ABSTRACT
Lung cancer is the leading cause of cancer-related death worldwide. Indeed, only small improvements in survival can be expected with the use of conventional therapies. This observation highlights the need for new treatment options. Evening primrose oil (EPO) contains a wide range of phytoconstituents with potent tumor suppressor activities. Here, we evaluated the potential for EPO to have an additive cytotoxic effect with cisplatin in A549 lung cancer cells. Interestingly, the MTT assay revealed a significant cytotoxic effect of EPO against A549 cells. EPO-induced apoptosis was evidenced by an increase in PI-positive cells analyzed by FACS. Moreover, a significant increase in pro-apoptotic proteins (BAX, cytochrome c, c-caspase 3, and c-PARP) was observed. In addition, a significant upregulation of p53 and PUMA was reported upon treatment with EPO against cells. Cisplatin was also observed to induce apoptosis by upregulating pro-apoptotic proteins. Interestingly, the co-treatment of both cisplatin and EPO showed the highest apoptotic percentage of A549 cells with increased apoptotic bodies compared to control cells and single treatment with cisplatin. This suggests that EPO exhibited anticancer properties in A549 cells and enhanced the effectiveness of cisplatin. This proposes EPO supplementation as a potential adjuvant and sensitizer in lung cancer therapy.
Introduction
Lung cancer is the leading cause of cancer-related death globally. The most prevalent lung cancer is non-small cell lung cancer (NSCLC) [Citation1]. Metastatic NSCLC is currently an incurable disease for which standard chemotherapy provides only a minor improvement in overall survival. In addition, less than 30% of patients with advanced-stage NSCLC show a response to platinum-based chemotherapy like cisplatin [Citation2]. Moreover, the combination therapy consisting of cisplatin and pemetrexed did not enhance the median overall survival [Citation3]. Indeed, discovery of new drugs and adjuvant supplementation is necessary to reduce mortality and improve therapeutic outcomes. Research on natural derivatives and herbal medications to be used in cancer therapy is of current interest and has recently gained attention [Citation4]. It is well reported that several naturally occurring herbal medications have the ability to influence different molecular pathways that stop the progression of the cancer cell cycle and trigger apoptosis [Citation4].
The Oenothera biennis, a medicinal plant with the commercial name of evening primrose ‘EPO’ grows in different parts of the world. The extracted oil contains various phytoconstituents including phenolic acids, gamma-linolenic acid, flavonoids, sterols, and chalcone [Citation5]. Recently, clinical trials have examined and reported the effectiveness of EPO in the treatment of a number of conditions, including diabetic neuropathy and rheumatoid arthritis [Citation6,Citation7]. EPO is proposed to achieve anticancer effects through regulation of the hypoxic microenvironment, anti-inflammatory mechanisms and eventually mitochondria-mediated cell death [Citation8]. Up to now, there have been no preclinical findings regarding the potential anticancer effects of EPO in lung cancer. In this study, we have evaluated the anticancer potential of EPO in combination with cisplatin against A549 lung cancer cell and have investigated the underlying mechanism of action.
Materials and methods
Culture of A549 cells and reagents
A549 lung cancer cell line was provided from the ATCC collection (ATCC, Rockville, MD, USA). The cells were cultured in RPMI that was enhanced with 10% fetal bovine serum (FBS) and 50 μg/ml gentamycin. The cells were subcultured two or three times a week and kept at 37°C in a humidified environment with 5% CO2. Sigma (St. Louis, USA) provided cisplatin, dimethyl sulfoxide (DMSO), FBS, trypan blue, and MTT. EPO was provided by Jilin Baili Biotechnology Co., Ltd. This study was approved by the ethical committee of the medical research institute, Alexandria University (Ref. No. 719PB11).
MTT assay for cell viability
Three duplicates of ascending concentrations of cisplatin and EPO were applied to 5 × 103 cells/well in 96-well plate. The MTT test was used to calculate the number of viable cells [Citation9] during a 24-h incubation period. After treatment, the 96-well plate’s medium was taken out and replaced with 100 µl of new culture medium. Each well, including the untreated controls, then received 10 µl of the 12 mM MTT stock solution. After that, plates were incubated for four hours at 37°C. After removing 85 µl aliquot of the media from each well, 50 µl of DMSO was added. After that, cell viability measured using a microplate reader at 590 nm (SunRise, TECAN, Inc., USA). The percentage of viability was then computed as (ODt/ODc) ×100%.
