ABSTRACT
Frontal lobe organisation displays a functional gradient, with overarching processing goals located in parts anterior to more subordinate goals, processed more posteriorly. Functional specialisation for syntax and phonology within language relevant areas has been supported by meta-analyses and reviews, but never directly tested experimentally. We tested for organised functional specialisation by manipulating syntactic case and phonotactics, creating violations at the end of otherwise matched and predictable sentences. Both violations led to increased activation in expected language regions. We observe the clearest signs of a functional gradient for language processing in the medial frontal cortex, where syntactic violations activated a more anterior portion compared to the phonotactic violations. A large overlap of syntactic and phonotactic processing in the left inferior frontal gyrus (LIFG) supports the view that general structured sequence processes are located in this area. These findings are relevant for understanding how sentence processing is implemented in hierarchically organised processing steps in the frontal lobe.
1. Introduction
The frontal lobe is associated with a diverse set of cognitive processes, including goal-directed cognition as well as language comprehension and production. These are examples of cognitive processes that can be viewed as hierarchically organised into overarching and subordinate processes, and a 2014 review has suggested that the prefrontal cortex (PFC) plays a crucial role in processing hierarchical processes from different domains. Jeon (Citation2014), Badre and d’Esposito (Citation2009) and Koechlin and Summerfield (Citation2007) proposed an anterior-posterior gradient organisation of the dorsolateral (PFC), which parallels the hierarchical processing steps, with evidence from the domains of goal-directed action (Badre & D’Esposito, Citation2009) and cognitive control (Koechlin & Summerfield, Citation2007). It has been proposed that the principles that govern PFC organisation of hierarchical processing in the domains of cognitive control and action, also hold for functional specialisation in frontal language areas: specifically functional specialisation of different aspects of language processing such as syntax and phonology. Syntax and phonology both contain structured sequences of words and phonemes, respectively (Udden & Bahlmann, Citation2012). In this study, we test whether there is an anterior-posterior gradient organisation of frontal lobe involvement in language comprehension and processing. Such an organisation would provide evidence for hierarchically organised processing steps involved in language processing (Udden et al., Citation2020).
Sentence processing consists of many known subprocesses. For instance, the sentence is unified (Hagoort, Citation2005; Vosse & Kempen, Citation2000) according to syntactic and phonological features with regularities on timescales spanning the sublexical and sentence level. Hagoort (Citation2005) argues that the prefrontal area underlying language unification processes includes BA44, BA45 and BA47, in other words, the entire left inferior frontal gyrus (LIFG). The involvement of BA47 has been supported by, e.g. meta-analysis (Hagoort & Indefrey, Citation2014) and techniques such as connectivity analyses (Ardila et al., Citation2017). While some prominent models of structure building in the brain emphasise the function of LIFG (in particular the posterior LIFG, the ventral BA44) in merging lexical items (Friederici et al., Citation2017), the alternative unification account of structure building suggests that the LIFG unifies across the lexical and sublexical level (Hagoort & Levelt, Citation2009). Sublexical phonological structure, e.g. phonotactics (phonotactics is the part of phonology studying the rules according to which sounds are formed into well-formed syllables in a language) has regularities on a relatively short timescale that can be considered to be partially nested within regularities of the overarching sentence level syntactic structure. This nesting relation of regularities in a sequential aspect of language structure emphasises the relation to the literature mentioned above on action sequences, where action sequences are understood as hierarchically organised into overarching goals and subgoals nested within those goals. To give an example from language processing, relevant to the current study, consider that words are grouped into well-formed sentences on the basis of syntactic structure. This structure enables the prediction of upcoming words and their syntactic features (such as their case). Once a word is predicted, phonotactic regularities for how sounds are grouped into well-formed syllables and words, enables the prediction of upcoming speech sounds (Heinz & Idsardi, Citation2011). The prediction of speech sounds uses information from the overarching sentence level syntactic unification process and can thus be considered, at least to some extent, as nested within this process.
An early review, Bookheimer (Citation2002) suggested that phonological processing and syntactic processing (and semantic processing, here not discussed) were supported by slightly different parts of the LIFG, with phonology being processed in the most posterior part of the LIFG and syntax more anteriorly, in the middle part. Such functional segregation is interesting when considering formal grammar theory. The Chomsky Hierarchy (Chomsky, Citation1956) divides all logically possible patterns into nested regions of complexity. Various features of natural language occupy different regions of the hierarchy. Certain sentence level syntactic regularities occupy higher than finite state grammars, whereas finite state grammars suffice to represent phonological regularities. This division into anterior vs. posterior portions of LIFG also followed the organisational principle found for action sequence processing in PFC. This is since the overarching processes with regularities at longer timescales (i.e. semantics but also importantly syntax) was generally processed more anteriorly to phonology. These results however showed considerable spatial overlap across LIFG for syntax and phonology (Bookheimer, Citation2002). While there indeed was a bias for the syntactic task to concern more overarching sentence aspects (e.g. syntactic violations) and for the phonological task to concern lexical aspects (e.g. rhyme judgments), the tasks used across studies in any review are naturally mixed. We are not aware of any other meta-analytic or experimental evidence supporting a distinction between syntax and phonology in the lateral frontal lobe. The goal of the current study was to experimentally test whether there is functional specialisation following an anterior-posterior gradient, where overarching sentence level syntactic processing is localised more anteriorly to sublexical phonological processing (phonotactics). A gradient organisation has been suggested as an organisational principle for the dorsolateral frontal lobe (Badre & D'Esposito, Citation2009; Koechlin & Summerfield, Citation2007) and for the medial frontal lobe (Badre & Nee, Citation2018). It has even been suggested that gradients are present throughout the entire cortex (Margulies et al., Citation2016). While we were interested in testing for gradients across (Fedorenko et al., Citation2012) the frontal lobe and elsewhere, our primary region of interest was the LIFG. This was due to the review findings (Bookheimer, Citation2002) already mentioned, as well as its role in structured sequence processing of natural and artificial languages (Bahlmann et al., Citation2008) (note that this is a function among other functions of LIFG and Broca’s area (Fedorenko et al., Citation2012; Friederici et al., Citation2017; Grodzinsky & Amunts, Citation2006; Matchin et al., Citation2017)). We opted to use the term LIFG instead of Broca’s area since Broca’s area classically only includes Brodmann Area (BA) 44 and BA45, while we were also interested in BA47.
