ABSTRACT
Translocated promoter region (TPR) serves as a crucial component within the nuclear pore complex (NPC), situated in the nuclear basket region. Initially identified through the TPR-Met oncogene fusion, TPR exhibits a diverse array of cellular functions encompassing nucleocytoplasmic transport, transcriptional regulation, mitosis, and autophagy. Notably, recent research has underscored TPR’s significance in oncogenesis across various cancer types, such as ependymoma, cervical, and colorectal cancer. In this discourse, we provide an overview of TPR’s multifaceted roles and explore its implications in carcinogenesis, with a particular focus on its involvement in autophagy and its association with cancer progression.
KEYWORDS:
Introduction
The translocated promoter region (TPR) constitutes an integral part of nuclear pore complexes (NPCs), which are macromolecular assemblies comprising approximately 30 distinct proteins referred to as nucleoporins (Nups) [Citation1]. The nuclear basket, a visually prominent component of NPCs, is composed of TPR, Nup153, and Nup50 (). TPR, with a molecular size of 267 kDa, is tethered to the NPC through its interaction with Nup153 [Citation2]. Additionally, TPR binds to Nup98, although this interaction does not govern TPR’s incorporation into the NPC [Citation3].
During mitosis, both the nuclear envelope and the nuclear pore complex (NPC) disassemble, generating nuclear envelope fragments containing Nups complexes that play crucial roles [Citation4]. Among these, nucleoporin TPR has been recognized for its involvement in the mitotic spindle assembly checkpoint (SAC) through interactions with Mad1 and Mad2, pivotal components of SAC [Citation5]. The SAC functions to prevent aberrant mitotic processes leading to aneuploidy, a hallmark of cancer. TPR interacts with Mad1 and Mad2 in distinct regions; Mad1 binds to TPR’s N-terminal region, while Mad2 binds to its C-terminal region. Phosphorylation of TPR at the S2059 residue by CDK1 is crucial for TPR-Mad1 interaction. Disruption of S2059 phosphorylation abolishes this interaction, compromising the localization of both Mad1 and Mad2, thereby inducing cell cycle abnormalities [Citation6].
Methodology
This review encompasses a broad selection of journal articles, with no restriction on publication year. The literature search utilized key terms such as ‘TPR’, ‘autophagy’, ‘cancer therapy’, ‘carcinogenesis’, and ‘nucleoporin’ to locate relevant studies. Sources were identified through various electronic databases, including Google Scholar, PubMed, ScienceDirect, and Scopus. A total of 66 articles were selected for this review, and their findings are presented in a narrative format. To manage references efficiently, we used EndNote 20.5 software. This review only evaluated articles published in English.
The role of autophagy in cancer
Autophagy, the process of degrading cellular organelles within autophagosomes, encompasses several types including macroautophagy, microautophagy, and chaperone-mediated autophagy. Among these, macroautophagy, hereafter referred to simply as autophagy, is the most extensively studied and is active in all cells [Citation7–9]. Under normal conditions, autophagy plays a vital role in maintaining biological function and homeostasis by breaking down misfolded proteins and damaged organelles. However, its rate can increase in response to starvation, serving as an internal mechanism for ATP synthesis until external nutrients become available [Citation9,Citation10].
Autophagy is also integral to physiological cellular processes such as development, cell death, and tumor suppression [Citation11]. In cancer biology, it assumes dual roles in both tumor promotion and suppression [Citation12,Citation13]. The regulation of autophagy contributes to the expression of tumor suppressor proteins or oncogenes. Tumor suppressor proteins are negatively regulated by mTOR and AMPK, leading to autophagy induction and suppression of carcinogenesis [Citation14]. Conversely, oncogenes may be activated by mTOR, PI3K, and AKT, inhibiting autophagy and initiating cancer formation [Citation15].
