavior and the brain and liver transcriptome of juvenile yellow catfish (tachysurus fulvidraco): implications for the feeding and growth axis. J Hazard Mater. 2021;409:124974. doi: 10.1016/j.jhazmat.2020.124974
- Lozano AFQ, Moura MS, Tavares BM, et al. Exposure of pregnant rats to stress and/or sertraline: side effects on maternal health and neurobehavioral development of male offspring. Life Sci. 2021;285:119960. doi: 10.1016/j.lfs.2021.119960
- Jing S-Q, Wang S-S, Zhong R-M, et al. Neuroprotection of Cyperus esculentus L. orientin against cerebral ischemia/reperfusion induced brain injury. Neural Regen Res. 2020;15(3):548. doi: 10.4103/1673-5374.266063
- Arogundade T, Yawson E, Gbadamosi I, et al. Behavioural cellular and neurochemical alterations in rat prefrontal cortex and hippocampus exposed to tigernut (Cyperus esculentus) treatment. J Environ Toxicol Public Health. 2018;3:38–46.
- Saeed MM, Fernández-Ochoa Á, Saber FR, et al. The Potential Neuroprotective Effect of Cyperus esculentus L. Extract in Scopolamine-Induced Cognitive Impairment in Rats: Extensive Biological and Metabolomics Approaches. Molecules. 2022;27(20):7118. doi: 10.3390/molecules27207118
- Udefa AL, Amama EA, Archibong EA, et al. Antioxidant, anti-inflammatory and anti-apoptotic effects of hydro-ethanolic extract of Cyperus esculentus L.(tigernut) on lead acetate-induced testicular dysfunction in Wistar rats. Biomed Pharmacother. 2020;129(129):110491. doi: 10.1016/j.biopha.2020.110491
- Umukoro S, Okoh L, Igweze SC, et al. Protective effect of Cyperus esculentus (tiger nut) extract against scopolamine-induced memory loss and oxidative stress in mouse brain. Drug Metabol Personal Ther. 2020;35(3):20200112. doi: 10.1515/dmpt-2020-0112
- Ntukidem V, Ukwo P, Udoh I, Umoinyang E. Influence of different pretreatments on the nutritional and organoleptic properties of vegetable milk produced locally from tiger-nut (Cyperus esculentus) tubers. IOSR-JESTFT. 2019;13(6): 55–61. doi: 10.9790/2402-1306025561
- Adebayo-Oyetoro AO, Ogundipe OO, Adeyeye SAO, et al. Production and evaluation of tiger nut (Cyperus esculentus) milk flavoured with Moringa oleifera leaf extract. Curr Res Nutr Food Sci. 2019;7(1):265–271. doi: 10.12944/CRNFSJ.7.1.26
- Willis S, Jackson C, Verghese M. Effects of processing on antioxidant capacity and metabolizing enzyme inhibition of tiger nut tubers. Food Nutr Sci. 2019;10(9):1132. doi: 10.4236/fns.2019.109082
- Imam T, Aliyu F, Umar H. Preliminary phytochemical screening, elemental and proximate composition of two varieties of Cyperus esculentus (tiger nut). Nig J Bas App Sci. 2013;21(4):247–251. doi: 10.4314/njbas.v21i4.1
- Selma-Royo M, García-Mantrana I, Collado MC, et al. Intake of natural, unprocessed tiger nuts (Cyperus esculentus L.) drink significantly favors intestinal beneficial bacteria in a short period of time. Nutrients. 2022;14(9):1709. doi: 10.3390/nu14091709
- Nwosu LC, Edo GI, E Ö. The phytochemical, proximate, pharmacological, GC-MS analysis of Cyperus esculentus (tiger nut): a fully validated approach in health, food and nutrition. Food Biosci. 2022;46:101551. doi: 10.1016/j.fbio.2022.101551
- Adebisi I, Mahmud M, Ibrahim Y, et al. Exacerbation of symptoms of renal toxicity by aqueous extract of Cyperus esculentus in a gentamicin nephrotoxicity Model. Nig J Bas App Sci. 2022;30(2):139–146. doi: 10.4314/njbas.v30i2.19
- Bezerra MS, Martins ABM, Trajano FMG, et al. Fluoxetine and sertraline effects on rat distal cauda epididymis contraction, sperm count and sperm transit time trough epididymis. Eur J Pharmacol. 2019;865(865):172774. doi: 10.1016/j.ejphar.2019.172774
- Misik J, Kassa J. A comparison of cholinesterase inhibitors in the treatment of quinuclidinyl benzilate-induced behavioural deficit in rats performing the multiple T-maze. J Appl Biomed. 2014;12(4):211–217. doi: 10.1016/j.jab.2014.01.006
- Ohkawa H, Ohishi N, Yagi K. Assay for lipid peroxides in animal tissues by thiobarbituric acid reaction. Anal Biochem. 1979;95(2):351–358. doi: 10.1016/0003-2697(79)90738-3
- Sandouka S, Shekh-Ahmad T. Induction of the Nrf2 pathway by sulforaphane is neuroprotective in a rat temporal lobe epilepsy model. Antioxidants. 2021;10(11):1702. doi: 10.3390/antiox10111702
- Aebi H. [13] Catalase in vitro. Methods Enzymol. 1984;105:121–126. Elsevier.
