ABSTRACT
Bacterial lipopolysaccharide (LPS) is the typical inflammatory and oxidative stress agent; ginger (Zingiber officinale) has long been utilized to treat inflammatory conditions. This study aimed to evaluate the possible therapeutic role of ginger extract (GE) against LPS-induced hepato-renal and testicular injury. Male rats received GE (150 mg/kg, orally, for 28 days) after the inflammation shock was provoked by a single intraperitoneal injection of LPS (5 mg/kg). Rats treated with LPS exhibited marked inflammation and oxidative stress, in addition to liver and kidney injury and disruption of male sex hormones. The treatment of LPS-injected rats with GE resulted in a remarkable return to normalcy of the oxidative stress in the rats, as evidenced by a notable decrease MDA and an amelioration of SOD and CAT activities. On the other hand, the rats administered LPS and GE had considerably decreased serum levels of TNF-α and IL-6, improvement in serum albumin, total bilirubin and ALT and AST activity, urea, creatinine, and uric acid as well as FSH and testosterone levels. This indicating improved liver, kidney and testicular functions. In conclusion, GE attenuates the inflammatory damage and oxidative stress caused by LPS, which helps to restore organ function.
Introduction
A greater variety of studies have suggested that the bacterial endotoxin lipopolysaccharide (LPS) may have an impact on inflammatory mediator levels, playing a significant role in the development of a systemic inflammatory response that may result in sepsis and be associated with acute kidney and liver impairment. LPS has therefore been given to animals to create trustworthy experimental models that offer mechanistic insights into how cells and organs respond to inflammation; these models have then been extensively used to assess strategies to prevent or attenuate the systemic inflammatory response or multiple organ failure [Citation1–3]. In vivo and in vitro studies involving the administration of LPS, a major pathogenic component of the cell wall of Gram-negative bacteria, are a common experimental approach used to evaluate inflammatory responses [Citation4,Citation5]. Alterations in metabolism and catabolic signals are intimately linked to endotoxemia-induced responses in organs and tissues. During the inflammatory process, metabolites in the plasma, such as lipids, amino acids, and glucose, have been identified [Citation6,Citation7].
Pathogens may trigger an acute inflammatory response in response to LPS. Due to its ability to induce the production of inflammatory cytokines in a variety of cell types, such as interleukin (IL)-8, IL-6, and IL-1β, bacterial LPS has been widely employed to create an inflammatory model [Citation8]. The LPS receptor on the cell surface is called toll-like receptor 4 (TLR4). Lymphocyte antigen 96 (MD-2), glycosylphosphatidylinositol (GPI)-anchored monocyte differentiation antigen CD14 (CD14), and lipopolysaccharide-binding protein (LBP) are involved in the regulation of TLR4 activation. LBP attaches itself to the lipid A component of LPS and transfers it to CD14, ensuring and enhancing signaling via the TLR4/MD-2 complex [Citation9]. TLR4 activation initiates two signaling pathways in total. The first one involves recruiting and activating Myeloid Differentiation Primary Response Protein MyD88 (MyD88) and Toll/interleukin-1 receptor domain-containing adapter protein (TIRAP), which in turn activates NF-κB and mitogen-activated protein kinases (MAPKs). TLR domain-containing adaptor molecule 1 (TRIF) and TIR domain-containing adapter molecule 2 (TRAM) influence the other pathway. TLR4 internalization is necessary for IκB kinase and interferon (IFN) regulatory factor 3 (IRF3) activation, which induce type 1 IFN genes [Citation10]. The release of pro- and anti-inflammatory substances and inflammatory cytokines is ultimately regulated by these cascaded transcriptional processes, which also strongly express thousands of genes. Thus, the TLR4/NF-κB/MAPKs pathways are thought to be among the main signaling routes that contribute to the activation of inflammatory response factor 3 (IRF3) and the subsequent expression of type 1 IFN genes [Citation11].
LPS was reported to induce inflammation in the brain and the blood-testis barrier (BTB) as well [Citation12,Citation13]. A variety of microorganisms, including bacteria, viruses, and parasites, have the ability to infect the male reproductive system and cause a variety of inflammatory reactions that reduce male fertility [Citation14]. Bacteria usually infect the urethra, seminal vesicles, prostate, epididymis, vas deferens, and testes retrograde through the reproductive tract. Additionally, infection caused by bacteria was found to contribute to 15% of male infertility cases [Citation15–17]. Following the pathogenic bacteria’s invasion of the male reproductive system, gonad cells may trigger autophagy and oxidative stress. While high oxidative stress can cause testicular damage or even result in infertility, moderate oxidative stress aids in the pathogen’s elimination [Citation18]. Cells and tissue are toxically affected by abnormally high oxidative stress, which can harm nearly every component of a cell, including lipids, proteins, and DNA.
Enhancing the qualities of natural plants to create new antioxidant types through economical and environmentally sustainable methods has gained more traction recently [Citation19]. Zingiber officianale, the ginger plant, is a member of the Zingiberaceae family of perennial herbs. More than a hundred different compounds have been identified in ginger, including phenolic and flavonoid components that have applications in food and medicine [Citation20]. Therefore, ginger is one of the most used plants in traditional medicine as it has non-significant side effects without drug or herb interactions. Ginger can prevent oxidative damage by integrating the lipid bilayer and scavenging peroxyl radicals [Citation21]. Regarding this, in vitro [Citation22] and in vivo studies have verified ginger’s antioxidant qualities [Citation20]. Additionally, ginger contains anti-inflammatory qualities [Citation23] and can protect against hepatotoxicity [Citation24], nephrotoxicity [Citation25], and apoptosis [Citation20] induced by pesticides. Ginger has been used to treat primary dysmenorrhea, vomiting, fever, and stomachaches [Citation26,Citation27] and has anti-inflammatory, anti-microbial, and anti-oxidative properties [Citation28]. Strong anti-inflammatory properties and the ability to stop the release of cytokines that induce inflammation are included in the Zingiber officinale extract [Citation29]. Accordingly, this work is mainly designed to evaluate the potential therapeutic role of ginger extract against bacterial LPS-induced hepato-renal and testicular toxicity.
Material and methods
Lipopolysaccharides (LPS)
LPS from Escherichia coli (Sero-type, 055:B5) was purchased from Sigma-Aldrich, St. Louis, USA).
Ginger extract (GE)
One kilogram of fresh ginger rhizomes was obtained from Damanhour local market. The botanists from Damanhour University’s Faculty of Science’s Botany Department identified the rhizomes. The ginger rhizomes were crushed in a blender and then extracted with 95% ethanol. A dose of 150 mg/kg of ginger extract (GE) was prepared according to Bhandari et al. [Citation30].