In order to test whether phonotactic and syntactic processing can be separated in the frontal lobe, we created predictable sentences (in German, see Table S1), to avoid confusion about thematic role assignment and other confounding sentence level semantic differences between the correct and the violation conditions, as much as possible. The sentences contained violations to the overarching syntactic structure (case violation) or the sublexical phonotactic structure. While case violations are sometimes viewed as morphosyntactic violations, in German, they are indicated by the article preceding the noun phrase (not inflection) and which case is required depends on the verb and syntactic construction that is used. We thus refer to the current case manipulation as syntactic throughout the paper. Generally, violating predictions results in an increased local BOLD signal in the regions used to make the prediction (den Ouden et al., Citation2010), thus this design tests for functional specialisation for processing of syntactic vs. phonotactic structure. Both violations were located in a sentence final noun phrase. We chose to use predictable sentences in order to, as much as possible, avoid a lexical retrieval confound between conditions and tested the predictability of the sentence final noun phrase using a cloze test (see Figure S2 in the Supplementary Material). The two violation conditions are also similar on the surface, since for both of them, just one sound in the entire sentence was changed resulting in a syntactic case violation or a violation of the phonotactic rules of German. We kept the two conditions similar on a surface level to enable a comparison between two different linguistic domains: syntax and phonotactics and the underlying rules. While all words in the correct and syntactic violation (SV) conditions are known lexical items, the phonotactic violations necessarily result in pseudowords, which although highly reminiscent of actual German words and highly predictable from the sentence context, might lead to word retrieval difficulties in non-predictable sentences ().
Table 1. Example of a stimulus triplet.
A prominent suggestion is the following: when the prediction of upcoming words based on the sentence context is possible, this will be achieved through the use of sentence production machinery (Dell & Chang, Citation2014; Kittredge & Dell, Citation2016; Pickering & Garrod, Citation2007) or alternatively through abstract representations common to comprehension and production (Segaert et al., Citation2012). Our research question concerned the neural implementation of potentially hierarchical processing steps and functional specialisation during sentence processing in general. While we measure these processes during comprehension and model the sentence presentation only, we note that there might be underlying production processes engaged as well, perhaps in particular as we used predictable sentences (Matchin et al., Citation2017). Task demands have been observed to influence brain states even when the stimuli presented are identical, we therefore assume that the covert production task influenced comprehension of sentences across all conditions (Sakai & Passingham, Citation2003). To direct participants’ attention to the sentence final noun phrase and to make them predict the correct noun phrase, we instructed them to covertly repeat the correct/expected form of the sentence final noun phrase on each trial, during an 8–9 s long inter-trial interval. Thus, we are not asking questions on comprehension vs. production, comprehension or production in isolation or similar, but on sentence processing, in general, during the presentation of sentence material, assuming that comprehension and production processes are related through prediction. The study is however still informative for comprehension if this assumption would turn out not to be correct.
2. Methods
2.1. Participants
Twenty-six right-handed German native speakers, 16 females and 10 males, between the age of 28 and 20 (mean age = 23.8) participated in this experiment. The sample size was determined based on a power calculation using estimated effect sizes from a pilot experiment (N = 5) using the same task (for details see Supplementary Material). The participants had no hearing or reading impairment and no neurological impairments. Before the experiment, all participants gave informed consent and were told that they could stop the experiment at any moment without having to state their reasons for it. The study was approved by the local ethics committee (CMO, the local “Committee on Research Involving Human Subjects” in the Arnhem-Nijmegen region) and followed the guidelines of the Declaration of Helsinki. One participant was excluded due to scanner spikes in the data (resulting in 25 participants in total).
2.2. Experimental procedures
To ensure that participants attentively listened to and understood the sentences, we gave them comprehension questions on 24 randomly selected catch trials. To make sure the participants would never listen to the same sentence in a correct and an incorrect condition, we divided the sentences into three lists. Each list only contained one version of one triplet and each participant listened to the sentences from one list. Thus, the design was fully controlled, up to using identical lexical items between conditions, on the group level. The correct sentences were used as filler sentences in this design.
The presentation of the stimuli was controlled using the presentation software (Neurobehavioral Systems, Albany, CA, USA). All visual displays were in black on grey background. The participants first saw a fixation cross, and then the sentence was played. After the offset of the sentence, the participant covertly produced the expected sentence final noun phrase (correcting errors if they were perceived) during a silent inter-trial-interval (ITI) of 8–9 s. Sentence presentation was randomised, so the participants did not anticipate whether or not the next sentence would contain an error they had to correct. The depiction of the events in the experiment is viewed in .
Figure 1. Course of events in the experiment: During the entire experiment, participants saw a grey screen with a black fixation cross. We presented German sentences over scanner appropriate headphones. Participants were instructed to listen attentively to the sentences. If they heard an error at the sentence final noun phrase they were instructed to correct the error in their head (covert production). Errors only appeared at the sentence final noun phrase. For the general linear model, we modelled the sentence final noun phrase starting from the onset of the article.
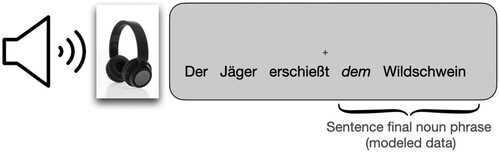
2.2.1. Stimulus material
The stimuli consisted of 165 spoken sentences that could appear in three forms (stimulus triplets). Each stimulus triplet contained three forms of essentially the same sentence: one correct (C) or filler–sentence version, one syntactically violated (SV) version and one phonotactically violated (PV) version. The sentences were identical except for the violation. On average, the sentences were 2.18 s long.
2.2.2. The violations
The first part of the sentences was designed such that the sentence final noun phrase was highly predictable. We carried out a cloze test to validate the predictability of the sentence final noun phrase on an independent group of participants (see results in the Supplementary Material). The cloze test confirmed that the sentence final noun phrase was highly predictable. On average 80.1% of sentence final noun phrases were predicted correctly.
In the syntactically incorrect condition, the manipulation occurred at the determiner of the sentence final noun phrase. The verb and overall structure of the sentence necessitates that a specific case is used on the object of the sentence which is expressed on the determiner of the sentence final noun phrase in German. The syntactic violation, while being just a small change in speech sounds, has global consequences for the sentence given that one sound change on the determiner changes the case of the noun phrase in German.