Basal autophagy is considered to be a factor in cancer suppression [Citation16,Citation17]. Reduced autophagy inhibits the degradation of damaged organelles or misfolded proteins, thus initiating cancer. Basal autophagy acts as a tumor suppressor mechanism by reducing damaged cellular components and maintaining cellular homeostasis [Citation18] (). Numerous studies have reported that depletion of BECN1 (encoding Beclin 1), an autophagy-related gene, is observed in human breast, prostate, and ovarian cancers [Citation17,Citation19], suggesting its function as a tumor suppressor. Other studies have linked the suppression of autophagic factors such as ATG3, ATG5, and ATG9 to oncogenesis [Citation20–22]. Additionally, autophagy prevents tumor initiation by regulating reactive oxygen species (ROS). Mitochondrial damage induces high ROS production, promoting carcinogenesis [Citation23–25]. These findings underscore the crucial role of autophagy in inhibiting tumor initiation, with aberrant autophagy potentially leading to carcinogenesis. According to a recent review, modulation of autophagy exhibits anti-tumor properties in several pediatric brain tumors, including ependymoma, medulloblastoma, retinoblastoma, atypical teratoid, and gliomas [Citation26].
Autophagy, acting as a double-edged sword, has been implicated in both promoting tumor survival and growth in advanced cancers [Citation27,Citation28]. Cancer cells confront highly stressful environments characterized by factors such as hypoxia and nutrient scarcity. In this context, autophagy aids cancer cells in surmounting these challenges by recycling intracellular components to provide metabolic substrates [Citation28,Citation29]. Consequently, autophagy assumes a pivotal role in bolstering cancer cell survival by augmenting stress tolerance and meeting the metabolic demands of malignancies. Depletion of autophagy-related genes can lead to tumor cell death, underscoring the critical role of autophagy in cancer cell viability [Citation30] (). Furthermore, inhibition of autophagy-related factors contributes to the accumulation of damaged mitochondria and impedes cell growth [Citation31,Citation32].
The role of TPR in autophagy
Nuclear pore complexes (NPCs) serve as vital conduits for nuclear transport, facilitating the movement of proteins and RNAs between the cytoplasm and nucleoplasm. Depletion of TPR has been shown to increase p53 nuclear accumulation and promote autophagy in HeLa cells [Citation33]. P53, a transcription factor with diverse regulatory functions, including the modulation of autophagy genes, oversees the expression of several downstream targets such as damage-regulated autophagy modulator (DRAM) and Isg20L, Ulk1, and Atg7 [Citation34–36]. TPR depletion facilitates autophagy by modulating the export of heat shock protein (HSP)-70 and heat shock transcription factor (HSF)-1 mRNA. HSF1, activated under stress conditions, induces the expression of HSP70 and HSP90, thereby suppressing MAPK8/JNK activity and preserving mTORC1 integrity and function, a key regulator of autophagy [Citation37,Citation38]. Additionally, HSF1-mediated phosphorylation of SQSTM1/p62 promotes aggrephagy, a stress response pathway facilitating protein aggregate clearance [Citation39] ().
Figure 3. The role of TPR in autophagy. TPR overexpression prevents autophagy induction (above), and its depletion responsible for autophagy induction (below).
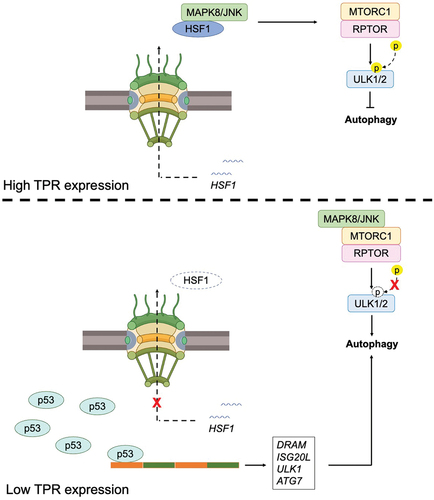
Highly elevated levels of TPR have been observed in human ependymoma tumors, coinciding with the decreased expression of autophagy-related genes such as ATG3, ATG5, ATG12, and BECN1. This dysregulation in autophagy is further confirmed by alterations in the levels of LC3-II and p62 proteins. Intriguingly, knocking down TPR restores the expression of these genes and triggers autophagy induction. Notably, TPR depletion induces nuclear membrane blebbing, indicating a potential role in impeding nucleophagy, a selective autophagy process targeting nuclear components, thereby promoting tumorigenesis in ependymoma. Similar observations were made in a xenograft mouse model, where downregulation of TPR using rapamycin resulted in inhibited tumor growth in vivo. These findings underscore the importance of upregulating autophagy by targeting TPR to mitigate the tumorigenic potential of ependymoma, offering a promising therapeutic strategy for patients [Citation40]. Additionally, another reported that TPR mediates an autophagy transcriptional program induced by IFN-γ in leprosy-stimulated cells [Citation41]. Elevated TPR levels in type 1 reaction (T1R) cells, triggered by IFN-γ stimulation, coincide with autophagy blockade observed during T1R development in multibacillary patients [Citation42]. Interaction prediction tools reveal direct interaction between TPR and the central autophagy regulator mTOR, linking to RPTOR and UVRAG. These findings suggest a potential regulatory role of TPR in autophagy induction in both paucibacillary (PB) leprosy and T1R in multibacillary (MB) patients [Citation41].