Experimental design
Forty adult male Wister albino rats (160–180 g) were used in this study. The animals were housed in typical lighting conditions in a room with wire-bottom cages, with a 12-hour light-dark cycle, 25 ± 1°C temperature, and 50% relative humidity. They had unlimited access to tap water and a balanced diet of libitum. After acclimatization for one week, the rats were randomly divided into four groups, each consisting of ten rats (n = 10). Group 1 (control) rats were fed a normal diet plus normal saline for four weeks. Group 2: rats treated orally with ethanolic GE (150 mg/kg b/wt) daily for 4 weeks. Group 3 (LPS-treated) rats were injected with a single dose of LPS (5 mg/kg, i.p., dissolved in normal saline) and left for four weeks. Group 4 (LPS+GE) rats were injected with LPS and left for 4 weeks, followed by treatment with GE (150 mg/kg) daily for 4 weeks. The lethal dose (LD50) of LPS in rats was previously reported to exceed 20 mg/kg in normal rats [Citation31]. For rats, a single intraperitoneal injection of 5 mg/kg of LPS is the recommended dosage. Dose selection was prepared according to the previous researches [Citation32–34]. At the end of the experiment (four weeks for groups 1, 2, and 3 and eight weeks for group 4), the blood samples were collected from the tail vein, then anaesthetized by IP injection of ketamine (75 mg/kg) and xylazine (10 mg/kg) and dissected to obtain the liver, kidneys, and testes. The blood was centrifuged at 1922 g for 15 min at 4°C. The serum was isolated and stored at 20°C until biochemical analysis. The liver, kidneys, and testes were immediately cleaned and washed in an ice-cold saline solution, then processed for histological investigation. All animal experiments were approved by the Experimental Animal Ethics Committee of the Faculty of Science, Damanhour University (DMU-SCI-CSRE-23-12-01).
Investigated parameters
Measurement of tumor necrosis factor-α (TNF-α) and interleukin-6 (IL-6)
The serum levels of TNF-α and IL-6 were estimated with rat-specific ELISA kits: TNF-α (cat. no. ELS-2855-1) and IL-6 (cat. no. ELS-2866-1) (Hefei Bomei Biotechnology Co., Ltd., Hefei, China). The levels of TNF-α and IL-6 were expressed as pg/mL.
Determination of serum SOD, CAT, and MDA levels
The level of superoxide dismutase (SOD) was measured by a detection kit (Ransod and Ransel kits, respectively; RANDOX Company, UK) [Citation35]. Catalase (CAT) was determined according to methods described by Sinha [Citation36]. The level of malondialdehyde (MDA) was evaluated by a modified method as described previously [Citation37].
Estimation of serum levels of ALT, AST, total bilirubin, and albumin
An autoanalyzer (Beckman Coulter AU680, California, USA) was used to estimate the activity of serum alanine aminotransferase (ALT) [Citation38] and aspartate aminotransferase (AST) [Citation39]. The levels of total bilirubin (TB) and albumin (ALB) were measured spectrophotometericaly as previously described [Citation38,Citation40].
Measurement of serum creatinine, urea nitrogen and uric acid
Serum creatinine and blood urea nitrogen were detected using automatic cell analyzers (ARCHITECT c 8000, Abbott Corporation, Chicago, USA). The level of serum uric acid (UA) was carried out using the uric acid uricase/peroxidase kit: [BioSystems S.A., Barcelona, Spain] [Citation41].
Measurement of serum FSH,LH and testosterone
The levels of serum FSH (cat. No. ZB101825R9648) and LH (cat. no: ZB10179SR9648) were measured using the commercial ELISA kits (Zellbio GmbH, Ulm, Germany) based on the absorbance at 450 nm according to the manufacturer’s instructions. Serum levels of testosterone were quantified by relevant ELISA (BioVendor Research and Diagnostic Products, Czechia).
Preparation of histological sections
Segments of liver, kidneys, and testes were preserved in 10% neutral buffered formalin for 48 hours, then dehydrated in ethanol, embedded in paraffin, and sectioned into 5 µm. Sections were de-waxed, rehydrated, dried overnight, and stained with hematoxylin and eosin (H&E) for histological examination under a light microscope (Leica DM500).
Statistical analysis
Data was fed to the computer and analyzed using IBM SPSS software package version 20.0. (Armonk, NY: IBM Corp). For continuous data, they were tested for normality by the Shapiro-Wilk test. Quantitative data were expressed as mean and standard deviation. One way ANOVA test was used for comparing the four studied groups and followed by Post Hoc test (Tukey) for pairwise comparison. The significance of the obtained results was judged at the 5% level.
Results
Changes in the levels of IL-6 and TNF-α
Serum IL-6 and TNF-α levels in male rats given LPS treatment were significantly higher (p < 0.001) than those in the control group. The levels of these two indicators considerably decreased but remained significantly higher than control in rats given LPS and received GE supplementation ().
Changes in serum SOD, CAT, and MDA
Treatment of male rats with a single dose of LPS resulted in a significant reduction (p < 0.001) in the activities of serum SOD and CAT, while a significant increase in the level of MDA was noticed compared with the control rats. Supplementation of GE to LPS-treated rats showed a significant increase in the activities of SOD and CAT, while the level of MDA was markedly decreased compared with the LPS group. The activity of SOD and MDA is still significantly lower than that of control ().
Changes in liver functions
When male rats were given a single dose of LPS, their blood ALT and AST activities, as well as their bilirubin content, significantly increased (p < 0.001) in comparison to the control group of rats. On the other hand, the concentration of ALB was markedly decreased in the same rats. When GE was injected into LPS-treated rats, these liver function indicators significantly improved as compared to the LPS group ().
Changes in renal functions
The measured renal functions appeared elevated in LPS-treated rats, where it was a significant increase (p < 0.001) in the levels of serum creatinine, urea nitrogen, and uric acid compared with the control. Post-supplementation of GE to LPS-exposed rats successfully decreased the levels of creatinine and urea if compared with LPS alone, but still significantly higher than control, while the level of uric acid showed no significant change (P > 0.001) with control ().
Changes in serum levels of FSH, LH and testosterone
Serum levels of testosterone, LH, and FSH were considerably lower in the LPS-treated group than the control group (p < 0.001). Nevertheless, following GE treatment, these hormone levels were significantly higher (p < 0.001) than in rats treated with LPS, although they remained lower than in the control group ().
Histological investigations
The liver
The liver sections from control and GE-treated rats displayed normal histological architecture, represented by a well-organized central vein, hepatic strands, and portal area (). In LPS-treated rats, the liver sections showed pronounced histopathological signs. These included a dilated central vein with damaged endothelium, dilated blood sinusoids, pyknotic hepatocytes, and infiltrated cells. Additionally, the hepatic portal vein appeared dilated with obvious thickening (). Treatment of LPS-intoxicated rats with GE markedly alleviated the histopathological signs caused by LPS ().
Figure 6. Images of histological sections of liver among the different studied groups of rats (images a&b: control, images c&d: GE, images e&f: LPS, and images g&f LPS+GE). The liver sections in images e&f show a dilated central vein with a degenerated endothelium (white arrowhead), infiltrated cells (green arrowhead), scattered pyknotic hepatocytes (PH), dilated blood sinusoids (yellow stars), a thickened wall of the hepatic portal vein (red arrowheads), and a profibrotic portal area (yellow arrowheads). (stain: Hx&E, scale bar: 100).