Apoptosis assay
The Annexin V-FITC assay was used for apoptosis analysis [Citation10]. 1 × 106 A549 cells/sample were cultivated and then treated with cisplatin and EPO. The collected cells were washed twice with PBS. Cells are re-suspended in 400 µl PBS +100 µl binding buffer (50 mM HEPES, 700 mM NaCl, 12.5 mM CaCl2, pH 7.4) with 2 µl of Annexin (1 mg/ml) (Becton Dickinson BD, Germany). Following a wash, the cells were re-suspended in 400 µl PBS +100 µl binding buffer with 2 µl of propidium iodide (PI) (1 mg/ml) (Invitrogen, Life Technologies, Germany). Eventually, the cells were analyzed using the flow cytometer BD FACS.
Electron microscopic investigations
5×105 A549 cells were seeded in 6 well plate then treated with cisplatin alone or EPO or both. At the end of the treatment, cells were first fixed in 2.5% glutaraldehyde, then post-fixed in 1% osmium tetroxide (OsO4) and finally dehydrated in an escalating sequence of ethyl alcohol, commencing at 50% alcohol and concluding with three changes of 100% alcohol. The samples were twice rinsed in phosphate buffer pH 7.4. Alcohol residue was eliminated using propylene oxide before being imbedded in an epon medium. Joel electron microscopy was used to study the ultrathin, 50–60 nm thick sections after they were cut using an ultramicrotome, picked onto copper grids and stained with lead citrate and uranyl acetate. The samples were examined using a Hitachi H-7500 transmission electron microscope (Hitachi High Technologies, Tokyo, Japan) [Citation11].
Protein expression analysis
5×105 A549 cells/well were seeded in 6 well plate and treated with cisplatin alone or EPO alone or both. At the end of the treatment, cells were washed twice with cold PBS and cell pellets were collected by centrifugation. Cells were solubilized in lysis buffer and incubated on ice for 30 min. then centrifuged to remove insoluble material. Aliquots of supernatant were transferred to new tubes. The cell lysate was stored at −80°C until used to assay the quantitative levels of c-caspase, BAX, c-PARP and cytochrome c using specific enzyme-linked immunosorbent assay (ELISA) [Citation12] according to the manufacturer’s instructions (Lifespan Biosciences; MyBioSource, USA; eBioscience, USA).
Gene expression analysis
Total RNA (500 ng) from 5 × 105 A549 cells/sample was purified using RNA extraction kit. cDNA was synthesized and was then diluted 10 times. Using TOPreal SYBR Green qPCR PreMIX, a preassembled liquid mixture including nTaq-HOT (Cat. P725), its reaction buffer, dNTPs, stabilizing agents, ROX reference dye, and SYBR Green I dye in two-fold (2×) concentrations, relative quantification of P53 and PUMA expression was carried out. GAPDH was used as a housekeeping gene. By using TOPreal SYBR Green qPCR PreMIX, primer dimer formation and nonspecific amplification are reduced, and amplification specificity is increased. In this investigation, the primer sequences listed below were utilized [Citation13].
Statistical analysis
All data were expressed as mean ± standard deviation (SD) from three independent repeats of the experiment. Statistical comparisons between two experimental groups were determined using a two-tailed Student’s t-test (Excel 2013 Microsoft, USA). Results were considered statistically significant when the p-value < 0.05.
Results
Effect of EPO and cisplatin on A549 cell viability
EPO effect in combination with cisplatin treatment on lung cancer cell proliferation was evaluated using MTT as a cytotoxicity assay [Citation9]. First, the cells were treated with different doses of cisplatin (30–450 μM), and vehicle treated cells were used as control (). On the other hand, A549 cells were treated with increasing doses of EPO (50–700 mg/ml) (). After 24 h, cytotoxic effect of cisplatin and EPO was determined. The results showed a significant effect on lung cancer cell viability in a dose-dependent manner when treated with cisplatin or EPO (). From the MTT data, the IC50 values for cisplatin and EPO in A549 cells were 233.6 ± 8.32 μM and 320.09 ± 10.74 mg/ml, respectively.