The phonotactic manipulation contained an altered sound cluster at the sentence final word. One sound of a consonant sound cluster was exchanged to form another sound cluster that was phonotactically illegal according to German phonotactic rules. The violation appeared at the end of the word in order to enable the participant to retrieve the word before the violation appeared. This violation was highly similar to the syntactic violation as again only one speech sound was changed, but the consequences were not for the whole sentence syntax but instead just for the phonotactics of the word, transforming a word following the German phonotactic rules into a highly similar pseudoword that contains a phonotactic rule violation. The contrast between syntactic and phonotactic violation was not only between the linguistic level of analysis but also a contrast between global and local violations, similar to how levels of cognitive control have been operationalised in Koechlin and Summerfield (Koechlin & Summerfield, Citation2007). Participants were instructed to listen attentively to the sentences presented. If they perceived an error, they were instructed to covertly repeat the correct version of the phrase, starting from the word that was incorrect. We tested whether participants carried out the instruction using a post-scanning questionnaire.
2.3. fMRI data acquisition
The fMRI data were acquired using a 3T Siemens TRIO scanner. We scanned 29 slices in ascending order (FOV64 × 64). The voxels were 3 × 3 × 3 mm in size. We used a single spin-echo sequence with a TR of 2 s, a TE of 35 ms, and a flip angle of 90°. The structural images were acquired using an MPRAGE T1 sequence with a flip angle of 8° and with a TE of 3.03 ms and a TR of 2300 ms, 192 slices, each 1 mm thick, were acquired for each participant.
2.4. Data analysis
2.4.1. Preprocessing
SPM8 was used to analyse the fMRI data (https://www.fil.ion.ucl.ac.uk/spm/software/spm8/). Images were realigned to correct for individual motion of participants and slice timing differences were corrected. The images were then co-registered to the individual structural scans and normalised to the MNI template. Finally, the images were smoothed using a 3D isotropic Gaussian kernel with FWHM of 10 mm.
2.4.2. Whole brain analysis
For the whole brain analysis, we used the standard general linear model approach. We modelled the onset of the sentences until the onset of the sentence final noun phrase as a separate sentence event irrespective of the manipulation since the differences between conditions only appeared after the onset of the sentence final noun phrase. The time between the onset of the sentence final noun-phrase and the onset of the ITI was modelled as “correct event”, “syntactically incorrect event” or “phonotactically incorrect event”, depending on which condition the presented sentence had belonged to. The sentence final noun phrase was always modelled as one event, independent of whether they appeared in a correct, syntactically incorrect or phonotactically incorrect sentence. We modelled the time after the onset of the ITI until the onset of the next sentence as an ITI-event. Note that the covert production took place only during the ITI, thus no covert production activity was used to make the contrast we test. The comprehension questions were also modelled as “question event” spanning from the onset of the question to the answer. The events of the design were convolved with the canonical hemodynamic response function that is included in the SPM8 package. Motion parameters from realignment and parameters correcting for global fluctuations in the signal were also included.
We tested four contrasts. The first two tested the two violation contrasts SV > C and PV > C. For the second level analysis, we used the contrast images obtained for the two violation contrasts to test whether the two violation contrasts activated LIFG significantly at the group level. We analysed the violation contrast on the second level using a one-way ANOVA, entering the first level contrast images for both violation contrasts. We also carried out a conjunction analysis as described in Nichols et al. (Citation2005) and implemented in SPM8.
Finally, for completeness, we also tested the two direct contrasts SV > PV and PV > SV. These two contrasts were of less interest, as there are issues with interpreting two violation conditions compared against each other. We still included these contrasts, as the two ways of constructing contrasts (violations against correct and two violations against each other) represent parallel and complementary perspectives on the data.
We report significant clusters for P < .05 family-wise-error-rate (FWE)-corrected. We created results images with a cluster forming threshold of P < .001 uncorrected. The table reports clusters significant at P < .05 FWE corrected. All local maxima are reported as MNI coordinates. Assignment of clusters and voxels to gyri, sulci and Brodmann areas (for LIFG) was done using the AAL toolbox implemented in SPM (Tzourio-Mazoyer et al., Citation2002).
3. Results
3.1. Behavioural results
On average participants answered 21.15 (SD = 2.20) of the 24 questions correctly.
3.2. fMRI whole brain results
For both violation contrasts, three clusters reached significance at the whole brain level. For the phonotactic violations, we observed activity in the LIFG (BA 44, 45 and 47) (cluster extent and P-values are reported in ). The results are viewed in and . The cluster also covered the anterior and middle insula, Heschl’s gyrus, the precentral gyrus, the middle and inferior temporal gyrus as well as parietal regions, namely the supramarginal gyrus and the inferior parietal lobule. This cluster also extended into the fusiform gyrus in the occipital lobe. A second cluster observed in the right hemisphere included the RIFG (BA 44 and 45), the right insula, the right temporal pole as well as the right superior and middle temporal gyrus. The phonotactic violations also elicited activity in a third cluster in the left supplemental motor area.
Figure 2. Whole brain results. The figure shows the two main contrasts: phonological violation vs. correct (in blue) and syntactic violation vs. correct (in orange). In purple, we display the conjunction of these two contrasts. All clusters in the images are significant at corrected PFWE < 0.05. To the left, we display an axial plane (z = 11), indicated by a line crossing the upper sagittal plane, one step to the right. The locations of the two sagittal planes are depicted by two lines crossing the axial plane. In the lower sagittal plane, the locations of the three coronal planes in are indicated. All brain figures were drawn using the mango software (http://ric.uthscsa.edu/mango/mango.html).
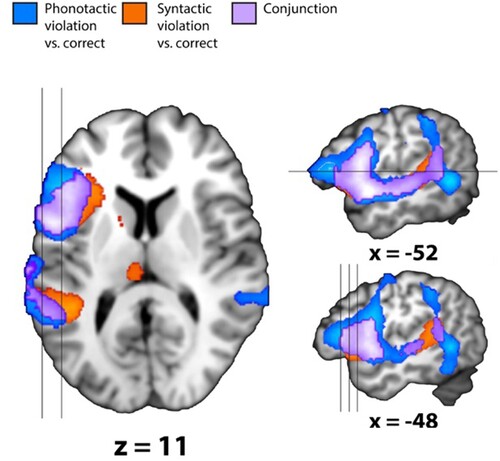
Figure 3. Medial view (medial frontal gyrus, whole brain results). In a more posterior section (y = 20, in the middle), there was an overlap (purple) and also some activity for phonotactics (blue, dorsally) and syntax (orange, ventrally), separately. In the lower sagittal plane of , the locations of these three coronal planes are indicated. All clusters in the images are significant at corrected PFWE < 0.05.
