ABSTRACT
Despite recent advances in our understanding of epigenetic regulation of central nervous system development, little is known regarding the effects of epigenetic dysregulation on neurogenesis and brain function in adulthood. In the present study, we show that prenatal deletion of DNA methyltransferase 1 (Dnmt1) in neural stem cells results in impaired neurogenesis as well as increases in inflammatory features (e.g., elevated glial fibrillary acidic protein [GFAP] expression in astrocytes and increased numbers of microglia) in the adult mouse brain. Moreover, these mice exhibited anxiety-like behavior during an open-field test. These findings suggest that Dnmt1 plays a critical role in regulating neurogenesis and behavior in the developing brain and into adulthood.
Adult neurogenesis occurs in 2 restricted brain regions: the subventricular zone (SVZ) of the lateral ventricles and the dentate gyrus (DG) in the hippocampus, in which neural stem cells (NSCs) are maintained throughout life.Citation1-4 New neurons produced in these regions are functionally integrated into the neural circuitry, and research has indicated that these neurons may contribute to a wide variety of brain functions.Citation5,6 Indeed, neurogenesis in the DG has been known to participate in learning and memory, whereas newly generated neurons in the rodent SVZ migrate into the olfactory bulb and contribute to olfactory-related behaviors. Furthermore, accumulating evidence has implicated the dysregulation of adult neurogenesis in several diseases, including Alzheimer's disease, epilepsy, and various psychological disorders.Citation7-10 Recently, research has revealed that exposure to stress during prenatal and neonatal stages of development reduces neurogenesis in the adult stage, and that these decreases are strongly associated with the development of psychological disorders.Citation11 These findings suggest that early life experiences strongly affect both neurogenesis and brain function in adults, though the mechanisms underlying this association remain unknown.
NSCs in the adult brain are maintained in a specific microenvironment or “niche,” which is comprised of those cells and structures that surround and support the NSCs, such as astrocytes, blood vessels, and microglia.Citation12 Extracellular cues released from these niche cells play a key role in controlling the proliferation and determination of NSC fate under both normal physiological and pathological conditions. For example, activation of microglia under epileptic conditions results in the subsequent release of tumor necrosis factor α (TNF-α), which attenuates the abnormal proliferation of NSCs and aberrant neurogenesis induced by seizure activity.Citation13 In addition to the contributions of the niche, processes of epigenetic regulation—such as DNA methylation and histone modification—have been observed to play key roles in regulating adult neurogenesis.Citation14 Inhibition of histone deacetylase (HDAC) activity decreases proliferation of NSCs,Citation15 while deletion of DNA methyltransferase 1 (Dnmt1), the gene responsible for encoding the enzyme that maintains DNA methylation in NSCs, impairs the survival of newly generated neurons in the adult DG.Citation16
During development of the central nervous system (CNS), epigenetic regulation plays a pivotal role in the proliferation of embryonic NSCs as well as in the determination of NSC fate.Citation17 Accumulating evidence has also indicated that epigenetic regulation provides significant contributions to both cortical and DG development.Citation18,19 Previously, we observed that prenatal exposure to valproic acid (VPA) impairs neurogenesis and hippocampus-dependent learning and memory even into adulthood.Citation20 Furthermore, we have also reported that prenatal deletion of Dnmt1 in NSCs impairs DG development by attenuating neurogenesis in the DG.Citation19 The results of these studies have raised the possibility that appropriate epigenetic regulation during development of the prenatal brain is critical for the establishment and maintenance of proper niche environments and NSCs in adulthood. Therefore, in the present study, we aimed to investigate the role of Dnmt1 in DG neurogenesis by examining the behavior of adult mice in which the Dnmt1 gene had been deleted during a late prenatal stage, and by assessing the effects of this mutation on brain structure.
The deletion of Dnmt1 in NSCs at early gestational stages leads to neonatal lethality due to respiratory failure.Citation21 To avoid this complication, we crossed Dnmt1flox/flox mice with Nestin-CreERT2 mice and administered tamoxifen to the mice at embryonic day (E) 15, achieving tamoxifen-inducible deletion of Dnmt1 in NSCs (cKO mice, ). After tamoxifen treatment at E15, the offspring were analyzed at an adult stage (8-week old) (). Mice that had undergone prenatal deletion of Dnmt1 were allowed to mature until adulthood and displayed no obvious defects in body size, brain structure, or brain weight.Citation19 Previously, we reported that prenatal deletion of Dnmt1 impairs adult neurogenesis in the DG.Citation19 However, the influence of such deletion on adult neurogenesis in the SVZ remains to be examined. Therefore, in the present study, we further investigated the effects of prenatal Dnmt1 deletion on adult neurogenesis in both neurogenic niches, placing particular emphasis on adult neurogenesis in the SVZ. Consistent with our previous findings, immunostaining of sagittal brain sections against the immature neuron marker doublecortin (DCX) revealed that cKO mice exhibited significant reductions in the number of DCX+ cells as well as decreased granule layer volume in the DG (). Furthermore, we observed that DCX+ newborn neurons in the DG of cKO mice exhibited abnormal morphology and arrangement relative to controls (). Similarly, we also observed marked reductions in the number of newborn neurons in the SVZ of cKO mice (). However, unlike DG, the morphological difference of DCX+ cells between control and cKO mice was not observed in the SVZ.