Figure 1. The effect of cisplatin and EPO on A549 cell viability. (a) MTT assay for A549 cells treated with ascending concentrations of cisplatin. (b) MTT assay for A549 cells treated with ascending concentrations of EPO. The data represents the mean ± SD of three independent experiments.
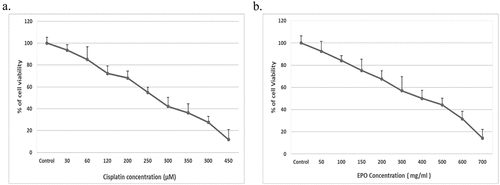
On the basis of the anti-tumor potential of EPO, we further examined whether EPO could enhance cisplatin sensitivity by MTT assay. A549 cells were treated with cisplatin (120 μM) and EPO (200 μg/ml), then the cytotoxic effect was assessed. Cisplatin showed slight inhibition of A549 cell growth, while cell viability was evidently suppressed after the addition of EPO (). The data showed a synergistic interaction between EPO and cisplatin for the proliferation suppression of A549 cells ().
Figure 2. Synergetic effect of EPO on cisplatin action on A549 cells … . EPO improves cisplatin effect on A549 cells. (a) the cell viability of A549 cells treated with cisplatin and EPO, alone or in combination. (*) represents significance compared to the untreated control group. (#) represents significance compared to the cisplatin treated group. Significant at p *< 0.05, **< 0.01, ***< 0.001. (b) Morphological changes of A549 cells after treatment. A549 cells were treated with cisplatin (120 μM) and/or EPO (200 μg/ml) for 24 h and the morphological changes examined by the inverted microscope (200X).
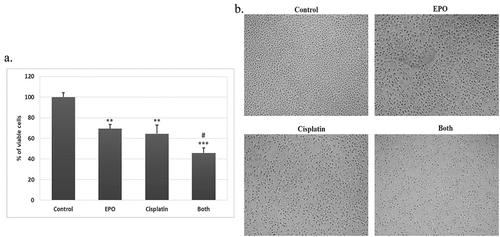
A number of morphological changes were observed in A549 cells following the treatment of cisplatin alone, or EPO alone or in combination as the morphology of the cells was highly altered after co-treatment with cisplatin and EPO. A549 cells in the control group show normal morphological features of the cells (). Treatment with cisplatin for 24 h clearly affects the morphology of the cells as it became rounder and smaller with the observed floating cells (). Interestingly, most of the cells were adhesive in EPO treated group with slight changes in morphology as the cytoplasm became rougher (). In the co-treatment group, the cells had the highest morphological changes; the number of cells was significantly reduced, the original shape was gone as the cells had irregular cell walls ().
Effect of EPO and cisplatin on A549 cell apoptosis
To further investigate the influence of treatment with EPO on lung cancer cell death, flow cytometry analysis of cell apoptosis was performed after 48 h of treatment with cisplatin alone or EPO alone or co-treatment. Interestingly, the concomitant treatment of cisplatin and EPO resulted in a marked increase of A549 cell death compared with the treated control (). The percentages of cell death were significantly increased from 7.56% in control cells to 74.81% in co-treatment cells with both cisplatin and EPO, while in cisplatin alone or EPO alone the percentage of cell death was 17.79% and 18.11%, respectively (). A clearly higher ratio of apoptotic cells () after co-treatment of both cisplatin and EPO was reported. As in , the percentage of apoptotic (early and late) cells after exposed to cisplatin and EPO was 73.62% as compared to 4.33% in control, while 11.09% and 5.75% were detected after treatment with cisplatin alone or EPO alone, respectively.
Figure 3. Effect of cisplatin and EPO on A549 cell apoptosis. (a) the cell death of A549 cells treated with cisplatin alone, EPO alone and in combination. (b) % of gated cells in each quarter (normal, necrotic, early and late apoptotic cells) after treatment with cisplatin alone, EPO alone and in combination.