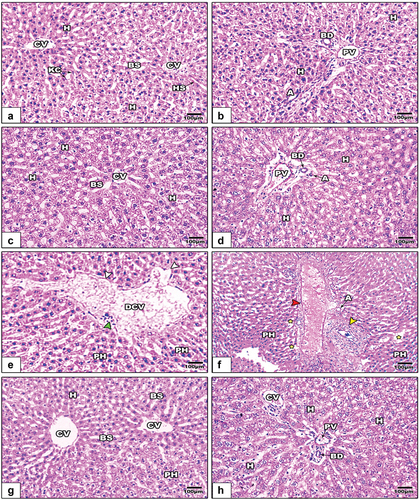
The kidney
The renal cortical sections of control and GE-treated rats showed normal renal corpuscles and tubules (). In LPS-treated rats, the renal corpuscles appeared atretic with congested glomeruli and wide Bowman’s space, the cortical tubules appeared dilated with degenerated epithelium, and the interstitial capillaries appeared congested (). On the other hand, GE attenuated the renal histopathological signs induced by LPS ().
Figure 7. Images of histological sections of renal cortex among the different studied groups of rats (image a: control, image b: GE, images c-e: LPS, and image f: LPS+GE). In images c-e, the glomeruli appear atretic and congested with obvious dilated Bowman’s space (red arrowhead), the proximal and distal tubules appear dilated (yellow stars) with obvious degenerated epithelium (green arrow heads), the walls of collecting tubules appear fragmented (red stars) and dilated (green stars), and dilated capillary (blue arrowhead), thickened blood capillary (white arrowhead). (stain: Hx&E, scale bar: 25).
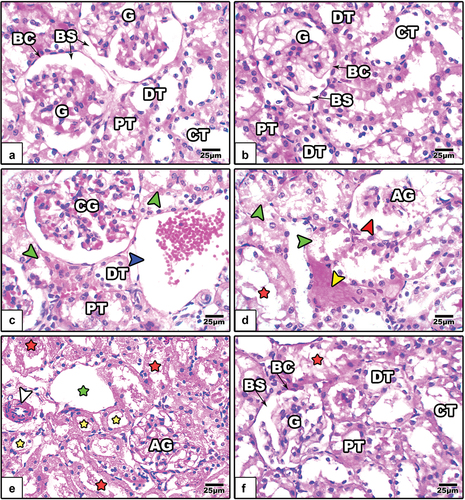
The testes
Severe histomorphological signs appeared in the testicular sections of LPS-treated rats; These included congested testicular capillary, atrophied seminiferous tubules, scarce sperms in some tubules, degenerated spermatogenic stages, fragmented basal lamina, and wide intertubular spaces (). After treatment with GE, the testicular histopathological Changes apparently disappeared ().
Figure 8. Histological images from the testicular sections among the different studied groups of rats (images a&b: control, images c&d: GE, images e&f: LPS, and images g&f LPS+GE). In images e&f, congested testicular capillary (green arrowhead), atrophied seminiferous tubules (AST), sperm lost (blue stars), degenerated spermatogenic stages (yellow stars), fragmented basal lamina (white arrow heads), and wide inter-tubular spaces (red stars). (stain: Hx&E, scale bar: 25).
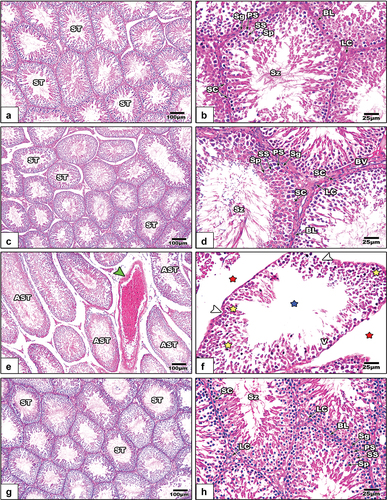
Discussion
It is commonly known that inflammatory cytokines and macrophage activation play significant roles in various organs damage [Citation42–44]. It had been reported that bacterial infection can induce tissue damage through the induction of oxidative stress and the release of pro-inflammatory cytokines [Citation45]. Accordingly, this study aimed to evaluate the potential therapeutic role of ginger extract against bacterial LPS-induced dysfunction in the liver, kidneys, and testes of male rats.
In the current work, administration of a single dose of bacterial LPS (5 mg/kg) resulted in a significant elevation of serum pro-inflammatory cytokines markers (IL-6 and TNF-α), disrupted oxidative stress parameters (MDA, SOD, and CAT activity), kidney function indexes (urea, creatinine, and uric acid), and liver function indicators (ALT, AST, total bilirubin, and albumin). Also, there were remarkable changes in levels of sexual hormones (FSH, LH, and testosterone). Additionally, severe histopathological signs were recorded in the examined liver, kidney, and testes after the injection of LPS. On the other hand, treatment with ginger extract successfully alleviated the pathophysiological changes induced by LPS.
Previous reports revealed significant elevations in the levels of serum IL-6 and TNF-α after treatment with bacterial LPS [Citation46,Citation47]. McDonald et al. explained that LPS can cause liver inflammation by attracting neutrophils [Citation48], which then triggers the development of inflammatory proteins like TNF α, and liberation of excess ROS. LPS can trigger the overproduction of ROS and the excessive release of pro-inflammatory cytokines by binding with Toll-like receptor 4 on the surface of Küpffer cells [Citation49], promoting necrosis and hepatic cell apoptosis [Citation50]. Oxidative stress results from overproduction of ROS and/or depletion of antioxidant enzymes [Citation51] and excess production of lipid peroxidation [Citation52], can induce cell damage through mitochondrial dysfunction, and further harm different tissues [Citation53]. Furthermore, oxidative stress is linked to a higher level of MDA, as a consequence of polyunsaturated fatty acid peroxidation [Citation54]. SOD converts hydroxyl radicals and ROS into hydrogen peroxide, which can be degraded by CAT [Citation55]. In this work, oxidative stress damage was indicated by a significant decrease in the levels of serum SOD and CAT and a significant rise in MDA levels in the LPS-treated rats. Previous studies have reported that the oxidative stress caused by LPS administration can induce hepatic cell damage through an elevation of liver enzymes and total bilirubin and a remarkable decrease of albumin [Citation56–58].
Badr et al. proposed that, in comparison to tumor-induced mice, ginger extract administration results in a considerable rise in serum SOD and CAT activity [Citation59]. Active phenolic chemicals found in ginger, such as paradol, shogaol, and gingerol, have anti-oxidant properties [Citation60]. Ginger rhizome extracts have been found to contain over fifty different types of antioxidants [Citation61]. Strong antioxidant activity was proven by the nonvolatile portion of the ginger root extract. According to Lebda et al. [Citation62], these phenolic antioxidants could improve the amounts of SH-containing compounds like glutathione while also preserving antioxidant enzymes. This is in accordance with our obtained results, where GE managed considerable improvement of the serum CAT and SOD in rats that had been exposed to lipopolysaccharide (LPS).