TPR interacts with activating molecule in Beclin 1-regulated autophagy protein 1 (Ambra1), suggesting a potential association between Ambra1 and nucleoporins during nuclear entry or as part of its nuclear activity. Ambra1, recognized for its roles in autophagy, neurogenesis, and cancer metastasis, undergoes ULK1-mediated phosphorylation during autophagy initiation, promoting phagophore formation [Citation43–45]. Although TPR-Ambra1 interaction has been reported, its significance in the autophagy process remains elusive.
Targeting TPR in cancer therapy
TPR plays a diverse role in various cellular functions, spanning autophagy, mitosis, transcriptional regulation, chromatin organization, and nuclear transport [Citation4,Citation40,Citation46,Citation47]. The expression level of TPR has been noted to be elevated in various cancers, rendering it a potential prognostic biomarker and therapeutic target for hepatocellular carcinoma, ependymoma, breast cancer, lung cancer, and colorectal cancer [Citation4,Citation40,Citation46,Citation48–50]. Originally identified in oncogenic fusions with receptor tyrosine kinases such as Met, TRK, Raf, and ALK [Citation47,Citation49], TPR’s involvement in cancer pathogenesis is multifaceted. For instance, the fusion of TPR with the Met receptor, a hepatocyte growth factor (HGF) receptor, leads to aberrant Met localization and activity. This fusion product, TPR-Met, localizes to the cytoplasm and can activate signaling pathways without HGF binding, ultimately promoting proliferation, migration, and invasion of cancer cells through continuous Met activation [Citation51]. Further supporting TPR’s role in cancer, the TPR-ALK fusion has been identified in lung adenocarcinoma patients. This fusion protein contains coiled-coil and kinase domains, granting it transforming capabilities and sensitivity to ALK inhibitors, which makes it a significant therapeutic target for cancer treatment [Citation49]. Elevated TPR expression in lung cancer tissues has been correlated with a poorer prognosis. In lung cancer cell lines, knockdown of TPR inhibits tRNA nuclear export, disrupts protein synthesis, and slows cell growth, indicating that TPR is crucial for cancer cell survival and could be a strategic point for therapeutic intervention [Citation50].
Although human nucleoporin TPR is frequently deregulated in cancer, its exact roles remain largely unexplored. Recent studies suggest that depleting TPR induces transcription-dependent replication stress, DNA breaks, and genomic instability. This effect appears to be connected to TPR’s interaction with GANP, a component of the TREX-2 complex. The relationship between TPR and GANP is further supported by their shared protein level changes in a cohort of ovarian carcinomas. This points to an intriguing network linking nucleoporins with DNA transcription and replication, revealing a physical connection between replication forks, transcription, splicing, and mRNA export machinery [Citation52]. Despite TPR’s primary function in nuclear transport, it has also been implicated in other processes that may influence cancer progression. Chromosomal translocations leading to fusions between TPR and kinases are known to drive oncogenesis, suggesting that TPR might play a role in promoting carcinogenesis through various pathways, including autophagy regulation, cell cycle checkpoints, and MAPK signaling [Citation47]. In breast cancer, TPR expression is elevated, regulated by long non-coding RNA (LINC)1705 via competitive binding to miR-186-5p [Citation46]. Depletion of TPR in HeLa cells results in increased nuclear accumulation of p53, thereby upregulating the expression of tumor suppressor genes p21 and p16 [Citation33]. Additionally, TPR depletion induces autophagy in ependymoma and breast cancer cell lines, leading to the inhibition of cancer cell growth [Citation33,Citation40]. These multifaceted roles underscore TPR’s significance in various cellular processes and its contribution to cancer pathogenesis.