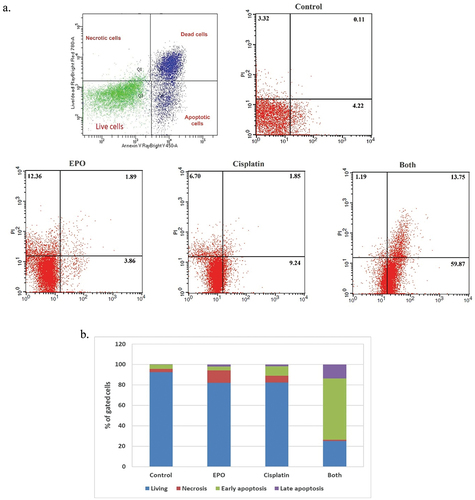
Effect of EPO and cisplatin on A549 cell ultrastructure
To determine the cytotoxic effects on the inhibition of cell growth, we examined morphological changes in A549 lung cancer cells treated with cisplatin and EPO (). Ultrastructural analysis of the control A549 lung cancer cells showed an intact cell membrane and a large, centralized nucleus with clearly visible nucleoli surrounded by a regular nuclear membrane (). Normal distribution of heterochromatin and cellular organelles in the cytoplasm, including pleomorphic mitochondria, endoplasmic reticulum, and cytoskeleton were observed in control cells. A549 lung cancer cells treated with cisplatin had an irregular plasma membrane and deeply invaginated nucleus, with chromatin condensation at the periphery of the nuclear membrane. Rod-shaped mitochondria, dilated endoplasmic reticulum, high incidence of autophagosomes, and Golgi apparatus fragmentation were reported after cisplatin treatment (). Treatment with EPO revealed an invaginated plasma membrane, oval pyknotic nucleus, swollen destructive mitochondria, and heavily vacuolized cytoplasm in the presence of autophagosomes and autolysosomes. Moreover, an increase in the number of apoptotic bodies and lipid droplets was observed (). Combined cisplatin and EPO treatment resulted in severe cell damage with ruptured cell membranes, complete cytoplasmic dissolution and frequent apoptotic bodies ().
Figure 4. Transmission Electron Microscopy (TEM) micrographs of A549 lung cancer cell illustrating ultrastructural features of control cell (a and b) showing intact cell membrane and centric large nucleus (N) with obvious nucleoli (NU) surrounded by regular nuclear membrane. Normal distribution of heterochromatin (H) and cellular organelles in cytoplasm including pleomorphic mitochondria (M), endoplasmic reticulum (ER), and cytoskeleton (c)are noticed. Cisplatin group (c-e) showing irregular plasma membrane, deeply invaginated nucleus (dash-arrow) with chromatin condensation (H) at the periphery of the nuclear membrane. Rod-shaped mitochondria (M) dilated endoplasmic reticulum (ER), high incidence of autophagosome (AP) and Golgi apparatus fragmentation (G) are observed. Primrose oil group (f and g) showing invaginated plasma membrane (↑), oval pyknotic nucleus (N), swollen destructive mitochondria (M), and heavily vacuolized cytoplasm (V) with the presence of autophagosomes (AP) and autolysosome (AL). Increased number of apoptotic bodied (*) and lipid droplets (L) are detected. Cisplatin and primrose oil group (h and i) showing severe cell damage with ruptured cell membrane (↑), complete cytoplasmic dissolution (#) and frequent apoptotic bodies (*).
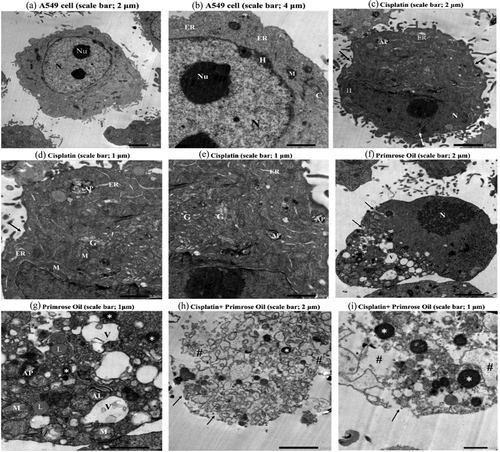
Effect of cisplatin and EPO on apoptotic signalling pathway
Based on the preceding results, we further investigated the role of apoptotic proteins (caspase3, Bax, PARP and cytochrome c) in cisplatin plus EPO co-treatment group. The ELISA results showed a significant (p < 0.05) upregulation in the protein levels of cleaved-caspase 3, Bax, cleaved-PARP and cytochrome c proteins in all treated groups when compared with the control. In addition, the co-treatment group showed highly significant changes as compared with single treated groups (). The results of the detected apoptosis activity by FACS were consistent with related protein expression levels.