Figure 1. Prenatal deletion of Dnmt1 impairs cell proliferation and neurogenesis in the adult brain. (A) Diagram of the Dnmt1 conditional knockout strategy in NSCs. Dnmt1flox/flox mice were crossed with Nestin-CreERT2 mice, yielding progeny in which tamoxifen administration results in the deletion of Dnmt1 (exons 4 and 5) in Nestin-expressing NSCs. (B) Experimental scheme for assessing the effect of Dnmt1 deletion on cortical development and neurogenesis. Pregnant mice were injected with tamoxifen (2 mg) at E15 to induce deletion of Dnmt1 in NSCs, and the brains of the offspring were analyzed at 8-week old. (C) Representative immunofluorescence images of DCX (red) in sagittal adult (8-week old) brain sections from control and cKO mice. White open rectangles indicating the RMS and DG are shown in higher magnification images, respectively. (D) DCX immunostaining images (white) of representative newborn neurons in the DG of control and cKO mice. (E) DCX immunostaining images (red) of representative SVZ neurons in coronal adult brain sections from control and cKO mice. White open rectangles in the left panel images are shown in higher magnification in the right images. (F) DCX+ cells in (E) were quantified along the lateral wall of the lateral ventricle (mm) (Control = 3, cKO = 3). (G) Ki67 immunostaining (green) images of representative SVZ neurons in coronal adult brain sections from control and cKO mice. The nucleus was stained with Hoechst (Blue). (H) Ki67+ cells in (G) were quantified along the lateral wall of the lateral ventricle (mm) (Control = 3, cKO = 3). Scale bars are indicated in each figure. Values represent mean ± SEM; *P < 0.05, ***P < 0.001. Student's t-test. NSC: neuronal stem cells; Dnmt1: DNA methyltransferase 1; DCX: doublecortin; RMS: rostral migratory stream; DG: dentate gyrus; SVZ: subventricular zone.
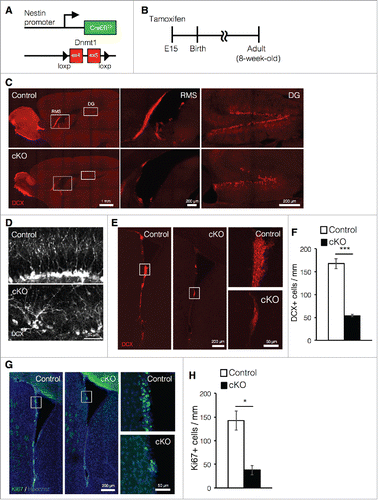
Newly generated neurons in the SVZ migrate into the olfactory bulb via the rostral migratory stream (RMS).Citation5 In cKO mice, we observed a drastic reduction in the number of DCX+ cells in both the RMS and olfactory bulb relative to controls. Ki67 is a typical marker for proliferating cells, including NSCs, transit-amplifying cells and neuroblasts,Citation23 and we also observed significant reductions in the number of Ki67+-proliferating cells in cKO mice relative to controls (). Taken together, these data indicate that prenatal deletion of Dnmt1 impairs neurogenesis in both the DG and SVZ in the adult brain.
In embryonic stages, Dnmt1 plays an essential role in preventing precocious astrocyte production in NSCs,Citation24-27 and deletion of Dnmt1 prior to the onset of astrocyte production in cortical development leads to increased expression of the astrocyte marker glial fibrillary acidic protein (GFAP) at postnatal week one.Citation24 In light of these findings, we then investigated whether loss of Dnmt1 during the late embryonic stage—by which time astrocyte production has already begun—also increases expression of GFAP in postnatal stages. In contrast to our results obtained regarding Dnmt1 deletion prior to the onset of astrocyte production, loss of Dnmt1 during late embryonic development did not significantly increase GFAP expression in the cortex, at least within the first week of postnatal development (). However, we observed significant increases in GFAP expression throughout the cortex of cKO mice in the adult stage (). Furthermore, such increases in GFAP expression were also observed in the DG and striatum near the SVZ in cKO mice (). We further revealed that differences in cortical GFAP expression between control and cKO mice could be observed as early as postnatal day (P) 21, becoming significant at P35 ().
Figure 2. Prenatal deletion of Dnmt1 increases expression of GFAP in astrocytes (A) Representative immunofluorescence images of GFAP (red) in the cortex of coronal brain sections from control and cKO mice at various time points. (B) GFAP immunostaining images (red, left panels) of representative cortical neurons in coronal adult brain sections from control and cKO mice. The nucleus was stained with Hoechst (gray, right panels). Ctx: cortex. (C) GFAP immunostaining images (red) of representative regions including the SVZ in coronal adult brain sections from control and cKO mice. (D) Representative GFAP immunofluorescence images (red) of cortical neurons in coronal brain sections from control and cKO mice at various time points. (E) GFAP (red) and S100β (green) immunostaining images of representative cortical astrocytes in coronal brain sections from adult control and cKO mice. (F) Representative image of staining for S100β (green) in the cortex of coronal brain sections from adult control and cKO mice. (G) Quantification of S100β+ cells in the cortex in (F). (Control = 3, cKO = 3). Scale bars are indicated in each figure. Values represent mean ± SEM; n.s > 0.05. Student's t-test. GFAP: glial fibrillary acidic protein.