Previous research has highlighted the pivotal role of TPR as an anchor for spindle assembly checkpoint (SAC) components during both interphase and mitosis. TPR forms direct interactions with Mad1 and Mad2, key proteins central to SAC functionality. Depletion of TPR in HeLa cells using siRNA disrupts the association between Mad1 and Mad2, culminating in aneuploidy and multinucleated cells [Citation5,Citation53,Citation54]. Additionally, TPR interacts with A-kinase anchoring protein 95 (AKAP95), an essential protein for SAC integrity. The involvement of AKAP95 in SAC function is likely mediated through its interaction with TPR [Citation55]. Another study revealed that TPR downregulation alters centrosome localization and Aurora A phosphorylation, leading to chromosome lagging, tetraploidy, and polyploidy [Citation56]. Furthermore, glycogen synthase kinase-3β inhibition causes TPR depletion and mislocalization, along with reduced levels of the motor protein dynein, ultimately triggering mitotic catastrophe in colorectal cancer cells [Citation4]. In addition to TPR, other nucleoporins significantly contribute to the proper functioning of mitosis. For example, Nup58, which is initially located at centrosomes and the mitotic spindle during metaphase, later relocates to midbodies during abscission, indicating its critical role in the mitotic process [Citation57]. Meanwhile, Nup358/RanBP2 plays a key role in maintaining mitotic progression and chromosomal segregation. Its depletion leads to mitotic arrest and subsequent cell death, highlighting its essential role in the cell cycle [Citation58]. Nup62 is another crucial nucleoporin, as its loss in human cells results in multinucleated cells, multipolar centrosomes, spindle orientation defects, and disorganized centrosome components, which in turn disrupt cell bipolarity [Citation59]. The Rae1-binding domain of NuMA also impacts mitosis. When overexpressed in HeLa cells, it induces abnormal spindle formation, suggesting the Rae1-NuMA interaction is crucial for normal spindle assembly [Citation60]. These findings collectively underscore the critical role of TPR and other nucleoporin in ensuring accurate spindle assembly and chromosome segregation during cell division, underlining the importance of their functions in maintaining cell cycle integrity.
Mitotic catastrophe, a form of cell death resulting from aberrant mitosis, often culminates in the formation of multinucleated cells [Citation61]. Key hallmarks of irreversible mitotic catastrophe include chromosome fragmentation and the emergence of micronuclei [Citation62]. Targeting TPR in cancer cells can potentially induce the formation of polyploid cells, leading to irreversible mitotic cell death. However, it is noteworthy that aneuploidy and polyploidy can confer a growth advantage and facilitate cellular transformation [Citation63]. The consequences of aneuploidy are increasingly recognized as context-dependent, where in certain scenarios, aneuploidy might suppress tumor development. This paradoxical observation suggests that low rates of chromosome mis-segregation could promote cancer development, while higher levels may trigger mitotic cell death and impede tumorigenesis. Thus, understanding the delicate balance between aneuploidy-induced growth promotion and mitotic catastrophe-induced tumor suppression is crucial for elucidating cancer progression mechanisms.
Depletion of TPR has been shown to disrupt the centrosomal localization of Aurora A and p53, potentially leading to errors in centrosome and chromosome segregation [Citation56]. Notably, p53 has been implicated as an inhibitor of both Mad2 and Aurora A, suggesting a regulatory role in mitotic processes. P53-deficient cells exhibit premature chromosome separation and accumulate lagging chromosomes, further highlighting its involvement in maintaining genomic stability [Citation64]. Additionally, TPR depletion enhances p53 nuclear accumulation and promotes autophagy [Citation33]. In breast cancer, inhibition of Aurora A has been linked to autophagy induction, as evidenced by increased levels of autophagosomes and LC3-II, accompanied by a reduction in SQSTM1 [Citation65]. Interestingly, p53 has been shown to inhibit both Mad2 and Aurora A during mitosis, further emphasizing its multifaceted regulatory functions [Citation66]. These findings underscore the intricate interplay between TPR, p53, Aurora A, and autophagy in maintaining cellular homeostasis and regulating mitotic processes.