Figure 5. The effect of cisplatin and EPO treatment on the protein level of proapoptotic factors: (a) c-caspase3, (b) Bax, (c) cytochrome c and (d) c-PARP protein levels. The data represents at least three independent experiments as mean ± SD. (*) represents significance compared to the untreated control group. (#) represents significance compared to the cisplatin treated group. Significant at p *< 0.05, **< 0.01, ***< 0.001.
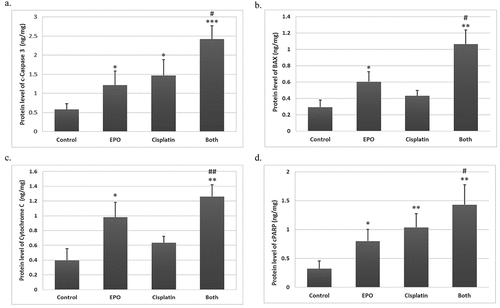
p53 is playing a key role in the response to chemotherapeutic agents to induce cell apoptosis [Citation14]. P53 as a transcription factor associated with tumor suppressor action regulates a number of genes that co-ordinately force cells to stop division via trans-activation of p21Waf1/Cip1 (p21). Stopping cell division triggers activation of DNA repair mechanisms that eventually end into apoptosis via trans-activation of PUMA if the DNA damage cannot be repaired [Citation15]. Interestingly, the gene expression levels of p53 increased following exposure to cisplatin alone or EPO alone (). A subsequent increase in PUMA mRNA levels as compared to control was reported. The change in these gene expressions was highly significantly increased as compared to single treatment ().
Figure 6. The effect of cisplatin and EPO treatment on the expression of tumour suppressor genes: (a) the relative mRNA abundance of p53 and (b) PUMA were monitored by using qRT-PCR. GAPDH was used as housekeeping gene. The graph shows mean values and experimental variation of three independent experiments. (*) represents significance compared to the untreated control group. (#) represents significance compared to the cisplatin treated group. Significant at p *< 0.05, **< 0.01, ***< 0.001.
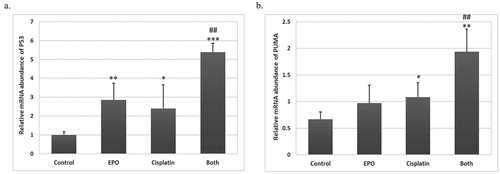
Discussion
Cisplatin is a chemotherapeutic agent with obvious clinical effects in patients with lung cancer. However, there is a limitation and challenge to use it as the patients by time become refractory to the drugs [Citation3]. Recently, a growing body of research has shed light on medicinal plants that have been extensively studied to improve the efficacy of chemotherapeutic agents [Citation16]. The combinatorial cytotoxic effect of medicinal plants and chemotherapeutic agents is evolved as a promising strategy to enhance the efficacy of cancer treatment. The medicinal plants are generally safe and might have a synergistic therapeutic impact to decrease the toxicity and dose of chemotherapeutic agents [Citation17]. Oenothera biennis, evening primrose oil, emerged as a promising rich source of γ-linolenic acid with prominent therapeutic effect in breast and pancreatic cancer [Citation18,Citation19]. This study aimed to determine the anticancer properties of EPO and to evaluate the combinatorial cytotoxic effect of cisplatin in lung cancer A549 cell line.