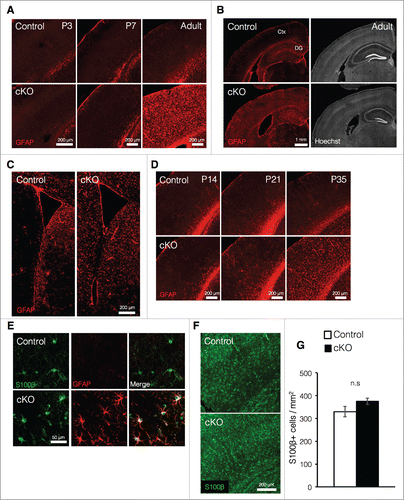
It is generally known that the cortex contains several subtypes of astrocytes, such as fibrous and protoplasmic astrocytes.Citation28 Fibrous astrocytes reside in white matter and express GFAP rather highly. On the other hand, protoplasmic astrocytes, which reside in the gray matter, exhibit relatively less GFAP expression than fibrous astrocytes. In control mice, GFAP expression in S100β+ (an astrocyte marker) cells was not clearly observed even in the adult cortex (). However, S100β+ cells in cKO mice exhibited dramatic increases in GFAP expression (). These observations suggest that elevated GFAP expression in cKO mice may not result from increased astrocyte production during development, but rather from upregulation of GFAP expression in protoplasmic astrocytes. The comparable numbers of S100β+ cells observed in cKO and control mice further support this possibility (). Collectively, these results suggest that prenatal deletion of Dnmt1 in NSCs does not increase astrogliogenesis but instead acts to upregulate the expression of GFAP in existing astrocytes as maturation progresses.
Increased expression of GFAP in astrocytes is one of the hallmark features of reactive astrocytes, which are produced in response to inflammation in the brain under pathologic conditions such as injury or disease, (e.g., autoimmune disease, multiple sclerosis, and Alzheimer's disease).Citation29-31 The inflammatory response is triggered by the activation of microglia, which releases pro-inflammatory factors and chemokines that recruit other microglia to the lesion site.Citation32 Microglia also proliferate in response to inflammation.Citation32,33 To address the possibility that prenatal deletion of Dnmt1 in NSCs produces inflammation in the brain, we measured the number of microglia in the cortex at P21 and P35 when increased GFAP expression was observed in cKO mice. A larger number of Iba1+ microglia were detected in cKO mouse cortices compared with those of control mice at both P21 and P35 (). Interestingly, microglia in the DG were most enriched at P14 and exhibited gradual reductions as maturation progressed in control mice (). Although similar reductions in DG IbaI+ cells were also observed in cKO mice throughout maturation, more enriched Iba1+ cells were observed in cKO mice than in control mice from P14 to P35 ().
Figure 3. Prenatal deletion of Dnmt1 increases microglia in the adult brain. (A) Representative Iba1 immunofluorescence images (green) in the cortex of coronal brain sections from control and cKO mice at various time points. (B) Quantification of IbaI+ cells in the cortex at P35. (Control = 3, cKO = 3). (C) Iba1 immunostaining image (green) of representative DG neurons in coronal brain sections from control and cKO mice at various time points. The nucleus was stained with Hoechst (blue). (D) Quantification of IbaI+ cells in the DG at P35. (Control = 3, cKO = 3) (E) Representative immunofluorescence images of Iba1 (green) and CD68 (red) in the DG of coronal adult brain sections from control and cKO mice at indicated time point. The nucleus was stained with Hoechst (blue). Scale bars are indicated in each figure. Values represent mean ± SEM; *P < 0.05, **P < 0.01. Student's t-test.
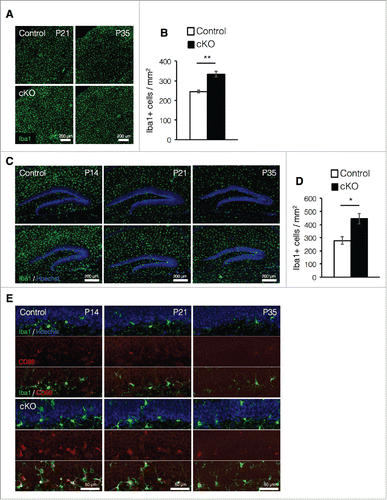
Microglia highly express CD68 in response to inflammation.Citation13 We then assessed microglial activation and observed that the number of activated microglia expressing CD68 increased in the DG of cKO mice relative to controls at each postnatal stage (). In control mice, activated microglia in the DG were observed at young postnatal stages (P14 and P21) but were rarely observed at P35. However, activated microglia were clearly detected in cKO mice even at P35. Taken together, these data suggest that prenatal deletion of Dnmt1 increases the number of activated microglia most likely due to the induction of inflammatory processes in the brain.
