ABSTRACT
This fMRI study explored the relationship between social interactions and neural representations of emotionality in a foreign language (LX). Forty-five late learners of Japanese performed an auditory Japanese lexical decision task involving positive and negative words. The intensity of their social interactions with native Japanese speakers was measured using the Study Abroad Social Interaction Questionnaire. Activity in the left ventral striatum significantly correlated with social interaction intensity for positive words, while the right amygdala showed a significant correlation for negative words. These results indicate neural representations of LX emotional words link with the intensity of social interactions. Furthermore, LX negative words activated the left inferior frontal gyrus more than positive and neutral words, suggesting greater cognitive effort for processing negative words, aligning with a bias in adult social interactions towards more positively-valenced language. Overall, our findings underscore the importance of social interaction experiences in the processing of LX emotional words.
Introduction
An understanding of emotional expression and perception is essential for human life. Humans generally understand the emotions of people with whom they interact daily, whether in a first language (L1) or foreign language (LX).Footnote1 However, LX learners and users may struggle to perceive the emotional weight (emotionality) of LX discourse because they lack actual emotional experience in that language (Caldwell-Harris et al., Citation2011; Graham et al., Citation2001; Rintell, Citation1984). Therefore, an LX is often reported to be less emotional than an L1. The lack of emotionality in late LX learners, especially individuals who learned the LX in a classroom setting, is primarily related to the different contexts in which the languages are learned. L1 acquisition occurs naturally through social interactions with caregivers and other L1 users in various real-world contexts that can be highly emotional. As noted in the emotional contexts of learning theory (Harris et al., Citation2006), exposure to and participation in LX social interactions in real-world contexts (hereafter referred to as immersion/social interactions) play essential roles in the perception of emotional weight in the LX.
Despite the importance of social interactions in real-world contexts during language acquisition, there is limited research on the effects of immersion/social interactions on the neural representation of LX emotional words in late LX learners. Some neuroimaging studies have explored the neural underpinnings and processing differences of emotional words across bilinguals’ languages. These studies have uncovered distinct neural pathways for processing emotional versus neutral words in L1 (see Citron, Citation2012, for a review on L1 studies) as well as in LX (Chen et al., Citation2015; Rosselli et al., Citation2017). Specifically, the emotion effect appears to be more pronounced in the L1, where a distinct neural network is engaged for emotional as opposed to neutral words (see Kazanas et al., Citation2019 for a review). However, findings from bilingual studies have been inconsistent, likely due to large individual differences among LX learners in factors such as age of acquisition, proficiency level, and the intensity of social interaction during learning.
Although many studies have suggested the important role of social experience in LX emotional word processing (see Pavlenko, Citation2008, Citation2012, for reviews), few neuroimaging studies have investigated the effect of social interaction. In contrast, previous studies have reported that the age of acquisition (early vs. late) and proficiency level (high vs. low) are two major determinants of the neural correlates of LX processing (see Perani & Abutalebi, Citation2005; Sulpizio et al., Citation2019, for reviews). However, recent brain imaging studies focused on social learning have shown that acquiring LX vocabulary through social interactions, whether real or simulated, enhances semantic representations of the LX in the brain (e.g. Jeong et al., Citation2021; see Li & Jeong, Citation2020, for a review). As a result, these representations in LX become more similar to those of the L1 (Jeong et al., Citation2010). The present study explores the relationship between social interactions and LX emotionality perception by recruiting late adult learners who study abroad and experience varying intensities of social interactions.
Previous studies of bilingual emotion perception
Previous studies on the processing of emotional expressions in bilingual individuals have highlighted the age of acquisition and level of language proficiency as the most critical determinants of the ability to perceive the emotionality of language (Bloom & Beckwith, Citation1989; Caldwell-Harris et al., Citation2012; Eilola et al., Citation2007). However, to fully interpret findings on emotional expression processing, one must consider the extent of social interactions and the broader LX environment. For example, Graham et al. (Citation2001) reported that an increase in LX proficiency did not improve the ability to perceive emotionality in the LX. They recruited L1 users and LX learners (with low and high levels of proficiency in the LX) and asked both groups to rate the emotionality of LX expressions. There was a significant difference in the ability to identify emotional expressions between L1 users and LX learners. However, among LX learners, proficiency levels did not yield any significant differences. The authors speculated that the inability of both low- and high-proficiency LX learners to judge emotional weight in the LX may have been related to a lack of immersion in the LX environment (i.e. authentic social interactions with L1 users). In contrast, Ferré et al. (Citation2010) found that when highly proficient LX users performed recall tasks with emotional words, emotionality perceptions were identical between L1 and LX. Notably, the participants in the study by Ferré et al. (Citation2010) were Spanish-Catalan bilinguals who had acquired their LX in an immersive environment. This may explain the discrepancy between their results and those of Graham et al. (Citation2001).
Previous physiological studies involving skin conductance response (SCR) measurements have also provided evidence that LX emotionality perception is highly sensitive to the frequency or extent of LX usage in daily life (Ayçiçegi & Harris, Citation2004; Caldwell-Harris et al., Citation2011; Harris et al., Citation2003). If LX users frequently use certain emotional expressions in their LX during daily life, they can have stronger emotional responses in their LX compared to their L1 (Caldwell-Harris et al., Citation2011). For example, Caldwell-Harris et al. (Citation2011) recruited Chinese-English bilinguals living in the U.S. The bilingual participants were asked to rate the emotional intensity of auditory phrases in both L1-Chinese and LX-English while their SCRs were recorded. Although there were no differences in the ratings of emotional intensity between LX and L1 for expressions of endearment, larger SCRs were found for LX-English expressions of endearment (i.e. “I miss you” and “I love you”) than for L1-Chinese ones, indicating that LX endearment elicited greater emotional arousal. These findings imply that the amount and quality of daily social interactions in the LX can influence how emotional expressions are processed.
Although intense social interactions often lead to high levels of proficiency in an LX, it is important to note that high LX proficiency does not invariably imply a history of intense social interactions (for a review, see Caldwell-Harris, Citation2014). This distinction arises because language proficiency does not directly reflect the learning processes, such as the intensity of immersion or social interactions. Instead, it reflects the outcomes of language learning, such as fluency and metalinguistic knowledge. LX users who achieve the same learning outcomes (e.g. equivalent proficiency in metalinguistic knowledge) can still differ in how they perceive the emotionality of the LX due to variations in their learning experiences. In the study by Dewaele (Citation2008), multilingual individuals perceived the emotional weight of “I love you” differently depending on how they acquired the LX. Specifically, those who learned the LX in the classroom were likely to perceive the phrase as having less emotional weight in their LX compared to those who learned through social interactions, even when both groups had comparable levels of LX proficiency.
Based on a similar rationale, Dewaele et al. (Citation2021) investigated differences in self-reported emotional reactions in English between 271 British English L1 users and 553 English LX users (Greeks and Hungarians living in their home country). A four-item composite score was used: frequency of feeling emotional when watching the news, frequency of feeling emotional when watching a film, frequency of laughter when watching a funny film, and degree of trust in the news. The English LX users scored significantly lower on the composite measure of emotional reactions compared to the L1 users. However, it was the frequency of watching television in English that predicted the emotional reactions among LX users, not their proficiency as measured by a lexical decision task. This suggests that simply watching films and television in the LX may provide LX users with a moderately immersive “virtual” experience, allowing them to understand how emotions are communicated in that language. Overall, the data indicate that exposure to and participation in authentic social interactions in an LX, independent of proficiency and age of acquisition, may improve the ability to perceive emotionality in that language.
The findings from the aforementioned bilingual studies indicate that language proficiency is not the sole factor influencing emotional processing in LX. An individual's social experience, including the extent and context of target language usage, also appears to exert a significant influence. Accordingly, it is essential to investigate whether the qualitative and quantitative aspects of language use correlate with the neural mechanisms underlying the processing of LX emotion words, particularly among LX learners who acquired their second language in later stages of life.
Previous neurobiological studies of emotional word processing
Thus far, we have reviewed behavioural studies on LX and L1 emotionality perception, establishing that authentic language use in social contexts could be an influential factor in the processing of LX emotional words. However, few bilingual neuroimaging studies have examined the neural basis of LX emotionality perception in the context of social interactions.