Conclusion
TPR plays a pivotal role in various pathways associated with carcinogenesis, notably including autophagy. Depletion of TPR results in the nuclear accumulation of both heat shock factor 1 (HSF1) and p53, correlating with the induction of autophagy. HSF1 exerts its effect by suppressing MAPK8/JNK, thereby preserving the integrity and activity of mTORC1, the master regulator of autophagy. Additionally, p53 serves as a transcription factor responsible for regulating numerous autophagy-related genes such as Isg20L, Ulk1, and Atg7. These findings highlight the multifunctional nature of TPR, underscoring the importance of understanding its expression levels in various cellular functions.
Disclosure statement
No potential conflict of interest was reported by the author(s).
Data availability statement
The authors declare that all data generated or analyzed during this study are available within the article.
Additional information
Funding
References
- Wong RW, New activities of the nuclear pore complexes.Cells, 2021. 10(8). doi: 10.3390/cells10082123
- Hase ME, Cordes VC, Direct interaction with nup153 mediates binding of tpr to the periphery of the nuclear pore complex. Mol Biol Cell, 2003. 14(5):1923–1940. doi: 10.1091/mbc.e02-09-0620
- Fontoura BM, Dales S, Blobel G, et al. The nucleoporin Nup98 associates with the intranuclear filamentous protein network of TPR. Proc Natl Acad Sci U S A. 2001;98(6):3208–3213. doi: 10.1073/pnas.061014698
- Dewi FRP, Domoto T, Hazawa M, et al., Colorectal cancer cells require glycogen synthase kinase-3beta for sustaining mitosis via translocated promoter region (TPR)-dynein interaction. Oncotarget, 2018. 9(17):13337–13352. doi: 10.18632/oncotarget.24344
- Nakano H, Funasaka T, Hashizume C, et al. Nucleoporin translocated promoter region (tpr) associates with dynein complex, preventing chromosome lagging formation during mitosis. J Biol Chem. 2010;285(14):10841–10849. doi: 10.1074/jbc.M110.105890
- Rajanala K, Sarkar A, Jhingan GD et al., Correction: phosphorylation of nucleoporin Tpr governs its differential localization and is required for its mitotic function. J Cell Sci, 2022. 135(6). doi: 10.1242/jcs.260004
- Mizushima N, Levine B, Cuervo AM, et al. Autophagy fights disease through cellular self-digestion. Nature. 2008;451(7182):1069–1075. doi: 10.1038/nature06639
- Kraft C, Martens S. Mechanisms and regulation of autophagosome formation. Curr Opin Cell Biol. 2012;24(4):496–501. doi: 10.1016/j.ceb.2012.05.001
- Maiuri MC, Tasdemir E, Criollo A, et al. Control of autophagy by oncogenes and tumor suppressor genes. Cell Death Differ. 2009;16(1):87–93. doi: 10.1038/cdd.2008.131
- Mah LY, O’Prey J, Baudot AD, et al. DRAM-1 encodes multiple isoforms that regulate autophagy. Autophagy. 2012;8(1):18–28. doi: 10.4161/auto.8.1.18077
- Yun CW, Lee SH, The roles of autophagy in cancer. Int J Mol Sci, 2018. 19(11). 19 11. doi: 10.3390/ijms19113466
- Rosenfeldt MT, Ryan KM. The multiple roles of autophagy in cancer. Carcinogenesis. 2011;32(7):955–963. doi: 10.1093/carcin/bgr031
- Gewirtz DA. The four faces of autophagy: implications for cancer therapy. Cancer Res. 2014;74(3):647–651. doi: 10.1158/0008-5472.CAN-13-2966