The cKO mice exhibited reduced adult neurogenesis and abnormal morphology of newborn neurons in the DG (). Although the precise functions of newly generated neurons in the adult DG remain controversial, increasing evidence suggests that reduced adult neurogenesis and neuroinflammation are implicated in disorders of mood regulation, such as depression and certain forms of anxiety.Citation11,34 Therefore, we investigated whether prenatal deletion of Dnmt1 could produce anxiety-like behavior in mice during an open-field test. Since mice fear open spaces, they avoid entering the center of the open field in the testing arena.Citation35 Thus, we recorded the time spent in the center area in order to assess the degree of anxiety. While the distance traveled was comparable between control and cKO mice (), cKO mice spent less time in the center area than controls (). These results suggest that Dnmt1 deletion in the prenatal stage induces anxiety-like behavior in adult mice.
Figure 4. Prenatal deletion of Dnmt1 induces anxiety-like behavior in adult offspring. (A-C) Exploratory behavior in the open-field test. Histograms show the total distance moved in the test (A) and proportion of time spent in the center area of arena (B) (Control = 6, cKO = 6). (C) Digital tracking of mice exposed to the open-field test. Representative traces of mice activity (gray) obtained from video tracking. Blue box indicates the center area of arena. Values represent mean ± SEM; n.s > 0.05, *P < 0.05. Student's t-test.
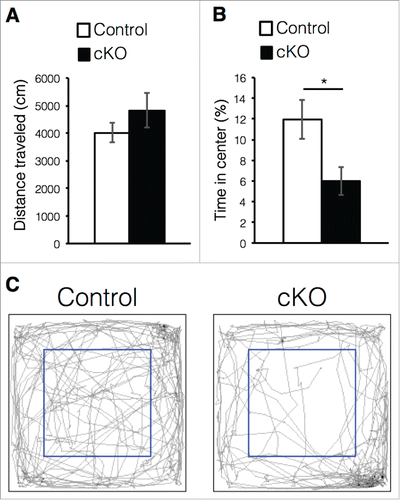
In this study, our data have indicated that prenatal deletion of Dnmt1 in NSCs compromises neurogenesis in both the SVZ and DG in the adult brain. We have previously reported that the enzyme DMNT1 is highly expressed in NSCs in both embryonic and adult brains.Citation19,36 However, in contrast to the results obtained for prenatal deletion, loss of Dnmt1 in NSCs during the adult stage impairs neither proliferation nor neuronal differentiation of NSCs.Citation16 Furthermore, upregulation of GFAP expression and activation of microglia in the adult brain were not observed in mice lacking Dnmt1 in NSCs (data not shown). Although the precise mechanisms underlying the observed differences between prenatal and adult Dnmt1 ablation remain unknown, it is possible that the effects of Dnmt1 deletion in NSCs on neurogenesis occur over a longer period of time, though further studies are required in order to more fully examine this point.
While DNMT1 is well known as a key enzyme for DNA methylation, recent functional analyses have suggested that Dnmt1 can regulate gene expression even without DNA methyltransferase activity.Citation36-38 Research has indicated that DNMT1 interacts with histone modification enzymes, such as HDAC, enhancer of zeste homolog 2 (Ezh2), and lysine-specific histone demethylase 1 (LSD1), suggesting that Dnmt1 is involved in modulating histone modification via interaction with these enzymes.Citation36 In fact, we previously reported that deletion of Dnmt1 in NSCs derived from postnatal DGs decreased repressive histone modification markers in the promoter region of a cell cycle inhibitor p57kip2 and increased its expression, impairing proliferation of NSCs.Citation19 In the present study, although we could not verify the exact mechanisms whereby prenatal deletion of Dnmt1 influences the behavior of NSCs in the adult mouse brain, our results may indicate that defects in NSCs induced by Dnmt1 deletion at the prenatal stage persist into adulthood and impair adult neurogenesis.
We have further shown in the present study that prenatal deletion of Dnmt1 induces inflammatory processes in the brain, including elevated expression of GFAP in cortical astrocytes and increased numbers of activated microglia in the cortex and DG. Deletion of Dnmt1 in NSCs during early stages of CNS development leads to hypomethylation in neurons as well as increased neuronal cell death,Citation21,24 suggesting that the inflammatory features observed in the brains of cKO mice may be due to the death of neurons that have differentiated from NSCs. Notably, the timeline during which we observed activated microglia in the DGs of cKO mice in the current study is consistent with the timing of the onset of increased apoptosis, as reported in our previous paper.Citation19 Thus, the observed increases in cell death may explain why microglia are activated in the DG of cKO mice. Researchers have reported that pro-inflammatory factors derived from activated microglia inhibit proliferation of NSCs and neurite outgrowth/branching.Citation39-41 In light of these findings, it is possible that the decreased neurogenesis and abnormal morphology of newborn neurons in the DG of cKO mice are attributable to pro-inflammatory factors released by activated microglia.