- Nishikimi M, Rao NA, Yagi K. The occurrence of superoxide anion in the reaction of reduced phenazine methosulfate and molecular oxygen. Biochem Biophys Res Commun. 1972;46(2):849–854. doi: 10.1016/S0006-291X(72)80218-3
- Jiang H, Li F, Liu S, et al. Acute pancreatitis affects the metabolism of catecholamine neurotransmitters in rats. Neuroscience. 2014;268:112–117. doi: 10.1016/j.neuroscience.2014.03.022
- Bancroft JD, Layton C. 10 - the hematoxylins and eosin. In: Suvarna S, Layton C, and Bancroft J, editors Bancroft’s theory and practice of histological techniques. 8th ed. China: Elsevier; 2019. pp. 126–138.
- Varghese F, Bukhari AB, Malhotra R, et al. IHC profiler: an open source plugin for the quantitative evaluation and automated scoring of immunohistochemistry images of human tissue samples. PLoS One. 2014;9(5):e96801. doi: 10.1371/journal.pone.0096801
- Ogunsuyi OB, Omage FB, Ijomone OM, et al. Effect of chlorogenic acid plus donepezil on critical neurocortical enzyme activities, inflammatory markers, and synaptophysin immunoreactivity in scopolamine‐assaulted rats, supported by multiple ligand simultaneous docking. J Food Biochem. 2022;46(11):e14312. doi: 10.1111/jfbc.14312
- Sharma N, Jamwal S, Kumar P. Beneficial effect of antidepressants against rotenone induced Parkinsonism like symptoms in rats. Pathophysiology. 2016;23(2):123–134. doi: 10.1016/j.pathophys.2016.03.002
- Ilgin S, Kilic V, Baysal M, et al. Evidence for cardiotoxicity associated with sertraline in rats. Toxicol Res. 2018;7(5):817–825. doi: 10.1039/C8TX00072G
- Almansour MI, Jarrar YB, Jarrar BM. In vivo investigation on the chronic hepatotoxicity induced by sertraline. Environ Toxicol Pharmacol. 2018;61(61):107–115. doi: 10.1016/j.etap.2018.05.021
- Matt S, Nolan R, Channer B, et al. Antidepressants and dopamine levels induced by substance misuse regulate inflammation and HIV in Myeloid Cells. JPET. 2023;385:271.
- Cai L, Wang C, Huo X-K, et al. Effect of alkaloids isolated from phyllodium pulchellum on monoamine levels and monoamine oxidase activity in rat brain. Evid Based Complement Alternat Med. 2016;2016:1–6. doi: 10.1155/2016/6826175
- Arihan O, Yabanoglu SC, Ucar G, et al. Effects of two selected SSRIs on hemorheological parameters in rats. Clin Hemorheol Microcirc. 2019;71(1):27–38. doi: 10.3233/CH-170353
- Azimzadeh K, Jafarpour H, Adldoost S. Sertraline alters level of adenosine deaminase activity, oxidative stress markers and cardiac biomarkers (homocysteine cardiac troponin I) in rats. Pharma Biomed Res. 2017;3(3):17–22. doi: 10.29252/pbr.3.3.17
- Liaquat L, Sadir S, Batool Z, et al. Acute aluminum chloride toxicity revisited: study on DNA damage and histopathological, biochemical and neurochemical alterations in rat brain. Life Sci. 2019;217:202–211. doi: 10.1016/j.lfs.2018.12.009
- Ugbaja RN, Akinhanmi TF, Onunkwor BO, et al. Flavonoid-rich fractions of C. volubile and V. amygdalina alleviates arsenic-induced neurotoxicity by improving neurosignaling and antioxidant capacity in rats’ brain. Brain Disord. 2022;1–11. doi: 10.1016/j.dscb.2022.100050
- Owoeye O, Salami O. Monosodium glutamate toxicity: Sida acuta leaf extract ameliorated brain histological alterations, biochemical and haematological changes in wistar rats. Afr J Biomed Res. 2017;20(2):173–182.