Most previous neuroimaging studies examined neural correlates of L1 and LX emotional processing by comparing positive, negative, and neutral words (see Citron, Citation2012, for a review on L1 studies; see Rosselli et al., Citation2017; and Kazanas et al., Citation2019, for reviews on bilingual studies). There were two major findings in the previous studies. First, the difference between emotional words and neutral words (emotion effect) is more salient and consistent in bilinguals’ L1 than LX: L1 emotional words cause greater activity in emotion-related brain areas than L1 neutral and LX emotional words; LX emotional words recruit more lexicosemantic and cognitive control areas when compared to LX neutral words because of lower proficiency or less emotional experience in the LX. For example, Chen et al. (Citation2015) investigated how late Chinese-English bilinguals process emotional words in their two languages via lexical decision tasks. They observed that the L1 emotional word processing exhibited greater depth and displayed more distinct patterns than LX emotional word processing. Second, monolingual studies have reported that there is a difference in how negative and positive words are processed in the brain (Citron, Citation2012; Hamann & Mao, Citation2002; Nakic et al., Citation2006). For instance, Hamann and Mao (Citation2002) explored the neural correlates of L1 negative and positive words through comparison with L1 neutral words. They found that positive words exclusively increased activity in the dorsal and ventral striatum, which is presumably related to social reward and positive emotion processing; negative words caused greater activation of the left amygdala than neutral words.
Another intriguing observation in the realm of bilingual neurobiological research is that negative words may be more likely to elicit a less emotional response compared with positive words, due to a phenomenon known as positivity bias in social interactions. The concept of positivity bias is grounded in the Pollyanna hypothesis, which posits that human interactions generally have an emotional positivity bias, regardless of whether they occur in an individual’s L1 or LX (Boucher & Osgood, Citation1969). Accordingly, positive emotions are more accessible and more commonly experienced than negative emotions in typical social interactions.
In line with this, some bilingual studies have shown that this positivity bias influences emotional word processing (Conrad et al., Citation2011; Wu & Thierry, Citation2012). For example, Conrad et al. (Citation2011) conducted an event-related potential method to study Spanish-German bilinguals and identified early posterior negativity and a late positive complex, both indicative of emotion-related processing, for positive words in both L1-Spanish and LX-German, compared to neutral words. Intriguingly, such emotional effects were absent for these LX negative words. Conrad et al. interpreted this lack of emotional effect in LX negative words as a consequence of positivity bias in social interactions.
To gain a better understanding of the role of social interactions in the acquisition of LX emotionality, and of the neural mechanisms that underlie the effects of positivity bias, it is essential to measure the quantity and quality of social interactions in each learner. Additionally, the effects of these interactions on the brain activity during LX emotional word processing should be assessed.
Remaining issues and aims of the present study
Our literature review suggests that social interactions with native speakers can enhance LX learners’ ability to perceive emotionality in the LX. Given its potential impact, the intensity of social interaction deserves greater consideration in future studies in emotionality perception in LX. If individual experiences through authentic social interactions are critical for understanding the emotional weight of language, then increased social interactions in an immersive environment should lead to improved emotional processing in LX.
This study first examined whether and how individual experience accrued through authentic social interactions (i.e. exposure to and participation in LX social interactions with native speakers in real-world contexts) is linked to the neural mechanisms underlying the processing of emotionality in LX words. According to the positivity bias hypothesis (Boucher & Osgood, Citation1969), positive emotions are more accessible, and more likely to be acquired through social interactions, compared with negative emotions; thus, we examined how LX social interactions correlate with brain activation during the processing of LX positive and negative emotional words. To address these two research questions, the present study used functional magnetic resonance imaging (fMRI). We recruited L1 Mandarin Chinese speakers who were late learners of Japanese, began learning Japanese with a mean starting age of 19 years, and were residing in Japan at the time of the experiment. These participants had extensive experience in various social interactions in the LX, which for this study was Japanese. While inside an fMRI scanner, the participants were asked to perform an auditory lexical decision task, which included LX words with different emotional valences (i.e. positive, negative, and neutral words) and nonwords.
Moreover, a specialised social network questionnaire was used to comprehensively measure the participants’ use of LX in social contexts, including the amount of language use, usage scenarios, and interlocutors of language use (see Methods for details). The current study addressed the core research question of whether the intensity of social interaction, as determined by questionnaire scores, is associated with the neural mechanisms involved in the processing of emotional words in LX. The level of LX proficiency of each participant was also assessed with a Japanese language proficiency test (Eckes & Grotjahn, Citation2006; Shin, Citation1990; Suzuki, Citation2015). Drawing on prior research, we hypothesised that the intensity of social interaction, rather than linguistic proficiency, would show a detectable correlation with brain activity in emotion-related regions (e.g. the ventral striatum and amygdala) during the processing of emotional words in LX. Furthermore, we posited that such correlation with the intensity of social interaction would be more pronounced when processing positive words than negative words, due to a presumed positivity bias.
Materials and methods
Participants
Forty-five Chinese L1 speakers participated in the experiment. The final data analysis included 41 participants (mean age = 25 years, standard deviation (SD) = 2.47 years; 26 female); some participants were excluded based on task accuracy rate and head movement criteria (see the ‘Data analyses’ and ‘Results’ sections). The participants were fluent in Mandarin Chinese and had learned English in middle and high school. They had studied Japanese primarily in classroom settings in mainland China (mean age at the start of learning Japanese = 19.3 years, SD = 2.85 years) and came to Japan for university or postgraduate study. According to the participants’ self-reports, Japanese was their main language while living in Japan. Their length of residence in Japan ranged from 17 to 70 months (mean = 31.4, SD = 16.44).
The participants had all achieved the N1 level on the Japanese Language Proficiency Test (mean score = 131.92, SD = 22.11) administered by the Japan Foundation and Japan Educational Exchanges and Services, indicating that they were highly proficient in Japanese (Nishizawa et al., Citation2022). We also measured their metalinguistic proficiency (i.e. vocabulary, grammar, and reading comprehension ability) at the time of the experiment using the Japanese C-test (Shin, Citation1990). The C-test is a commonly used objective test measuring the metalinguistic proficiency of a second language in written modality (e.g. Eckes & Grotjahn, Citation2006; Suzuki, Citation2015). In the Japanese C-test, participants are required to fill in missing words that have been deleted from a short text. In order to successfully complete the test, the participants must have a good understanding of Japanese grammar and syntax, a wide range of vocabulary, and the ability to comprehend written text and deduce missing information based on context. Therefore, the C-test scores are a good indicator of the participants’ metalinguistic ability.
All participants were right-handed with normal or corrected-to-normal vision. None of participants had a history of neurological or mood disorders. Handedness was evaluated using the Edinburgh Handedness Inventory (Oldfield, Citation1971). Written informed consent was obtained from each participant. This study was approved by the Ethical Committee of the Institute of Development, Aging, and Cancer, Tohoku University.
Questionnaire
The present study used the Study Abroad Social Interaction Questionnaire (SASIQ) developed by Dewey and colleagues (Dewey et al., Citation2012; Dewey et al., Citation2013). This questionnaire has been validated and is commonly used to investigate the relationship between social interaction and language gain in immersive LX settings.
The development of SASIQ was based on the social interactionist approach to second language acquisition (Gass & Selinker, Citation1983, pp. 167–193), which posits that social interactions with target language speakers can enhance language learning by providing a linguistically rich environment, opportunities for negotiating meaning, receiving feedback, and practicing language skills. Dewey et al. (Citation2012) utilised the SASIQ questionnaire to investigate the impact of social interaction (i.e. language use and social network development) on LX speaking proficiency gains during study abroad. They found that hours spent speaking with close native speaker friends were the most significant predictor of gains in speaking proficiency. Other studies also support the idea that language use with close friends can facilitate language learning (Pellegrini et al., Citation2002). For instance, a study by Pellegrini et al. (Citation2002) discovered that language learning was more effective between close friends than between unfamiliar peers, and emotional terms were used more often between friends than among unfamiliar peers during language learning. These findings suggest that the closeness of the relationship with target language speakers and the amount of language use are crucial components of the social interaction effect on language learning.
The Study Abroad Social Interaction Questionnaire, developed by Dewey et al. (Citation2012), includes a series of questions designed to capture both the quantity and quality of social interactions (i.e. social interaction intensity). This assessment identified the types of social networks participants had formed in Japan (e.g. “How many social groups are you a part of?”), their social qualities (e.g. “How close are you with each contact?”), and the amount of Japanese used within their social networks. Participants were asked to list contacts with whom they regularly spoke Japanese; rate the closeness with each listed contact on an eight-point Likert scale, ranging from “1” (acquaintance) to “8” (very close friend), and estimate how many hours per week they communicated with each listed contact in Japanese.