We also observed that prenatal deletion of Dnmt1 causes anxiety-like behavior in adult mice. Recent studies have shown that exposure to prenatal and neonatal stress—such as prenatal infection, forced exercise, or maternal separation—augment anxiety-like behavior in adult offspring,Citation11 yet the mechanisms underlying these effects remain elusive. It has become apparent that epigenetic modifications are susceptible to environmental changes.Citation42-44 Although a direct relationship between early-life stress and epigenetic modifications in the brain has yet to be verified, it is conceivable that dysregulation of epigenetic modifications may occur due to prenatal and neonatal stress exposure, resulting in depression and anxiety-like behavior. However, further studies are required in order to more fully elucidate the mechanisms underlying the development of psychological disorders in humans.
Methods
Animals
Generation of Nestin-CreERT2/Dnmt1-conditional mutant mice
For tamoxifen-inducible Cre-mediated Dnmt1 deletion in NSCs, Dnmt1flox/flox miceCitation21 were crossed with Nestin-CreERT2 transgenic mice in which CreERT2 was expressed under the Nestin promoter and enhancer.Citation22 Tamoxifen administration in Nestin-CreERT2/Dnmt1-conditional mutant mice (cKO) inactivates Dnmt1 via deletion of exons 4 and 5 of Dnmt1 in Nestin-expressing NSCs. Either Nestin-CreERT2, Dnmt1flox/+, or CreERT2-negative mice were used as controls. For in vitro assay of NSCs, ICR background mice were used. All pregnant mice (ICR background) were obtained from SLC (Shizuoka, Japan). For timed mating, the day of vaginal plug appearance was considered embryonic day (E) 0.5, and the day of birth was considered postnatal day (P) 0. Both male and female mice were analyzed, with no distinction. All mice used in this study were maintained on a 12-h light/dark cycle with free access to food and water. All animal procedures were in accordance with the animal experimentation guidelines of the Nara Institute of Science and Technology, which follow the National Institutes of Health Guide for the Care and Use of Laboratory Animals. All efforts were made to minimize animal suffering and to reduce the number of animals used. Tamoxifen (Sigma, T5648) was dissolved in sesame oil at 10 mg/ml. Intraperitoneal injections of tamoxifen (2 mg) were administered to pregnant mice using 27-gauge needles.
Immunocytochemistry
Mice were deeply anesthetized with sodium pentobarbital (50 mg/kg i.p.) prior to perfusion with 4% PFA in PBS. Brains were dissected and postfixed with 4% PFA in PBS overnight at 4°C. For cryoprotection, fixed brains were stored in 15% sucrose in PBS overnight at 4°C, and then transferred into 30% sucrose in PBS overnight at 4°C. One side of the brain was embedded in optimal cutting temperature (OCT) compound (Tissue Tek, Sakura Finetek, 25608–930) and frozen at −80°C for cryosectioning. Frozen brains were serially sectioned with the Leica CM 1900 (Leica Microsystems, Wetzlar, Germany) in the coronal or sagittal plane at 40 μm thickness. Cryosections were washed with PBS and blocked for 1 h at room temperature with blocking solution (3% FBS and 0.1% Triton X-100), and incubated overnight at 4°C with primary antibodies diluted in blocking solution. The following primary antibodies were used in the present study: mouse anti-Ki67 (1:500; BD Biosciences, 550609); goat anti-DCX (1:100; Santa Cruz Biotechnology, sc-8066); chick anti-GFAP (1:500; Millipore, AB5541); rabbit anti-IbaI (1:500, Wako, 019–19741); rat anti CD68 (1:500, AbD Serotec, FA-11). For staining of Ki67, antigen retrieval was performed by heating sections in the target retrieval solution (DAKO) at 105°C for 15 min prior to blocking. After three washes in PBS, sections were incubated for 2 h with corresponding secondary antibodies: CF488 donkey anti-mouse IgG (H+L), highly cross-adsorbed (1:500; Biotium, 20014); CF543 donkey anti-rabbit IgG (H+L), highly cross-adsorbed (1:500; Biotium, 20038); CF647 donkey anti-goat IgG (H+L), highly cross-adsorbed (1:500; Biotium, 20048); CF647 donkey anti-rabbit IgG (H+L), highly cross-adsorbed (1:500; Biotium, 20047); and CF568 donkey anti-rat IgG (H+L), highly cross-adsorbed (1:500; Biotium, 20092). Hoechst 33258 (1:500; Nacalai Tesque) was used for nuclear staining. After a final rinse with PBS, sections were mounted on glass slides with Immu-Mount (Thermo Scientific, 9990412), and images were taken using a Zeiss LSM 780 confocal microscope (Carl Zeiss, Germany) or Leica AF600 fluorescence microscope (Leica Microsystems GmbH, Germany).
Open-field test
The open-field test was performed with 8-week old male mice. Each mouse was placed in the corner of the open-field apparatus (50 cm × 50 cm × 30 cm; O'Hara & Co., Tokyo, Japan), which was illuminated at 100 lx. Total distance traveled (cm) and time spent in the center area were recorded. The center area was defined as the 30 cm × 30 cm area located at the center of the open field. Data were collected for 10 min.
