ABSTRACT
Obesity-related health problems are worsening in various societies worldwide. Artemisia anuua L. (AA) has distinctive health benefits, including anti-inflammatory, antioxidant, and insulin-sensitizing properties. Nanoformulation of herbal extracts is a new approach to enhance the bioavailability of natural products. This study aimed to evaluate the efficacy of AA extract, either the conventional extract (AAE) or its nano-form (AAEN), in modulating obesity-related metabolic complications in hypercaloric (high-fat/high-sucrose) diet-induced obese rats. Forty-eight rats were divided into two groups fed either a basal or hypercaloric diet for twelve weeks. Starting from the eighth week, each main group was subdivided into three subgroups and treated orally with either saline, AAE, or AAEN until the end of the experiment. Both AAE and AAEN exerted antiadipogenic effects. They reduced body weight and epididymal fat, mitigated dyslipidemia, improved glucose utilization and insulin resistance, reduced hyperleptinemia, increased serum adiponectin, and decreased oxidative stress and inflammatory markers. These results were mediated by regulating SIRT-1, PGC-1α, SREBP-1, PPAR-γ, FAS, LPL, IRS 1, and GLUT4 gene expression, suggesting an effect of AA on de novo lipogenesis and glucose utilization. The AAEN produced more expressive ameliorating effects than the conventional extract. AAE administration ameliorated the obesity-associated metabolic complications induced by feeding a hypercaloric diet, implying that this extract may be used as a complementary treatment option for obesity and its metabolic consequences. The antiadipogenic effectiveness of AAE was enhanced by its nanoformulation, suggesting that the nanoparticle system can be applied to boost other herbal medicine effectiveness.
Introduction
Obesity is a growing health problem affecting billions of people around the world. It has been expected that more than 57.8% of adults and approximately 3.3 billion individuals globally will encounter overweight or obesity problems by 2030 [Citation1]. Excessive energy consumption in the form of a Western diet, which is frequently combined with a sedentary lifestyle, has been proposed to be the main contributor to the obesity epidemic [Citation2]. Chronic excessive consumption of saturated fat, cholesterol, and sugars may cause an alteration in the activity of transcription factors, leading to a dysregulation of gene expression and promoting intraorgan lipid buildup [Citation3]. Many metabolic and endocrine disorders can be caused by the abnormal expansion of body fat, including dyslipidemia, hypertension, and insulin resistance, which increase the risk of type 2 diabetes (T2DM) [Citation4]. These metabolic abnormalities are associated with the release of pro-inflammatory adipocytokines as elements of a mild to moderately severe systemic inflammatory response and elevated oxidative stress. Several transcription factors regulate adipocyte differentiation and lipogenesis. One of lipid metabolism’s most critical transcription factors is the peroxisome proliferator-activated receptor γ (PPARγ). PPARγ is involved in the transcription regulation of adipocyte differentiation, lipid storage, and glucose homeostasis [Citation5]. Sterol regulatory element-binding protein (SREBP) is another transcription factor that controls lipid metabolism [Citation6]. SREBP-1 targets lipogenic genes, such as fatty acid synthase (FAS) and acetyl-CoA carboxylase (ACC), involved in fatty acid synthesis, as well as β-Hydroxy β-methylglutaryl-CoA (HMG-CoA), which is a rate-limit enzyme for cholesterol synthesis [Citation7]. Additionally, SIRT1, a member of the mammalian sirtuin family, plays a significant role in metabolism, influencing mitochondrial biogenesis and glycolysis [Citation8]. Sirt-1 regulates multiple transcription factors in endocrine signaling pathways, most notably peroxisome proliferator-activated receptor gamma coactivator-1 alpha (PGC-1α) and glucose transporter-4 (GLUT-4), thus enhancing insulin sensitivity [Citation9]. Additionally, Sirt-1 overexpression protects against high-fat diet metabolic repercussions and insulin resistance in diabetic animals [Citation8].
The primary approach for obesity management is through dietary intervention and increasing physical activity. However, anti-obesity medication is recommended if this approach is ineffective. Nevertheless, the weight loss drugs prescribed in conventional medicine induce many unfavorable side effects, primarily affecting monoamine neurotransmitters and promoting drug abuse [Citation9]. Approved medications for long-term use in the treatment of obesity are orlistat and sibutramine. However, these agents have many adverse effects, such as gastrointestinal disturbances and increased incidence of cardiovascular disorders [Citation10].Owing to these unfavorable consequences, traditional herbal medicine has gained popularity as an obesity management strategy. Various studies have shown that several medicinal herbs, including oolong tea, ginger, moringa oleifera, green tea, camellia sinensis, nigella sativa, and seaweed laminaria, have beneficial impacts on obesity and its metabolic implications [Citation11]. Many medicinal plants have hypolipidemic properties, which are efficacious in the management of obesity [Citation10]. Oolong tea, nigella sativa, and camellia sinensis have been demonstrated to significantly lower body weight and reduce triacylglycerols, low-density lipoprotein (LDL) cholesterol, and fasting blood glucose levels. Other herbs and their metabolites, such as epigallocatechin-3-gallate and green tea, speed up metabolic rate, body fat metabolism, and oxidation. In the same way, ginger promotes lipolysis, suppresses adipogenesis by blocking PPAR-γ action, and limits lipid droplet development in 3T3-L1 pre-adipocytes [Citation12]. Likewise, moringa oleifera has demonstrated effectiveness in lowering body weight by downregulating the mRNA expression of resistin and leptin and upregulating the mRNA expression of adiponectin, which is the primary mechanism for ameliorating adiposity [Citation13]. Other herbs with anti-obesity properties, like nigella sativa and green tea, seem to function by lowering liver or plasma lipid peroxidation. However, the efficacy and safety of these herbal medicines are still critical points that should be evaluated [Citation14].
One of the several beneficial medicinal herbs is Artemisia anuua L. (AA), a plant in the genus Artemisia, which belongs to the Asteraceae family and has more than 500 species worldwide. It is often utilized as an herbal tea, spice, and medicinal herb [Citation15–17]. Artemisia annua has been reported to have in vitro free radical scavenging and antioxidant activities attributed to the presence of certain families of bioactive compounds, namely terpenes, flavonoids, coumarins, and essential oils [Citation15]. In a D-galactose-induced oxidative stress mice model, the aqueous ethanol extract of AA leaves reduced serum levels of malondialdehyde and 8-hydroxy-2’-deoxyguanosine, biomarkers for lipid peroxidation and DNA damage, respectively, and increased the activity of the antioxidant marker enzyme, NAD(P)H quinone oxidoreductase [Citation16]. Moreover, earlier research has shown that the extract from AA had inhibitory effects on the production of inflammatory cytokines in lipopolysaccharide-stimulated macrophages, including interleukin-1beta (IL-1β), tumor necrosis factor-alpha (TNF-α), cyclooxygenase-2 (COX-2) and inducible nitric oxide synthase (iNOS) [Citation17]. Artemisia extract also ameliorated fatty liver and inflammatory responses in mice fed a high-fat diet [Citation18]. Additionally, AA extracts were shown to mitigate insulin resistance in STZ- and HFD-induced diabetic rats by modulating their lipid profile and adipocytokines [Citation19,Citation20]. In alloxan-induced diabetic rats, the AA aqueous extract lowered fasting blood glucose, insulin, and LDL/HDL levels [Citation21]. In addition, Artesunate and artemisinic acid, natural products derived from AA, prevented adipogenesis in 3T3-L1 preadipocytes [Citation22] and suppressed adipogenic differentiation of human adipose tissue-derived mesenchymal stem cells [Citation23].
Despite medicinal plants’ potential benefits in managing obesity and associated complications, their bioactivity is reduced because of their limited bioavailability [Citation24]. One of the possible approaches to enhance the bioavailability of plant natural products is by formulating these products into nano-sized forms [Citation25]. Nanonization enhances these compounds’ oral bioavailability and therapeutic efficacy by expanding their surface area. The nanoparticles’ extensive surface area increases the adhesion surface area between nanoparticles and the mucin layer that lines the villi intestinal epithelium, facilitating the nanosized drug to traverse the mucin layer and the epithelial cells. It has been observed that in a Caco-2 cell model, the absorption efficiency increases with decreasing particle size [Citation26]. Similarly, smaller particles of drugs are taken up more readily by macrophages and achieve a higher deposition rate, hence a better therapeutic index [Citation27]. The current approaches for nanoparticle synthesis focus on greener aspects that eliminate or minimize the use of hazardous chemicals or conventional energy sources. Distinctive greener methods apply using sonochemistry, microwave, electrochemical, hydrothermal, biosynthesis, and solar energy. The sonochemical method for producing nanoparticles is a well-established and extensively examined field because of its versatility and simplicity. The fundamental concept of sonochemistry originates from acoustic cavitation, which involves the production of bubbles followed by their expansion and implosive collapse, which leads to high temperature and pressure followed by rapid cooling. The size and shape of the created nanomaterial can be manipulated by accommodating the precursors, the liquid in which the reaction happens, and the ultrasonic wave frequency [Citation28].
Many studies have used AAE as a reducing and capping agent for gold, copper, and silver nanoparticle production, exploiting the phytochemical properties of this plant [Citation29–31]. However, very few or no studies examined the use of the sonochemistry technique for nanonization of AAE. Thus, the novelty of this work is the nanoformulation of AAE using a sonochemical technique and the evaluation of its anti-obesity efficacy in animal models. The present study hypothesis was that nanoformulation of AA extracts could enhance the therapeutic potentials and boost the extract’s efficacy to control the obesity-related metabolic complications in rats fed a hypercaloric diet more than conventional extract. Consequently, in this work, the effects of A. annua ethanolic extract and its nanoparticles on adiposity, blood lipid profile, glucose tolerance, insulin resistance, adipocytokines, inflammatory and oxidative stress markers, and the regulation of SIRT-1, PGC-1α, SREBP-1, PPAR-γ, FAS, LPL, IRS 1, and GLUT4 genes expression that involved in carbohydrate and lipid metabolism were evaluated and compared in rats fed hypercaloric diet.
Materials and methods
Herb collection and extract preparation
Plants of the genus Artemisia anuua L. (family Asteraceae) were obtained from the Agriculture Research Centre, Giza, Egypt. Leaves of Artemisia anuua (AA) were cut from the stalks, cleaned using distilled water, dried in an oven heated to 30°C, and manually crushed. Approximately 300 g of the dried leaves were decocted and refluxed thrice with ethanol (900 mL) and then filtered and concentrated utilizing a rotary evaporator. The filtrate was lyophilized, and the lyophilized powders of AAE were kept until usage at room temperature [Citation32].
Preparation of Artemisia nanoparticles
The synthesis of AAEN was carried out via sonochemical technique utilizing an ultrasonic probe device (Hielscher model, up 400 s; Teltow, Germany). Briefly, 20 g of AAE was added to one liter of doubled deionized water and subjected to ultrasonic pressure under the following conditions: increases every 2 sec at 95% amplitude power and maximum temperature (60 ◦C); rest time, 1 sec.; and total time of sonication, 4 h. Ultrasonic irradiation caused micron-sized bubbles to develop and proliferate, with tremendous pressure and temperature on both their inner and outer surfaces. Upon collapsing, the AAE molecules underwent nucleated formation, followed by fast cooling, producing AAE nanoparticles [Citation33].