We used a composite measure, the total hours of communication with close friends (contacts with a score ≥ 5 on the closeness rating task), as an indicator of social interaction intensity in our study. Specifically, we calculated the total minutes of communication between participants and each of their close friends with a closeness score equal to or greater than 5, and this sum value represents the participants’ social interaction intensity. This measure reflects both the quantity and quality of LX use and is based on previous research that reported a positive correlation between language gains and communication with close friends in the target language (e.g. Dewey et al., Citation2012). We chose to create this composite measure because interactions with close friends are more likely to involve emotional experiences than interactions with acquaintances (e.g. a convenience store clerk or swimming pool receptionist, rated as ≤ 4 in the closeness rating task). Planalp and Benson (Citation1992) note that conversational topics are broader, and communication is more flexible, efficient, and emotional with greater relationship closeness.
The reported total minutes of communication with close friends varied widely among participants (Mean = 503, SD = 516, ranging from 0 to 2040, skewness = 1.2), which is consistent with our interest. Higher scores indicated that a participant had formed more intense social relations with Japanese speakers and presumably had more opportunities to use Japanese in various emotional contexts. See Supplementary Table 1 for questionnaire item statistics.
Stimuli
To select suitable auditory word stimuli for the fMRI experiment, we conducted two pilot experiments: the first experiment invited native speakers to score the vocabulary variables, and the second experiment invited Japanese learners to eliminate the difficult words. The following procedures were used to prepare the stimulus. First, we selected 572 words based on a textbook for learners of Japanese (A Practical Guide to Japanese Expressions for Daily Life; Ogasawara, Citation2003). The textbook is tailored for LX learners and comprises only words that are frequently used in daily life. Next, 457 candidate words were first selected based on (1) the absence of homophones, (2) the absence of unrelated multiple meanings, and (3) the frequency of words. Then two colleagues (one native Japanese speaker and one advanced LX Japanese user) preselected the words based on their intuitive valence ratings, resulting in a final stimulus list of 400 words for participants. Then using these words, twenty Japanese L1 speakers (mean age = 22.3 years, SD = 2.49 years; 10 female and 10 male) rated 400 Japanese words according to two emotion-related variables: arousal (i.e. the excitement elicited by a word) and valence (i.e. the pleasant feelings elicited by a word) on a 7-point Likert scale (see the Supplementary Materials for the instructions for arousal and valence rating questionnaires). The words included nouns, verbs, adjectives, verbal-adjectives, adverbs, as well as words that could function either as nouns or verbs, and words that could serve as either nouns or verbal-adjectives (see the Supplementary Materials S-Table 2 for the proportions of each word class), and their frequency were obtained from the Balanced Corpus of Contemporary Written Japanese (https://ccd.ninjal.ac.jp/bccwj/en/).
Using two-tailed one-sample t-tests on valence rating scores for each word, we categorised the words (Gotoh & Ohta, Citation2001; Higami et al., Citation2015; Honma, Citation2014). Words that significantly deviated from the neutral Likert scale value of 4 (p < .01) were labelled as emotional words. Within this set, words with a mean valence score surpassing 4 were deemed positive, while those below 4 were classified as negative. Words not showing a significant deviation from the neutral score of 4 (p > .05) were categorised as neutral words. To ensure a balanced presentation of emotional arousal across different word categories, we handpicked 180 words (comprising 60 positive, 60 neutral, and 60 negative words) out of the initial 400 candidates. We also created 100 meaningless but pronounceable nonwords by randomly combining Japanese kana sounds. Nonwords were used as a control condition to minimise possible effects caused by varying proficiency and social experiences in language learning, as they have no meaning and were equally new to all participants. Two Japanese native speakers checked the nonword stimuli, selecting 80 for the experiments based on the number of syllables and absence of word meanings. The words were spoken by a female native Japanese speaker with no dialectal accent, in a neutral tone in a soundproof room, and recorded using the ICD-SX1000 device (Sony, Tokyo, Japan). All auditory stimuli were recorded as 14-bit detailed WAV files, sampled at 44.1 kHz, and normalised to a mean of 75 dB using Audacity software. The length of each auditory word was approximately 1 s (mean = 0.942 s; SD = 0.13 s). To ensure comprehension among Japanese learners, another pilot test was conducted with 11 Chinese learners. These learners were similar to the fMRI experimental group in age, Japanese proficiency, and experience in learning Japanese. Participants were instructed to listen to each word and immediately indicate their understanding by pressing “1” for “yes” or “0” for “no”. We selected words that were recognised by at least 8 of the 11 participants.
Based on this procedure, we selected 40 positive words, 40 neutral words, 40 negative words, and 80 nonwords for the fMRI experiment. To verify the similarity of other lexical variables across the three categories of real words, we performed a one-way analysis of variance (ANOVA). The results indicated no significant differences in the number of syllables among the four categories of words; there was a difference in the emotional valence across three types of real words, and the arousal level for neutral words was lower than for positive and negative words, which is consistent with the goal of this study (see ).
Table 1. Descriptive statistics for the task stimuli.
Task and procedure
We used an event-related design, in which auditory words in four categories were randomly presented. The participants performed the lexical decision task while inside the fMRI scanner; they were asked to judge whether each word was real or not. Stimuli of all word types were presented randomly across participants. A trial lasted for 4000 ms, including the presentation of the audio stimulus for approximately 1000 ms and the time to press the response button. The intertrial-interval was randomly varied between 1000 and 4000 ms. The participants were instructed to respond as quickly and accurately as possible by pressing one of two buttons corresponding to “yes” (right index finger) and “no” (right middle finger). The task was designed using E-Prime 3.0 software (Psychology Software Tools, Inc., Pittsburgh, PA, USA) and divided into two sessions. Each participant completed two sessions with a rest between sessions; each session consisted of 100 trials and lasted for 11 min 37 s. During fMRI scanning, the participants were asked to fix their gaze on a cross (+) presented at the center of the visual field on the screen although there was no visual stimulation. The auditory words were delivered via fMRI-compatible optical headphones with active noise cancellation (OptoActive; Optoacoustics Ltd., Or Yehuda, Israel), which reduced the scan noise level by approximately 60 dB and allowed participants to hear the auditory stimuli well during scanning. All participants completed 20 practice trials prior to the fMRI scan.
After scanning, a post-questionnaire was administered to measure participants’ perceptions of the semantic and affective meanings of the LX (Japanese) words. The participants were asked to listen to the word stimuli again (40 positive, 40 neutral, and 40 negative words) and rate how pleasing they were on a 7-point scale (1 = unpleasing, 4 = neutral, 7 = pleasing). To confirm that they fully understood the meanings of the words, the participants were also asked to write the Chinese translation of each Japanese word (translation task). The mean accuracy of the translation task was 93.41% (SD = 6.88%), indicating that the participants had a good understanding of the words used. The valence rating scores of the participants were closely matched to those of Japanese L1 speakers in the pilot experiment. The positive, neutral, and negative word classification accuracy rates were 85%, 85% and 95%, respectively (based on the ratings by Japanese L1 speakers in the pilot experiment). Moreover, the participants considered both positive and negative words to be significantly more emotionally valenced than neutral words (p < .001 for both comparisons).
Data analyses
Data from forty-one of the forty-five participants were included in the final analysis. Three participants were excluded from the analysis because their accuracy rates in the lexical decision task and translation task were below 90%. One participant exhibited excessive head movement exceeding 4 mm during fMRI scanning.
Behavioural data analysis
The accuracy rates and reaction times (RTs) for the lexical decision task were recorded during the fMRI scan. Initially, to compare the effect of type of words on behavioural performance, one-way repeated ANOVA was conducted separately on RTs and accuracy rates in Pos, Neg, Neu, and Non conditions. Second, to examine the relationship between LX proficiency level and social interaction intensity, correlation analysis was carried out.