- Comel A, Sorrentino G, Capaci V, et al., The cytoplasmic side of p53‘s oncosuppressive activities.FEBS Lett, 2014. 588(16):2600–2609. doi: 10.1016/j.febslet.2014.04.015
- Choi AM, Ryter SW, Levine B, Autophagy in human health and disease. N Engl J Med, 2013. 368(7):651–662. doi: 10.1056/NEJMra1205406
- Wang T, Hu J, Luo H, et al., Photosensitizer and autophagy promoter coloaded ROS-Responsive dendrimer-assembled carrier for synergistic enhancement of tumor growth suppression. Small, 2018. 14(38):e1802337. doi: 10.1002/smll.201802337
- Qu X, Yu J, Bhagat G, et al. Promotion of tumorigenesis by heterozygous disruption of the beclin 1 autophagy gene. J Clin Invest. 2003;112(12):1809–1820. doi: 10.1172/JCI20039
- Tang D, Kang R, Livesey KM, et al. Endogenous HMGB1 regulates autophagy. J Cell Bio. 2010;190(5):881–892. doi: 10.1083/jcb.200911078
- Shen Y, Li D-D, Wang L-L, et al. Decreased expression of autophagy-related proteins in malignant epithelial ovarian cancer. Autophagy. 2008;4(8):1067–1068. doi: 10.4161/auto.6827
- Kuma A, Hatano M, Matsui M, et al. The role of autophagy during the early neonatal starvation period. Nature. 2004;432(7020):1032–1036. doi: 10.1038/nature03029
- Komatsu M, Waguri S, Ueno T, et al. Impairment of starvation-induced and constitutive autophagy in Atg7-deficient mice. J Cell Bio. 2005;169(3):425–434. doi: 10.1083/jcb.200412022
- Sou YS, Waguri S, Iwata J-I, et al. The Atg8 conjugation system is indispensable for proper development of autophagic isolation membranes in mice. Mol Biol Cell. 2008;19(11):4762–4775. doi: 10.1091/mbc.e08-03-0309
- Moloney JN, Cotter TG, ROS signalling in the biology of cancer. Semin Cell Dev Biol, 2018. 80:50–64. doi: 10.1016/j.semcdb.2017.05.023
- Avalos Y, Canales J, Bravo-Sagua R, et al., Tumor suppression and promotion by autophagy. Biomed Res Int, 2014. 2014:1–15. doi: 10.1155/2014/603980
- Filomeni G, De Zio D, Cecconi F. Oxidative stress and autophagy: the clash between damage and metabolic needs. Cell Death Differ. 2015;22(3):377–388. doi: 10.1038/cdd.2014.150
- Gatto F, Milletti G, Carai A, et al. Recent advances in understanding the role of autophagy in paediatric brain tumours. Diagn (Basel). 2021;11(3):481. doi: 10.3390/diagnostics11030481
- Luo T, Fu J, Xu A, et al. PSMD10/gankyrin induces autophagy to promote tumor progression through cytoplasmic interaction with ATG7 and nuclear transactivation of ATG7 expression. Autophagy. 2016;12(8):1355–1371. doi: 10.1080/15548627.2015.1034405
- Liu EY, Ryan KM. Autophagy and cancer–issues we need to digest. J Cell Sci. 2012;125(Pt 10):2349–2358. doi: 10.1242/jcs.093708
- Rabinowitz JD, White E, Autophagy and metabolism. Science, 2010. 330(6009):1344–1348. doi: 10.1126/science.1193497
- Wei H, Wei S, Gan B, et al. Suppression of autophagy by FIP200 deletion inhibits mammary tumorigenesis. Genes Dev. 2011;25(14):1510–1527. doi: 10.1101/gad.2051011
- Guo JY, Karsli-Uzunbas G, Mathew R, et al. Autophagy suppresses progression of K-ras-induced lung tumors to oncocytomas and maintains lipid homeostasis. Genes Dev. 2013;27(13):1447–1461. doi: 10.1101/gad.219642.113