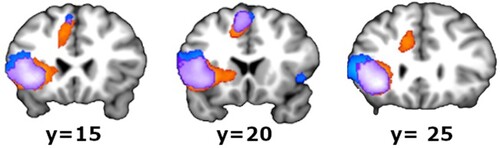
Table 2. Activations for the two main effects SV > C and PV > C.
For the syntactic violations, we observed a cluster including several regions, from the left frontal operculum to the LIFG, the insula and the left temporal gyrus including Heschl’s gyrus. This cluster also included the caudate nucleus, the putamen and the pallidum. A second cluster active for syntactic violation was observed in the left anterior cingulate, the left posterior medial frontal cortex and the left supplementary motor area. Finally, a smaller cluster was observed in the left and right middle cingulate gyrus and thalamus.
In the conjunction of the two violation contrasts, overlapping activity was observed in two clusters: a larger cluster spanned the area of the LIFG (BA 44, 45 and 47), the left superior and middle temporal gyrus including the insula and Heschl’s gyrus and extended into the left inferior parietal lobule. A second cluster was observed in the regions of the left and right supplementary motor area and left and right posterior medial frontal cortex. Cluster and P-values are reported in .
Table 3. Activations for the two main effects SV > C and PV > C.
3.3. Results of the direct comparison of the two violation contrasts
Apart from comparing the syntactic and phonotactic violations with correct sentences, we also directly compared the two violations with each other on the whole brain level. The results of this analysis are reported in more detail in and . For the contrast SV > PV, we observed activation in six clusters across the brain. There were two large clusters in right and left occipital areas and three in frontal areas, including the superior frontal gyrus, the middle frontal gyrus and the superior orbital gyrus. Finally, one cluster was located in the cerebellum. For PV > SV contrast, we observed activation in two clusters that were both located in temporal regions: one in the left middle and inferior temporal gyrus and the other in the right superior and middle temporal gyrus.
Table 4. Activations for the direct comparison SV > PV.
Table 5. Activations for the direct comparison PV > SV whole brain.
4. Discussion
Syntactic case violations as well as phonotactic violations activated the whole LIFG (relative to correct sentences). This result supports the relevance of the structured sequence processing account of LIFG function, also in the context of natural language processing, see further Bahlmann et al. (Citation2008). We could not find any support for a rostro-caudal gradient in the lateral frontal lobe, which has been observed for hierarchically organised action sequences (Badre & D’Esposito, Citation2009; Koechlin & Summerfield, Citation2007), extending to sentence processing. We did observe spatial differences between the two conditions (phonotactic violations and syntactic violations) across frontal and temporal areas. Particularly, we did observe functional specialisation in the medial frontal gyrus, where syntactic violations activated a more anterior part than the phonotactic violations. We believe that the fact that these observed functional specialisations are following the direction of the theorised organisation of the frontal cortex add some support to a rostro-caudal gradient in this region. A rostro-caudal gradient organisation of the medial frontal cortex, parallel to the organisation in the lateral frontal lobe, has been reported before in the context of cognitive control tasks operating on different hierarchical levels (sensorimotor, episodic and contextual; Kouneiher et al., Citation2009). The action and control tasks we have discussed (Badre & D’Esposito, Citation2009) – (Koechlin & Summerfield, Citation2007; Kouneiher et al., Citation2009) crucially involve the processing of action sequences that link several actions in a structured manner. It has been suggested that structured sequence processing is common to action and language (Bahlmann et al., Citation2008; Jackendoff, Citation2009; Jeon, Citation2014), but see (Pulvermuller, Citation2014) and (Moro, Citation2014). Our novel finding of a gradient organisation in the medial frontal gyrus (see also the global results in the next paragraph) supports these parallels, at least for some brain networks. With respect to LIFG (more specifically BA 44), there are recent results supporting a distinction between action and language in this region (Papitto et al., Citation2020).
4.1 Global differences between conditions
As already stated, the whole brain analysis did not provide evidence for or against functional specialisation for syntactic vs. phonotactic structured sequence processing in LIFG. Differences between the two violation conditions were however observed in several other regions. In the nearby region of the left frontal operculum/insula, the syntactic cluster extended further in the medial direction (see ), in particular into the anterior insula. A similar medial extension for syntax was present in the posterior superior/middle temporal gyrus (pSTG and pMTG). The posterior MTG has been linked to syntactic processing for both comprehension and production in a recent model of speech processing by Matchin and Hickok (Citation2020). They argue that the pMTG is a key region for hierarchical syntactic sentence comprehension based on both experimental (Matchin et al., Citation2017) and patient studies. Our results are in line with their suggested function of pMTG.
In addition, left hemisphere basal ganglia structures (the left caudate nucleus, putamen and pallidum) were activated for syntax only. On the other hand, the phonotactics contrast generally extended more dorsally (and anteriorly) in the lateral frontal lobe (dorsally towards the inferior frontal sulcus and middle frontal gyrus and anteriorly towards BA 10). Left lateral parietal, right superior temporal and left posterior inferior temporal activity was much more extensive for phonotactics, compared to syntax. The complementary analysis comparing the two violation conditions against each other should be interpreted with caution (precisely as they are two different violation conditions). The global pattern of results suggested more frontal activity for the syntactic violations and more (low-level sensory) temporal activity for the phonotactic condition. The increased activity in low-level sensory temporal areas (e.g. also in the right STG) was also observed in the analysis comparing phonotactics vs. correct sentences, but not for syntactic violations vs. correct sentences. The pattern of results described in this section can tentatively be understood as supporting a relative hierarchical relation between syntax and phonotactics at a global brain level, but this should be further formally tested. For instance, a design where phonological rules are dependent on predictions from sentence level syntax would be appropriate. We are not aware of any study on natural languages that achieves such a truly nested design (for instance, it does not seem to be the case in Jeon & Friederici, Citation2013). Bahlmann et al. (Citation2015) used a design with hierarchical (nested) processing steps using language stimuli to establish a rostro-caudal organisation in dorsal parts of the frontal love. However, the study was not designed to ask questions on hierarchical processes in natural language, but is rather an action study with language stimuli.