Characterization of Artemisia nanoparticles
The identification, morphology, and surface area of the prepared Artemisia nanoparticles were examined using multiple analysis techniques, including Fourier transform infrared (FT-IR), X-ray diffraction (XRD), transmission electron microscopy (TEM), and scanning electron microscopy (SEM) and energy dispersive X-ray (EDX). The functional groups present over the surface of AAEN were explored by FT-IR analysis. The FT-IR spectra were analyzed using the Bruker FTIR instrument. XRD (D8 Discovery – Bruker Company) was used to determine the material’s crystallinity in the temperature range 2 V (5 − 80°C) with a scanning step size of 0.016°/s. The morphological characteristics of the AAENs were determined using the TEM (Electron Microscope-2100 model High-Resolution- Japan) to determine the roughness, surface morphology, concentration, agglomeration, and shape of the nanoparticles. Scanning electron microscopy examination with energy dispersive X-ray (EDX) was performed to study the AAEN morphology and elemental composition. EDX analysis setup was coupled with the SEM instrument. The surface area was determined by the Brunauer, Emmett, and Teller (BET) method (degassing was performed to remove moisture content and dust at 80°C under vacuum) using a surface area and pore size analyzer (Nova Touch LX2, Quantachrome Co. USA). The particle size was determined utilizing dynamic light scattering (DLS). In the DLS study, a laser beam illuminates the sample suspension, and then the laser light scatters in all directions. Over time, light scattering is observed at a certain angle. The random Brownian motion of the particles causes signal variation. The Stokes-Einstein equation was used to calculate the particle size from the angular intensity distribution [Citation34].
Diets
A basal diet and a high-fat diet were used in this study. The basal diet used in this study was based on AIN-93 recommendations [Citation35]. The composition of the basal and high-fat diets is described in .
Table 1. Composition of experimental diets.
Animals and ethical statement
Forty-eight Sprague Dawley-albino adult male rats weighing approximately 130–150 g were purchased from the Helwan Farm of Serum and Vaccine, Cairo, Egypt. In stainless steel cages, the animals were housed (one rat in each cage) in an adequately ventilated house under healthy conditions (23°C; 12 h of natural light and 12 h of darkness; and 45–50% relative humidity). They were acclimatized to the animal housing conditions (one week) and permitted free basal diet and water access. This study was endorsed by the Ethics Committee in Women’s College, Ain Shams University, Egypt (approval No. sci1532309001).
Experimental design
After a week of accommodation, the rats were categorized into two primary groups. The rats in the first group were fed a basal diet for 12 weeks. Starting from the eighth week, the rats were subdivided into three subgroups: the first was treated orally with saline (2 ml/kg body weight, negative control group); the other two groups were given a daily oral dosage (50 mg/kg body weight, p.o. [Citation37], of AAE (AAE group) or AAEN (AAEN group). Meanwhile, rats in the second group were fed a hypercaloric diet (high-fat diet + 15% sucrose in drinking water) for 12 weeks. Starting from the eighth week, the rats were subdivided into three subgroups: the first group was treated orally with saline (positive control, HF/HS group), and the other two groups were treated daily with an oral dose of AAE (HF/HS+AAE group) or AAEN (HF/HS+AAEN group) until the end of experimentation (12 weeks).
Tissue preparation and sampling
Upon completion of the experiment, all rats were fasted for 10 h; drinking water was not restricted. Blood samples were taken under anesthesia with isoflurane inhalation from the retroorbital venous plexus of the eyes in sanitized gel tubes. Then, blood samples were spun for 15 minutes at 3000 rpm for serum separation, which was split into many aliquots and maintained at −20°C until biochemical analysis [Citation38]. The rats were then sacrificed by isoflurane overdose. Each rat’s liver, spleen, kidneys, epididymal fat, and brain were instantly removed, rinsed in buffered saline (0.9% sodium chloride solution), blotted using filter paper, and weighed. Liver and epididymal fat segments were dipped in a 10% paraformaldehyde solution for histopathological investigations. Another part of the epidydimal fat was homogenized with Tris hydrochloride buffer (0.01 mol/L and 7.4 pH) and subjected to 10,000 g ultracentrifugation at 4°C for 30 min. The supernatant that results was utilized to estimate TNF-⍺, IL-1β, and iNOS. The remaining liver parts, hypothalamus, and epididymal fat were preserved at − 80°C for quantitative real-time polymerase chain reaction (qPCR) analysis.
Glucose tolerance test (GTT)
One week before rats were sacrificed, an oral GGT (OGTT) was performed on each rat. For this purpose, rats were starved all night long, and the next morning, the fasting blood glucose was determined via perforation of the venous tail by a Glucometer (ARKRAY Factory, Inc., Koji, Japan). Then, a glucose bolus (two g/kg body weight) was orally administered to rats, and blood glucose (BG) levels were assessed 30, 60, and 120 minutes afterward. The area under the curve (AUC) for the glucose response was estimated using the following equation:
AUC = 0.5 × (Fasting BG + BG 30)/2 + 0.5 × (BG 30 + BG 60)/2 + 1×(BG 60 + BG 120)/2 [Citation39]
Biochemical analysis
Serum triacylglycerol (TAG), total cholesterol (TC), and high-density lipoprotein (HDL) cholesterol were determined colorimetrically using standard kits according to the methods of Fossati & Prencipe [Citation40], Guder et al. [Citation41] and Rifal and Warnick [Citation42], respectively. The levels of very low-density lipoprotein (VLDL) cholesterol (TAG/5) and low-density lipoprotein (LDL) cholesterol were assessed by the equation: [LDL=TC-(HDL-cholesterol + TAG/5) [Citation43]. The serum level of leptin was assessed by rat-specific ELISA kits provided by My BioSource (Netherlands), and the serum adiponectin level was measured by rat-ELISA kits supplied by CUSABIO (USA) following the manufacturer’s instructions.
The serum glucose levels were measured utilizing a glucose-oxidase enzymatic kit (BIO MED Diagnostic, Egypt) and determined colorimetrically at 546 nm with a spectrophotometer (Synergy, BioTek Instruments, Inc., Winooski, VT. USA) according to the manufacturer’s protocol. Serum insulin levels were measured by a rat-specific insulin enzyme-linked immunosorbent assay (ELISA) commercial kit from Ray Biotech (USA). Then, the insulin resistance index (homeostasis model assessment HOMA) was calculated using the formula fasting HOMA= glucose (mM)/22.5 × fasting insulin (µU/mL) [Citation39].
Aspartate transaminase (AST), alanine transaminase (ALT), and alkaline phosphatase (ALP) serum activities were assessed colorimetrically using BIO MED Diagnostic kits (Egypt) following the standards and protocols provided by the manufacturer.
The level of serum malondialdehyde (MDA) and the activity of superoxide dismutase (SOD) were determined utilizing commercially available kits (Bio-Diagnostic, Egypt) following the guidelines supplied by the manufacturer. TNF-α levels in the epidydimal fat were measured using a rat ELISA kit supplied by CUSABIO (USA). IL-1β and iNOS levels in the epidydimal adipose tissue were determined using rat kits provided by My BioSource (Netherlands) as described in the manufacturer’s protocol.
Reverse transcription-quantitative polymerase chain reaction (RT-qPCR)
The extraction of total RNA from the liver, epididymal fat, and hypothalamus tissues was performed utilizing a miRNeasy Mini Kit (Qiagen, USA) based on the instructions provided by the manufacturer. The level of RNA was quantified using a NanoDrop ND- 1000 spectrophotometer at 260 nm (Thermo Fisher Scientific, Waltham, USA), and the RNA purity was tested employing the absorbance ratio at a wavelength of 260/280 nm. The integrity of RNA was validated using gel electrophoresis on an ethidium bromide-stained agarose gel. Afterward, a high-capacity complementary DNA (cDNA) reverse transcription kit was used to reverse-transcribe the extracted RNA into cDNA (Fermentas, USA). To measure the mRNA expression of FAS, GLUT4, insulin receptor substrate 1 (IRS 1), lipoprotein lipase (LPL), leptin receptor (OB R), PPAR-γ, PGC-1α, SIRT-1, and sterol SREBP-1, qPCR was done utilizing an ABI PRISM 7500 fast sequence detection system (Applied Biosystems, Carlsbad, CA) applying typical cycling circumstances (10 min 95°C, 40 cycles of 15 s at 95°C and 60 s at 60°C). The PCR primer pair sequences for each gene are listed in . The data was analyzed by the ABI Prism sequence detection system software and PE Biosystems’ v1–7 Sequence Detection Software (Foster, California, USA). The genes’ relative expression was estimated by a comparable cycle threshold technique. Every value was normalized to the β-actin gene, and its relative copy number (RCN) was expressed. The cycle threshold (Ct) estimates for every sample were determined, and transcript levels were assessed by the equation R = 2−ΔΔCt [Citation44].
Table 2. List of sequences of primers used in real-time qPCR analysis.
Histopathological investigation
For histopathological examination, liver and epididymal fat samples were collected from eight rats per group and then processed after being fixed in 10% neutral buffered formalin. Sections of 5 μm thickness were prepared, stained with hematoxylin and eosin (H&E), and examined by a light microscope (Olympus BX50, Tokyo, Japan). The liver and adipose tissue histopathological lesions were rated and scored from zero to three by the calculation of the lesions’ percentage in five randomly selected microscopic fields per rat as follows: (zero) revealed no alterations, and (one), (two), and (three) denoted mild, moderate, and severe alterations, respectively. The grading was assessed by percentage as follows: (less than 30%) indicated mild changes, (30% − 50%) denoted moderate changes, and more than 50% revealed severe alteration [Citation45].
Statistical analysis
The statistical analysis of the data was conducted with SPSS 18.0 software (SPSS, Chicago, IL, USA) and is presented as the mean ± standard deviation (mean±SD). One-way analysis of variance was performed, followed by Post hoc tests for comparison among multiple groups. A significance level of less than 0.05 was deemed to be statistically significant.
Results
Artemisia extract nanoparticle characterization
FT-IR analysis was conducted to determine the various functional groups in AAEN. The phenolic compounds are the primary components of Artemisia extract that have antioxidant and anti-inflammatory effects. They include hydroxyl and carboxylic groups [Citation15–17]. The FT-IR spectra of AAEN are illustrated in . AAEN shows a typical absorption peak at 1737 cm−1 due to lactone’s C–O stretching vibrations. The absorption peaks between 800 cm−1 and 1200 cm−1 are attributed to the O–O and C–O modes of O–O–C (peroxide). High concentrations of phenols with O–H stretches of phenols and N–H amines may be seen in the broad observation peaks at 3350 and 3250 cm−1. These results have shown a close agreement with the previously reported publication [Citation46].
Figure 1. FT-IR spectrum of the green synthesized Artemisia anuua extract nanoparticles using sonochemical technique.
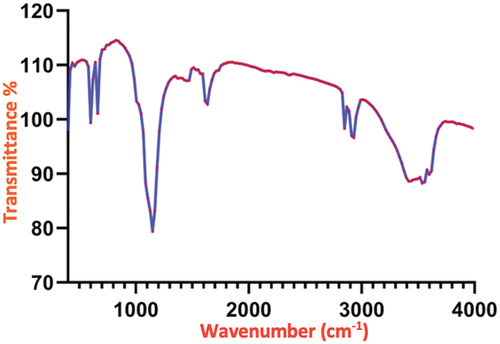
shows an XRD graph exhibiting the amorphous nature of Artemisia nanoparticles. The observed XRD spectrum’s broad diffraction peak is distinctive of an amorphous material, suggesting the absence of long-range crystalline order within the nanoparticles. The scarcity of distinct and sharp diffraction peaks further proves the disordered, amorphous character of the Artemisia nanoparticles. The amorphous structure is often ascribed to the rapid nucleation and growth kinetics during nanoparticle formation, leading to quenched, metastable-in a disordered state. The amorphous character is further enhanced by the increased surface area and decreased dimensionality in the nanoscale range as the surface impacts become more prevailing, disrupting the periodic lattice arrangement. Artemisia nanoparticles’ amorphous form may significantly impact their physicochemical characteristics, specifically how they dissolve and degrade, as well as how they interact with biological systems.