The results of the current study showed a significant cytotoxic effect of cisplatin and EPO on A549 cell line. Interestingly, EPO was observed to induce cell death in A549 cells. This was obvious by the increase in PI-positive cells observed in flow cytometry analysis. It was demonstrated that EPO induces apoptosis in Ehrlich ascites tumor cells [Citation20], pancreatic ductal adenocarcinoma cell lines [Citation19] and cell death in HT-29 colorectal cancer cells [Citation21]. Moreover, the results clearly showed that the cytotoxic effect of cisplatin was dose-dependent. Accordingly, we investigated the EPO effect in combination with cisplatin as this might be a promising strategy to potentiate its effect. Interestingly, the current results showed a synergistic effect of EPO with cisplatin in the treatment of A549 lung cancer cells.
Cell apoptosis analysis showed that the highest apoptotic percentage of A549 cells was observed in combination-treated group, compared to control and other single treated groups. Apoptosis was demonstrated by increase in PI positive cells and upregulation of pro-apoptotic protein levels such as BAX and cytochrome c. The activity of caspase-3 that is playing a pivotal role in apoptosis, also increased after cisplatin treatment. Inactivation of PARP was also observed after treatment. The changes in the expression of the previous apoptotic mediators were highly significant in combined EPO and cisplatin treated cells as compared to control or other single treatments. In line with our findings, it was reported that EPO exhibited anticancer activity [Citation22]. EPO was reported to potentiate tamoxifen’s anticancer activity against breast cancer by inducing apoptosis [Citation18]. In addition, Zeppa et al. showed that EPO enhanced paclitaxel efficacy and reduced PDAC cell viability [Citation19].
It was reported that linoleic acid in EPO is converted to γ-Linolenic acid in the company of ∆-6 desaturase and might exhibit antitumor activity by altering cyclooxygenase (COX) metabolism. As a consequence, the produced prostaglandin E1 has been reported to disrupting cell-cycle progression. This triggers reactive oxygen species and caspase activation, cytochrome c release, and at the end induction of apoptosis occurred [Citation23].
Cytochrome c is located in the mitochondrial membrane, and various proapoptotic stimuli triggers the release of cytochrome c. that mediates the allosteric activation of apoptosis-protease activating factor 1 (APAF-1). This factor is required for the proteolytic maturation of caspases. Caspase-3 is one of the important molecules in cellular suicide that is activated by the upstream caspase-8 and caspase-9 [Citation24]. Caspases pledge cell death by cleaving and activating effector caspases that eventually drive the process of apoptosis [Citation25]. PARP, Poly(ADP-ribose) polymerase, is a cellular substrate of caspases. Cleavage of PARP by caspases is a hallmark of apoptosis process [Citation26]. It was reported that inactivation of PARP has prevented the depletion of NAD, a PARP substrate and ATP. This is thought to be required for later events in apoptosis. In line with this finding, the expression of cytochrome-c, BAX, cleaved caspase-3 and cleaved PARP in our study were highly significantly upregulated upon EPO and cisplatin co-treatment compared to control cells, suggesting involvement of the intrinsic apoptotic pathway.
Interestingly, cisplatin treatment results in oxidative stress that induces cellular stress responses often results in cell death to remove the stressed cell from the organism. The p53 acts as mediator of the apoptotic stress response [Citation14]. It is a transcription factor that regulates cascades of genes that control the suppression of tumors. For instant, p53 activates transcription of p53-upregulated modulator of apoptosis (PUMA). It is a member of the Bcl-2 protein family and after its activation, PUMA interacts with all the anti-apoptotic Bcl-2 family members, resulting in Bax release. Eventually, Bax induces apoptosis in the mitochondria [Citation27]. Mitochondrial translocation of BAX is a critical step in mitochondrial-intrinsic apoptotic pathway [Citation27]. This finding validated by ultrastructural morphological analysis as treatment with EPO and cisplatin revealed an invaginated plasma membrane, oval pyknotic nucleus, and swollen destructive mitochondria with observed increase in apoptotic bodies in case of combined treatment of both cisplatin and EPO. The ultrastructure finding of this study was in line with previous reports that documented the ultrastructural morphological signs of apoptosis, including blebbing of cell membrane and chromatin condensation at the periphery of the nuclear membrane, as well as the development of apoptotic bodies and pyknotic nuclei [Citation28,Citation29]. From the current results, it is suggested that the activation of p53 upon EPO treatment results in induction of PUMA that causes BAX release, which is highly significant in EPO and cisplatin co-treated cells as compared to control and other treated groups. This clearly indicated that the occurred apoptosis resulting in mitochondrial dependent cell death.