Third, a multiple regression analysis was performed to explore the extent to which social interaction intensity and LX proficiency level could predict RTs or accuracy rates in the four conditions (Pos, Neg, Neu, and Non) during the lexical decision task. In this model, RTs or accuracy rates of the four types of words were the dependent variables, while social interaction intensity or LX proficiency level were included as independent variables. The multicollinearity assumption was satisfied, as indicated by the Variance Inflation Factor (VIF) being less than 10 and the tolerance being greater than .02.
fMRI data acquisition and preprocessing
An echo-planar magnetic resonance imaging sequence (Achieva dStream 3.0T; Philips, Amsterdam, Netherlands) was used to acquire 32 gradient-echo images with the following settings: echo time = 30 ms, flip angle = 80°, slice thickness = 3.0 mm, slice gap = 0 mm, slice orientation = transverse, slice acquisition order = sequential (ascending), phase encoding direction = A/P, field of view = 192 mm, and matrix size = 64 × 64. The images covered the entire brain, with a repetition time of 2,000 ms. After excluding three dummy scans performed due to the T1 saturation effect, 315 volumes were obtained for each participant and session.
Statistical Parametric Mapping (SPM12) software (Wellcome Department of Imaging Neuroscience, London, UK), run in the MATLAB environment (MathWorks, Natick, MA, USA), was used to preprocess the data for subsequent statistical analysis. Preprocessing involved the following steps. First, to correct for temporal errors and misalignment in functional images, all functional volumes for each participant were time-corrected using the 16th slice as a reference and then spatially realigned to the first echo-planar imaging volume. Second, for the alignment of functional and anatomical images, the anatomical T1 image was co-registered to the mean functional image generated during the realignment process. It was then normalised to Montreal Neurological Institute (MNI) space, and deformation field parameters were obtained from this normalised anatomical T1 image. Finally, all echo-planar images were spatially smoothed using a Gaussian kernel with a full-width at half-maximum (FWHM) of 8 mm to enhance the signal.
fMRI data analyses
We adopted a conventional two-level approach to analyze the event-related fMRI data using SPM12. At the first level, we estimated the extent of activation by conducting a voxel-by-voxel multiple regression analysis of time courses. For each participant, a general linear model was constructed to analyze the hemodynamic response. We anticipated that brain activity would peak once participants comprehended each word; therefore, we modelled the hemodynamic response function based on the timing of the response to auditory words as the trial onset time. Four regressors for each type of wordsFootnote2 (negative word: Neg, positive word: Pos, neutral word: Neu, nonword: Non) were created for each session to model hemodynamic responses. We modeled incorrect response trials across all types of words as a separate no interest regressor. Furthermore, we modeled six movement parameters (three translations and three rotations) as regressors of no interest. Additionally, we incorporated the word frequency of each word in the Neg, Pos, and Neu conditions as a parametric modulation to control for its effect on brain activation. We generated contrast images for each participant by comparing real words and nonwords (Neg > Non, Pos > Non, Neu > Non), emotional words versus neutral words (Neg > Neu and Pos > Neu), and positive words versus negative words (Pos > Neg and Neg > Pos). Finally, we conducted statistical inference on contrasts of parameter estimates using a random effects model one sample t-test at the second level analysis.
In the second-level group analysis, we first compared each word type with nonwords ([Neg > Non], [Pos > Non], and [Neu > Non]) to explore the brain activation in each word type. Then, to explore differential brain activation between real word types, we tested each contrast of [Neg vs. Neu], [Pos vs. Neu], [Neg vs. Pos], and [Pos vs. Neg]. In the brain regions observed in the whole-brain analysis, when it was necessary to compare the activation profile among conditions, we performed a one-way repeated ANOVA analysis using the mean parameter estimates in the observed clusters from each contrast ([Neg > Non], [Pos > Non], and [Neu > Non]) for each participant with the Marsbar toolbox (Brett et al., Citation2002).
Second, to investigate the effect of social interaction intensity and LX proficiency level on each type of word processing, we performed a voxel-wise multiple regression analysis with two independent variables (the social interaction intensity scores and the LX proficiency scores) of each participant at the whole-brain level. These two independent variables were entered as covariates for each contrast of [Neg > Non], [Pos > Non], and [Neu > Non] in SPM 12 model matrix. Since neutral words are also likely to be affected by social interaction and LX proficiency (Jeong et al., Citation2021; see Li & Jeong, Citation2020 for a review), testing these contrasts allows us to examine how the intensity of social interaction and the level of LX proficiency associate with brain activity during each type of word processing at the whole-brain level, and in which brain regions this correlation occurs, since nonwords were equally novel to all participants and thus have no social interaction effect or proficiency effect.
The statistical threshold was established at p < .05 using multiple comparison correction with cluster size at the whole brain-level (Slotnick, Citation2017). We utilised Monte Carlo simulation with 10,000 iterations on a whole-brain grid (64 × 64 × 32) smoothed with an 8 mm FWHM Gaussian kernel, yielding a voxel threshold of p < .001, corrected for multiple comparisons to p < .05 with a cluster extent threshold of 45 voxels. Additionally, for regions where we had a priori hypotheses related to emotion (e.g. bilateral amygdala), we applied small volume correction (SVC) using anatomical masks for voxel-level multiple comparisons, with a threshold of p < .05 after Family-Wise Error (FWE) correction. The Wake Forest University PickAtlas toolbox (Maldjian et al., Citation2003, Version 3.0.5) was used to create anatomical masks through automated anatomical labelling (Tzourio-Mazoyer et al., Citation2002). Activation peak coordinates were reported in Montreal Neurological Institute space, and activated brain regions were identified using the automated anatomical labelling atlas in SPM12. To illustrate the activation profile in observed brain areas, we extracted mean parameter estimates from each contrast ([Neg > Non], [Pos > Non], and [Neu > Non]) for each participant using the Marsbar toolbox (Brett et al., Citation2002).
Results
Behavioural results
shows the mean accuracy rates and RTs for all conditions. One-way repeated ANOVA revealed no significant effect among word conditions (Pos, Neg, Neu, Non) on accuracy rate, F(3, 120) = .48, p = .64, η2 = .01. There was a significant effect for reaction time, F(3, 120) = 30.03, p < .001, η2 = .43. Post hoc comparisons with Bonferroni correction indicated that reaction time of negative words was significantly slower than neutral words, t = 5.45, d = 62.22, p < .001 and positive words, t = 2.82, d = 35.02, p = .015; positive words were slower than neutral words, t = 2.60, d =27.19, p = .015. Nonwords were significantly slower than negative words, t = 4.58, d = −128.46, p < .001, positive words, t = 5.87, d = −163.49, p < .001, and neutral words, t = 6.39, d = −190.68, p < .001.
Table 2. Mean accuracy rates and reaction times.
Pearson correlation analysis examined the relationship between individual scores of social interaction intensity and LX proficiency (i.e. Japanese c-test) and revealed no significant correlation between the two factors, r = −.292, p = .064.
A multiple regression analysis was conducted to determine whether social interaction intensity and LX proficiency level predicted behavioural performance (RTs and accuracy rate) in the lexical decision task. Social interaction intensity was not significantly correlated with either reaction time [R2 = .023, adjusted R2 = −.086, F(4, 36) = .211, p = .931] or accuracy rate [R2 = .120, adjusted R2 = .022, F(4, 36) = 1.226, p = .317] in any condition. There was also no significant relationship between LX proficiency level and reaction time in any condition [R2 = .008, adjusted R2 = −.102, F(4, 36) = .071, p = .99]. In contrast, LX proficiency accounted for significantly better accuracy rates [R2 = .300. adjusted R2 = .222, F(4, 36) = 3.853, p = .01]. Among the four conditions, only the accuracy rate for neutral words showed a significant correlation with LX proficiency level [95% CI [.27, .97], β = .537, t = 3.613, p < .001], but there was no such correlation for positive words [95% CI [−.28, .32], β = .025, t = .141, p = .889] or negative words [95% CI [−.48, .32], β =−.07, t = −.39, p = .702] or nonwords [95% CI [−.40, .24], β = −.08, t = −.53, p = .599].
fMRI results
Effect of type of words
In comparisons of emotional word and the neutral word conditions, the negative words enhanced significantly greater activation in the left triangular part of the IFG than the neutral words (see , ). In contrast, no significant differential activation was observed on the whole brain level between positive and neutral words, as well as between positive and negative words. To clarify activation patterns in the left IFG across three conditions, we extracted mean parametric estimates in observed cluster from each contrast of Neg-Non, Pos-Non, and Neu-Non for each participant. One-way repeated ANOVA revealed significant differential activation among types of words [F(2, 80) = 9.00, p < .001, η2 = 0.18]. Post hoc comparisons showed that negative words (mean ± SD = 1.57 ± 1.47) enhanced significantly greater activation in this area than positive (mean ± SD = .97 ± 1.38, t = 2.63, d = .59, p = .043) and neutral words (mean ± SD = 0.68 ± 1.15, t = 4.11, d = .89, p < .001). No difference was observed between positive words and neutral words (p = .15) (see ).