Statistical analysis
At least 3 mice per group were analyzed for cell counting, and 6 mice per group were used for behavioral testing. Statistical analyses were performed using Student's t-test. All experiments were independently replicated at least 3 times. Differences were considered statistically significant at p < 0.05. Asterisks indicate significant differences (* < 0.05; ** < 0.01, *** < 0.001).
Abbreviations
cKO | = | conditional knockout |
CNS | = | central nervous system |
DCX | = | doublecortin |
DG | = | dentate gyrus |
Dnmt1 | = | DNA methyltransferase 1 |
Ezh2 | = | enhancer of zeste homolog 2 |
GFAP | = | glial fibrillary acidic protein |
HDAC | = | histone deacetylase |
LSD1 | = | lysine-specific histone demethylase 1 |
NSC | = | neural stem cell |
RMS | = | rostral migratory stream |
SVZ | = | subventricular zone |
TNF-α | = | tumor necrosis factor α |
VPA | = | valproic acid |
WT | = | wild-type |
Disclosure of potential conflicts of interest
No potential conflicts of interest were disclosed.
Acknowledgments
We thank T. Matsuda and S. Katada for valuable discussions. We are grateful to Dr. R. Jaenisch for Dnmt1flox mice, Dr. R. Kageyama for Nestin-CreERT2 mice. We are very grateful to M. Tano and Y. Nakagawa for their excellent secretarial assistance. We appreciate the technical assistance from the Research Support Center, Research Center for Human Disease Modeling, Kyushu University Graduate School of Medical Sciences. We would like to thank Editage for their editorial support of manuscript.
Funding
This research was supported by the Japan Agency for Medical Research and Development, Core Research for Evolutional Science and Technology (AMED-CREST) to K.N., and JSPS KAKENHI Grant-in-Aid for Scientific Research (C) (no. JP26430083) to M.N., and a Grant-in-Aid for JSPS Fellows (no. JP13J09870) and a Sasakawa Scientific Research Grant to H.N.
References
- Altman J, Das GD. Autoradiographic and histological evidence of postnatal hippocampal neurogenesis in rats. J Comp Neurol 1965; 124:319-35; PMID:5861717; http://dx.doi.org/10.1002/cne.901240303
- Lois C, Alvarez-Buylla A. Proliferating subventricular zone cells in the adult mammalian forebrain can differentiate into neurons and glia. Proc Natl Acad Sci U S A 1993; 90:2074-7; PMID:8446631; http://dx.doi.org/10.1073/pnas.90.5.2074
- Kuhn HG, Dickinson-Anson H, Gage FH. Neurogenesis in the dentate gyrus of the adult rat: age-related decrease of neuronal progenitor proliferation. J Neurosci 1996; 16:2027-33; PMID:8604047
- Eriksson PS, Perfilieva E, Björk-Eriksson T, Alborn A-M, Nordborg C, Peterson DA, Gage FH. Neurogenesis in the adult human hippocampus. Nat Med 1998; 4:1313-7; PMID:9809557; http://dx.doi.org/10.1038/3305
- Imayoshi I, Sakamoto M, Ohtsuka T, Takao K, Miyakawa T, Yamaguchi M, Mori K, Ikeda T, Itohara S, Kageyama R. Roles of continuous neurogenesis in the structural and functional integrity of the adult forebrain. Nat Neurosci 2008; 11:1153-61; PMID:18758458; http://dx.doi.org/10.1038/nn.2185
- Deng W, Aimone JB, Gage FH. New neurons and new memories: how does adult hippocampal neurogenesis affect learning and memory? Nat Rev Neurosci 2010; 11:339-50; PMID:20354534; http://dx.doi.org/10.1038/nrn2822
- Duan X, Chang JH, Ge S, Faulkner RL, Kim JY, Kitabatake Y, Liu XB, Yang CH, Jordan JD, Ma DK, et al. Disrupted-In-Schizophrenia 1 regulates integration of newly generated neurons in the adult brain. Cell 2007; 130:1146-58; PMID:17825401; http://dx.doi.org/10.1016/j.cell.2007.07.010
- Ming G-l, Song H. Adult neurogenesis in the mammalian brain: significant answers and significant questions. Neuron 2011; 70:687-702; PMID:21609825; http://dx.doi.org/10.1016/j.neuron.2011.05.001
- Winner B, Kohl Z, Gage FH. Neurodegenerative disease and adult neurogenesis. Eur J Neurosci 2011; 33:1139-51; PMID:21395858; http://dx.doi.org/10.1111/j.1460-9568.2011.07613.x
- Snyder JS, Soumier A, Brewer M, Pickel J, Cameron HA. Adult hippocampal neurogenesis buffers stress responses and depressive behaviour. Nature 2011; 476:458-61; PMID:21814201; http://dx.doi.org/10.1038/nature10287
- Schoenfeld TJ, Cameron HA. Adult neurogenesis and mental illness. Neuropsychopharmacology 2015; 40:113-28; PMID:25178407; http://dx.doi.org/10.1038/npp.2014.230
- Fuentealba LC, Obernier K, Alvarez-Buylla A. Adult neural stem cells bridge their niche. Cell stem cell 2012; 10:698-708; PMID:22704510; http://dx.doi.org/10.1016/j.stem.2012.05.012
- Matsuda T, Murao N, Katano Y, Juliandi B, Kohyama J, Akira S, Kawai T, Nakashima K. TLR9 signalling in microglia attenuates seizure-induced aberrant neurogenesis in the adult hippocampus. Nature communications 2015; 6:6514; PMID:25751136; http://dx.doi.org/10.1038/ncomms7514
- Murao N, Noguchi H, Nakashima K. Epigenetic regulation of neural stem cell property from embryo to adult. Neuroepigenetics 2016; 5:1-10.