Figure 2. XRD of the green synthesized Artemisia anuua extract nanoparticles using sonochemical technique.
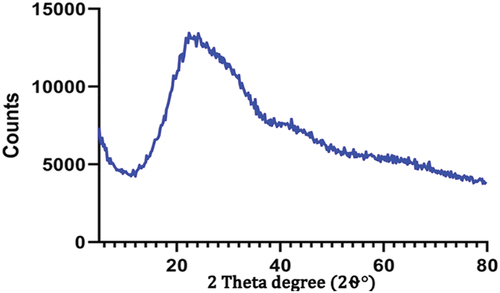
The photomicrographs obtained through transmission electron microscope (TEM) and scanning electron microscope (SEM) demonstrate critical structural insights into the synthesized Artemisia nanoparticles. In , SEM and TEM images illustrate that the Artemisia spherical-shaped nanoparticles are around 34 nanometers, with a confined size distribution, great uniformity, and monodispersity, indicating excellent size control during synthesis. The spherical shape implies controlled nucleation and growth mechanisms during the synthesis process that reduce anisotropic effects. The spherical morphology and small size in the nanoscale range (1–100 nm) imply a high surface area to volume ratio for the Artemisia nanoparticles. This could significantly affect their physiochemical properties, particularly for applications mandating maximized surface interactions. The characterization data confirm the successful synthesis of well-defined Artemisia nanoparticles with controlled dimensions in the nanoscale range.
Figure 3. TEM (a), SEM (b), and EDX (c) Images for the green synthesized Artemisia anuua extract nanoparticles using sonochemical technique.
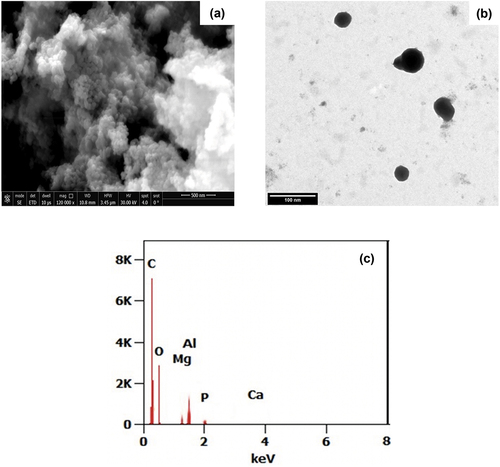
The energy-dispersive X-ray spectroscopy (EDX) analytical technique identifies and measures the substance’s elemental composition. The EDX chart demonstrated in ) confirms the existence of carbon and oxygen at 0.2 and 0.5 KeV with weight and atomic percentages of 88%, which could be related to the abundance of organic components of the extract [Citation46].
depicts dynamic light scattering (DLS) analysis, revealing a narrow, monodisperse size distribution centered at approximately 45 nm, confirming the excellent size control achieved through the synthesis method. The monomodal peak suggests uniform nucleation and growth conditions during particle formation. Zeta potential analysis () displays a highly negative value of −34.1 mV for the Artemisia nanoparticles. The large negative surface charge enhances electrostatic repulsion between particles, encouraging colloidal stability and preventing agglomeration. The specific surface area, measured via Brunauer – Emmett – Teller (BET) analysis, displays a remarkably high value of 96.2 m2/g for the Artemisia nanoparticles. This large surface area directly results from their nanoscale dimensions and spherical morphology, as marked in the electron microscopy images. The high surface area to volume ratio significantly impacts nanoparticle reactivity, dissolution kinetics, and interaction capacity with target molecules or biological systems.
Body and organ weights
summarizes the body and organ weight changes in the different groups. Chronic consumption of the hypercaloric diet resulted in a considerable increase in the final body weight (47.28%, p < 0.001), BMI (20.63%, p < 0.01), relative weights of the liver (18.7%, p < 0.05), spleen (20.5%, p < 0.05) and epididymal fat (120.3%, p < 0.001) and a significant (p < 0.05) decrease in brain relative weight (14%) compared to the negative control. Heart and kidney relative weights showed no significant changes (p > 0.05) in HF/HS-fed rats compared to the negative control. Oral treatment of HF/HS-fed rats with AAE or AAEN significantly (p < 0.05) decreased the final body weight (15.53% and 16.18%), BMI (15.7% and 17.1), and relative weights of the liver (18.6 and 18.6%, p < 0.01), spleen (17.07% and 19.5%, p < 0.01), and epidydimal adipose tissue (38.9% and 44.3%, p < 0.001), respectively, as compared to the positive control. The relative weight of the brain was significantly (p < 0.01) increased to reach the normal value and 18.3% more than the corresponding values in the positive control in HF/HS-fed rats treated orally with AAEN.
Table 3. Effects of Artemisia extract and its nanoparticle form on body weight, body mass index (BMI), and relative organ weights in rats fed a basal diet or hypercaloric diet.
Serum lipid profile
elicits serum lipid profile alterations in different groups. Consumption of the HF/HS diet resulted in dyslipidemia, as evidenced by substantial (p < 0.001) elevation in serum levels of triacylglycerol (46%), total cholesterol (70.55%), VLDL cholesterol (46%) and LDL cholesterol (158.5%) and a remarkable decline (p < 0.001) in serum levels of HDL cholesterol (53.8%) as compared with the negative control. The two tested treatments mitigated these alterations in serum lipid profile, with more effective improvement achieved by the Artemisia nanoparticle extract. Oral administration of AAE and its nanoparticles to HF/HS-fed rats significantly lowered serum levels of triacylglycerol (12.6%, p < 0.05 and 26.6%, p < 0.01) and total cholesterol (26.9%. p < 0.01 and 37.1%, p < 0.001), VLDL cholesterol (12.6%, p < 0.05 and 26.6%, p < 0.01) and LDL cholesterol (42.8% and 55.9%, p < 0.001) and significantly restored the serum levels of HDL cholesterol to normalcy as they significantly (p < 0.001) increased serum HDL by 92.18% and 111.1%, respectively, as compared to the positive control ().
Figure 5. Effects of Artemisia extract and Artemisia extract nanoparticles on the serum lipid profile (a) Triacylglycerols, (b) Total cholesterol, (c) High-density lipoprotein (HDL) cholesterol, (d) Very low-density lipoprotein (VLDL) cholesterol, and (e) Low-density lipoprotein (LDL) cholesterol in rats fed on a basal diet or HF/HS diet. Data are displayed as the mean ± SD for 8 rats. The letters a, b, and c represent significant differences from the negative control, positive control, and HF/HS + AAE groups, respectively. †, #, and * demonstrate statistical significance at p < 0.05, p < 0.01, and p < 0.001, respectively, using one-way ANOVA followed by the Post hoc tests.
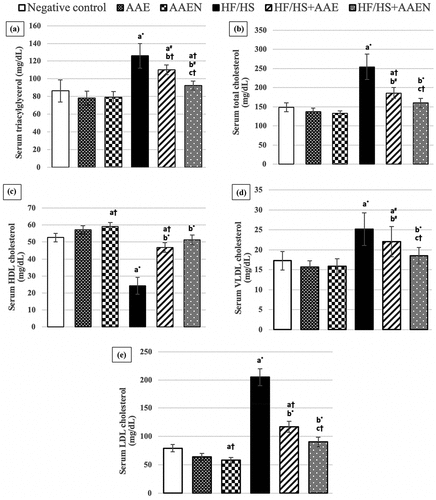
Expression of critical genes involved in lipid metabolism
displays the detected alterations in the expression of crucial genes related to lipid metabolism. Feeding an HF/HS diet instigated substantial (p < 0.001) downregulation in hepatic SIRT-1(82.1%) and PGC-1α (78.2%) gene expression and significant (p < 0.001) upregulation in hepatic SREBP-1 gene expression (4.45-fold) compared to the negative control. Such alterations were effectively alleviated by oral administration of AAE and AAEN as demonstrated by a significant (p < 0.001) upregulation in SIRT-1 (2.94- and 3.5-fold) and PGC-1α (2.09- and 2.727-fold) and a significant (p < 0.001) decline in SREBP-1 gene expression (56.8% and 68.6%), respectively, compared to the corresponding levels in the HF/HS diet-fed rats. Furthermore, feeding an HF/HS diet induced a remarkable (p < 0.001) upregulation in the epidydimal adipose tissue PPAR-γ, FAS, and LPL gene expression (1.29-, 5.19-, and 4.14-fold, respectively) compared to basal diet-fed rats. This gene dysregulation was notably mitigated by oral supplementation of HF/HS diet-fed rats with AAE or AAEN as manifested through marked (p < 0.001) downregulation of PPAR-γ (27.96% and 44.91%), FAS (51.1% and 56.23%) and LPL (50.3% and 64.8%), respectively, as compared to the positive control. It is worth noting that AAEN treatment was more efficient in restoring the alteration in gene expression than AAE treatment ().
Figure 6. Effects of Artemisia extract and Artemisia extract nanoparticles on hepatic relative mRNA gene expression of (a) SREBP-1, (B) SIRT-1, and (c) PGC-1⍺, and epididymal fat relative mRNA gene expression of (d) PARP- γ, (e) FAS and (f) LPL in rats fed on a basal diet or HF/HS diet. Data are displayed as the mean ± SD. The letters a, b, and c represent significant differences from the negative control, positive control, and HF/HS + AAE groups, respectively. †, #, and * demonstrate statistical significance at p < 0.05, p < 0.01, and p < 0.001, respectively, using one-way ANOVA followed by the Post hoc tests.
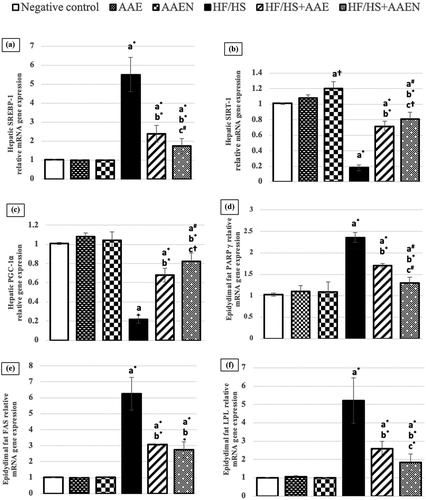
Leptin and adiponectin serum concentrations OB R gene expression in the hypothalamus and epididymal fat
The data presented in demonstrate that HF/HS diet consumption elicited a substantial elevation (p < 0.001) in the serum level of leptin (3.66-folds) and a remarkable decline in the serum adiponectin level (63%) compared to the negative control. These alterations were effectively reinstated by the two tested treatments (AAE and AAEN), with marked improvement attained by oral supplementation of AAEN compared to that of AAE. The serum leptin level displayed a marked decrease (50.1% and 74.6%), and serum adiponectin showed a marked increase (85.5% and 130%) in HF/HS diet-fed rats treated with AAE and AAEN, respectively, compared to the corresponding levels in HF/HS diet-fed rats.
Figure 7. Effects of Artemisia extract and Artemisia extract nanoparticles on serum (a) Leptin and (b) Adiponectin and OB R gene expression in (c) Hypothalamus and (d) Epididymal fat in rats fed on a basal diet or HF/HS diet. Data are displayed as the mean ± SD. The letters a, b, and c represent significant differences from the negative control, positive control, and HF/HS + AAE groups, respectively. †, #, and * demonstrate statistical significance at p < 0.05, p < 0.01, and p < 0.001, respectively, using one-way ANOVA followed by the Post hoc tests.