- Bauchi Z, Kizito D, Alhassan A, et al. Effect of aqueous seed extract of Nigella sativa on lead-induced cerebral cortex toxicity in long evans rats. Bayero J Pure App Sci. 2016;9(1):48–52. doi: 10.4314/bajopas.v9i1.8
- Abou-Zeid SM, Tahoun EA, AbuBakr HO. Ameliorative effects of jojoba oil on fipronil-induced hepatorenal-and neuro-toxicity: the antioxidant status and apoptotic markers expression in rats. Environ Sci Pollut Res. 2021;28(20):25959–25971. doi: 10.1007/s11356-020-12083-2
- Mandour DA, Bendary M, Alsemeh AE. Histological and imunohistochemical alterations of hippocampus and prefrontal cortex in a rat model of Alzheimer like-disease with a preferential role of the flavonoid “hesperidin”. J Mol Histol. 2021;52(5):1043–1065. doi: 10.1007/s10735-021-09998-6
- Niu H-M, Ma D-L, Wang M-Y, et al. Epimedium flavonoids protect neurons and synapses in the brain via activating NRG1/ErbB4 and BDNF/Fyn signaling pathways in a chronic cerebral hypoperfusion rat model. Brain Res Bull. 2020;162:132–140. doi: 10.1016/j.brainresbull.2020.06.012
- Shalaby AM, Sharaf Eldin HE, Abdelsameea AA, et al. Betahistine attenuates seizures, neurodegeneration, apoptosis, and Gliosis in the Cerebral Cortex and hippocampus in a mouse Model of epilepsy: a histological, immunohistochemical, and biochemical study. Microsc Microanal. 2022;28(5):1734–1748. doi: 10.1017/S1431927622012107
- Abd Elmaaboud MA, Awad MM, El-Shaer RA, et al. The immunomodulatory effects of ethosuximide and sodium butyrate on experimentally induced fibromyalgia: the interaction between IL-4, synaptophysin, and TGF-β1/NF-κB signaling. Int Immunopharmacol. 2023;118(118):110061. doi: 10.1016/j.intimp.2023.110061
- Farag EA, Filobbos SA, Afifi NM, et al. Thyroxine restores hippocampal neurogenesis and synaptogenesis in a male rat model of carbimazole-induced hypothyroidism: a histological study. Beni-Suef Univ J Basic Appl. 2023;12(1):1–17. doi: 10.1186/s43088-023-00395-4
- Josiah SS, Umar HI, Saliu IO, et al. Quercetin and catechin assuage redox imbalance and neurochemical dysfunction in rotenone-induced neurotoxicity: a comparative in vivo experiment supported by in silico study. Phytomed Plus. 2021;1(4):100077. doi: 10.1016/j.phyplu.2021.100077
- Zhu LJ, Zhang C, Chen C. Research progress on vesicle cycle and neurological disorders. J Pharm Pharm Sci. 2021;24:400–412. doi: 10.18433/jpps31458
- Kumar B, Kumar V, Prashar V, et al. Dipropargyl substituted diphenylpyrimidines as dual inhibitors of monoamine oxidase and acetylcholinesterase. Eur J Med Chem. 2019;177(177):221–234. doi: 10.1016/j.ejmech.2019.05.039
- Rad I, Salari M, Dehghani A, et al. Effect of chronic exposure to sertraline on social and cognitive behavior of adult male and female rats under social isolation stress. Learn Motiv. 2022;78:101807. doi: 10.1016/j.lmot.2022.101807
- Patil SS, Sunyer B, Höger H, et al. Evaluation of spatial memory of C57BL/6J and CD1 mice in the Barnes maze, the Multiple T-maze and in the Morris water maze. Behav Brain Res. 2009;198(1):58–68. doi: 10.1016/j.bbr.2008.10.029
- Owoeye O, Akinbami R, Thomas M. Neuroprotective potential of Citrullus lanatus seed extract and vitamin E against mercury chloride intoxication in male rat brain. Afr J Biomed Res. 2018;21(1):43–49.
- Adefegha SA, Dada FA, Oyeleye SI, et al. Effects of berberine on cholinesterases and monoamine oxidase activities, and antioxidant status in the brain of streptozotocin (STZ)-induced diabetic rats. J Basic Clin Physiol Pharmacol. 2021;33(4):389–397. doi: 10.1515/jbcpp-2020-0173
- Olajide OJ, Gbadamosi IT, Yawson EO, et al. Hippocampal degeneration and behavioral impairment during Alzheimer-like pathogenesis involves glutamate excitotoxicity. J Mol Neurosci. 2021;71(6):1205–1220. doi: 10.1007/s12031-020-01747-w
- Badgujar SB, Bandivdekar AH. Evaluation of a lactogenic activity of an aqueous extract of Cyperus rotundus Linn. J Ethnopharmacol. 2015;163(163):39–42. doi: 10.1016/j.jep.2015.01.019
- Ekpenyong NE, Ogar OP, Ekpo, AE. Feeding Value of Tiger Nut (Cyperus Esculentus) Meal for Growing Rabbits. Sumerianz Journal of Agriculture and Veterinary. 2020;3(7):85–89.
- Enye LA, Edem EE, Onyeogaziri LI, et al. Tiger nut/coconut dietary intervention as antidotal nutritional remediation strategy against neurobehavioural deficits following organophosphate-induced gut-brain axis dysregulation in mice. Toxicol Rep. 2024;12:23–40. doi: 10.1016/j.toxrep.2023.12.003
- Dhiman P, Malik N, Sobarzo-Sánchez E, et al. Quercetin and related chromenone derivatives as monoamine oxidase inhibitors: targeting neurological and mental disorders. Molecules. 2019;24(3):418. doi: 10.3390/molecules24030418
- Allouh MZ, Daradka HM, Ghaida JHA. Influence of Cyperus esculentus tubers (Tiger Nut) on male rat copulatory behavior. BMC Complement Altern Med. 2015;15(1):1–7. doi: 10.1186/s12906-015-0851-9