- Guo JY, White E, Autophagy is required for mitochondrial function, lipid metabolism, growth, and fate of KRAS(G12D)-driven lung tumors. Autophagy, 2013. 9(10):1636–1638. doi: 10.4161/auto.26123
- Funasaka T, Tsuka E, Wong RW, Regulation of autophagy by nucleoporin Tpr. Sci Rep, 2012. 2:878. doi: 10.1038/srep00878
- Crighton D, Wilkinson S, O’ Prey J, et al., DRAM, a p53-induced modulator of autophagy, is critical for apoptosis. Cell, 2006. 126(1):121–134. doi: 10.1016/j.cell.2006.05.034
- Eby KG, Rosenbluth JM, Mays DJ, et al., ISG20L1 is a p53 family target gene that modulates genotoxic stress-induced autophagy. Mol Cancer, 2010. 9:95. 1. doi: 10.1186/1476-4598-9-95
- Kenzelmann Broz D, Spano Mello S, Bieging KT, et al. Global genomic profiling reveals an extensive p53-regulated autophagy program contributing to key p53 responses. Genes Dev. 2013;27(9):1016–1031. doi: 10.1101/gad.212282.112
- Morimoto RI. Regulation of the heat shock transcriptional response: cross talk between a family of heat shock factors, molecular chaperones, and negative regulators. Genes Dev. 1998;12(24):3788–3796. doi: 10.1101/gad.12.24.3788
- Zhang B, Fan Y, Cao P, et al. Multifaceted roles of HSF1 in cell death: a state-of-the-art review. Biochim Biophys Acta Rev Cancer. 2021;1876(2):188591. doi: 10.1016/j.bbcan.2021.188591
- Watanabe Y, Tsujimura A, Taguchi K, et al. HSF1 stress response pathway regulates autophagy receptor SQSTM1/p62-associated proteostasis. Autophagy. 2017;13(1):133–148. doi: 10.1080/15548627.2016.1248018
- Dewi FRP, Jiapaer S, Kobayashi A, et al., Nucleoporin TPR (translocated promoter region, nuclear basket protein) upregulation alters MTOR-HSF1 trails and suppresses autophagy induction in ependymoma. Autophagy, 2021. 17(4):1001–1012. doi: 10.1080/15548627.2020.1741318
- Silva BJA, Bittencourt TL, Leal-Calvo T, et al., Autophagy-Associated IL-15 Production Is Involved in the pathogenesis of leprosy type 1 reaction. Cells, 2021. 10(9). 2215 doi: 10.3390/cells10092215
- de Mattos Barbosa MG, de Andrade Silva BJ, Assis TQ et al., Autophagy impairment is associated with increased inflammasome activation and reversal reaction development in multibacillary leprosy. Front Immunol, 2018. 9:1223. doi: 10.3389/fimmu.2018.01223
- Schoenherr C, Byron A, Sandilands E, et al., Ambra1 spatially regulates src activity and Src/FAK-mediated cancer cell invasion via trafficking networks. Elife, 2017. 6. 6. doi: 10.7554/eLife.23172
- Schoenherr C, Byron A, Griffith B, et al. The autophagy protein Ambra1 regulates gene expression by supporting novel transcriptional complexes. J Biol Chem. 2020;295(34):12045–12057. doi: 10.1074/jbc.RA120.012565
- Sun WL. Ambra1 in autophagy and apoptosis: implications for cell survival and chemotherapy resistance. Oncol Lett. 2016;12(1):367–374. doi: 10.3892/ol.2016.4644
- Du C, Zhang J-L, Wang Y, et al., The long non-coding RNA LINC01705 regulates the development of breast cancer by sponging miR-186-5p to mediate TPR expression as a competitive endogenous RNA. Front Genet, 2020. 11:779. doi: 10.3389/fgene.2020.00779
- Snow CJ, Paschal BM, Roles of the nucleoporin tpr in cancer and aging. Adv Exp Med Biol, 2014. 773:309–322.