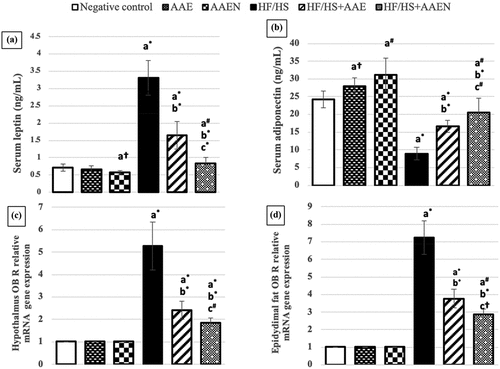
In addition, demonstrates that feeding an HF/HS diet instigated substantial (p < 0.001) upregulation in OB R gene expression levels in the hypothalamus and epididymal fat (4.2- and 6.158-fold, respectively). Both tested treatments substantially attenuated such changes. Oral supplementation with AAE and AAEN elicited a significant (p < 0.001) decrease in OB R gene expression in the hypothalamus (54.1% and 64.6%, respectively) and epididymal fat (47.8% and 60.1%, respectively) in HF/HS diet fed rats compared to the positive control. Notably, AAEN treatment displayed a marked (p < 0.05) attenuation effect compared to AAE treatment.
Histological changes in epididymal adipose tissue in different groups
Epididymal fat sections from the negative control rats and the rats from the AAE and AAEN groups demonstrated the typical histological architecture of adipocytes (), respectively. Otherwise, epididymal fat in the HF/HS diet-fed rats depicted hypertrophy of adipocytes, congested blood vessels, and mononuclear cell infiltration (). On the other hand, sections of epididymal fats of the rats from either the HF/HS+AAE or HF/HS +AAEN groups exhibited notable regression of lesions with restoration of the architecture of adipose tissue to appear histologically normal (). The histopathological lesion scores in the rats’ epididymal adipose tissues throughout all the experimental groups are shown in .
Figure 8. Representative photomicrographs of adipose tissue sections of rats: (a, b, and c) negative control, AAE, and AAEN groups, respectively, showing the normal histological architecture of adipocytes. (d) HF/HS group showing hypertrophy of adipocytes (black arrow), congested blood vessels (red arrow), and mononuclear cell infiltration (blue arrow) (H & E, scale bar, 100 μm). (e) and (F) HF/HS+AAE and HF/HS+AAEN groups restore adipose tissue architecture to appear histologically normal. (g), (h), and (i) Show the scores for histological lesions in the rats’ epididymal fat tissues throughout the various experimental groups.
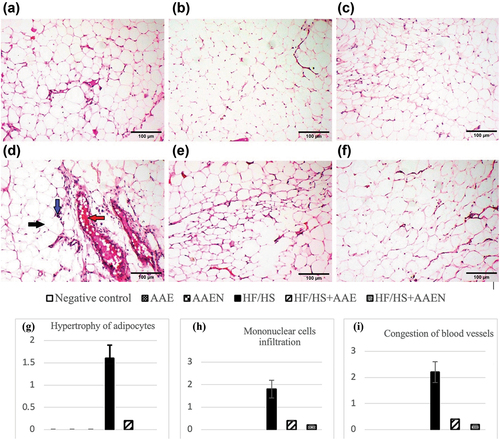
Oral glucose tolerance test
demonstrates the rats’ blood glucose levels following an oral glucose dose of 2 g/kg. Thirty minutes after supplying glucose, the blood glucose level rose in all groups, with a more remarkable rise in the HF/HS group compared to the negative control rats. Blood glucose levels were still elevated 60 min after glucose load in all experimental groups, with a considerable rise in the HF/HS-fed rats compared to the negative control rats. Two hours after the oral glucose bolus, rats in the negative control, AAE, and AAEN groups restored their basal glucose levels; meanwhile, blood glucose levels remained elevated in the HF/HS-fed rats. The HF/HS-fed rats treated with AAE and its nanoparticles displayed a notable decrease (p < 0.05) in blood glucose concentrations at 1/2 h, one h, and two h compared to positive control rats. Furthermore, the AUC was significantly (p < 0.05) higher (13.7%) in the HF/HS diet-fed rats than in the negative control. Both Artemisia and Artemisia nanoparticle supplementation normalized and significantly (p < 0.05) reduced the value of AUC in the HF/HS diet-fed rats by 13.27% and 16.26%, respectively, compared to the positive control ().
Figure 9. Effects of Artemisia extract and Artemisia extract nanoparticles on (a) Oral glucose tolerance test and (b) Area under the curve in rats fed on a basal diet or HF/HS diet. Data are displayed as the mean ± SD. The letters a, b, and c represent significant differences from the negative control, positive control, and HF/HS + AAE groups, respectively. †, #, and * demonstrate statistical significance at p < 0.05, p < 0.01, and p < 0.001, respectively, using one-way ANOVA followed by the Post hoc tests.
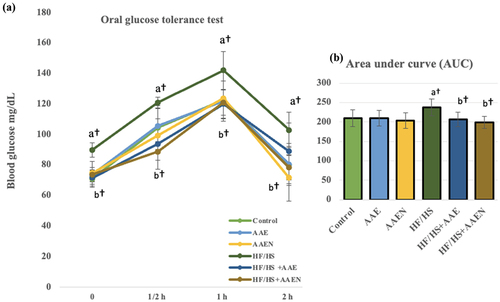
Serum glucose, insulin, and HOMA index
The results illustrated in revealed a significant (p < 0.001) upsurge in serum glucose (2.5-fold), insulin (1.8-fold), and HOMA index (8.89-fold) in HF/HS-fed rats compared to normal rats. Both tested treatments counteracted the HF/HS diet-induced insulin resistance with effective normalization achieved by AAEN treatment as manifested by the significant (p < 0.001) decrease in serum glucose (44.1% and 65.5%), insulin (31.9% and 55.85%), and HOMA index (61.9% and 84.7%) in the HF/HS diet fed-rats treated with AAE and AAEN, respectively, compared to the positive control ().
Figure 10. Effects of Artemisia extract and Artemisia extract nanoparticles on (a) Serum glucose, (b) Serum insulin (c) HOMA index and relative mRNA gene expression of (d) IRS 1 in the hypothalamus (e) IRS 1 in epididymal fat and (f) GLUT4 in epididymal fat in rats fed on a basal or HF/HS diet. Data are displayed as the mean ± SD. The letters a, b, and c represent significant differences from the negative control, positive control, and HF/HS + AAE groups, respectively. †, #, and * demonstrate statistical significance at p < 0.05, p < 0.01, and p < 0.001, respectively, using one-way ANOVA followed by the Post hoc tests.
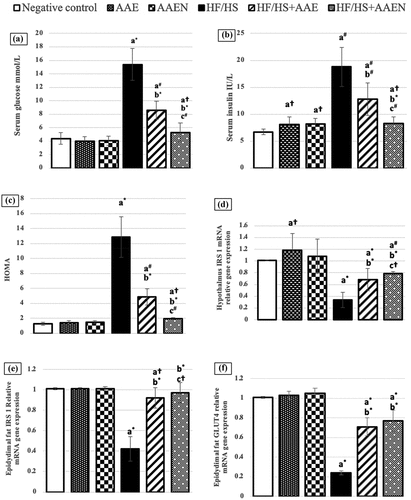
HF/HS diet consumption incited a dramatic downregulation in IRS 1 gene expression in the hypothalamus (66.3%) and epididymal fat (58.4%) as well as a substantial (p < 0.001) downregulation in the mRNA level of GLUT4 (76.2%) in epididymal fat compared to the negative control. These alterations in gene expression levels were effectually reinstated by the tested treatments, with marked improvement accomplished by AAEN treatment. Oral supplementation with AAE and AAEN elicited a significant (p < 0.001) increase in IRS 1 gene expression in the hypothalamus (one- and 1.323-fold) and IRS 1 (1.19- and 1.309-fold) and GLUT4 (1.95% and 2.2-fold), in the epididymal fat of the rats fed the HF/HS diet as compared to their corresponding values in the positive control ().
Liver function parameters
The observed changes in liver function tests in different groups are illustrated in . HF/HS-fed rats exhibited a deteriorated liver function manifested by dramatic elevation (p < 0.001) in the serum activities of AST, ALT, and ALP (1.56-, 3-, and 1.02- fold, respectively) compared to negative control rats. Oral supplementation of HF/HS diet-fed rats with AAE and AAEN achieved substantial alleviation of such alterations as manifested by the substantial decline in the AST (33.39% and 43.2%, p < 0.001), ALT (44.8% and 65.8%, p < 0.001), and ALP (29.6%, p < 0.01 and 39.7%, p < 0.001) activities compared to the corresponding levels in the positive control rats. However, it is worth noting that oral supplementation with the AAEN elicited higher efficacy in restoring liver function than the AAE supplementation and that the two extracts had no effects on the activity of these enzymes in normal rats.
Figure 11. Effects of Artemisia extract and Artemisia extract nanoparticles on liver function (a) Serum AST activity, (b) Serum ALT activity, and (c) Serum ALP activity in rats fed on a basal diet or HF/HS diet. Data are displayed as the mean ± SD. The letters a, b, and c represent significant differences from the negative control, positive control, and HF/HS + AAE groups, respectively. †, #, and * demonstrate statistical significance at p < 0.05, p < 0.01, and p < 0.001, respectively, using one-way ANOVA followed by the Post hoc test.
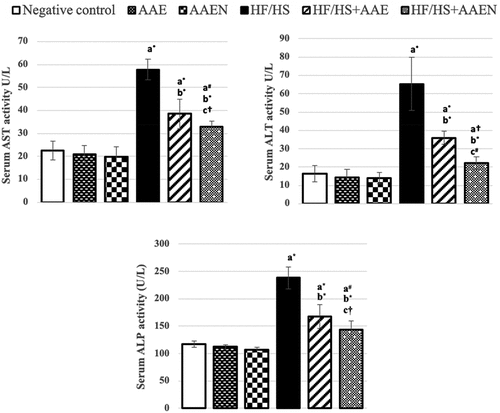
Histological changes in liver tissue in different groups
Light microscopic examination of hepatic sections of negative control rats and rats in the AAE and AAEN groups depicted the typical histoarchitecture of the hepatic lobules comprising normal central veins and normal liver cells (, respectively). In contrast, the liver sections of rats from the HF/HS group showed numerous histopathological lesions characterized by Kupffer cell activation, hepatocellular vacuolization, edema in the portal triad in line with fibroplasia, newly formed bile ductules, and inflammatory cell infiltration (). Otherwise, the hepatic tissues of the rats in the HF/HS + AAE group showed an improvement in the histological appearance; the examined sections displayed slight activation of Kupffer cells, hepatocellular vacuolization of some centrilobular hepatic cells, and sparse necrosis of hepatocytes (). Furthermore, marked regression of the histopathological damage with restoration of the normal histology of hepatic tissue was recorded in the liver of the rats from the HF/HS +AAEN group. Hepatic sections from this group exhibited slight central vein congestion and little vacuolization of sporadic hepatocytes (). ) demonstrate the histopathological lesion scores in the hepatic tissues of the rats in the different experimental groups.
Figure 12. Photomicrographs of the liver sections of rats in a) negative control, illustrating the normal histological arrangement of the hepatic lobule, (b) and (c) Rats from the AAE and AAEN groups showed the absence of histopathological changes, respectively, (d) Positive control (HF/HS) group, showing hepatocellular vacuolization (black arrow), fibroplasia (red arrow), and inflammatory cell infiltration in the portal triad (blue arrow), (e) HF/HS + AAE group, showing hepatocellular vacuolization of some centrilobular hepatocytes (black arrow) and sparse necrosis of hepatocytes (red arrow), (f) HF/HD+AAEN group, showing slight congestion of central vein (blue arrow) and slight vacuolization of sporadic hepatocytes (black arrow) (h & e, scale bar, 100 μm). (g), (h), (i), and (j) Show the histological lesion scores in the rats’ liver tissue within all of the experimental groups.