Figure 1. Brain area showing higher activation for negative versus neutral words. Greater activity in the triangular part of left IFG (inferior frontal gyrus) was observed under the Negative > Neutral contrast. The statistical threshold was set at FWE-corrected p < .05 with cluster-level in the whole brain. To further clarify the activation pattern in the left IFG among conditions, a one-way repeated ANOVA and post-hoc analyses were performed using the mean parameter estimates from each contrast ([Neg > Non], [Pos > Non], and [Neu > Non]). Greater IFG activation was observed for negative words compared to positive (p = .024*) and neutral (p < .001***) words; however, no significant difference was found between positive and neutral words (p = .15). X-axis: IFG mean parametric estimates; Y-axis: conditions. Error bars: standard errors.
![Figure 1. Brain area showing higher activation for negative versus neutral words. Greater activity in the triangular part of left IFG (inferior frontal gyrus) was observed under the Negative > Neutral contrast. The statistical threshold was set at FWE-corrected p < .05 with cluster-level in the whole brain. To further clarify the activation pattern in the left IFG among conditions, a one-way repeated ANOVA and post-hoc analyses were performed using the mean parameter estimates from each contrast ([Neg > Non], [Pos > Non], and [Neu > Non]). Greater IFG activation was observed for negative words compared to positive (p = .024*) and neutral (p < .001***) words; however, no significant difference was found between positive and neutral words (p = .15). X-axis: IFG mean parametric estimates; Y-axis: conditions. Error bars: standard errors.](/cms/asset/2a96eadf-899b-4969-8714-c6cb855f2fdb/plcp_a_2307630_f0001_oc.jpg)
Table 3. Brain areas activated by the emotional word versus neutral word contrast.
Effect of social interaction intensity
A voxel-wise multiple regression analysis with two independent variables (the social interaction intensity scores and the LX proficiency scores) implemented in SPM revealed that there were correlations between social interaction intensity and brain regions in both positive and negative word conditions, but not the neutral word condition (see and ). While a significant positive correlation between activity in the left ventral striatum and the social interaction intensity score was found in the contrast of [Pos > Non] (whole brain cluster-level FWE-corrected, p < .05), activity in the right amygdala was positively correlated with social interaction intensity in the contrast of [Neg > Non] (SVC, voxel-wise FWE-corrected, p < .05). None of the correlation was observed in [Neg > Neu], [Pos > Neu] and [Neu > Non] contrasts.
Figure 2. Brain areas correlated with social interaction intensity scores for emotional words. Upper image depicts the left ventral striatum (−4, 4, −12), demonstrating a significant positive correlation with social interaction intensity scores in the Positive > Nonword contrast (FWE-corrected p < .05 at the cluster-level in the whole brain). Bottom image depicts the right amygdala (18, 4, −16), showing a significant positive correlation with social interaction intensity scores in the Negative > Nonword contrast (FWE-corrected p < .05 at the voxel-level in SVC). Y-axis: mean parametric estimates for each area; X-axis: social interaction intensity scores.
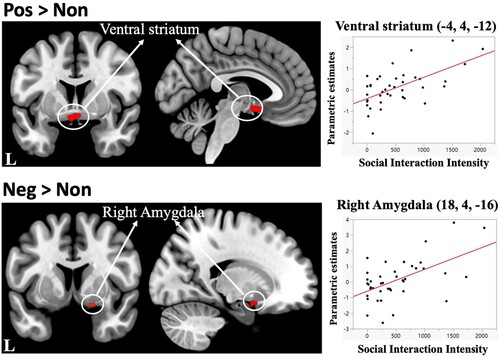
Table 4. Brain areas that showed a positive correlation between activity and social interaction intensity scores.
Effect of LX linguistic proficiency
A voxel-wise multiple regression analysis with two variables (the social interaction intensity scores and the LX proficiency scores) implemented in SPM revealed that the level of LX linguistic proficiency was not correlated in any brain areas in either negative or positive word conditions. In contrast, a significant positive correlation was found in the anterior cingulate cortex, the right cerebellum, left superior temporal gyrus (STG), and the left supramarginal gyrus (SMG) in the neutral word condition, indicating that the higher the level of LX linguistic proficiency, the more these brain areas involved in processing neutral words (see and ).
Figure 3. Brain areas correlated with linguistic proficiency for neutral words. Brain regions showing a positive correlation with Japanese (LX) linguistic proficiency scores in the [Neu > Non] contrast include: ACC (anterior cingulate cortex), left cerebellum, Left STG (superior temporal gyrus), and Left SMG (supramarginal gyrus). FWE-corrected p < .05 at the cluster-level in the whole brain. Y-axis: mean parametric estimates for each area; X-axis: Japanese linguistic proficiency scores.
![Figure 3. Brain areas correlated with linguistic proficiency for neutral words. Brain regions showing a positive correlation with Japanese (LX) linguistic proficiency scores in the [Neu > Non] contrast include: ACC (anterior cingulate cortex), left cerebellum, Left STG (superior temporal gyrus), and Left SMG (supramarginal gyrus). FWE-corrected p < .05 at the cluster-level in the whole brain. Y-axis: mean parametric estimates for each area; X-axis: Japanese linguistic proficiency scores.](/cms/asset/1138b5b3-fecd-45b8-aca1-c1c63fb82b10/plcp_a_2307630_f0003_oc.jpg)
Table 5. Brain areas that showed a positive correlation between activity and Japanese linguistic proficiency.
Discussion
This study examined the correlation between brain activity associated with the processing of LX (in this case, Japanese) emotional words and the intensity of social interactions with native Japanese speakers among Chinese L1 speakers in an immersive environment. Additionally, we investigated whether these correlations were more robust for LX positive words, indicative of a positivity bias. We found that the intensity of social interaction was positively correlated with brain activity in the left ventral striatum during the processing of LX positive emotional words and in the right amygdala during the processing of LX negative emotional words. Conversely, LX linguistic proficiency showed a positive correlation with brain activity in the ACC, the left cerebellum, the left STG and SMG during the processing of LX neutral words. Furthermore, LX negative words elicited greater activity in the triangular part of the left IFG (BA 45) when compared to LX positive and neutral words, suggesting a greater cognitive demand for processing negative words.
Overall, these results suggest that positive and negative emotional words are processed differently in the brain, and that the individual’s experiences of real-life social interaction using LX is likely linked to the neural representation of LX emotional words in late LX learners. Bilinguals who engage in more meaningful social interactions with speakers of the target language are possibly more attuned to the emotionality in that language. In the following sections, we discuss neural correlates of LX word processing in terms of social interaction intensity, LX proficiency, and cognitive load in processing negative words.
Correlations between social interaction intensity and LX emotional word processing
Significant positive correlations were observed between the intensity of LX social interaction and neural activity in specific brain regions: the left ventral striatum was implicated in the processing of LX positive emotional words, whereas the right amygdala was involved in the processing of LX negative emotional words. These findings suggest that the neural mechanisms underlying the processing of the LX emotional words are intricately linked to the extent of social interaction in the LX contexts.
The left ventral striatum and the right amygdala, as parts of the limbic system (e.g. Citron, Citation2012), are crucial brain areas for the processing of emotional information (Barrett & Bliss-Moreau, Citation2009; Lindquist et al., Citation2016). The amygdala is typically more activated in response to negative emotions such as fear, anxiety, and anger (see Baxter & Murray, Citation2002 for a review). In contrast, the ventral striatum is typically more activated in response to positive emotions like pleasure, reward, and motivation (Berridge & Kringelbach, Citation2015). Previous studies on the processing of emotional words have documented the activation of these regions in response to emotionally laden written stimuli (Chen et al., Citation2015; Citron, Citation2012; Hamann & Mao, Citation2002). For example, during an emotional word perception task, Hamann and Mao (Citation2002) found that the ventral striatum was activated by positive words, and the amygdala was activated by negative words. Thus, it can be inferred that the activation of the left ventral striatum and right amygdala is associated with the perception of positive and negative emotion words. We speculate that authentic social interaction with native speakers rendered LX emotional words more salient and emotionally charged for our participants. Consequently, this salient emotional information was likely processed initially by the salience network, including regions such as the striatum and amygdala (Seeley et al., Citation2007). These regions may serve as the first responders, preparing emotional stimuli for further processing, such as emotion categorisation and emotion regulation, which engage cognitive and modulatory systems.