It remains unclear to us why the SV > PV contrast generated activity in the occipital lobes, which may be interpreted as a finding not following the hierarchical principle we have discussed. In summary, we observed many differences between phonotactics and syntax across language relevant regions, globally suggesting a more low-level sensory location of phonotactic processing (and tentatively more frontal syntactic processing).
4.2. Functional specialisation in the medial frontal cortex
To further understand the functional specialisation between conditions in the medial frontal cortex we observe, it is interesting to note that research on rhesus monkeys shows that the basic pattern of lamination and frequency of projections extending in a rostral-to-caudal direction (less laminated areas having more connections) is present both in the lateral and ventromedial frontal lobe (Badre & D’Esposito, Citation2009). As such, the same causes of a rostro-caudal functional gradient in the frontal cortex are present for both lateral and medial frontal cortex. Summarising this finding, we have observed a pattern in the context of a language processing task that supports a rostro-caudal gradient, but in the medial frontal cortex. This novel finding extends the previous knowledge on the extent of the functional organisation of the frontal lobe into a rostro-caudal gradient across cognitive domains. As our task is constructed based on the structured sequence processing perspective, corresponding tasks could easily be constructed for comparative work on other species. As already stated, the underlying neural gradient architecture is at least present in rhesus monkeys, but the question is how this is used functionally, e.g. in the context of processing sequential regularities.
More generally, we may attribute the observed activity in the medial frontal cortex for both violation contrasts to error monitoring functions of this region (which includes the anterior cingulate cortex, ACC). Whether language-specific error monitoring is carried out by domain-general mechanisms is still not understood (Acheson & Hagoort, Citation2014). Domain-general cognitive control mechanisms and language-specific conflict and error monitoring processes may be operating in parallel (Hsu et al., Citation2017), and our finding might be a part of the underlying architecture for either language-specific or domain-general aspects.
4.3. Previous literature and the formal grammar perspective
There is now a fair amount of converging evidence (Bahlmann et al., Citation2015; Jeon & Friederici, Citation2013) for an rostro-caudal organisation (Badre & D’Esposito, Citation2009) of the more dorsal parts of the frontal lobe when this part of cortex is recruited in language tasks. There is less evidence for a functional gradient in the LIFG in language tasks, with one exception. This study focused purely on language (Jeon & Friederici, Citation2013) and used grammaticality judgments in a simplified (artificial) Korean language and in German to test whether a gradient like organisation would be present for syntactic processing on different temporal scales. Grammaticality judgments on the lowest temporal scale elicited activation in BA 44, the medial temporal scale elicited activation in BA 46 and the highest temporal scale lead to increased activation in BA 47. Jeon and Friederici (Citation2013), diverging from the views taken by both Koechlin and Summerfield (Citation2007) and Badre and d’Esposito (Citation2009), view automaticity as the feature that drives the gradient organisation. Our results are not generally in line with the results by Jeon and Friederici (Citation2013), but they do not invalidate them, either.
In this context, it is noteworthy that in formal grammar theory (or at least in some accounts thereof), the kind of sequential regularities present in syntax and phonology (hence phonotactics as well) are divided into two complexity classes (Berwick & Chomsky, Citation2017; Heinz & Idsardi, Citation2011). Sentence level syntactic regularities are often represented with higher than finite-state grammars (Badre & D’Esposito, Citation2009) whereas a finite state grammar suffices to represent phonological regularities. The functional specialisation for syntax vs. phonotactics that we observe (e.g. in regions such as anterior insula, the basal ganglia and the medial frontal cortex but potentially also the tentative division in LIFG reported on in the supplement, into a syntactic focus in ventral BA 44 with phonotactics just anterior and posterior of it) could thus also be considered as a consequence of the different classes of computational complexity present in sentence level syntax vs. phonotactics. On the other hand, the extensive overlap we observe in, e.g. LIFG (as statistically tested on whole brain level), can at the same time be considered as empirical evidence rather emphasising the similarities of the sequence processing mechanisms in syntax and phonotactics. Thus, with respect to this issue, what our data show is which brain regions show functional specialisation for sequence processing in syntax vs. phonotactics and which do not.
We opted for a design controlling for the exact words used. This led us to modify the sentence final noun-phrase in sentences with a similar structure. In this context, predictions on the upcoming syntax and phonotactic sequence are both at the same time predictions on the exact noun phrase to come. The noun phrase was considered as one unit in the design and modelled as one event. However, bottom-up effects of the realisation of the violated expectation, which had to be on determiners vs. nouns in this context of sentence-final noun-phrases, cannot be excluded.
4.4. Phonotactic violations
Our results showing increased activation of the LIFG during phonotactic violations are also consistent with previous research studying phonotactics in pseudowords (Jacquemot et al., Citation2003). In another study, the presentation of words, containing higher vs. lower phonotactic frequency of sound clusters, led to an increase in the anterior LIFG (Vaden et al., Citation2011). All these results suggest a role of LIFG for structured sequence processing, not only for syntactic processing but also phonotactics, but we are crucially extending the results on isolated words (Jacquemot et al., Citation2003; Vaden et al., Citation2011), to a full sentence context. While the link to LIFG is strengthened, it is at the same time clear that phonotactic frequency cannot be directly mapped onto an increase or decrease in the BOLD signal, when considering these three studies (39, 40 and the current study) together. However, as our presentation of sound clusters (and the presentation in (Jacquemot et al., Citation2003)) with a phonotactic frequency of zero led to an increased BOLD response in LIFG, there is now converging evidence for violations leading to activity in LIFG, relative to correct phonotactic sequences.