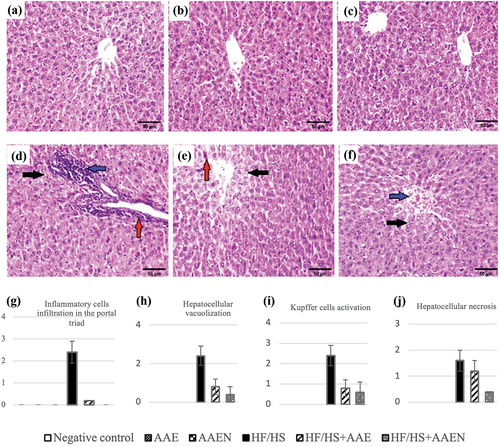
Oxidative stress and inflammatory biomarkers
As shown in , the rats that consumed the HF/HS diet displayed a significant (p < 0.001) increase in lipid peroxidation, as evidenced by a substantial spike in serum MDA (3-fold the negative control value), which was effectively reinstated (p < 0.001) by treatment of HF/HS-fed rats with AAE (48.6%) and AAEN (66.59%) compared to the positive control rats. On the other hand, the antioxidant activities were compromised in the HF/HS diet-fed rats, as evidenced by a notable (p < 0.001) decline in the SOD activity (68.9%) compared to the negative control group. Such a decline in serum SOD activity was notably (p < 0.05) abrogated by the two tested treatments, AAE (1.11-fold) and AAEN (2.02-fold), compared to the positive control rats. A more efficient effect was achieved by the AAEN treatment, which returned SOD activity to normal level ().
Figure 13. Effects of Artemisia extract and Artemisia extract nanoparticles on oxidative stress parameters (A) serum MDA and (B) serum SOD activity, and inflammatory markers in epididymal fat (C) TNF- α, (D) IL- 1β and (E) iNOS in rats fed on a basal diet or HF/HS diet. Data are displayed as the mean ± SD. The letters a, b, and c represent significant differences from the negative control, positive control, and HF/HS + AAE groups, respectively. †, #, and * demonstrate statistical significance at p < 0.05, p < 0.01, and p < 0.001, respectively, using one-way ANOVA followed by the Post hoc test.
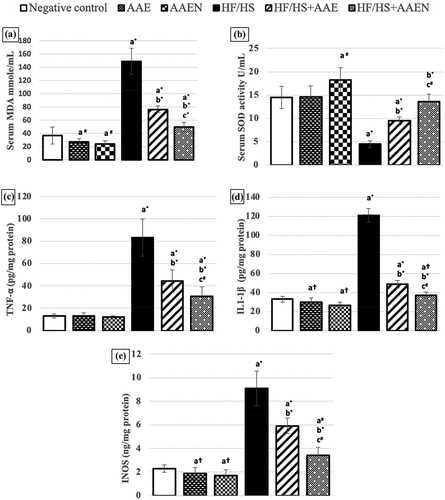
An intense inflammatory response was elicited by feeding the HF/HS diet as manifested by a dramatic elevation (p < 0.001) in the levels of TNF-α (5.45-fold), IL-1β (2.66-fold), and iNOS (2.95-fold) in adipose tissue compared with the normal rats. Oral administration of AAE or AAEN mitigated the HF/HS-induced inflammation through a considerable decrease (p < 0.001) in the epidydimal fat TNF-α (46.8% and 63.2%), IL-1β (59.8% and 69.2%), and iNOS (35.1% and 62.6%), respectively, in contrast to the respective values in the HF/HS diet-fed rats ().
Discussion
Obesity-related metabolic complications are a growing health problem in numerous societies worldwide. Excessive intake of a diet high in fat and sugars substantially contributes to these health problems. The abnormal expansion of adipose tissue confers intense secretion of pathologic substances, including adipocytokines, involved in insulin resistance, inflammatory responses, and metabolic illnesses. Thus, the antiadipogenic agents may provide therapeutic promise against obesity-associated complications [Citation11,Citation15]. Artemisia annua L. (AA) is a medicinal herb with antioxidant, anti-inflammatory, and antiobesity effects [Citation16]. These biomedical activities of AA may be due to its chemical constituents, which include coumarins, flavonoids, and terpenoids [Citation15]. Considering that these constituents are a part of compounds with low solubility in water, their absorption could be restricted when administered orally [Citation24]. Nanoparticle formulation is an innovative approach to drug delivery with numerous benefits, such as improving the drug’s solubility, accelerating its dissolution rate, enhancing its bioavailability, and lowering the dosage needed to achieve the same effects [Citation25]. Previous research has demonstrated that the same dose of nanoparticle formulation had a superior pharmacological impact compared to its conventional drug [Citation47].
Models of experimental obesity utilizing a hypercaloric (HF/HS) diet have been broadly exploited to study the etiological characteristics of obesity in humans [Citation36,Citation39,Citation48]. In line with the preceding studies, this investigation confirmed that excessive consumption of fat associated with sugar might result in an oversupply of energy, causing an expansion in adipose tissue, eventually malfunctioning adipose tissue, and metabolic inflammation. Elevated circulating levels of free fatty acids can result in lipotoxicity to peripheral organs [Citation48], and the excessive inflow of such fatty acids into the liver produces VLDL triacylglycerols that aid in developing dyslipidemia; consequently, these deteriorations may result in metabolic syndromes [Citation39,Citation48]. Furthermore, HF/HS-fed rats exhibited hyperglycemia and insulin resistance, which might be associated with the decline in insulin receptors and the functioning of the glucose transport system [Citation36].
This study revealed that Artemisia annua ethanolic extract and its nanoformulation elicited antiadipogenic effects and significantly reduced final body weight and BMI in rats fed on HF/HS diet. Additionally, epididymal fat weights were markedly reduced in the Artemisia-treated rats. In addition, histological examination of adipocytes showed smaller fat cells in the epididymal fat tissue of both Artemisia-supplemented groups compared to that in the HF/HS diet group, indicating that the decreased body weight was due to reduced fat accumulation. This study’s findings also revealed that the extracts of AA showed hypolipidemic efficacy since AAE and AAEN-treated rats showed an enhanced lipid profile as Artemisia treatment significantly reduced serum triacylglycerols, total cholesterol, LDL, and VLDL cholesterol and increased HDL in the serum compared to the HF/HS diet-fed rats. These findings support several previous studies in which AA reduced the rate of weight gain and the plasma levels of TAG and total cholesterols in rats and mice, suggesting the use of AA as a complementary treatment to control obesity [Citation18,Citation21,Citation23]. Likewise, Artemisia herba-alba Asso had antihyperlipidemic effects in diabetic mice induced by an HFD [Citation49]. Additionally, intragastric administration of AA aqueous extract lowered the LDL/HDL ratio in alloxan-induced diabetic rats [Citation21]. Similarly, in streptozotocin (STZ)-induced diabetic rats, oral administration of Artemisia indica methanolic extract for 15 days alleviated dyslipidemia [Citation50].
To gain insight into the molecular mechanism underlying Artemisia extracts’ anti-obesity effects, the expressions of genes related to lipid metabolism and obesity, like PPAR-γ, FAS, LPL SIRT-1, PGC-1α, and SREBP-1, were examined. In the current investigation, mRNA levels of genes encoding the lipogenic proteins PPAR-γ and FAS were downregulated in the epididymal fat of Artemisia-treated rats, suggesting an effect of AA supplementation on de novo lipogenesis. PPARγ plays an essential role in adipocyte differentiation and increases the expression of genes implicated in free fatty acid uptake and subsequent triacylglycerol synthesis, increasing adipose tissue’s capacity to store lipids [Citation33]. White adipose tissue is reduced or impaired with PPAR-γ knockout in mice [Citation51]. The downregulation of PPAR-γ by pharmacological inhibitors reduced the accumulation of lipids during adipocyte differentiation [Citation52]. Additionally, AAE and AAEN oral treatments downregulated the expression of FAS, an enzyme involved in the metabolism and synthesis of fatty acids under the effect of PPAR-γ; hence, its expression is markedly upregulated when fatty acid synthesis rates are high in cells [Citation51]. Furthermore, LPL mRNA levels were decreased in AA-treated rats. This lipolytic enzyme mediates the internalization and deposition of the circulated lipids into peripheral organs, as it is the principal enzyme that promotes chylomicron- and triacylglycerol lipolysis [Citation53]. A surplus of the circulated lipids from the HF/HS diet may have triggered the upregulation of LPL; it has been proven that upregulation of LPL in the liver and skeletal muscle raises ectopic fat and leads to insulin resistance [Citation54]. Additionally, oral administration of AAE and its nanoformulation reduced the expression of hepatic SREBP-1. The lipogenic transcription factor SREBP-1 is essential in regulating fatty acid synthesis by controlling the expression of acetyl-CoA carboxylase and FAS [Citation55]. Contrariwise, the expression of hepatic SIRT 1 and PGC-1α was enhanced. It was reported that the increase in the SIRT1 activity upregulates gene-related metabolic functions, promotes insulin sensitivity, and reduces inflammatory gene expressions in the adipose tissue of diet-induced obese animals [Citation56]. In addition, PGC-1α, a key regulator for mitochondrial biogenesis and function, plays a central role in thermogenesis [Citation56]. These results indicate that AA affects lipid metabolism regulation and fat cell differentiation in HF/HS-fed rats. Our results align with those reported by Hwang et al. [Citation57], who demonstrated that essential oils from Artemisia annua might suppress 3T3-LI cell differentiation by inhibiting adipogenesis and activating lipid metabolism-related proteins. Likewise, suppression of the differentiation of adipocytes has been demonstrated for artesunate and artemisinic acid, small bioactive compounds extracted from Artemisia annua [Citation22,Citation23]. Artemisinic acid downregulates PPARγ expression, which in turn downregulates the expression of C/EBPα and lipoprotein lipase [Citation23]. Artesunate also reduces the expression of PPAR-γ, C/EBP-α, and FAS during the differentiation of adipocytes and inhibits triacylglycerol synthesis and fat accumulation in 3T3-L1 preadipocytes [Citation22]. Therefore, the reduced body and epididymal fat weights and the improved lipid profile observed in the rats treated with AAE and AAEN could be explained by the decreased lipogenesis due to the downregulation of the lipogenic transcription factors PPARγ, FAS, and SREBP-1 and the upregulation of SIRT 1 and PGC-1α that enhance thermogenesis.
Adipose tissue secretes a wide range of adipokines, such as leptin and adiponectin, which are involved in the endocrine regulation of energy balance [Citation58]. In this investigation, the antilipogenic influence of AA was accompanied by a reduction in the circulating leptin and an increase in adiponectin. The results of this study also demonstrate that Artemisia extracts decreased the regulation of leptin receptors in the hypothalamus and epididymal fats. Serum leptin levels are intimately related to fat mass, as demonstrated in human and rat research [Citation57]. Therefore, the decrease in circulating leptin seen in the current investigation might be related to the reduction in fat mass due to AA administration. A growing body of evidence indicates that while adiponectin helps to regulate obesity, leptin overexpression or secretion is associated with obesity and diseases linked to obesity [Citation59]. Assuming that AA lowered serum leptin and increased adiponectin, it is suggested that AA could be beneficial in treating and preventing obesity and associated metabolic disturbances in which overexpression of leptin is implicated.