In this study, LPS induced pathological damage and increased the ALT and AST activities and total bilirubin levels, with a dramatic decrease in the serum levels of albumin. Lin and Huang reported that ALT and AST enzymes are found in the cytoplasm of liver cells and are liberated into the blood when the cell membrane is disrupted [Citation63]. Also, it was confirmed that the presence of excess extracellular enzymes is considered a sign for hepatic cell damage [Citation64]. This is consistent with the results of the liver histopathological signs found in this study. Other reports revealed that the elevated activities of these enzymes are signals of the functional integrity of the cell membranes in the liver which are always linked with hepatonecrosis [Citation65,Citation66] and inflammatory reactions [Citation67]. The significant increase in bilirubin levels in LPS-intoxicated rats is in line with the findings of Ozmem and Ipek who reported a significant increase in the level of bilirubin in rats treated with a single dose of bacterial LPS [Citation68]. On the other hand, ginger therapy effectively decreased high blood ALT and AST activity in rats intoxicated with LPS. These results are consistent with those of Badr et al., who found that after GE treatment, sera ALT and AST activities are significantly less than in tumor-induced animals [Citation59]. Ginger has been shown to reduce the elevated levels of AST, ALT, and ALP in the serum [Citation69]. Ginger treatment before acetaminophen showed a notable hepatoprotective effect due to a reduction in the concentration of hepatic marker enzymes and total bilirubin in plasma [Citation70]. Other reports revealed that ginger may provide protection by stabilizing the cell membrane in LPS-induced liver injury [Citation71,Citation72].
The improvement of ginger extract to deleterious histological architecture of the liver induced by LPS may be attributed to the antioxidant compounds of ginger, like zingerone, gingerdiol, zingiberene, gingerols, shogaols, and tannins [Citation73]. These compounds may enhance hepatocyte structure and function, including proliferation, by acting as free radical scavengers, preventing lipid peroxidation, and damaging DNA [Citation64,Citation74]. The active component of ginger, 6-gingerol, has been shown to have strong antioxidant properties, and these properties have been associated with the activation of antioxidation defenses. Likewise, by lowering hepatic marker enzymes, this activity is linked to a reduction in liver damage [Citation75].
It has been reported that, exposure to LPS results in excessive production of pro-inflammatory cytokines like TNF-α, IL-1β, and IL-6, which are causative factors for acute kidney injury [Citation76,Citation77]. Kang et al. revealed that, the excess liberation of ROS and the significant depletion of antioxidant enzymes are implicated in the progression of renal dysfunction [Citation78]. In the current work, LPS markedly elevated the levels of urea, creatinine, and uric acid, the main indicators of renal damage. The obtained results agree with previous reports [Citation79,Citation80]. Histopathological examination of the kidneys of LPS injected rats showed atretic and congested glomeruli, relatively dilated Bowman’s space, dilated proximal and distal tubules with obvious degenerated epithelium, and thickened and dilated capillaries. Similar histopathological signs were recorded in LPS treated mice [Citation81]. Patil et al. demonstrated that high levels of inflammatory cytokines could increase renal vasoconstriction, which in turn decreases renal blood flow, lowering glomerular filtration rate and resulting in glomerular and tubular damage [Citation82]. TNF-α and other related pro-inflammatory factors, such as IL-1β and IL-6, promote the production of neutrophils, macrophages, and dendritic cells. Activation of immune cells increases reactive oxidative species (ROS), neutrophils, leading to tissue damage [Citation83,Citation84].
The treatment of rats with 150 mg/kg of GE for four weeks following LPS exposure in this study caused a significant improvement in renal functions, as indicated by a decrease in urea, creatinine, and uric acid levels. This reflects the potential crucial anti-oxidative and chelating effects of GE on LPS-induced nephrotoxicity. The phenolic and flavonoid components in GE have been associated with nephroprotective and antioxidant properties [Citation85,Citation86]. According to Gabr et al., ginger has high concentrations of flavonoids (9.33 mg) and polyphenolic (26.3 mg) components [Citation87], which have strong antioxidant and anti-free radical scavenging properties. Zingerone, shogaols, and gingerols, among many other active ingredients, were found in ginger, according to many phytochemical reports [Citation88,Citation89]. In addition to volatile oils like camphene, beta-phellandrene, and curcumin, it was also discovered that gingerol (polyphenol), was the main active ingredient in fresh ginger rhizomes [Citation86]. Because of these compounds, ginger is supported as a natural antioxidant that is essential for the chemoprevention of diseases brought on by oxidative stress from free radicals. Our results were consistent with those of other researchers who noticed a significant decrease in blood urea in experimental animals after a 20-day ginger extract therapy [Citation61]B. have reported the importance of ginger as a medicinal herbal medicine for managing renal function in patients with uremia [Citation90].
Shen et al. revealed that bacterial LPS can affect testicular function through systemic inflammation, which disrupts the hypothalamus-pituitary-gonad axis and impairs seminiferous tubules [Citation91]. A recent study declared that LPS can disrupt the blood testes-barrier (BTB) [Citation92] as weakened BTB adversely affects the architecture of seminiferous tubules and different stages of spermatogenic cells [Citation93]. Another study showed that LPS can induce pronounced inflammation inside the testis through elevation of IL6 and TNF-α level [Citation94]. Numerous studies have confirmed that Leydig cells and Sertoli cells liberate inflammatory cytokines in response to LPS and other inflammatory markers, resulting in remarkable inhibition of steroidogenesis and spermatogenesis [Citation95,Citation96]. Allen et al. explained that LPS can inhibit the synthesis of testosterone from Leydig cells by reducing the synthesis of steroidogenic enzymes [Citation97]. Other reports assure that LPS induced overproduction of free radicals can induce oxidative stress on the testicular cells, resulting in impairment in spermatogenesis [Citation98,Citation99]. In the current work, LPS was found to induce testicular dysfunction through impairment of serum FSH, LH, and testosterone, in addition to induction of histopathological changes in the testis.
Banihani reported that ginger can improve gonadal testosterone levels, which in turn enhances the primary sperm parameters [Citation100]. Gingerols, zingiberene, and shogaol compounds of ginger were found to enhance testicular function via the neutralization of oxidative stress [Citation101]. In the current work, supplementation of ginger extract to LPS-injected rats for 28 consecutive days markedly restored the altered levels of FSH, LH, and testosterone to near-normal levels along with a pronounced improvement in the histological architecture of seminiferous tubules. The current results agree with the previous reports [Citation102–104] which revealed that ginger can improve the testicular and pituitary-gonadal axis disruptions induced by ethanol. It has been reported that ginger or its derivatives can also enhance testosterone synthesis by various mechanisms [Citation105,Citation106]. Furthermore, in cryptorchid rats, ginger was observed to improve the characteristics of epididymal sperm and prevent oxidative damage in the testis [Citation106]. Additionally, ginger extract has been demonstrated by Mohammedi et al. to improve the levels of testosterone as well as the quantity of Sertoli and spermatogonial cells in seminiferous tubules [Citation103]. Khaki et al. found that ginger (50 and 100 mg/kg/day) can improve sperm viability, motility, and serum total testosterones [Citation107]. Gholami et al. added that ginger can increase the testicular blood flow by dilating blood vessels [Citation108].