- Hsieh J, Nakashima K, Kuwabara T, Mejia E, Gage FH. Histone deacetylase inhibition-mediated neuronal differentiation of multipotent adult neural progenitor cells. Proc Natl Acad Sci U S A 2004; 101:16659-64; PMID:15537713; http://dx.doi.org/10.1073/pnas.0407643101
- Noguchi H, Kimura A, Murao N, Matsuda T, Namihira M, Nakashima K. Expression of DNMT1 in neural stem/precursor cells is critical for survival of newly generated neurons in the adult hippocampus. Neurosci Res 2015; 95:1-11; PMID:25659757; http://dx.doi.org/10.1016/j.neures.2015.01.014
- Namihira M, Nakashima K. Mechanisms of astrocytogenesis in the mammalian brain. Curr Opin Neurobiol 2013; 23:921-7; PMID:23827784; http://dx.doi.org/10.1016/j.conb.2013.06.002
- MuhChyi C, Juliandi B, Matsuda T, Nakashima K. Epigenetic regulation of neural stem cell fate during corticogenesis. Int J Dev Neurosci 2013; 31:424-33; PMID:23466416; http://dx.doi.org/10.1016/j.ijdevneu.2013.02.006
- Noguchi H, Murao N, Kimura A, Matsuda T, Namihira M, Nakashima K. DNA Methyltransferase 1 Is Indispensable for Development of the Hippocampal Dentate Gyrus. J Neurosci 2016; 36:6050-68; PMID:27251626; http://dx.doi.org/10.1523/JNEUROSCI.0512-16.2016
- Juliandi B, Tanemura K, Igarashi K, Tominaga T, Furukawa Y, Otsuka M, Moriyama N, Ikegami D, Abematsu M, Sanosaka T, et al. Reduced Adult Hippocampal Neurogenesis and Cognitive Impairments following Prenatal Treatment of the Antiepileptic Drug Valproic Acid. Stem cell reports 2015; 5:996-1009; PMID:26677766; http://dx.doi.org/10.1016/j.stemcr.2015.10.012
- Fan G, Beard C, Chen RZ, Csankovszki G, Sun Y, Siniaia M, Biniszkiewicz D, Bates B, Lee PP, Kuhn R, et al. DNA hypomethylation perturbs the function and survival of CNS neurons in postnatal animals. J Neurosci 2001; 21:788-97; PMID:11157065
- Imayoshi I, Ohtsuka T, Metzger D, Chambon P, Kageyama R. Temporal regulation of Cre recombinase activity in neural stem cells. Genesis 2006; 44:233-8; PMID:16652364; http://dx.doi.org/10.1002/dvg.20212
- Murao N, Matsuda T, Noguchi H, Koseki H, Namihira M, Nakashima K. Characterization of Np95 expression in mouse brain from embryo to adult: a novel marker for proliferating neural stem/precursor cells. Neurogenesis 2014; 1:e976026; PMID:27504471
- Hutnick LK, Golshani P, Namihira M, Xue Z, Matynia A, Yang XW, Silva AJ, Schweizer FE, Fan G. DNA hypomethylation restricted to the murine forebrain induces cortical degeneration and impairs postnatal neuronal maturation. Hum Mol Genet 2009; 18:2875-88; PMID:19433415; http://dx.doi.org/10.1093/hmg/ddp222
- Takizawa T, Nakashima K, Namihira M, Ochiai W, Uemura A, Yanagisawa M, Fujita N, Nakao M, Taga T. DNA methylation is a critical cell-intrinsic determinant of astrocyte differentiation in the fetal brain. Dev Cell 2001; 1:749-58; PMID:11740937; http://dx.doi.org/10.1016/S1534-5807(01)00101-0
- Namihira M, Kohyama J, Semi K, Sanosaka T, Deneen B, Taga T, Nakashima K. Committed neuronal precursors confer astrocytic potential on residual neural precursor cells. Dev Cell 2009; 16:245-55; PMID:19217426; http://dx.doi.org/10.1016/j.devcel.2008.12.014
- Fan G, Martinowich K, Chin MH, He F, Fouse SD, Hutnick L, Hattori D, Ge W, Shen Y, Wu H, et al. DNA methylation controls the timing of astrogliogenesis through regulation of JAK-STAT signaling. Development 2005; 132:3345-56; PMID:16014513; http://dx.doi.org/10.1242/dev.01912
- Tabata H. Diverse subtypes of astrocytes and their development during corticogenesis. Front Neurosci 2015; 9:114; PMID:25904839; http://dx.doi.org/10.3389/fnins.2015.00114