4.5. Syntactic violations
We demonstrate that syntactic violations of predictions from a global sentence context on the case of a sentence final word, causes increased activation in the LIFG. This is in line with the view that structured sequence processing, subserved by the LIFG, is important for sentence level syntactic processing. To our knowledge, there are only three previous studies investigating the neural correlates of case violations. The first study by Nieuwland et al. (Citation2012) did not report increasing activity in the LIFG in response to case violations (in Basque). Instead, they reported increasing activation in parietal areas (Nieuwland et al., Citation2012), interpreted as related to problems with thematic role assignment as a consequence of the violation. These discrepancies might be accounted for by differences in the respective sentence materials, as we used predictable sentences and violated the sentence final noun phrase only. One study investigating English case processing by Yokoyama et al. (Citation2012) made the observation that activity in LIFG and the posterior superior temporal sulcus depended on whether nominative, genitive or accusative case was used (Yokoyama et al., Citation2012). Processing of case particles in Japanese was studied by Hashimoto et al. (Citation2014) who found that LIFG was more active when a syntactic task was performed on the particles, compared to a phonological task on particles (Hashimoto et al., Citation2014). It has to be said, however, that case is a diverse phenomenon that is expressed very differently in different languages. The observation that case violations activated LIFG is also in line with a study on noun phrases in isolation with grammatical gender violations which, similarly to case violations, are observed at the determiner in German. In this study, nouns preceded by the wrong article led to increased activation in ventral (z = 15) BA 44 (Heim et al., Citation2010).
4.6. The anterior insula, basal ganglia and temporal cortex
In the whole brain analysis, the region around the left frontal operculum/insula showed a further extension in the medial direction (see ) of the syntactic cluster, in particular into the anterior insula. While this region is not always reported in fMRI-studies of natural syntax, it is perhaps the most robust region where lesions cause impairments in syntactic comprehension and production (Bates et al., Citation2003; Dronkers, Citation1996). In addition, the region is often observed in the artificial grammar learning literature, in contrast to comparing syntactic violations to correct sentences (Udden & Männel, Citation2018). It may reflect a response to the salience of violations (Craig, Citation2009; Seeley et al., Citation2007), and in that case, we can speculate that phonotactic violations might be less salient, as phonological speech errors are more common than syntactic case errors in everyday language use. As can additionally be viewed in phonotactic violations did not lead to increased activation in more medial parts of the temporal lobe, suggesting possible functional specialisation on the medial-lateral axis in addition to the rostro-caudal axis, however further studies are needed to investigate whether this pattern can be observed in other experiments comparing syntax and phonology.
The left caudate nucleus, left putamen and left pallidum in the basal ganglia were also activated for syntax only (for previous similar findings see (Forkstam et al., Citation2006; Snijders et al., Citation2009)). The basal ganglia have been associated with the procedural part in the language system of the declarative/procedural model of lexicon and grammar (Ullman, Citation2001). They have been observed to underlie syntactic processing as specific syntactic operations are impaired in conditions that affect the basal ganglia such as Parkinson’s Disease and Huntington’s Disease (Ullman et al., Citation1997). However, there have also been questions raised whether this association is real for all syntactic rules (forming the past tense in English could be a possible counter example) (Longworth et al., Citation2005).
In the temporal lobe (superior temporal gyrus), the phonotactic violations produced bilateral activation, while the syntactic violation activated the left hemisphere only. This is in line with the previous literature on (sentence level) syntactic processing in the brain, which is one of the most left lateralised computations in language comprehension (Friederici & Alter, Citation2004). Phonotactic processing on the other hand is more closely related to the processing of primary auditory information and we thus expected these bilateral superior temporal activations (Gow, Citation2012; Hickok & Poeppel, Citation2007). As Gow describes, the pSTS is specifically associated with secondary steps of processing sensory input.
5. Summary and conclusion
While we observed many differences between phonotactics and syntax across language relevant regions, the whole brain analysis did not provide evidence for or against functional specialisation for syntactic vs. phonotactic structured sequence processing in LIFG [contrary to what some previous results in the literature (Bookheimer, Citation2002; Moro et al., Citation2001)]. The overlap for syntactic vs. phonotactic structured sequence processing in LIFG is a novel result that supports the relevance of the general structured sequence processing account of LIFG function, also in the context of natural language processing. In addition, with respect to existing but limited literatures on phonotactic processing and syntactic case processing, these two results provide additional evidence that phonotactics and syntactic case are processed in LIFG (along with other areas). Finally, we find a pattern of functional specialisation in the medial frontal cortex that we interpret as supporting rostro-caudal gradient in the expected direction (syntactic processing anterior to phonotactic processing) for the first time in the context of a language processing task. This novel finding extends previous knowledge on the extent of the functional organisation of the frontal lobe into a rostro-caudal gradient across cognitive domains.
Supplementary Material
Download MS Word (257.6 KB)Disclosure statement
No potential conflict of interest was reported by the author(s).
References
- Acheson, D. J., & Hagoort, P. (2014). Twisting tongues to test for conflict-monitoring in speech production. Frontiers in Human Neuroscience, 8, 206. https://doi.org/10.3389/fnhum.2014.00206
- Ardila, A., Bernal, B., & Rosselli, M. (2017). Should Broca’s area include Brodmann area 47? Psicothema, 29(1), 73–77. https://doi.org/10.7334/psicothema2016.11
- Badre, D., & D’Esposito, M. (2009). Is the rostro-caudal axis of the frontal lobe hierarchical? Nature Reviews Neuroscience, 10(9), 659–669. https://doi.org/10.1038/nrn2667
- Badre, D., & Nee, D. E. (2018). Frontal cortex and the hierarchical control of behavior. Trends in Cognitive Sciences, 22(2), 170–188. https://doi.org/10.1016/j.tics.2017.11.005
- Bahlmann, J., Blumenfeld, R. S., & D'Esposito, M. (2015). The rostro-caudal axis of frontal cortex Is sensitive to the domain of stimulus information. Cerebral Cortex, 25(7), 1815–1826. https://doi.org/10.1093/cercor/bht419
- Bahlmann, J., Schubotz, R. I., & Friederici, A. D. (2008). Hierarchical artificial grammar processing engages Broca’s area. Neuroimage, 42(2), 525–534. https://doi.org/10.1016/j.neuroimage.2008.04.249
- Bates, E., Wilson, S. M., Saygin, A. P., Dick, F., Sereno, M. I., Knight, R. T., & Dronkers, N. F. (2003). Voxel-based lesion-symptom mapping. Nature Neuroscience, 6(5), 448–450. https://doi.org/10.1038/nn1050
- Berwick, R. C., & Chomsky, N. (2017). Why only US: Language and evolution. The MIT Press.