In the current study, the intensity of social interaction was operationalised as the amount of time spent in conversations in the target language (i.e. Japanese) with close friends in various social situations. Thus, the composite score reflects both quantitative aspects (i.e. number of hours of conversation) and qualitative aspects (i.e. conversations with close friends) of social language use. According to Planalp and Benson (Citation1992), conversations among close friends tend to be more detailed, fluent, and emotionally charged compared to those with acquaintances. Conversations with acquaintances, on the other hand, tend to be limited to superficial topics and follow a question-and-answer format. As a result, social interactions with close friends not only heighten the authenticity of social language use but also potentially amplify sensitivity to emotional words as opposed to neutral ones. Such a notion aligns with our findings, demonstrating a positive correlation between that the intensity of social interaction with close friends and the activity in emotion-related brain regions during the processing of LX emotional words.
Previous behavioural and neuroimaging studies on language acquisition have reported that social interactions are beneficial for language learning (Dewey et al., Citation2012; Jeong et al., Citation2021; Pellegrini et al., Citation2002). For example, Jeong et al. (Citation2021) investigated the effects of social learning on language processing by asking 36 adult participants to learn novel words either through simulated social interactive videos or through written translation in their first language (L1). The authors reported that words learned through social interactive videos elicited brain activity patterns more similar to L1 processing than words learned through translation (see also Jeong et al., Citation2010). Specifically, brain regions associated with social, emotional, and perceptual-motor processing were more strongly activated in the social interaction condition than in the L1 translation condition. Their findings particularly suggest that social interaction may facilitate the development of rich semantic representations in the brain among late language learners, as indicated by better performance on post-learning vocabulary retention tests.
The present study extends these insights by showing a positive correlation between the intensity of social interaction and the activation of emotion-related brain regions during the processing of positive and negative LX words among late learners. These findings potentially underscore the role of authentic social language use in bolstering semantic representations in the brain, possibly contributing to the heightened emotional resonance in language. Given that the present study focused solely on correlational data, it is imperative that future research be conducted to establish cause-and-effect relationships. Research designs such as controlled experiments or longitudinal studies would provide a more comprehensive understanding of the role that social interaction plays in the acquisition of emotional resonance in a foreign language (LX). It is worth noting that although we observed the correlation between social intensity and amygdala activity only in the processing of LX negative words, it has been shown that the amygdala responds to both negative and positive emotions (Lewis et al., Citation2007; Sabatinelli et al., Citation2011). One possible explanation for the significant correlation between amygdala activity and social interaction intensity during negative word processing could be that negative words might be perceived as more salient (or more threatening) compared to positive words in social contexts. This could lead to a more robust amygdala response, even though the emotional arousal between negative and positive words was matched. However, as we did not directly measure the salience of negative words, this interpretation requires further testing in the future.
Effects of linguistics proficiency on LX neutral word processing
In our study, we found evidence supporting the effect of linguistic proficiency on the processing of neutral words, but not on emotional words. Specifically, we observed a positive correlation between Japanese linguistic proficiency, as measured by the Japanese C-test (Eckes & Grotjahn, Citation2006; Shin, Citation1990; Suzuki, Citation2015), and the brain activity in the left cerebellum, anterior cingulate cortex (ACC), left superior temporal gyrus, and supramarginal gyrus. These positive correlations may indicate that as participants’ linguistic proficiency improves, their ability to analyze spoken words and selective attention to linguistic stimuli also improves. Consistent with these neurological findings, our behavioural data showed a significant correlation between LX proficiency level and accuracy rates for neutral words. The Japanese C-test is a standardised test designed to assess learners’ metalinguistic proficiency in Japanese, evaluating their understanding and use of Japanese grammar, vocabulary, and sentence structures by requiring them to fill in the missing words in a written text. High scores on the Japanese C-test generally indicate a high proficiency in comprehending written Japanese (e.g. Suzuki, Citation2015).
The superior temporal gyrus and supramarginal gyrus are frequently reported to be involved in auditory word processing, and numerous studies have explored their relationship with language proficiency and behavioural performance (Booth et al., Citation2003; DeWitt & Rauschecker, Citation2012; Mechelli et al., Citation2004). These areas also participate in auditory short-term memory during language tasks (Buchsbaum, Citation2013; Leff et al., Citation2009).
While the left cerebellum and ACC are not typically viewed as language-related areas, emerging research suggests they play roles in language tasks requiring cognitive control, monitoring (Abutalebi & Green, Citation2007; Gauvin et al., Citation2016; Piai et al., Citation2013). Specifically, the ACC, a key component of the cognitive control network, plays a role in executing and monitoring various cognitive tasks, such as conflict monitoring and regulation (Abutalebi & Green, Citation2007). In language studies, it is often activated when metalinguistic knowledge is needed, such as in language error detection and production tasks (Gauvin et al., Citation2016; Piai et al., Citation2013). The cerebellum, meanwhile, is proposed to act as a ‘master coordinator’ for various cognitive processes, including attention, working memory, and language processing (Koziol et al., Citation2014; Lin & Weng, Citation2006). It achieves this by integrating and regulating information from different brain regions to enhance their collective efficiency.
Taken together, both our behavioural and brain data suggest that participants with higher scores on the Japanese C-test may process LX neutral words more efficiently. This increased efficiency likely involves the engagement of cognitive control networks, alongside core auditory processing areas. This is particularly true for the neutral words, which are more commonly encountered and learned in the written contexts.
Left IFG activation for LX negative emotional words
While LX learners exhibited comparable accuracy across the negative, positive, and neutral word conditions, greater brain activity was observed in the triangular part of the left IFG when processing negative words compared to neutral and positive words as illustrated in . This observation was consistent with the behavioural data, which also demonstrated significantly longer reaction times for LX negative words compared to neutral and positive words. Thus, greater activation in the left IFG may indicate increased cognitive demands for processing LX negative emotional words.
Previous research on word processing has frequently reported the involvement of the left IFG in the tasks requiring controlled word retrieval (Badre & Wagner, Citation2002; Glaser et al., Citation2013; Wagner et al., Citation2001). For instance, Wagner et al. (Citation2001) investigated the roles of the left IFG in word retrieval under varying cognitive demands. In their task, participants had to select words that were semantically related to a given cue from multiple options. When cues were less semantically related or when more alternative cues were available, the activation of left IFG increased due to the cognitive demand of controlled word processing.
On the other hand, tasks involving emotion regulation, such as suppressing an unwanted emotional response, have consistently implicated the left IFG, particularly its triangular part (Buhle et al., Citation2014; Ochsner et al., Citation2002; Wager et al., Citation2008). Ochsner et al. (Citation2002) demonstrated the involvement of the left IFG in cognitive strategies for regulating emotional responses. Specifically, they found activation in the left IFG when participants were prompted to re-evaluate or reinterpret the meaning of negative emotional stimuli. Emotional words carry both a linguistic meaning and an emotional valence. Given the roles of the IFG in controlled word processing and emotion regulation, activation of the left IFG may stem from the cognitive load associated with regulating the negative emotions triggered by LX negative words.
The heightened effort required during LX negative word processing discovered in this study could be linked to a positivity bias stemming from prior LX experiences (Conrad et al., Citation2011; Sheikh & Titone, Citation2016). The theory of positivity bias suggests that human communication tends to be positively skewed, resulting in more frequent use of positive words and fewer negative words in everyday interactions, regardless of L1/LX user/learner status (Boucher & Osgood, Citation1969). The cognitive load in processing negative LX words aligns with the findings of previous event-related potential studies (e.g. Conrad et al., Citation2011) and eye-tracking experiments (e.g. Sheikh & Titone, Citation2016). For example, Sheikh and Titone (Citation2016) conducted an eye-tracking experiment investigating the processing of emotional words in LX by requesting bilingual participants to read LX negative, positive, and neutral words. They found that LX negative words were read more slowly and had longer fixation times compared to positive and neutral words. This could possibly be attributed to a positivity bias in LX experience.