Conclusion
Ginger rhizome extract ameliorated organs damage via modulation of inflammation and oxidative stress caused by Gram-negative bacteria toxins, LPS. The current research points to a drug able utility of the GE and suggest the basis for the use of this natural product to other Gram-negative pathogens. However, further studies are needed to clarify the mechanism of action of ginger compounds against bacterial toxins.
Author contributions
LA, GM, and AFB designed the work protocol. KB and HM follow up the practical technique and provide comments. AFB and MA give the statistical analysis of results. All authors contribute to the writing and revision of the paper.
Ethics approval and consent to participate
All procedures were performed in accordance with the guidelines of the bioethics committee of faculty of Science, Damanhour University (DMU-SCI-CSRE-23-12-01).
Acknowledgments
The authors are grateful to the team of biochemical lab, faculty of science Damanhour University for their efforts to facilitate the completion of practical protocol.
Disclosure statement
No potential conflict of interest was reported by the author(s).
Additional information
Funding
References
- Calvano SE, Coyle SM. Experimental human endotoxemia: a model of the systemic inflammatory response syndrome? Surg Infect (Larchmt). 2012;13(5):293–9. doi: 10.1089/sur.2012.155
- Deng M, Scott MJ, Loughran P, et al. Lipopolysaccharide clearance, bacterial clearance, and systemic inflammatory responses are regulated by cell type-specific functions of TLR4 during sepsis. J Immunol. 2013;190(10):5152–5160. doi: 10.4049/jimmunol.1300496
- Page MJ, Kell DB, Pretorius E. The role of lipopolysaccharide-induced cell signalling in chronic inflammation. chronic stress (Thousand Oaks). 2022;6(24705470221076390):247054702210763. doi: 10.1177/24705470221076390
- Hedger M, Klug J, Frohlich S, et al. Regulatory cytokine expression and interstitial fluid formation in the normal and inflamed rat testis are under leydig cell control. J Androl. 2005;26(3):379–86. doi: 10.2164/jandrol.04149
- Winnall WR, Muir JA, Hedger MP. Differential responses of epithelial Sertoli cells of the rat testis to Toll-like receptor 2 and 4 ligands: implications for studies of testicular inflammation using bacterial lipopolysaccharides. Innate Immun. 2011;17(2):123–136. doi: 10.1177/1753425909354764
- Khovidhunkit W, Kim MS, Memon RA, et al. Effects of infection and inflammation on lipid and lipoprotein metabolism: mechanisms and consequences to the host. J Lipid Res. 2004;45(7):1169–1196. doi: 10.1194/jlr.R300019-JLR200
- Wang M, Feng J, Zhou D, et al. Bacterial lipopolysaccharide-induced endothelial activation and dysfunction: a new predictive and therapeutic paradigm for sepsis. Eur J Med Res. 2023;28(1):339. doi: 10.1186/s40001-023-01301-5
- Zhou S, Chen G, Qi M, et al. Gram negative bacterial inflammation ameliorated by the plasma protein beta 2-glycoprotein I. Sci Rep. 2016;6(1):33656. doi: 10.1038/srep33656
- Garay-Malpartida HM, Mourao RF, Mantovani M, et al. Toll-like receptor 4 (TLR4) expression in human and murine pancreatic beta-cells affects cell viability and insulin homeostasis. BMC Immunol. 2011;12(1):18. doi: 10.1186/1471-2172-12-18
- Kim JJ, Sears DD. TLR4 and Insulin Resistance. Gastroenterol Res Pract. Gastroenterol Res Pract. 2010;2010(212563):1–11. doi: 10.1155/2010/212563
- Wang G, Hu Z, Fu Q, et al. Resveratrol mitigates lipopolysaccharide-mediated acute inflammation in rats by inhibiting the TLR4/NF-κBp65/MAPKs signaling cascade. Sci Rep. 2017;7(1). doi: 10.1038/srep45006
- Singh AK, Jiang Y. How does peripheral lipopolysaccharide induce gene expression in the brain of rats? Toxicology. 2004;201(1–3):197–207. doi: 10.1016/j.tox.2004.04.015
- Jangula A, Murphy EJ. Lipopolysaccharide-induced blood brain barrier permeability is enhanced by alpha-synuclein expression. Neurosci Lett. 2013;551:23–27. doi: 10.1016/j.neulet.2013.06.058
- Bukharin OV, Kuz’min MD, Ivanov Iu B. [The role of the microbial factor in the pathogenesis of male infertility]. Zh Mikrobiol Epidemiol Immunobiol. 2000;2:106–110. https://www.ncbi.nlm.nih.gov/pubmed/10808590
- La Vignera S, Vicari E, Condorelli RA, et al. Male accessory gland infection and sperm parameters (review). Int J Androl. 2011;34(5 Pt 2):e330–47. doi: 10.1111/j.1365-2605.2011.01200.x
- Cai T, Wagenlehner FM, Mondaini N, et al. Effect of human papillomavirus and chlamydia trachomatis co-infection on sperm quality in young heterosexual men with chronic prostatitis-related symptoms. BJU Int. 2014;113(2):281–287. doi: 10.1111/bju.12244
- Erdem H, Elaldi N, Ak O, et al. Genitourinary brucellosis: results of a multicentric study. Clin Microbiol Infect. 2014;20(11):O847–53. doi: 10.1111/1469-0691.12680
- Marchlewicz M, Szypulska-Koziarska D, Grzegrzółka A, et al. Ochrona przed stresem oksydacyjnym w męskim układzie płciowym. Pomeranian Journal Of Life Sci. 2017;62(1). doi: 10.21164/pomjlifesci.169
- Magdy AM, Fahmy EM, Al-Ansary A, et al. Improvement of 6-gingerol production in ginger rhizomes (zingiber officinale Roscoe) plants by mutation breeding using gamma irradiation. Appl Radiat Isot. 2020;162:109193. doi: 10.1016/j.apradiso.2020.109193
- Beklar SY, Hamzeh M, Karimpour A, et al. zingiber officinale mitigates diazinon-induced testicular toxicity via suppression of oxidative stress and apoptosis in mice model. J Res Pharm. 2019;23(2):224–234. doi: 10.12991/jrp.2019.128
- Stoilova I, Krastanov A, Stoyanova A, et al. Antioxidant activity of a ginger extract (zingiber officinale). Food Chem. 2007;102(3):764–70. doi: 10.1016/j.foodchem.2006.06.023
- Gan Z, Liang Z, Chen X, et al. Separation and preparation of 6-gingerol from molecular distillation residue of Yunnan ginger rhizomes by high-speed counter-current chromatography and the antioxidant activity of ginger oils in vitro. J Chromatogr B. 2016;1011:99–107. doi: 10.1016/j.jchromb.2015.12.051
- Jeena K, Liju VB, Kuttan R. Antioxidant, anti-inflammatory and antinociceptive activities of essential oil from ginger. Indian J Physiol Pharmacol. 2013;57(1):51–62. https://www.ncbi.nlm.nih.gov/pubmed/24020099
- Haleagrahara N, Jackie T, Chakravarthi S, et al. Protective effect of Etlingera elatior (torch ginger) extract on lead acetate–induced hepatotoxicity in rats. J Toxicol Sci. 2010;35(5):663–671. doi: 10.2131/jts.35.663
- Ali DA, Abdeen AM, Ismail MF, et al. Histological, ultrastructural and immunohistochemical studies on the protective effect of ginger extract against cisplatin-induced nephrotoxicity in male rats. Toxicol Ind Health. 2015;31(10):869–80. doi: 10.1177/0748233713483198
- Terry R, Posadzki P, Watson LK, et al. The use of ginger (zingiber officinale) for the treatment of pain: a systematic review of clinical trials. Pain Med. 2011;12(12):1808–18. doi: 10.1111/j.1526-4637.2011.01261.x