Furthermore, the current investigation shows that Artemisia supplementation improved glucose utilization, manifested by the results from the OGTT and the reduction in serum glucose and insulin levels and HOMA-IR relative to the positive control group. Numerous research findings suggested that AA extracts or their biologically active components improve HOMA-IR. For example, the aqueous extract of AA decreased HOMA-IR in alloxan-induced diabetic rats [Citation21]. Women with gestational diabetes showed enhanced insulin sensitivity and reduced HOMA-IR after 10 weeks of treatment with Artemisia scoparia extract [Citation60]. Chronic oral treatment with Artemisia herba-alba for 18 weeks reduced elevated levels of HOMA-IR in mice with HFD-induced T2DM [Citation61]. Likewise, Kim et al. [Citation18] showed that Artemisia annua L. inhibited α-glucosidase activity in vitro even more effectively than acarbose, a known antidiabetic drug. Furthermore, myricetin, a flavonoid extracted from AA, enhanced insulin sensitivity in mice and rats fed high-fat diets [Citation62]. In the same context, the current study’s findings also revealed that treatment with Artemisia increased the gene expression of IRS 1 and GLUT4 in HF/HS diet-fed rats. GLUT4 is important in regulating glucose metabolism and maintaining glucose homeostasis in the body [Citation60–62]. The mechanisms proposed for the antidiabetic effect of Artemisia could be related to the upregulation of GLUT4 gene expression, with a consequent rise in GLUT4 protein levels, allowing for more substantial glucose transport inside cells, resulting in the notable improvement in insulin resistance [Citation60,Citation62]. Furthermore, the increased SIRT 1 and PGC-1α gene expression by Artemisia treatment could also contribute to enhanced glucose utilization and insulin sensitivity. SIRT 1 and PGC-1α are important insulin-dependent singling molecules that regulate glucose metabolism and enhance insulin sensitivity [Citation8,Citation9]. These molecular changes could explain the improved glucose utilization observed in the HF/HS diet-fed rats treated with AAE and AAEN.
Transaminase (AST and ALT) enzymes are employed as biomarkers to anticipate potential liver damage [Citation63]. Elevated serum transaminase activity indicated injury to the liver cells and the liver’s structural integrity. Therefore, increased serum transaminases and ALP activities in HF/HS diet-fed rats confirm damage to liver cells and the plasma membrane. Likewise, the histological analysis of liver sections from rats in the HF/HS group revealed many histopathological lesions, including hepatocellular vacuolization, activation of Kupffer cells, and edema linked to fibroplasia. In this study, rats fed on an HF/HS diet had higher serum triacylglycerol and cholesterol levels, which might initiate the development of lipotoxicity and lipid deposits in the liver [Citation63,Citation64]. Excessive lipid deposition in the liver is recognized as nonalcoholic fatty liver disease (NAFLD) that eventually leads to steatosis. Oral administration of AA and its nanoformulation attenuated the elevated activities of all investigated enzymes. They improved the histological picture of liver sections in obese rats compared to the positive control. These results may highlight the nontoxic properties of the extracts and their potential to mitigate liver damage due to HF/HS diet feeding.
Oxidative stress caused by consuming an HF/HS diet has been reported in numerous experimental models and clinical patients. Study evidence shows that consuming diets high in fat and sugars contributes to ROS production during the pathology of obesity and related metabolic complications [Citation63–65]. In alignment with previous studies revealing that animals fed a high-fat diet may have compromised antioxidant defenses [Citation18,Citation32,Citation65], this investigation showed that the activity of the cytoprotective enzyme SOD was decreased, and malondialdehyde, a product of lipid peroxidation, was increased, in the HF/HS diet-fed rats. It was suggested that excessive fat consumption accelerates the mitochondrial free fatty acid β-oxidation, which in turn causes an excess of electrons to be transferred via cytochrome c oxidase, increasing the generation of reactive oxygen species that oxidize unsaturated fatty acids in lipid deposits to cause lipid peroxidation. Furthermore, in hyperglycemia, the consequent overload of intracellular glucose and the gradient of protons across the mitochondria’s inner membrane result in an electron escape with superoxide anion generation [Citation66]. In this study, AAE and AAEN oral treatments attenuated oxidative stress and ameliorated tissue antioxidants in high-fat diet-fed rats. The antioxidant property of AA could be ascribed to the phenolic substances, including flavonoids and coumarins, which are antioxidant-active electrophiles that can activate the nuclear factor erythroid 2–related factor 2 (Nrf2) signaling pathway to produce phase 2 antioxidant enzymes [Citation67].
In the current investigation, the HF/HS diet evoked an intense state of inflammation manifested by the substantial elevation in the inflammatory markers TNF-⍺, IL-1 β, and iNOS in epidydimal fat. The histological examination of liver and epididymal fat sections from the HF/HS group consistently demonstrated inflammatory cell infiltration. Previous studies have suggested that adipocyte hypertrophy may have a role in constricted blood flow, which causes hypoxia, and infiltration and activation of macrophages (a source of reactive oxygen species), which induce inflammation and release proinflammatory adipokines into the circulation [Citation68]. In addition, hyperleptinemia triggers macrophage activation and produces TNF-α and NO synthase [Citation67]. Furthermore, elevated leptin levels cause oxidative stress and ROS generation [Citation69]. The production of ROS by high-fat diets has the potential to initiate proinflammatory signaling and activate the transcription factor nuclear factor kappa (NF-κB), which can lead to the induction of proinflammatory molecules that are dependent on NF-κB, including TNF-α, iNOS, and interferon-γ (IFN-γ) [Citation68]. A growing body of evidence has demonstrated that the development of persistent mild inflammation exacerbates adipose tissue malfunction, which is linked to obesity-associated insulin resistance [Citation18,Citation21,Citation49]. Type 2 diabetes-associated insulin resistance and adipocytokines have been related. Insulin signaling is compromised by TNF-α, both directly and indirectly, through an increase in free fatty acid production [Citation63]. In this investigation, oral administration of AAE and AAEN diminished the production of the inflammatory cytokines, TNF-α, IL-1β, and iNOS and attenuated the obesity-induced inflammation. However, the efficacy of AAEN treatment in reducing these cytokines was markedly higher than that of the original extract. Based on the above-mentioned findings, reducing the inflammatory cytokines, TNF-α, IL-1β, and iNOS, could explain the enhanced insulin sensitivity observed in this study. Consistent with this study’s findings, Artemisia dracunculus L. extract reduced proinflammatory cytokines and improved insulin sensitivity in human skeletal muscle culture [Citation70]. Additionally, this herb reduced macrophage IL-6 production [Citation71]. Another study revealed a decrease in the levels of proinflammatory cytokines such as TNF-α in HFD-STZ diabetic rats after 28 days of treatment with Artemisia judaica extract [Citation20]. These anti-inflammatory properties of AA observed in this study could be attributed to the presence of bioactive constituents such as polyphenols and flavonoids, as well as essential oils, which have been widely documented to possess anti-inflammatory characteristics [Citation69,Citation72].
Based on the aforementioned results, Artemisia extracts reduced body weight, epididymal fat, hyperlipidemia, hyperglycemia, serum leptin, and insulin resistance, as well as increased serum adiponectin, improved glucose, utilization, and reduced oxidative stress and inflammatory markers. These physiological outcomes are mediated by regulating genes encoding proteins involved in the regulation of lipid and glucose metabolism, like PPAR-γ, FAS, LPL SIRT-1, PGC-1α, and SREBP-1. Histologically, Artemisa extract treatments also demonstrated antiadipogenic and anti-inflammatory effects, evidenced by reduced adipocyte size in epididymal adipose tissue and low inflammatory cell infiltration in the epididymal adipose and hepatic tissues.
The antiadipogenic properties of Artemisia annua L reported in the current investigation might be attributed to the bioactive compounds in the Artemisia extract. Preliminary studies have demonstrated that AA contains significant phenolic compounds, such as rutin, kaempferol, quercetin, luteolin, and luteolin-7-glucoside [Citation15,Citation16]. Moreover, AA is abundant in sesquiterpenes and flavonoids, which are probably capable of inducing antioxidant enzymes and exhibiting in vivo antioxidative action [Citation73]. Myricetin, one of the flavonoids in the AA extract, may improve insulin sensitivity and act as an antioxidant [Citation18]. Furthermore, anti-inflammatory constituents in AA decrease the proinflammatory cytokine production [Citation69]. Moreover, the plant is well-known for its essential oils, mostly composed of camphor, trans-pinocarveol, b-selinene, b-caryophyllene, and Artemisia ketone. The essential oil significantly reduced the growth of gram-positive bacteria and fungi [Citation74]. Additionally, modest antioxidant action has been demonstrated by the Artemisia genus’s oils [Citation75].
In this work, the sonochemical nanoformulation of Artemisia annua L ethanolic extract boosted its positive benefits in reducing obesity-related metabolic dysfunction. The findings of this research displayed that nanoformulations of Artemisia extract had more pronounced antiadipogenic, hypolipidemic, hypoglycemic, and insulin-sensitizing effects and better-potentiated antioxidant and anti-inflammatory properties than the conventional extract. Consistent with this study, Salem et al. [Citation33] used a sonochemistry technique to nanoformulate the herbal tea mixture extract and evaluated its in vivo anti-obesity efficacy compared to the conventional extract. They reported that tea mixture nanoformulation is a hopeful therapeutic agent for the treatment of obesity. Sonochemistry is a laboratory approach for producing nanomaterials. This technique uses acoustic waves to induce cavitation events, which drive chemical reactions that result in the growth of nanoscale materials. Cavitation is the process by which microbubbles in a liquid rapidly store ultrasonic energy, grow, and eventually collapse, returning the ultrasonic energy into the environment [Citation28,Citation33]. Sonochemistry rarely requires harsh chemicals or organic solvents and is among the greenest techniques for synthesizing nanomaterials. Additionally, the energy required for the production of the ultrasonic is minimal compared to the energy required for other systems.
The enhanced beneficial effects of the green synthesized Artemesia nanoparticles might be related to the increased surface area to volume ratio of the nanoformulated extract. This large surface area directly results from their nanoscale dimensions and spherical morphology, as marked in the electron microscopy images in this study. The high surface area to volume ratio significantly impacts nanoparticle solubility, reactivity, dissolution kinetics, and interaction capacity with target molecules or biological systems. Furthermore, the large surface area increases the adhesion area between the nanoionized herbal extract and the mucin layer lining the microvilli of the small intestine. It thus enhances the absorption rate of the Artemisia extract nanoparticles [Citation25–27]. In this regard, numerous studies have shown that nanoformulations of plant extracts have shown a notable enhancement in their antidiabetic properties compared with conventional extracts [Citation76]. Likewise, the antibacterial characteristics of plant extract nanoparticles have offered excellent benefits for improving the complications related to diabetic wound healing [Citation25]. Consistently, the antihyperlipidemic potential of medicinal plants has shown the most significant impact on dyslipidemia when applied in nanoemulsion form [Citation24]. In addition, many previous studies confirmed that most phytomedicines could be effectively formulated by various nano-delivery techniques and thus successfully delivered to achieve the needed therapeutic effect [Citation24,Citation47]. Consequently, the nanoparticle system can be used in other inadequately soluble herbal medicines and is expected to enhance their solubility and rate of absorption.
The limitations of this study are associated with the translatability of findings from an animal model to humans. This study was carried out in rodents. Humans might respond differently. Animal studies investigating medicinal herbs are often misinterpreted because there is insufficient information on the predictability, human dose equivalent, and potential benefit. Therefore, additional research is needed in clinical trials. In addition, there is a lack of a precise mechanism for alleviating obesity and its complications. The proposed different mechanisms collectively contribute and cooperate to improve the complications of obesity. However, some regulated mechanisms likely contribute more to the treatment than others. Future in-depth mechanistic investigations are needed to resolve these issues.