- Brahmachari S, Fung YK, Pahan K. Induction of glial fibrillary acidic protein expression in astrocytes by nitric oxide. J Neurosci 2006; 26:4930-9; PMID:16672668; http://dx.doi.org/10.1523/JNEUROSCI.5480-05.2006
- Feresten AH, Barakauskas V, Ypsilanti A, Barr AM, Beasley CL. Increased expression of glial fibrillary acidic protein in prefrontal cortex in psychotic illness. Schizophr Res 2013; 150:252-7; PMID:23911257; http://dx.doi.org/10.1016/j.schres.2013.07.024
- Sofroniew MV. Astrocyte barriers to neurotoxic inflammation. Nat Rev Neurosci 2015; 16:249-63; PMID:25891508; http://dx.doi.org/10.1038/nrn3898
- Monif M, Burnstock G, Williams DA. Microglia: proliferation and activation driven by the P2X7 receptor. Int J Biochem Cell Biol 2010; 42:1753-6; PMID:20599520; http://dx.doi.org/10.1016/j.biocel.2010.06.021
- Diz-Chaves Y, Pernia O, Carrero P, Garcia-Segura LM. Prenatal stress causes alterations in the morphology of microglia and the inflammatory response of the hippocampus of adult female mice. J Neuroinflammation 2012; 9:71; PMID:22520439; http://dx.doi.org/10.1186/1742-2094-9-71
- Song C, Wang H. Cytokines mediated inflammation and decreased neurogenesis in animal models of depression. Prog Neuropsychopharmacol Biol Psychiatry 2011; 35:760-8; PMID:20600462; http://dx.doi.org/10.1016/j.pnpbp.2010.06.020
- Parks CL, Robinson PS, Sibille E, Shenk T, Toth M. Increased anxiety of mice lacking the serotonin1A receptor. Proc Natl Acad Sci U S A 1998; 95:10734-9; PMID:9724773; http://dx.doi.org/10.1073/pnas.95.18.10734
- Qin W, Leonhardt H, Pichler G. Regulation of DNA methyltransferase 1 by interactions and modifications. Nucleus 2011; 2:392-402; PMID:21989236; http://dx.doi.org/10.4161/nucl.2.5.17928
- Clements EG, Mohammad HP, Leadem BR, Easwaran H, Cai Y, Van Neste L, Baylin SB. DNMT1 modulates gene expression without its catalytic activity partially through its interactions with histone-modifying enzymes. Nucleic Acids Res 2012; 40:4334-46; PMID:22278882; http://dx.doi.org/10.1093/nar/gks031
- Espada J. Non-catalytic functions of DNMT1. Epigenetics : official journal of the DNA Methylation Society 2012; 7:115-8; http://dx.doi.org/10.4161/epi.7.2.18756
- Neumann H, Schweigreiter R, Yamashita T, Rosenkranz K, Wekerle H, Barde YA. Tumor necrosis factor inhibits neurite outgrowth and branching of hippocampal neurons by a rho-dependent mechanism. J Neurosci 2002; 22:854-62; PMID:11826115
- Guadagno J, Swan P, Shaikh R, Cregan SP. Microglia-derived IL-1beta triggers p53-mediated cell cycle arrest and apoptosis in neural precursor cells. Cell Death Dis 2015; 6:e1779; PMID:26043079; http://dx.doi.org/10.1038/cddis.2015.151
- Tang MM, Lin WJ, Pan YQ, Guan XT, Li YC. Hippocampal neurogenesis dysfunction linked to depressive-like behaviors in a neuroinflammation induced model of depression. Physiol Behav 2016; 161:166-73; PMID:27106565; http://dx.doi.org/10.1016/j.physbeh.2016.04.034
- Ostlund BD, Conradt E, Crowell SE, Tyrka AR, Marsit CJ, Lester BM. Prenatal Stress, Fearfulness, and the Epigenome: Exploratory Analysis of Sex Differences in DNA Methylation of the Glucocorticoid Receptor Gene. Front Behav Neurosci 2016; 10:147; PMID:27462209; http://dx.doi.org/10.3389/fnbeh.2016.00147
- Murgatroyd C, Patchev AV, Wu Y, Micale V, Bockmuhl Y, Fischer D, Holsboer F, Wotjak CT, Almeida OF, Spengler D. Dynamic DNA methylation programs persistent adverse effects of early-life stress. Nat Neurosci 2009; 12:1559-66; PMID:19898468; http://dx.doi.org/10.1038/nn.2436
- Wu Y, Patchev AV, Daniel G, Almeida OF, Spengler D. Early-life stress reduces DNA methylation of the Pomc gene in male mice. Endocrinology 2014; 155:1751-62; PMID:24506071; http://dx.doi.org/10.1210/en.2013-1868