- Chen CX, Barrett B, Kwekkeboom KL. Efficacy of oral ginger (zingiber officinale) for dysmenorrhea: a systematic review and meta-analysis. Evid Based Complement Alternat Med. 2016;2016(6295737):1–10. doi: 10.1155/2016/6295737
- Mashhadi NS, Ghiasvand R, Askari G, et al. Anti-oxidative and anti-inflammatory effects of ginger in health and physical activity: review of current evidence. Int J Prev Med. 2013;4(Suppl 1):S36–42. https://www.ncbi.nlm.nih.gov/pubmed/23717767
- Song MY, Lee DY, Park SY, et al. Steamed ginger extract exerts anti-inflammatory effects in Helicobacter pylori-infected Gastric Epithelial Cells through inhibition of NF-kappaB. J Cancer Prev. 2021;26(4):289–297. doi: 10.15430/JCP.2021.26.4.289
- Bhandari U, Kanojia R, Pillai KK. Effect of ethanolic extract of zingiber officinale on dyslipidaemia in diabetic rats. J Ethnopharmacol. 2005;97(2):227–30. doi: 10.1016/j.jep.2004.11.011
- Harry D, Anand R, Holt S, et al. Increased sensitivity to endotoxemia in the bile duct-ligated cirrhotic rat. Hepatology. 1999;30(5):1198–1205. doi: 10.1002/hep.510300515
- Li G, Liu Y, Tzeng NS, et al. Protective effect of dextromethorphan against endotoxic shock in mice. Biochem Pharmacol. 2005;69(2):233–40. doi: 10.1016/j.bcp.2004.10.003
- Qin L, Wu X, Block ML, et al. Systemic LPS causes chronic neuroinflammation and progressive neurodegeneration. Glia. 2007;55(5):453–62. doi: 10.1002/glia.20467
- Bossu P, Cutuli D, Palladino I, et al. A single intraperitoneal injection of endotoxin in rats induces long-lasting modifications in behavior and brain protein levels of TNF-α and IL-18. J Neuroinflammation. 2012;9(1). doi: 10.1186/1742-2094-9-101
- Misra HP, Fridovich I. The role of superoxide anion in the autoxidation of epinephrine and a simple assay for superoxide dismutase. J Biol Chem. 1972;247(10):3170–5. doi: 10.1016/S0021-9258(19)45228-9
- Sinha AK. Colorimetric assay of catalase. Anal Biochem. 1972;47(2):389–94. doi: 10.1016/0003-2697(72)90132-7
- Dousset JC, Trouilh M, Foglietti MJ. Plasma malonaldehyde levels during myocardial infarction. Clin Chim Acta. 1983;129(3):319–22. doi: 10.1016/0009-8981(83)90035-9
- Alanine and aspartate aminotransferase, principle and usage. In: Steven CK, James JT, Jenifer R editors. Liver function, in clinical chemistry theory, analysis and correlation, 3rd, pp. 504–527. Mosby: London. 1996.
- Reitman S, Frankel S. A colorimetric method for the determination of serum glutamic oxalacetic and glutamic pyruvic transaminases. Am J Clin Pathol. 1957;28(1):56–63. doi: 10.1093/ajcp/28.1.56
- Gustafsson JE. Improved specificity of serum albumin determination and estimation of “acute phase reactants” by use of the bromcresol green reaction. Clin Chem. 1976;22(5):616–622. doi: 10.1093/clinchem/22.5.616
- Barham D, Trinder P. An improved colour reagent for the determination of blood glucose by the oxidase system. Analyst. 1972;97(151):142–145. doi: 10.1039/an9729700142
- Rossignol DP, Lynn M. TLR4 antagonists for endotoxemia and beyond. Curr Opin Invest Drugs. 2005;6(5):496–502. https://www.ncbi.nlm.nih.gov/pubmed/15912963
- Biswas B, Yenugu S. Lipopolysaccharide induces epididymal and testicular antimicrobial gene expression in vitro: insights into the epigenetic regulation of sperm-associated antigen 11e gene. Immunogenetics. 2013;65(4):239–53. doi: 10.1007/s00251-012-0674-5
- Anderson ST, Commins S, Moynagh PN, et al. Lipopolysaccharide-induced sepsis induces long-lasting affective changes in the mouse. Brain Behav Immun. 2015;43:98–109. doi: 10.1016/j.bbi.2014.07.007
- Lowes DA, Webster NR, Murphy MP, et al. Antioxidants that protect mitochondria reduce interleukin-6 and oxidative stress, improve mitochondrial function, and reduce biochemical markers of organ dysfunction in a rat model of acute sepsis. Br J Anaesth. 2013;110(3):472–80. doi: 10.1093/bja/aes577
- Leisegang K, Henkel R, Agarwal A. Obesity and metabolic syndrome associated with systemic inflammation and the impact on the male reproductive system. Am J Reprod Immunol. 2019;82(5):e13178. doi: 10.1111/aji.13178
- Bhattacharya K, Sengupta P, Dutta S, et al. Obesity, systemic inflammation and male infertility. 2020;7(2):7. https://pubs.iscience.in/journal/index.php/cbl/article/view/1002
- McDonald B, Jenne CN, Zhuo L, et al. Kupffer cells and activation of endothelial TLR4 coordinate neutrophil adhesion within liver sinusoids during endotoxemia. Am J Physiol Gastrointest Liver Physiol. 2013;305(11):G797–806. doi: 10.1152/ajpgi.00058.2013
- Ohsaki Y, Shirakawa H, Hiwatashi K, et al. Vitamin K suppresses lipopolysaccharide-induced inflammation in the rat. Biosci Biotechnol Biochem. 2006;70(4):926–32. doi: 10.1271/bbb.70.926
- Wang Y, Gao LN, Cui YL, et al. Protective effect of danhong injection on acute hepatic failure induced by lipopolysaccharide and d-galactosamine in mice. Evid Based Complement Alternat Med. 2014;2014(153902). doi: 10.1155/2014/153902
- Davies KJ. Protein damage and degradation by oxygen radicals. I. general aspects. J Biol Chem. 1987;262(20):9895–901. doi: 10.1016/s0021-9258(18)48018-0
- Klein T, Neuhaus K, Reutter F, et al. Generation of 8-epi-prostaglandin F(2alpha) in isolated rat kidney glomeruli by a radical-independent mechanism. Br J Pharmacol. 2001;133(5):643–650. doi: 10.1038/sj.bjp.0704111
- Cadenas S, Cadenas AM. Fighting the stranger-antioxidant protection against endotoxin toxicity. Toxicology. 2002;180(1):45–63. doi: 10.1016/s0300-483x(02)00381-5
- Aziz IA, Yacoub M, Rashid L, et al. Malondialdehyde; lipid peroxidation plasma biomarker correlated with hepatic fibrosis in human schistosoma mansoni infection. Acta Parasitol. 2015;60(4):735–42. doi: 10.1515/ap-2015-0105
- Li R, Zhou X, Liu D, et al. Enhancing the activity and stability of Mn-superoxide dismutase by one-by-one ligation to catalase. Free Radic Biol Med. 2018;129:138–145. doi: 10.1016/j.freeradbiomed.2018.09.018
- Fang H, Liu A, Chen X, et al. The severity of LPS induced inflammatory injury is negatively associated with the functional liver mass after LPS injection in rat model. J Inflamm (Lond). 2018;15(1):21. doi: 10.1186/s12950-018-0197-4
- Gao H, Yang T, Chen X, et al. Changes of lipopolysaccharide-induced acute kidney and liver injuries in rats based on metabolomics analysis. J Inflamm Res. 2021;14:1807–1825. doi: 10.2147/JIR.S306789
- Hsing CH, Lin MC, Choi PC, et al. Anesthetic propofol reduces endotoxic inflammation by inhibiting reactive oxygen species-regulated Akt/IKKbeta/NF-kappaB signaling. PLoS One. 2011;6(3):e17598. doi: 10.1371/journal.pone.0017598
- Badr OM, Abd-Eltawab HM, Sakr SA. Ameliorative effect of ginger extract against pathological alterations induced in mice bearing solid tumors. J Biosci Appl Res. 2016;2(3):185–96. doi: 10.21608/jbaar.2016.106941
- Jeyakumar S, Nalini N, Menon VP. Antioxidant activity of ginger (zingiber officinale Rosc) in rats fed a high fat diet. Med Sci Res. 1999;27:341–344.