- Bookheimer, S. (2002). Functional MRI of language: New approaches to understanding the cortical organization of semantic processing. Annual Review of Neuroscience, 25(1), 151–188. https://doi.org/10.1146/annurev.neuro.25.112701.142946
- Chomsky, N. (1956). Three models for the description of language. IEEE Transactions on Information Theory, 2(3), 113–124. http://doi.org/10.1109/TIT.1956.1056813
- Craig, A. D. B. (2009). How do you feel – now? The anterior insula and human awareness. Nature Reviews Neuroscience, 10(1), 59–70. https://doi.org/10.1038/nrn2555
- Dell, G. S., & Chang, F. (2014). The P-chain: Relating sentence production and its disorders to comprehension and acquisition. Philosophical Transactions of the Royal Society B: Biological Sciences, 369(1634), 20120394. https://doi.org/10.1098/rstb.2012.0394
- den Ouden, H. E., Daunizeau, J., Roiser, J., Friston, K. J., & Stephan, K. E. (2010). Striatal prediction error modulates cortical coupling. Journal of Neuroscience, 30(9), 3210–3219. https://doi.org/10.1523/JNEUROSCI.4458-09.2010
- Dronkers, N. F. (1996). A new brain region for coordinating speech articulation. Nature, 384(6605), 159–161. https://doi.org/10.1038/384159a0
- Fedorenko, E., Duncan, J., & Kanwisher, N. (2012). Language-selective and domain-general regions lie side by side within Broca’s area. Current Biology, 22(21), 2059–2062. https://doi.org/10.1016/j.cub.2012.09.011
- Forkstam, C., Hagoort, P., Fernandez, G., Ingvar, M., & Petersson, K. M. (2006). Neural correlates of artificial syntactic structure classification. Neuroimage, 32(2), 956–967. https://doi.org/10.1016/j.neuroimage.2006.03.057
- Friederici, A. D., & Alter, K. (2004). Lateralization of auditory language functions: A dynamic dual pathway model. Brain and Language, 89(2), 267–276. https://doi.org/10.1016/s0093-934x(03)00351-1
- Friederici, A. D., Chomsky, N., Berwick, R. C., Moro, A., & Bolhuis, J. J. (2017). Language, mind and brain. Nature Human Behaviour, 1(10), 713–722. https://doi.org/10.1038/s41562-017-0184-4
- Gow, D. W., Jr. (2012). The cortical organization of lexical knowledge: A dual lexicon model of spoken language processing. Brain and Language, 121(3), 273–288. https://doi.org/10.1016/j.bandl.2012.03.005
- Grodzinsky, Y., & Amunts, K. (2006). Broca’s region. Oxford university press.
- Hagoort, P. (2005). On broca, brain, and binding: A new framework. Trends in Cognitive Sciences, 9(9), 416–423. https://doi.org/10.1016/j.tics.2005.07.004
- Hagoort, P., & Indefrey, P. (2014). The neurobiology of language beyond single words. Annual Review of Neuroscience, 37(1), 347–362. https://doi.org/10.1146/annurev-neuro-071013-013847
- Hagoort, P., & Levelt, W. J. M. (2009). The speaking brain. Science, 326(5951), https://doi.org/10.1126/science.1181675
- Hashimoto, Y., Yokoyama, S., & Kawashima, R. (2014). Neural differences in processing of case particles in Japanese: An fMRI study. Brain and Behavior, 4(2), 180–186. https://doi.org/10.1002/brb3.201
- Heim, S., van Ermingen, M., Huber, W., & Amunts, K. (2010). Left cytoarchitectonic BA 44 processes syntactic gender violations in determiner phrases. Human Brain Mapping, 31(10), 1532–1541. https://doi.org/10.1002/hbm.20957
- Heinz, J., & Idsardi, W. (2011). Sentence and word complexity. Science, 333(6040), 295–297. https://doi.org/10.1126/science.1210358
- Hickok, G., & Poeppel, D. (2007). The cortical organization of speech processing. Nature Reviews Neuroscience, 8(5), 393–402. https://doi.org/10.1038/nrn2113
- Hsu, N. S., Jaeggi, S. M., & Novick, J. M. (2017). A common neural hub resolves syntactic and non-syntactic conflict through cooperation with task-specific networks. Brain and Language, 166, 63–77. https://doi.org/10.1016/j.bandl.2016.12.006
- Jackendoff, R. (2009). Parallels and nonparallels between language and music. Music Perception, 26(3), 195–204. https://doi.org/10.1525/mp.2009.26.3.195
- Jacquemot, C., Pallier, C., LeBihan, D., Dehaene, S., & Dupoux, E. (2003). Phonological grammar shapes the auditory cortex: A functional magnetic resonance imaging study. The Journal of Neuroscience, 23(29), 9541–9546. https://doi.org/10.1523/JNEUROSCI.23-29-09541.2003
- Jeon, H. A. (2014). Hierarchical processing in the prefrontal cortex in a variety of cognitive domains. Frontiers in Systems Neuroscience, 8, 223. https://doi.org/10.3389/fnsys.2014.00223
- Jeon, H. A., & Friederici, A. D. (2013). Two principles of organization in the prefrontal cortex are cognitive hierarchy and degree of automaticity. Nature Communications, 4(1), 2041. https://doi.org/10.1038/ncomms3041
- Kittredge, A. K., & Dell, G. S. (2016). Learning to speak by listening: Transfer of phonotactics from perception to production. Journal of Memory and Language, 89, 8–22. https://doi.org/10.1016/j.jml.2015.08.001
- Koechlin, E., & Summerfield, C. (2007). An information theoretical approach to prefrontal executive function. Trends in Cognitive Sciences, 11(6), 229–235. https://doi.org/10.1016/j.tics.2007.04.005
- Kouneiher, F., Charron, S., & Koechlin, E. (2009). Motivation and cognitive control in the human prefrontal cortex. Nature Neuroscience, 12(7), 939–945. https://doi.org/10.1038/nn.2321
- Longworth, C. E., Keenan, S. E., Barker, R. A., Marslen-Wilson, W. D., & Tyler, L. K. (2005). The basal ganglia and rule-governed language use: Evidence from vascular and degenerative conditions. Brain, 128(Pt 3), 584–596. https://doi.org/10.1093/brain/awh387