Conclusion
The current investigation demonstrates the valuable potential of Artemisia annua L. extract in counteracting obesity-related metabolic complications induced by feeding a hypercaloric diet. This study shows that Artemisia extract had antiadipogenic and hypolipidemic effects, enhanced glucose utilization, reduced leptin and insulin resistance, and improved liver function by regulating the expression of relevant genes in lipid and carbohydrate metabolism and exerting antioxidant and anti-inflammatory effects. Nanoformulation of this extract using the sonochemical method boosted its anti-obesity efficacy, suggesting that the AAE nanoparticle can be an appropriate option for adjunctive therapy for obesity management. However, further studies are needed to validate these results, confirm their safety, and promote the development and application of novel herbal nanoparticles beneficial to human health.
Abbreviations
AA | = | Artemisia anuua L. |
AAE | = | Artemisia anuua L. extract |
AAEN | = | Artemisia anuua L. extract nanoparticles |
ALP | = | Alkaline phosphatase |
ALT | = | Alanine transaminase |
AST | = | Aspartate transaminase |
BET | = | Brunauer, Emmett, and Teller |
FAS | = | Fatty acid synthase |
HDL | = | High-density lipoprotein |
HF/HS | = | High-fat/high-sucrose |
GLUT4 | = | Glucose transporter protein type 4 |
IFN-γ | = | Interferon-γ |
HOMA-IR | = | Homeostasis model assessment |
iNOS | = | Inducible nitric oxide synthase |
IL-1β | = | Interleukin-1β |
LDL | = | Low-density lipoprotein |
IRS 1 | = | Insulin receptor substrate 1 |
MetS | = | Metabolic syndrome |
LPL | = | Lipoprotein lipase |
Nrf2 | = | Nuclear factor erythroid 2–related factor 2 |
NF-κB | = | Nuclear factor kappa |
PGC-1α | = | Peroxisome proliferator-activated receptor gamma coactivator 1- α |
OB R | = | Leptin receptor |
qPCR | = | Quantitative real-time polymerase chain reaction |
PPAR-γ | = | Peroxisome proliferator-activated receptors- γ |
SIRT-1 | = | Sirtuin 1 |
SEM | = | Scanning Electronic Microscope |
SREBP-1 | = | Sterol regulatory element-binding protein 1 |
SOD | = | Superoxide dismutase |
STZ | = | Streptozotocin |
T2DM | = | Type 2 diabetes |
TC | = | Total cholesterol |
TAG | = | Triacylglycerol |
TNF-⍺ | = | Tumor necrosis factor-α |
TEM | = | Transmission Electronic Microscope |
XRD | = | X-ray diffraction |
VLDL | = | Very low-density lipoprotein |
Authors’ contributions
MMM: Conceptualization, Investigation, Methodology, Data curation, Formal analysis, Writing-original draft, Validation, Writing-review. EAKM: Conceptualization, Investigation, Methodology. LAR: Methodology, Writing-original draft. KAA: Methodology, Writing-original draft. SHI: Methodology Writing-original draft. All authors read and approved the final manuscript.
Availability of data and materials
All data generated or analyzed during this study are included in this published article.
Ethics approval
All methods in the current research were approved by the Ethics Committee in Women’s College, Ain Shams University, Egypt (approval No. sci1532309001).
Acknowledgements
The authors express their gratitude for the technical support from the Laboratory of Biochemistry and Molecular Biology, Faculty of Medicine, Cairo University, Cairo, Egypt. We also thank the Faculty of Nanotechnology for Postgraduate Studies, Cairo University, for technical help in preparing and characterizing the extract nanoparticles.
Disclosure statement
No potential conflict of interest was reported by the author(s).
Additional information
Funding
References
- Li A, Shi W, Wang J, et al. The gene knockout of angiotensin II type 1a receptor improves high-fat diet-induced obesity in rat via promoting adipose lipolysis. PLoS One. 2022. doi: 10.1371/journal.pone.0267331
- Lugarà R, Renner S, Wolf E, et al. Crossbred sows fed a western diet during pre-gestation, gestation, lactation, and post-lactation periods develop signs of lean metabolic syndrome that are partially attenuated by spirulina supplementation. Nutrients. 2022;14(17):3574. doi: 10.3390/nu14173574
- Gabbia D, Roverso M, Guido M, et al. Western diet-induced metabolic alterations affect circulating markers of liver function before the development of steatosis. Nutrients. 2019;11(7):1602. doi: 10.3390/nu11071602.
- Kobi JBBS, Matias AM, Gasparini PVF. et al. High-fat, high-sucrose, and combined high-fat/high-sucrose diets effects in oxidative stress and inflammation in male rats under presence or absence of obesity. Physiological Reports 2023;11(7). doi: 10.14814/phy2.15635
- Evans RM, Barish GD, Wang YX. Ppars and the complex journey to obesity. Nat Med. 2004;10(4):355–361. doi: 10.1038/nm1025
- Eberle D, Hegarty B, Bossard P, et al. SREBP transcription factors: master regulators of lipid homeostasis. Biochimie. 2004;86(11):839–848. doi: 10.1016/j.biochi.2004.09.018
- Horton JD, Shimomura I, Ikemoto S, et al. Overexpression of sterol regulatory element-binding protein-1a in mouse adipose tissue produces adipocyte hypertrophy, increased fatty acid secretion, and fatty liver. J Biol Chem. 2003;278(38):36652–36660. doi: 10.1074/jbc.M306540200
- Knight JRP, Jo M. SIRT1, metabolism and cancer. Curr Opin Oncol. 2012;24(1):68–75. doi: 10.1097/CCO.0b013e32834d813b
- Coulter AA, Rebello CJ, Greenway FL. Centrally acting drugs for obesity: past, present, and future. Drugs. 2018;78(11):1113. doi: 10.1007/s40265-018-0946-y
- Hasani-Ranjbar S, Larijani B, Abdollahi M. A systematic review of Iranian medicinal plants useful in diabetes mellitus. Arch Med Sci. 2008;4:285–292.
- Naseri R, Farzaei F, Haratipour P, et al. Anthocyanins in the management of metabolic syndrome: a pharmacological and biopharmaceutical review. Front Pharmacol. 2018;9. doi: 10.3389/fphar.2018.01310
- Kim S, Lee MS, Jung S, et al. Ginger extract ameliorates obesity and inflammation via regulating microRNA-21/132 expression and AMPK activation in white adipose tissue. Nutrients. 2018;10(11):1567. doi: 10.3390/nu10111567
- Metwally FM, Rashad HM, Ahmed HH, et al. Molecular mechanisms of the anti-obesity potential effect of moringa oleifera in the experimental model. Asian Pac J Trop Biomed. 2017;7(3):214–221. doi: 10.1016/j.apjtb.2016.12.007
- Taghikhani A, Afrough H, Ansari-Samani R, et al. Assessing the toxic effects of hydroalcoholic extract of Stachys lavandulifolia Vahl on rat’s liver. Bratisl Lek Listy. 2014;115(3):121–124. doi: 10.4149/BLL_2014_026
- Rashid S, Rather MA, Shah WA, et al. Chemical composition, antimicrobial, cytotoxic and antioxidant activities of the essential oil of Artemisia indica willd. Food Chem. 2013;138(1):693–700. doi: 10.1016/j.foodchem.2012.10.102
- Kim MH, Seo JY, Liu KH, et al. Protective effect of Artemisia anuua l. extract against galactose-induced oxidative stress in mice. PLoS One. 2014;9(7):e101486. doi: 10.1371/journal.pone.0101486
- Qin DP, Li HB, Pang QQ, et al. Structurally diverse sesquiterpenoids from the aerial parts of Artemisia anuua (Qinghao) and their striking systemically anti-inflammatory activities. Bioorg Chem. 2020;103:104221. doi: 10.1016/j.bioorg.2020.104221
- Kim KE, Ko KH, Heo RW, et al. Artemisia anuua leaf extract attenuates hepatic steatosis and inflammation in high-fat diet-fed mice. J Med Food. 2016;19(3):290–299. doi: 10.1089/jmf.2015.3527
- Kartikadewi A, Prasetyo A, Budipradigdo L, et al. Artemisia anuua leaf extract increases GLUT-4 expression in type 2 diabetes mellitus rat. Indo Biomed J. 2019;h11(1):78–84.
- Albasher G, Alwahaibi M, Abdel-Daim MM, et al. Protective effects of Artemisia judaica extract compared to metformin against hepatorenal injury in high-fat diet/streptozotocine-induced diabetic rats. Environ Sci Pollut Res Int. 2020;27(32):40525–40536. doi: 10.1007/s11356-020-09997-2
- Helal E, Abou-Aouf N, Khattab AM, et al. Anti-diabetic effect of Artemisia anuua (kaysom) in alloxan-induced diabetic rats. Egypt J Hosp Med. 2014;31:1–16. doi: 10.12816/0008476
- Jang B. Artesunate inhibits adipogeneis in 3T3-L1 preadipocytes by reducing the expression and/or phosphorylation levels of C/EBP-α, PPAR-γ, FAS, perilipin A, and STAT-3. Biochem Biophys Res Commun. 2016;474(1):220–225. doi: 10.1016/j.bbrc.2016.04.109
- Lee J, Kim MH, Lee JH, et al. Artemisinic acid is a regulator of adipocyte differentiation and C/EBP δ expression. J Cell Biochem. 2012;113(7):2488–2499. doi: 10.1002/jcb.24124
- Merisko-Liversidge E, Liversidge GG, Cooper ER. Nanosizing: a formulation approach for poorly-water-soluble compounds. Eur J Pharmaceut Sci. 2003;18(2):113–120. doi: 10.1016/S0928-0987(02)00251-8
- Singla R, Soni S, Kulurkar PM, et al. In situ functionalized nanobiocomposites dressings of bamboo cellulose nanocrystals and silver nanoparticles for accelerated wound healing. Carbohydr Polym. 2017;155:152–162. doi: 10.1016/j.carbpol.2016.08.065
- Liao C, Hung W, Jan K, et al. Nano/sub-microsized lignan glycosides from sesame meal exhibit higher transport and absorption efficiency in caco-2 cell monolayer. Food Chem. 2010;119(3):896–902. doi: 10.1016/j.foodchem.2009.07.056
- Jia L. Nanoparticle formulation increases oral bioavailability of poorly soluble drugs: approaches experimental evidences and theory. Current Nanoscience; 2005. doi: 10.2174/157341305774642939
- Huston M, DeBella M, DiBella M, et al. Green synthesis of nanomaterials. Nanomaterials. 2021 doi: 10.3390/nano11082130
- Bharadwaj KK, Rabha B, Pati S, et al. Green synthesis of gold nanoparticles using plant extracts as beneficial prospect for cancer theranostics. Molecules (Basel, Switzerland); 2021. doi: 10.3390/molecules26216389
- Karimi B, Mardani M, Kaboutari J, et al. Green synthesis of copper oxide nanoparticles using Artemisia annua aqueous extract and its characterization, antioxidant, and burn wound healing activities. Chem Pap. 2024;78(1):231–243. doi: 10.1007/s11696-023-03069-8
- Aghajanyan A, Gabrielyan L, Schubert R, et al. Silver ion bioreduction in nanoparticles using Artemisia anuua L. extract: characterization and application as antibacterial agents. AMB Express; 2020. doi: 10.1186/s13568-020-01002-w
- Ahmed-Farid OA, Abdelrazek AM, Elwakel H, et al. Hordeum vulgare ethanolic extract mitigates high salt-induced cerebellum damage via attenuation of oxidative stress, neuroinflammation, and neurochemical alterations in hypertensive rats. Metab Brain Dis; 2023. doi: 10.1007/s11011-023-01277-5
- Salem MA, Aborehab NM, Abdelhafez MM, et al. Anti-obesity effect of a tea mixture nano-formulation on rats occurs via the upregulation of AMP-activated protein kinase/sirtuin-1/glucose transporter type 4 and peroxisome proliferator-activated receptor gamma pathways. Metabolites;2023. doi: 10.3390/metabo13070871.