Conclusion
This study demonstrated that EPO exhibited anticancer properties in A549 cells and enhanced the cytotoxic effect of cisplatin. This effect was most likely mediated by EPO-induced apoptosis, involving the upregulation of p53 and PUMA expression. This upregulation might trigger the caspase activation cascade, ultimately activating the intrinsic apoptotic pathway. Furthermore, in vivo studies would be crucial to confirm this synergistic effect in a whole organism setting and identify the optimal window for EPO administration to achieve sensitization.
Disclosure statement
No potential conflict of interest was reported by the author(s).
References
- Schabath MB, Cote ML. Cancer progress and priorities: lung cancer. Cancer Epidemiol Biomarkers Prev. 2019;28(10):1563–1579. doi: 10.1158/1055-9965.EPI-19-0221
- Miyawaki E, Kenmotsu H, Shintani Y, et al. Efficacy of platinum agents for stage III non-small-cell lung cancer following platinum-based chemoradiotherapy: a retrospective study. BMC Cancer. 2022;22(1):342. doi: 10.1186/s12885-022-09441-3
- Ashrafizadeh M, Zarrabi A, Hushmandi K, et al. Lung cancer cells and their sensitivity/resistance to cisplatin chemotherapy: role of microRNAs and upstream mediators. Cell Signalling. 2021;78:109871. doi: 10.1016/j.cellsig.2020.109871
- Ali M, Wani SUD, Salahuddin M, et al. Recent advance of herbal medicines in cancer- a molecular approach. Heliyon. 2023;9(2):e13684. doi: 10.1016/j.heliyon.2023.e13684
- Farag MA, Reda A, Nabil M, et al. Evening primrose oil: a comprehensive review of its bioactives, extraction, analysis, oil quality, therapeutic merits, and safety. Food Funct. 2023;14(18):8049–8070. doi: 10.1039/d3fo01949g
- Hajiaghayi M, Dolati-Somarin AH, Gholizadeh F, et al. The efficacy of evening primrose in rheumatoid arthritis: a systematic review. Res J Pharmacogn. 2024;11(2):71–79. doi: 10.22127/RJP.2023.414090.2212
- Prado MB Jr, Adiao KJB. Ranking alpha lipoic acid and gamma linolenic acid in terms of efficacy and safety in the management of adults with diabetic peripheral neuropathy: a systematic review and network meta-analysis. Can J Diabetes. 2024;29:S1499–2671(24)00023–6. doi: 10.1016/j.jcjd.2024.01.007
- Timoszuk M, Bielawska K, Skrzydlewska E. Evening primrose (Oenothera biennis) biological activity dependent on chemical composition. Antioxidants (Basel). 2018;7(8):108. doi: 10.3390/antiox7080108
- Buranaamnuay K. The MTT assay application to measure the viability of spermatozoa: a variety of the assay protocols. Open Vet J. 2021;11(2):251–269. doi: 10.5455/OVJ.2021.v11.i2.9
- Crowley LC, Marfell BJ, Scott AP, et al. Quantitation of apoptosis and necrosis by annexin V binding, propidium iodide uptake, and flow cytometry. Cold Spring Harb Protoc. 2016;2016(11):2016. doi: 10.1101/pdb.prot087288 PMID: 27803250.