- Margulies, D. S., Ghosh, S. S., Goulas, A., Falkiewicz, M., Huntenburg, J. M., Langs, G., Bezgin, G., Eickhoff, S. B., Castellanos, F. X., Petrides, M., Jefferies, E., & Smallwood, J. (2016). Situating the default-mode network along a principal gradient of macroscale cortical organization. Proceedings of the National Academy of Sciences, 113(44), 12574–12579. https://doi.org/10.1073/pnas.1608282113
- Matchin, W., Hammerly, C., & Lau, E. (2017). The role of the IFG and pSTS in syntactic prediction: Evidence from a parametric study of hierarchical structure in fMRI. Cortex, 88, 106–123. https://doi.org/10.1016/j.cortex.2016.12.010
- Matchin, W., & Hickok, G. (2020). The cortical organization of syntax. Cerebral Cortex, 30(3), 1481–1498. https://doi.org/10.1093/cercor/bhz180
- Moro, A. (2014). Response to Pulvermuller: The syntax of actions and other metaphors. Trends in Cognitive Sciences, 18(5), 221. https://doi.org/10.1016/j.tics.2014.01.012
- Moro, A., Tettamanti, M., Perani, D., Donati, C., Cappa, S. F., & Fazio, F. (2001). Syntax and the brain: Disentangling grammar by selective anomalies. Neuroimage, 13(1), 110–118. https://doi.org/10.1006/nimg.2000.0668
- Nichols, T., Brett, M., Andersson, J., Wager, T., & Poline, J. B. (2005). Valid conjunction inference with the minimum statistic. Neuroimage, 25(3), 653–660. https://doi.org/10.1016/j.neuroimage.2004.12.005
- Nieuwland, M. S., Martin, A. E., & Carreiras, M. (2012). Brain regions that process case: Evidence from Basque. Human Brain Mapping, 33(11), 2509–2520. https://doi.org/10.1002/hbm.21377
- Papitto, G., Friederici, A. D., & Zaccarella, E. (2020). The topographical organization of motor processing: An ALE meta-analysis on six action domains and the relevance of Broca’s region. Neuroimage, 206, 116321. https://doi.org/10.1016/j.neuroimage.2019.116321
- Pickering, M. J., & Garrod, S. (2007). Do people use language production to make predictions during comprehension? Trends in Cognitive Sciences, 11(3), 105–110. https://doi.org/10.1016/j.tics.2006.12.002
- Pulvermuller, F. (2014). The syntax of action. Trends in Cognitive Sciences, 18(5), 219–220. https://doi.org/10.1016/j.tics.2014.01.001
- Sakai, K., & Passingham, R. E. (2003). Prefrontal interactions reflect future task operations. Nature Neuroscience, 6(1), 75–81. https://doi.org/10.1038/nn987
- Seeley, W. W., Menon, V., Schatzberg, A. F., Keller, J., Glover, G. H., Kenna, H., Reiss, A. L., & Greicius, M. D. (2007). Dissociable intrinsic connectivity networks for salience processing and executive control. Journal of Neuroscience, 27(9), 2349–2356. https://doi.org/10.1523/JNEUROSCI.5587-06.2007
- Segaert, K., Menenti, L., Weber, K., Petersson, K. M., & Hagoort, P. (2012). Shared syntax in language production and language comprehension – An FMRI study. Cerebral Cortex, 22(7), 1662–1670. https://doi.org/10.1093/cercor/bhr249
- Snijders, T. M., Vosse, T., Kempen, G., Van Berkum, J. J., Petersson, K. M., & Hagoort, P. (2009). Retrieval and unification of syntactic structure in sentence comprehension: An FMRI study using word-category ambiguity. Cerebral Cortex, 19(7), 1493–1503. https://doi.org/10.1093/cercor/bhn187
- Tzourio-Mazoyer, N., Landeau, B., Papathanassiou, D., Crivello, F., Etard, O., Delcroix, N., Mazoyer, B., & Joliot, M. (2002). Automated anatomical labeling of activations in SPM using a macroscopic anatomical parcellation of the MNI MRI single-subject brain. Neuroimage, 15(1), 273–289. https://doi.org/10.1006/nimg.2001.0978
- Udden, J., & Bahlmann, J. (2012). A rostro-caudal gradient of structured sequence processing in the left inferior frontal gyrus. Philosophical Transactions of the Royal Society B: Biological Sciences, 367(1598), 2023–2032. https://doi.org/10.1098/rstb.2012.0009
- Udden, J., de Jesus Dias Martins, M., Zuidema, W., & Tecumseh Fitch, W. (2020). Hierarchical structure in sequence processing: How to measure it and determine Its neural implementation. Topics in Cognitive Science, 12(3), 910–924. https://doi.org/10.1111/tops.12442
- Udden, J., & Männel, C..2018). Artificial grammar learning and its neurobiology in relation to language processing and development. In S.-A. Rueschemeyer & M. G. Gaskell (Eds.), The Oxford Handbook of Psycholinguistics (2nd ed, pp. 755–783). Oxford University Press.
- Ullman, M. T. (2001). The declarative/procedural model of lexicon and grammar. Journal of Psycholinguistic Research, 30(1), 37–69. https://doi.org/10.1023/A:1005204207369
- Ullman, M. T., Corkin, S., Coppola, M., Hickok, G., Growdon, J. H., Koroshetz, W. J., & Pinker, S. (1997). A neural dissociation within language: Evidence that the mental dictionary is part of declarative memory, and that grammatical rules are processed by the procedural system. Journal of Cognitive Neuroscience, 9(2), 266–276. https://doi.org/10.1162/jocn.1997.9.2.266
- Vaden, K. I., Jr., Piquado, T., & Hickok, G. (2011). Sublexical properties of spoken words modulate activity in Broca’s area but not superior temporal cortex: Implications for models of speech recognition. Journal of Cognitive Neuroscience, 23(10), 2665–2674. https://doi.org/10.1162/jocn.2011.21620
- Vosse, T., & Kempen, G. (2000). Syntactic structure assembly in human parsing: A computational model based on competitive inhibition and a lexicalist grammar. Cognition, 75(2), 105–143. https://doi.org/10.1016/S0010-0277(00)00063-9
- Yokoyama, S., Maki, H., Hashimoto, Y., Toma, M., & Kawashima, R. (2012). Mechanism of case processing in the brain: An fMRI study. PLoS One, 7(7), e40474. https://doi.org/10.1371/journal.pone.0040474