- Mahboub HH, Eltanahy A, Omran A, et al. Chitosan nanogel aqueous treatment improved blood biochemicals, antioxidant capacity, immune response, immune-related gene expression and infection resistance of Nile tilapia. Comparative biochemistry and physiology. Comp Biochem Physiol B Biochem Mol Biol. 2024;269:110876. doi: 10.1016/j.cbpb.2023.110876
- Reeves PG, Nielsen FH, GCJr F. AIN-93 purified diets for laboratory rodents: final report of the American Institute of Nutrition ad hoc writing committee on the reformulation of the AIN-76A rodent diet. J Nutr. 1993;123(11):1939–1951. doi: 10.1093/jn/123.11.1939
- Moreno-Fernández S, Garcés-Rimón M, Vera G, et al. High fat/high glucose diet induces metabolic syndrome in an experimental rat model. Nutrients. 2018;10(10):1502. doi: 10.3390/nu10101502
- Alshehri MA. Cardioprotective properties of Artemisia herba alba nanoparticles against heart attack in rats: a study of the antioxidant and hypolipidemic activities. Saudi J Biol Sci. 2022;29(4):2336–2347. doi: 10.1016/j.sjbs.2021.12.009
- Abd Elwahab S, Abdel-Maksoud A, Sadak E, et al. (2022) Ethanolic extracts of grape and guava seeds ameliorate gentamicin-induced acute renal injury in rats. EGCHEM, doi: 10.21608/ejchem.2022166876.7056
- Tian X, Zhang Y, Li H, et al. Palmatine ameliorates high fat diet induced impaired glucose tolerance. Biol Res. 2020;53(1):39. doi: 10.1186/s40659-020-00308-0
- Fossati P, Prencipe L. Serum triglycerides determined colorimetrically with an enzyme that produces hydrogen peroxide. Clin Chem. 1982;28(10):2077–80. doi: 10.1093/clinchem/28.10.2077
- Guder WG, Fonseca-Wollheim FD, Heil WL, et al. The Haemolytic, Icteric and lipemic sample recommendations regarding their recognition and prevention of clinically relevant interferences. Recommendations of the Working Group on preanalytical variables of the. German Society for Clinical Chemistry and the German Society for Laboratory Medicine; 2000. doi: 10.1515/labm.2000.24.8.357
- Rifal N, Warnick GR, editors. Laboratory measurement of lipids, lipoproteins and apolipoproteins. Washington DC: American Association of Clinical Chemistry (AACC) 1994, p. 21–42.
- Friedewald WT, Levy RI, Fredrickson DS. Estimation of the concentration of low-density lipoprotein cholesterol in plasma, without use of the preparative ultracentrifuge. Clin Chem. 1972;18(6):499–502. doi: 10.1093/clinchem/18.6.499
- Livak KJ, Schmittgen TD. Analysis of relative gene expression data using real-time quantitative PCR and the 2− ΔΔCT method. Methods. 2001;25(4):402–408. doi: 10.1006/meth.2001.1262
- Suvarna SK, Layton C, Bancroft JD. Bancroft’s theory and practice of histological techniques. Oxford: Churchill Livingstone Elsevier. 2019.
- Javid S, Dilshad E. Artemisia carvifolia Buch silver nanoparticles downregulate the Rap2A gene in liver cancer. Sci Rep. 2023;13(1):21553. doi: 10.1038/s41598-023-48946-0
- Chen H, Wu J, Sun M, et al. N-trimethyl chitosan chloride-coated liposomes for the oral delivery of curcumin. J Liposome Res. 2012;22(2):100–109. doi: 10.3109/08982104.2011.621127
- Fenni S, Hammou H, Astier J, et al. Lycopene and tomato powder supplementation similarly inhibit high-fat diet-induced obesity, inflammatory response, and associated metabolic disorders. Mol Nutr Food Res. 2019;61(9):1601083. 2017. doi: 10.1002/mnfr.201601083
- Hamza N, Berke B, Cheze C, et al. Treatment of high fat diet induced type 2 diabetes in C57BL/6J mice by two medicinal plants used in traditional treatment of diabetes in the east of Algeria. J Ethnopharmacol. 2011;133(2):931–933. doi: 10.1016/j.jep.2010.11.019
- Ahmad W, Khan I, Khan MA, et al. Evaluation of the antidiabetic and antihyperlipidemic activity of Artemisia indica linn (aerial parts) in streptozotocin-induced diabetic rats. J Ethnopharmacol. 2014;151(1):618–623. doi: 10.1016/j.jep.2013.11.012
- Linhart HG, Ishimura-Oka K, DeMayo F, et al. C/EBPalpha is required for differentiation of white, but not brown, adipose tissue. Proc Natl Acad Sci USA. 2001. doi: 10.1073/pnas.211416898
- Rosen ED, MacDougald OA. Adipocyte differentiation from the inside out. Nat Rev Mol Cell Biol. 2006;7(12):885–896. doi: 10.1038/nrm2066
- Mead JR, Irvine SA, Ramji DP. Lipoprotein lipase: structure, function, regulation, and role in disease. J Mol Med. 2002;80(12):753–769. doi: 10.1007/s00109-002-0384-9
- Kim JK, Fillmore JJ, Chen Y, et al. Tissue-specific overexpression of lipoprotein lipase causes tissue-specific insulin resistance. Proc Natl Acad Sci USA. 2001. doi: 10.1073/pnas.121164498
- Yang ZH, Miyahara H, Takeo J, et al. Diet high in fat and sucrose induces rapid onset of obesity-related metabolic syndrome partly through the rapid response of genes involved in lipogenesis, insulin signaling, and inflammation in mice. Diabetol Metab Syndr. 2012;4(1). doi: 10.1186/1758-5996-432
- Nawaz A, Mehmood A, Kanatani Y, et al. Sirt1 activator induces proangiogenic genes in preadipocytes to rescue insulin resistance in diet-induced obese mice. Mice Sci Rep. 2018;8(1):11370. doi: 10.1038/s41598-018-29773-0
- Hwang DI, Won KJ, Kim DY, et al. Anti-adipocyte differentiation activity and chemical composition of essential oil from Artemisia anuua. Nat Prod Commun. 2016;11(4):539–542. doi: 10.1177/1934578X1601100430
- Fève B. Adipogenesis: cellular and molecular aspects. Best practice & research. J Clin Endocrinol Metab. 2005;19(4):483–499. doi: 10.1016/j.beem.2005.07.007
- Yadav A, Kataria MA, Saini V, et al. Role of leptin and adiponectin in insulin resistance. Clin Chim Acta. 2013;417:80–84. doi: 10.1016/j.cca.2012.12.007
- Sun X, Sun H, Zhang J, et al. Artemisia extract improves insulin sensitivity in women with gestational diabetes mellitus by up-regulating adiponectin. J Clin Pharmacol. 2016;56(12):1550–1554. doi: 10.1002/jcph.755
- Réggami Y, Benkhaled A, Boudjelal A, et al. Artemisia herba-alba aqueous extract improves insulin sensitivity and hepatic steatosis in rodent model of fructose-induced metabolic syndrome. Arch Physiol Biochem. 2021;127(6):541–550. doi: 10.1080/13813455.2019.1659825
- Liu IM, Tzeng TF, Liou SS, et al. Myricetin, a naturally occurring flavonol, ameliorates insulin resistance induced by a high-fructose diet in rats. Life Sci. 2007;81(21–22):1479–1488. doi: 10.1016/j.lfs.2007.08.045
- Cheraghpour M, Imani H, Ommi S, et al. Hesperidin improves hepatic steatosis, hepatic enzymes, and metabolic and inflammatory parameters in patients with nonalcoholic fatty liver disease: a randomized, placebo-controlled, double-blind clinical trial. Phytother Res. 2019;33(8):2118–2125. doi: 10.1002/ptr.6406
- Ulla A, Alam MA, Sikder B. et al. Supplementation of Syzygium cumini seed powder prevented obesity, glucose intolerance, hyperlipidemia and oxidative stress in high carbohydrate high fat diet induced obese rats. BMC Complement Altern Med 2017;17(1). doi: 10.1186/s12906-017-1799-8
- Arias-Chávez DJ, Mailloux-Salinas P, Altamirano J, et al. Consumption of combined fructose and sucrose diet exacerbates oxidative stress, hypertrophy and CaMKIIδ oxidation in hearts from rats with metabolic syndrome. Mol Cell Biochem. 2022;477(4):1309–1320. doi: 10.1007/s11010-022-04364-w
- Giacco F, Brownlee M, Schmidt AM. Oxidative stress and diabetic complications. Circ Res. 2010;107(9):1058–1070. doi: 10.1161/CIRCRESAHA.110.223545
- Weisberg SP, Hunter D, Huber R, et al. CCR2 modulates inflammatory and metabolic effects of high-fat feeding. J Clin Invest. 2006;116(1):115–124. doi: 10.1172/JCI24335
- Dalvi PS, Chalmers JA, Luo V, et al. High fat induces acute and chronic inflammation in the hypothalamus: effect of high-fat diet, palmitate and TNF-α on appetite-regulating NPY neurons. Int J Obesity. 2017;41(1):149–158. doi: 10.1038/ijo.2016.183 2005.
- Melillo de Magalhães P, Dupont I, Hendrickx A, et al. Anti-inflammatory effect and modulation of cytochrome P450 activities by Artemisia anuua tea infusions in human intestinal caco-2 cells. Food Chem. 2012;134(2):864–871. doi: 10.1016/j.foodchem.2012.02.195
- Vandanmagsar B, Haynie KR, Wicks SE, et al. Artemisia dracunculus L. extract ameliorates insulin sensitivity by attenuating inflammatory signalling in human skeletal muscle culture. Diabetes Obesity Metab. 2014;16(8):728–738. doi: 10.1111/dom.12274
- Aggarwal S, Shailendra G, Ribnicky DM, et al. An extract of Artemisia dracunculus L. Stimulates insulin secretion from β cells, activates AMPK and suppresses inflammation. J Ethnopharmacol. 2015;170:98–105. doi: 10.1016/j.jep.2015.05.003
- Li YJ, Guo Y, Yang Q, et al. Flavonoids casticin and chrysosplenol D from Artemisia anuua L. inhibit inflammation in vitro and in vitro. Toxicol Appl Pharmacol. 2015;286(3):151–158. doi: 10.1016/j.taap.2015.04.005
- Iqbal S, Younas U, Chan KW, et al. Chemical composition of Artemisia anuua L. leaves and antioxidant potential of extracts as a function of extraction solvents. Molecules. 2012;17(5):6020–6032. doi: 10.3390/molecules17056020
- Ho WE, Peh HY, Chan TK, et al. Artemisinins: Pharmacological actions beyond anti-malarial. Pharmacol Ther. 2014:142(1):126–139. doi: 10.1016/j.pharmthera.2013.12.001
- Juteau F, Masotti V, Bessiere JM, et al. Antibacterial and antioxidant activities of Artemisia anuua essential oil. Fitoterapia. 2002;73(6):532–535. doi: 10.1016/S0367-326X(02)00175-2
- Prabhu S, Vinodhini S, Elanchezhiyan C, et al. Retracted: evaluation of antidiabetic activity of biologically synthesized silver nanoparticles using Pouteria sapota in streptozotocin-induced diabetic rats. Diabetes. 2018;10(1):28–42. doi: 10.1111/1753-0407.12554