- Masuda Y, Kikuzaki H, Hisamoto M, et al. Antioxidant properties of gingerol related compounds from ginger. BioFactors. 2004;21(1–4):293–6. doi: 10.1002/biof.552210157
- Lebda M, Taha N, Korshom A, et al., Biochemical effect of ginger on some blood and liver parameters in male New Zealand rabbits. Online J Anim Feed Res, 2012;2:197–202. doi: 10.5555/20123245654
- Lin CC, Huang PC. Antioxidant and hepatoprotective effects of Acathopanax senticosus. Phytother Res. 2000;14(7):489–494. doi: 10.1002/1099-1573(200011)14:7<489:AID-PTR656>3.0.CO;2-G
- Atta AH, Elkoly TA, Mouneir SM, et al. Hepatoprotective effect of methanol extracts of zingiber officinale and cichorium intybus. Indian J Pharm Sci. 2010;72(5):564–70. doi: 10.4103/0250-474X.78521
- Naik SR, Panda VS. Hepatoprotective effect of Ginkgoselect Phytosome in rifampicin induced liver injury in rats: evidence of antioxidant activity. Fitoterapia. 2008;79(6):439–445. doi: 10.1016/j.fitote.2008.02.013
- Howell BA, Siler SQ, Shoda LK, et al. A mechanistic model of drug-induced liver injury AIDS the interpretation of elevated liver transaminase levels in a phase I clinical trial. CPT Pharmacometrics Syst Pharmacol. 2014;3(2):e98. doi: 10.1038/psp.2013.74
- Sidhu I, Bhatti J, Bhatti GK. Modulatory action of melatonin against chlorpyrifos induced hepatotoxicity in Wistar rats. Asian J Multidisc Stud. 2014;2:123–131.
- Özmen Ö, İ̇pek V. Effects of lacosamide in rats with lipopolysaccharide induced hepatic pathology. J Res Vet Med. 2020;39(1):54–60. doi: 10.30782/jrvm.665878
- Badawi MS. Histological study of the protective role of ginger on piroxicam-induced liver toxicity in mice. J Chin Med Assoc. 2019;82(1):11–18. doi: 10.1016/j.jcma.2018.06.006
- Abdel-Azeem AS, Hegazy AM, Ibrahim KS, et al. Hepatoprotective, antioxidant, and ameliorative effects of ginger (zingiber officinale Roscoe) and vitamin E in acetaminophen treated rats. J Diet Suppl. 2013;10(3):195–209. doi: 10.3109/19390211.2013.822450
- Lebda M, Taha N, Korshom M, et al. Ginger (zingiber officinale) potentiate paracetamol induced chronic hepatotoxicity in rats. J Med Plant Res. 2013;7:3164–3170. doi: 10.5897/JMPR2013.5252
- Jabran AG, Soeharto S, Sujuti H. The effect of ginger (zingiber officinale roscoe) extract on liver histopathology and alanine aminotransferase serum level in carbofuran-induced rats. Int J Pharmtech Res. 2015;8:889–897.