Furthermore, studies have reported the sensitivity of left IFG activity to language exposure (Perani et al., Citation2003; Vingerhoets et al., Citation2003). Perani et al. (Citation2003) observed that activity in the left IFG decreased with increasing LX exposure in early bilinguals (i.e. Spanish-Catalan and Catalan-Spanish bilinguals). In their study, early bilinguals with comparable levels of proficiency in both languages but varying exposure were tasked with silently recalling a word beginning with a specific letter in either their L1 or LX while inside the fMRI scanner. The results indicated that the less exposed language (LX) elicited greater activity in the left frontal lobe compared to the more exposed language (L1).
Based on these findings, we interpret the increased activation of the triangular part of the left IFG during LX negative word processing as an indication of higher cognitive demands associated with regulating the negative emotion triggered by negative words in LX learners. This heightened cognitive demand might be related to the positivity bias. The positivity bias in social interactions may reduce opportunities for the utilisation of negative words over positive and neutral words in actual social contexts, leading to an augmented cognitive demand and activation of the left IFG during negative word processing. The potential mechanism for these relationships needs to be explored through further experiments.
Limitations
One major limitation of this study lies in the lack of manipulation of social interaction; instead, natural social interactions were assessed using a questionnaire. Participants were asked to estimate the number of hours per week they spoke Japanese. As a result, we cannot establish a causal relationship between the intensity of social interaction and changes in brain activity based solely on these correlational findings. Additionally, an alternative explanation could be that LX learners who show higher brain activity in emotion-related areas may be more inclined to engage socially with speakers of the target language. Although this counter-hypothesis lacks empirical and theoretical support to the best of our knowledge, it cannot be ruled out.
Another methodological limitation arises from our exclusive focus on interactions with close friends when calculating the intensity of social interactions. Such interactions tend to be rich in emotional and linguistic diversity (Planalp & Benson, Citation1992), which could introduce a positivity bias (Boucher & Osgood, Citation1969). This consideration becomes especially relevant given that international students are often recipients of more favourable social treatment (Mak et al., Citation2014). Given these constraints, additional empirical evidence is needed to conclusively determine the role of social interactions in LX emotional word processing.
Despite the careful design of our study, aimed at using nonwords as a controllable baseline with respect to meaning and socio-linguistic experience, it's important to note that the phonetic features of a nonword might still induce emotion. While this is likely to have a minimal impact – given that all nonwords are novel to all participants – it does not entirely preclude the possibility. This suggests the need for future research that delves deeper into the relationship between phonetic features and emotional resonance.
Lastly, our participants, who began learning Japanese at an average age of 19, exhibited lower linguistic proficiency compared to those in existing bilingual emotion studies. This disparity could affect their ability to access emotional meanings, ultimately leading to no significant group-level emotional effects. To gain further insights into the neural mechanisms that may be influenced by social interactions on LX emotional word processing, future studies should aim to include a more diverse sample of LX learners. Subgroup analyses that contrast experiences between learners in formal settings (e.g. classrooms) and those with extensive real-world experience, and comparisons between groups with different language start ages, could offer valuable insights. Additionally, longitudinal studies are recommended to explore how social interactions might strengthen the neural pathways responsible for LX emotional word processing.
Conclusions
This research contributes valuable neuroimaging data that establish a strong relationship between the intensity of social interactions and neural responses during the processing of emotional words in LX. Specifically, we found a significant positive correlation between social interaction intensity and activity in the left ventral striatum for positive words and in the right amygdala for negative words. In addition to these core findings, we observed increased neural activity in the triangular part of the left inferior frontal gyrus (IFG) when participants were processing negative words in LX. This could potentially indicate that regulating negative emotion associated with LX words imposes greater cognitive demands on the LX learners, which is likely to activate this particular neural region more intensely. The present study's results are of particular significance, as they imply that the neural mechanisms for the emotional processing of language are sensitive to both the qualitative and quantitative aspects of language use. These findings suggest that the neural substrates supporting emotional processing of language are adaptable and responsive to language use patterns in adulthood.
Supplemental Material
Download MS Word (1.1 MB)Disclosure statement
No potential conflict of interest was reported by the author(s).
Correction Statement
This article has been corrected with minor changes. These changes do not impact the academic content of the article.
Additional information
Funding
Notes
1 In this article, LX refers to any foreign language that is not the first language (L1) (i.e. acquired before 3 years of age) with proficiency levels that can range from minimal to maximal. For a full explanation of the rationale for this terminology, see the work by Dewaele (Citation2018).
2 Our study follows the valence-categorical analysis paradigm common in bilingualism research, but it’s important to note its limitations. Categorizing word valence – positive, negative, and neutral – may obscure non-linear variable relationships (Altman & Royston, Citation2006). In response to an anonymous reviewer’s concerns, we also conducted parametric modulation analyses to model the non-linear relationship between brain activity and emotional valence (up to the 2nd order). Details of this approach are in the supplementary materials (pp. 3–8).
References
- Abutalebi, J., & Green, D. (2007). Bilingual language production: The neurocognition of language representation and control. Journal of Neurolinguistics, 20(3), 242–275. https://doi.org/10.1016/j.jneuroling.2006.10.003
- Altman, D. G., & Royston, P. (2006). The cost of dichotomising continuous variables. BMJ, 332(7549), 1080. https://doi.org/10.1136/bmj.332.7549.1080
- Ayçiçegi, A., & Harris, C. (2004). Brief report bilinguals’ recall and recognition of emotion words. Cognition and Emotion, 18(7), 977–987. https://doi.org/10.1080/02699930341000301
- Badre, D., & Wagner, A. D. (2002). Semantic retrieval, mnemonic control, and prefrontal cortex. Behavioral and Cognitive Neuroscience Reviews, 1(3), 206–218. https://doi.org/10.1177/1534582302001003002
- Barrett, L. F., & Bliss-Moreau, E. (2009). Chapter 4 affect as a psychological primitive. In Advances in experimental social psychology (Vol. 41, pp. 167–218). Elsevier. https://doi.org/10.1016/S0065-2601(08)00404-8
- Baxter, M. G., & Murray, E. A. (2002). The amygdala and reward. Nature Reviews Neuroscience, 3(7), 563–573. https://doi.org/10.1038/nrn875
- Berridge, K. C., & Kringelbach, M. L. (2015). Pleasure systems in the brain. Neuron, 86(3), 646–664. https://doi.org/10.1016/j.neuron.2015.02.018
- Bloom, L., & Beckwith, R. (1989). Talking with feeling: Integrating affective and linguistic expression in early language development. Cognition and Emotion, 3(4), 313–342. https://doi.org/10.1080/02699938908412711
- Booth, J. R., Burman, D. D., Meyer, J. R., Gitelman, D. R., Parrish, T. B., & Mesulam, M. M. (2003). Relation between brain activation and lexical performance. Human Brain Mapping, 19(3), 155–169. https://doi.org/10.1002/hbm.10111
- Boucher, J., & Osgood, C. E. (1969). The pollyanna hypothesis. Journal of Verbal Learning and Verbal Behavior, 8(1), 1–8. https://doi.org/10.1016/S0022-5371(69)80002-2
- Brett, M., Anton, J. L., Valabregue, R., & Poline, J. B. (2002, June). Region of interest analysis using an SPM toolbox. In 8th international conference on functional mapping of the human brain (Vol. 16, No. 2, p. 497).
- Buchsbaum, B. R. (2013). The role of consciousness in the phonological loop: Hidden in plain sight. Frontiers in Psychology, 4, 496. https://doi.org/10.3389/fpsyg.2013.00496
- Buhle, J. T., Silvers, J. A., Wager, T. D., Lopez, R., Onyemekwu, C., Kober, H., Weber, J., & Ochsner, K. N. (2014). Cognitive reappraisal of emotion: A meta-analysis of human neuroimaging studies. Cerebral Cortex, 24(11), 2981–2990. https://doi.org/10.1093/cercor/bht154
- Caldwell-Harris, C. L. (2014). Emotionality differences between a native and foreign language: Theoretical implications. Frontiers in Psychology, 5. https://doi.org/10.3389/fpsyg.2014.01055
- Caldwell-Harris, C. L., Staroselsky, M., Smashnaya, S., & Vasilyeva, N. (2012). Emotional resonances of bilinguals’ two languages vary with age of arrival: The Russian–English bilingual experience in the US. Dynamicity in Emotion Concepts, 373–395.