- Tizro P, Choi C, Khanlou N. Sample preparation for transmission electron microscopy. Methods Mol Biol. 2019;1897:417–424. doi: 10.1007/978-1-4939-8935-5_33
- Hornbeck PV. Enzyme-linked immunosorbent assays. Curr Protoc Immunol. 2015;110(1):2.1.1–23. doi: 10.1002/0471142735.im0201s110
- Singh C, Roy-Chowdhuri S. Quantitative real-time PCR: recent advances. Methods Mol Biol. 2016;1392:161–176. doi: 10.1007/978-1-4939-3360-0_15
- Wang J, Thomas HR, Li Z, et al. Puma, noxa, p53, and p63 differentially mediate stress pathway induced apoptosis. Cell Death Dis. 2021;12(7):659. doi: 10.1038/s41419-021-03902-6
- Rodriguez-Ramirez C, Nör JE. p53 and cell fate: sensitizing head and neck cancer stem cells to chemotherapy. Crit Rev Oncog. 2018;23(3–4):173–187. doi: 10.1615/CritRevOncog.2018027353
- Atanasov AG, Zotchev SB, Dirsch VM. International natural product sciences taskforce; supuran CT: natural products in drug discovery: advances and opportunities. Nat Rev Drug Discov. 2021;20:200–216. doi: 10.1038/s41573-020-00114-z
- Cheon C, Ko SG. Synergistic effects of natural products in combination with anticancer agents in prostate cancer: a scoping review. Front Pharmacol. 2022;13:963317. doi: 10.3389/fphar.2022.963317
- Abd-Alhaseeb MM, Massoud SM, Elsayed F, et al. Evening primrose oil enhances Tamoxifen’s anticancer activity against breast cancer cells by inducing apoptosis, inhibiting angiogenesis, and arresting the cell cycle. Molecules. 2022;27(8):2391. doi: 10.3390/molecules27082391
- Zeppa L, Aguzzi C, Versari G, et al. Evening primrose oil improves chemotherapeutic effects in human pancreatic ductal adenocarcinoma cell lines—a preclinical study. Pharmaceuticals (Basel). 2022;15(4):466. doi: 10.3390/ph15040466
- Arimura T, Kojima-Yuasa A, Suzuki M, et al. Caspase-independent apoptosis induced by evening primrose extract in Ehrlich ascites tumor cells. Cancer Lett. 2003;201:9–16. doi: 10.1016/S0304-3835(03)00440-3
- Montserrat-de la Paz S, Fernández-Arche MA, Bermúdez B, et al. The sterols isolated from evening primrose oil inhibit human colon adenocarcinoma cell proliferation and induce cell cycle arrest through upregulation of LXR. J Funct Foods. 2015;12:64–69. doi: 10.1016/j.jff.2014.11.004
- Lewandowska U, Owczarek K, Szewczyk K, et al. Differentiated impact of procyanidins from evening primrose on human breast cancer cells. Cent Eur J Biol. 2014;9(6):647–658. doi: 10.2478/s11535-014-0299-9
- Miyake JA, Gomes RN, Colquhoun A. Gamma-linolenic acid alters migration, proliferation and apoptosis in human and rat glioblastoma cells. Prostaglandins Other Lipid Mediat. 2020;150:106452. doi: 10.1016/j.prostaglandins.2020.106452
- Hussar P. Apoptosis regulators Bcl-2 and caspase-3. Encyclopedia. 2022;2(4):1624–1636. doi: 10.3390/encyclopedia2040111
- Bertheloot D, Latz E, Franklin BS. Necroptosis, pyroptosis and apoptosis: an intricate game of cell death. Cell Mol Immunol. 2021;18(5):1106–1121. doi: 10.1038/s41423-020-00630-3
- Chaitanya GV, Alexander JS, Babu PP. PARP-1 cleavage fragments: signatures of cell-death proteases in neurodegeneration. Cell Commun Signal. 2010;8(1):31. doi: 10.1186/1478-811X-8-31
- Zhang Y, Xing D, Liu L, et al. PUMA promotes Bax translocation by both directly interacting with Bax and by competitive binding to Bcl-X L during UV-induced apoptosis. Mol Biol Cell. 2009;20(13):3077–3087. doi: 10.1091/mbc.e08-11-1109
- Zhang Y, Chen X, Gueydan C, et al. Plasma membrane changes during programmed cell deaths. Cell Res. 2018;28(1):9–21. doi: 10.1038/cr.2017.133
- Xu X, Lai Y, Hua ZC. Apoptosis and apoptotic body: disease message and therapeutic target potentials. Biosci Rep. 2019;39(1):18–39. doi: 10.1042/BSR20180992