- Ghasemzadeh A, Jaafar HZ, Rahmat A. Antioxidant activities, total phenolics and flavonoids content in two varieties of Malaysia young ginger (zingiber officinale Roscoe). Molecules. 2010;15(6):4324–4333. doi: 10.3390/molecules15064324
- . Sakr S, Mahran H, Lamfon H. Protective effect of ginger (zingiber officinale) on adriamycin -induced hepatotoxicity in albino rats. J Med Plant Res. 2010;5. Available from: https://academicjournals.org/journal/JMPR/article-full-text-pdf/CF5E4B716805
- Alsahli MA, Almatroodi SA, Almatroudi A, et al. 6-gingerol, a major ingredient of ginger attenuates diethylnitrosamine-induced liver injury in rats through the modulation of oxidative stress and anti-inflammatory activity. Mediators Inflamm. 2021;2021:6661937. doi: 10.1155/2021/6661937
- Simmons EM, Himmelfarb J, Sezer MT, et al. Plasma cytokine levels predict mortality in patients with acute renal failure. Kidney Int. 2004;65(4):1357–1365. doi: 10.1111/j.1523-1755.2004.00512.x
- Zheng C, Zhou Y, Huang Y, et al. Effect of ATM on inflammatory response and autophagy in renal tubular epithelial cells in LPS-induced septic AKI. Exp Ther Med. 2019;18(6):4707–4717. doi: 10.3892/etm.2019.8115
- Kang DG, Sohn EJ, Moon MK, et al. Rehmannia glutinose ameliorates renal function in the ischemia/reperfusion-induced acute renal failure rats. Biol Pharm Bull. 2005;28(9):1662–7. doi: 10.1248/bpb.28.1662
- Vaden SL. Glomerular disease. Top Companion Anim Med. 2011;26(3):128–34. doi: 10.1053/j.tcam.2011.04.003
- Tseng CY, Yu PR, Hsu CC, et al. The effect of isovitexin on lipopolysaccharide-induced renal injury and inflammation by induction of protective autophagy. Food Chem Toxicol. 2023;172:113581. doi: 10.1016/j.fct.2022.113581
- Zhang D, Ji P, Sun R, et al. Corrigendum to “Ginsenoside Rg1 attenuates LPS-induced chronic renal injury by inhibiting NOX4-NLRP3 signaling in mice.” [Biomed. Pharmacother. 150 (2022) 112936]. Biomed Pharmacother. 2024;170(115965). doi: 10.1016/j.biopha.2023.115965
- Patil VP, Salunke BG. Fluid Overload and Acute Kidney Injury. Indian J Crit Care Med. 2020;24(Suppl 3):S94–S97. doi: 10.5005/jp-journals-10071-23401
- Aslan A, van den Heuvel MC, Stegeman CA, et al. Kidney histopathology in lethal human sepsis. Crit Care. 2018;22(1):359. doi: 10.1186/s13054-018-2287-3
- Huang Z, Fu Z, Huang W, et al. Prognostic value of neutrophil-to-lymphocyte ratio in sepsis: a meta-analysis. Am J Emerg Med. 2020;38(3):641–47. doi: 10.1016/j.ajem.2019.10.023
- Shan B, Cai YZ, Sun M, et al. Antioxidant capacity of 26 spice extracts and characterization of their phenolic constituents. J Agric Food Chem. 2005;53(20):7749–59. doi: 10.1021/jf051513y
- Policegoudra RS, Aradhya SM, Singh L. Mango ginger (Curcuma amada roxb.)–a promising spice for phytochemicals and biological activities. J Biosci. 2011;36(4):739–48. doi: 10.1007/s12038-011-9106-1
- Gabr SA, Alghadir AH, Ghoniem GA. Biological activities of ginger against cadmium-induced renal toxicity. Saudi J Biol Sci. 2019;26(2):382–89. doi: 10.1016/j.sjbs.2017.08.008
- Haniadka R, Saldanha E, Sunita V, et al. A review of the gastroprotective effects of ginger (zingiber officinale Roscoe). Food Funct. 2013;4(6):845–55. doi: 10.1039/c3fo30337c
- Palatty PL, Haniadka R, Valder B, et al. Ginger in the prevention of nausea and vomiting: a review. Crit Rev Food Sci Nutr. 2013;53(7):659–69. doi: 10.1080/10408398.2011.553751
- Maghsoudi S, Gol A, Dabiri S, et al. Preventive effect of ginger (zingiber officinale) pretreatment on renal ischemia-reperfusion in rats. Eur Surg Res. 2011;46(1):45–51. doi: 10.1159/000321704
- Shen P, Ji S, Li X, et al. LPS-Induced systemic inflammation caused mPOA-FSH/LH disturbance and impaired testicular function. Front Endocrinol. 2022;13(886085). doi: 10.3389/fendo.2022.886085
- Feng R, Adeniran SO, Huang F, et al. The ameliorative effect of melatonin on LPS-induced Sertoli cells inflammatory and tight junctions damage via suppression of the TLR4/MyD88/NF-kappaB signaling pathway in newborn calf. Theriogenology. 2022;179:103–116. doi: 10.1016/j.theriogenology.2021.11.020
- Cheng CY, Mruk DD. A local autocrine axis in the testes that regulates spermatogenesis. Nat Rev Endocrinol. 2010;6(7):380–95. doi: 10.1038/nrendo.2010.71
- Hedger MP. Toll-like receptors and signalling in spermatogenesis and testicular responses to inflammation–a perspective. J Reprod Immunol. 2011;88(2):130–141. doi: 10.1016/j.jri.2011.01.010
- Jegou B, Cudicini C, Gomez E, et al. Interleukin-1, interleukin-6 and the germ cell-Sertoli cell cross-talk. Reprod Fertil Dev. 1995;7(4):723–730. doi: 10.1071/rd9950723
- O’Bryan MK, Schlatt S, Phillips DJ, et al. Bacterial Lipopolysaccharide-Induced Inflammation Compromises Testicular Function at Multiple Levels in Vivo 1. Endocrinology. 2000;141(1):238–46. doi: 10.1210/endo.141.1.7240
- Allen JA, Diemer T, Janus P, et al. Bacterial endotoxin lipopolysaccharide and reactive oxygen species inhibit leydig cell steroidogenesis via perturbation of mitochondria. Endocrine. 2004;25(3):265–275. doi: 10.1385/ENDO:25:3:265
- Fijak M, Pilatz A, Hedger MP, et al. Infectious, inflammatory and ‘autoimmune’ male factor infertility: how do rodent models inform clinical practice? Hum Reprod Update. 2018;24(4):416–441. doi: 10.1093/humupd/dmy009
- Kumar J, Haldar C, Verma R. Effect of Quinalphos on testes of long day seasonal breeder golden hamster, Mesocricetus auratus. J Sci Res. 2020;64(1):97–102. doi: 10.37398/jsr.2020.640114
- Banihani SA. Testosterone in males as enhanced by onion (Allium Cepa L.). Biomolecules. 2019;9(2):75. doi: 10.3390/biom9020075
- Idris NA, Yasin HM, Usman A. Voltammetric and spectroscopic determination of polyphenols and antioxidants in ginger (zingiber officinale Roscoe). Heliyon. 2019;5(5):e01717. doi: 10.1016/j.heliyon.2019.e01717
- Aktan F, Henness S, Tran VH, et al. Gingerol metabolite and a synthetic analogue Capsarol inhibit macrophage NF-kappaB-mediated iNOS gene expression and enzyme activity. Planta Med. 2006;72(8):727–734. doi: 10.1055/s-2006-931588
- Mohammadi F, Nikzad H, Taghizadeh M, et al. Protective effect of zingiber officinale extract on rat testis after cyclophosphamide treatment. Andrologia. 2014;46(6):680–6. doi: 10.1111/and.12135
- Akbari A, Nasiri K, Heydari M, et al. The protective effect of hydroalcoholic extract of zingiber officinale Roscoe (ginger) on ethanol-induced reproductive toxicity in male rats. J Evid Based Complementary Altern Med. 2017;22(4):609–17. doi: 10.1177/2156587216687696
- Shalaby MA, Hamowieh AR. Safety and efficacy of zingiber officinale roots on fertility of male diabetic rats. Food Chem Toxicol. 2010;48(10):2920–4. doi: 10.1016/j.fct.2010.07.028
- Afolabi A. Beneficial effects of ethanol extract of zingiber officinale (ginger) rhizome on epididymal sperm and plasma oxidative stress parameters in experimentally cryptorchid rats. Annual Res Review In Biol. 2014;4(9):1448–1460. doi: 10.9734/arrb/2014/6078
- Khaki AA. Pp-1 the effects of ginger on spermatogenesis and sperm parameters of rat. Reprod Biomed Online. 2012;24(S5). doi: 10.1016/s1472-6483(12)60133-3
- Gholami-Ahangaran M, Karimi-Dehkordi M, Akbari Javar A, et al. A systematic review on the effect of Ginger (zingiber officinale) on improvement of biological and fertility indices of sperm in laboratory animals, poultry and humans. Vet Med Sci. 2021;7(5):1959–1969. doi: 10.1002/vms3.538