- Long T, Wu W, Wang X, et al. TPR is a prognostic biomarker and potential therapeutic target associated with immune infiltration in hepatocellular carcinoma. Mol Clin Oncol. 2024;20(4):27. doi: 10.3892/mco.2024.2725
- Choi YL, Lira ME, Hong M, et al. A novel fusion of TPR and ALK in lung adenocarcinoma. J Thorac Oncol. 2014;9(4):563–566. doi: 10.1097/JTO.0000000000000093
- Chen M, Long Q, Borrie MS, et al. Nucleoporin TPR promotes tRNA nuclear export and protein synthesis in lung cancer cells. PloS Genet. 2021;17(11):e1009899. doi: 10.1371/journal.pgen.1009899
- Peschard P, Park M. From tpr-met to met, tumorigenesis and tubes. Oncogene. 2007;26(9):1276–1285. doi: 10.1038/sj.onc.1210201
- Kosar M, Giannattasio M, Piccini D, et al. The human nucleoporin Tpr protects cells from RNA-mediated replication stress. Nat Commun. 2021;12(1):3937. doi: 10.1038/s41467-021-24224-3
- Lee SH, Sterling H, Burlingame A, et al., Tpr directly binds to Mad1 and Mad2 and is important for the Mad1–Mad2-mediated mitotic spindle checkpoint. Genes Dev, 2008. 22(21):2926–2931. doi: 10.1101/gad.1677208
- Schweizer N, Ferrás C, Kern DM, et al. Spindle assembly checkpoint robustness requires Tpr-mediated regulation of Mad1/Mad2 proteostasis. J Cell Bio. 2013;203(6):883–893. doi: 10.1083/jcb.201309076
- Lopez-Soop G, Rønningen T, Rogala A, et al. AKAP95 interacts with nucleoporin TPR in mitosis and is important for the spindle assembly checkpoint. Cell Cycle. 2017;16(10):947–956. doi: 10.1080/15384101.2017.1310350
- Kobayashi A, Hashizume C, Dowaki T, et al. Therapeutic potential of mitotic interaction between the nucleoporin tpr and aurora kinase a. Cell Cycle. 2015;14(9):1447–1458. doi: 10.1080/15384101.2015.1021518
- Hartono, H, Hazawa M, et al., Nucleoporin Nup58 localizes to centrosomes and mid-bodies during mitosis. Cell Div, 2019. 14:7. 1. doi: 10.1186/s13008-019-0050-z
- Hashizume C, Kobayashi A, Wong RW. Down-modulation of nucleoporin RanBP2/Nup358 impaired chromosomal alignment and induced mitotic catastrophe. Cell Death Dis. 2013;4(10):e854. doi: 10.1038/cddis.2013.370
- Hashizume C, Moyori A, Kobayashi A, et al., Nucleoporin Nup62 maintains centrosome homeostasis. Cell Cycle, 2013. 12(24):3804–3816. doi: 10.4161/cc.26671
- Wong RW, Blobel G, Coutavas E. Rae1 interaction with NuMA is required for bipolar spindle formation. Proc Natl Acad Sci U S A. 2006;103(52):19783–19787. doi: 10.1073/pnas.0609582104
- Kimura M, Yoshioka T, Saio M, et al. Mitotic catastrophe and cell death induced by depletion of centrosomal proteins. Cell Death Dis. 2013;4(4):e603. doi: 10.1038/cddis.2013.108
- Ha GH, Kim H-S, Lee CG, et al. Mitotic catastrophe is the predominant response to histone acetyltransferase depletion. Cell Death Differ. 2009;16(3):483–497. doi: 10.1038/cdd.2008.182
- Holland AJ, Cleveland DW, Losing balance: the origin and impact of aneuploidy in cancer. EMBO Rep, 2012. 13(6):501–514. doi: 10.1038/embor.2012.55
- Nam HJ, van Deursen JM. Cyclin B2 and p53 control proper timing of centrosome separation. Nat Cell Biol. 2014;16(6):538–549. doi: 10.1038/ncb2952
- Zou Z, Yuan Z, Zhang Q, et al. Aurora kinase a inhibition-induced autophagy triggers drug resistance in breast cancer cells. Autophagy. 2012;8(12):1798–1810. doi: 10.4161/auto.22110
- de Carcer G, Malumbres M. A centrosomal route for cancer genome instability. Nat Cell Biol. 2014;16(6):504–506. doi: 10.1038/ncb2978