- Caldwell-Harris, C. L., Tong, J., Lung, W., & Poo, S. (2011). Physiological reactivity to emotional phrases in mandarin—English bilinguals. International Journal of Bilingualism, 15(3), 329–352. https://doi.org/10.1177/1367006910379262
- Chen, P., Lin, J., Chen, B., Lu, C., & Guo, T. (2015). Processing emotional words in two languages with one brain: ERP and fMRI evidence from Chinese–English bilinguals. Cortex, 71, 34–48. https://doi.org/10.1016/j.cortex.2015.06.002
- Citron, F. M. M. (2012). Neural correlates of written emotion word processing: A review of recent electrophysiological and hemodynamic neuroimaging studies. Brain and Language, 122(3), 211–226. https://doi.org/10.1016/j.bandl.2011.12.007
- Conrad, M., Recio, G., & Jacobs, A. M. (2011). The time course of emotion effects in first and second language processing: A cross cultural ERP study with German? Spanish bilinguals. Frontiers in Psychology, 2. https://doi.org/10.3389/fpsyg.2011.00351
- Dewaele, J.-M. (2008). The emotional weight of I love you in multilinguals’ languages. Journal of Pragmatics, 40(10), 1753–1780. https://doi.org/10.1016/j.pragma.2008.03.002
- Dewaele, J.-M. (2018). Why the dichotomy ‘L1 versus LX user’ is better than ‘native versus non-native speaker. Applied Linguistics, amw055. https://doi.org/10.1093/applin/amw055
- Dewaele, J.-M., Lorette, P., Rolland, L., & Mavrou, E. (2021). Differences in emotional reactions of Greek, Hungarian and British users of English when watching English television. International Journal of Applied Linguistics, 31(3), 345–361. https://doi.org/10.1111/ijal.12333
- Dewey, D. P., Bown, J., & Eggett, D. (2012). Japanese language proficiency, social networking, and language use during study abroad: Learners’ perspectives. The Canadian Modern Language Review, 68(2), 111–137. https://doi.org/10.3138/cmlr.68.2.111
- Dewey, D. P., Ring, S., Gardner, D., & Belnap, R. K. (2013). Social network formation and development during study abroad in the Middle East. System, 41(2), 269–282. https://doi.org/10.1016/j.system.2013.02.004
- DeWitt, I., & Rauschecker, J. P. (2012). Phoneme and word recognition in the auditory ventral stream. Proceedings of the National Academy of Sciences, 109(8), E505–E514. https://doi.org/10.1073/pnas.1113427109
- Eckes, T., & Grotjahn, R. (2006). A closer look at the construct validity of C-tests. Language Testing, 23(3), 290–325. https://doi.org/10.1191/0265532206lt330oa
- Eilola, T. M., Havelka, J., & Sharma, D. (2007). Emotional activation in the first and second language. Cognition and Emotion, 21(5), 1064–1076. https://doi.org/10.1080/02699930601054109
- Ferré, P., García, T., Fraga, I., Sánchez-Casas, R., & Molero, M. (2010). Memory for emotional words in bilinguals: Do words have the same emotional intensity in the first and in the second language? Cognition & Emotion, 24(5), 760–785. https://doi.org/10.1080/02699930902985779
- Gass, S. M., & Selinker, L. (1983). Language transfer in language learning. Issues in second language research. Newbury House Publishers, Inc.
- Gauvin, H. S., De Baene, W., Brass, M., & Hartsuiker, R. J. (2016). Conflict monitoring in speech processing: An fMRI study of error detection in speech production and perception. NeuroImage, 126, 96–105. https://doi.org/10.1016/j.neuroimage.2015.11.037
- Glaser, Y. G., Martin, R. C., Van Dyke, J. A., Hamilton, A. C., & Tan, Y. (2013). Neural basis of semantic and syntactic interference in sentence comprehension. Brain and Language, 126(3), 314–326. https://doi.org/10.1016/j.bandl.2013.06.006
- Gotoh, F., & Ohta, N. (2001). Affective valence of two-compound kanji words. Tsukuba Psychological Research, 23, 45–52.
- Graham, C. R., Hamblin, A. W., & Feldstein, S. (2001). Recognition of emotion in English voices by speakers of Japanese, Spanish and English. IRAL - International Review of Applied Linguistics in Language Teaching, 39(1). https://doi.org/10.1515/iral.39.1.19
- Hamann, S., & Mao, H. (2002). Positive and negative emotional verbal stimuli elicit activity in the left amygdala. Neuroreport, 13(1), 15–19. https://doi.org/10.1097/00001756-200201210-00008
- Harris, C. L., Ayçiçeği, A., & Gleason, J. B. (2003). Taboo words and reprimands elicit greater autonomic reactivity in a first language than in a second language. Applied Psycholinguistics, 24(4), 561–579. https://doi.org/10.1017/S0142716403000286
- Harris, C. L., Gleason, J. B., & Ayçiçeǧi, A. (2006). 10. When is a first language more emotional? Psychophysiological evidence from bilingual speakers. In A. Pavlenko (Ed.), Bilingual minds (pp. 257–283). Multilingual Matters. https://doi.org/10.21832/9781853598746-012
- Higami, K., Fujida, C., Kaneko, Y., Suyama, H., Ito, R., Sato, H., Matsumoto, T., & Suzuki, H. (2015). Affective valence and emotionality of two-kanji-compound words. Academia, Journal of the Nanzan Academic Society. Humanities and Natural Sciences, 10, 195–204.
- Honma, Y. (2014). Drawing up of the Japanese word stimulus based on the emotional valence and arousal of the word. Bulletin of Aichi Institute of Technology, 49, 13–24.
- Jeong, H., Li, P., Suzuki, W., Sugiura, M., & Kawashima, R. (2021). Neural mechanisms of language learning from social contexts. Brain and Language, 212, 104874. https://doi.org/10.1016/j.bandl.2020.104874
- Jeong, H., Sugiura, M., Sassa, Y., Wakusawa, K., Horie, K., Sato, S., & Kawashima, R. (2010). Learning second language vocabulary: Neural dissociation of situation-based learning and text-based learning. Neuroimage, 50(2), 802–809. https://doi.org/10.1016/j.neuroimage.2009.12.038
- Kazanas, S. A., McLean, J. S., & Altarriba, J. (2019). Emotion and emotion concepts: Processing and use in monolingual and bilingual speakers. In J. Schwieter & M. Paradis (Eds.), The handbook of the neuroscience of multilingualism (pp. 313–334). John Wiley & Sons. https://doi.org/10.1002/9781119387725.ch15.
- Koziol, L. F., Budding, D., Andreasen, N., D’Arrigo, S., Bulgheroni, S., Imamizu, H., Ito, M., Manto, M., Marvel, C., Parker, K., Pezzulo, G., Ramnani, N., Riva, D., Schmahmann, J., Vandervert, L., & Yamazaki, T. (2014). Consensus paper: The cerebellum’s role in movement and cognition. The Cerebellum, 13(1), 151–177. https://doi.org/10.1007/s12311-013-0511-x
- Leff, A. P., Schofield, T. M., Crinion, J. T., Seghier, M. L., Grogan, A., Green, D. W., & Price, C. J. (2009). The left superior temporal gyrus is a shared substrate for auditory short-term memory and speech comprehension: Evidence from 210 patients with stroke. Brain, 132(12), 3401–3410. https://doi.org/10.1093/brain/awp273
- Lewis, P., Critchley, H., Rotshtein, P., & Dolan, R. (2007). Neural correlates of processing valence and arousal in affective words. Cerebral Cortex, 17(3), 742–748. https://doi.org/10.1093/cercor/bhk024
- Li, P., & Jeong, H. (2020). The social brain of language: Grounding second language learning in social interaction. Npj Science of Learning, 5(1), 8. https://doi.org/10.1038/s41539-020-0068-7
- Lin, C., & Weng, X. (2006). Motor, language, and learning: Functional magnetic resonance imaging of the cerebellum. Advances in Psychological Science, 14(4), 532.
- Lindquist, K. A., Satpute, A. B., Wager, T. D., Weber, J., & Barrett, L. F. (2016). The brain basis of positive and negative affect: Evidence from a meta-analysis of the human neuroimaging literature. Cerebral Cortex, 26(5), 1910–1922. https://doi.org/10.1093/cercor/bhv001
- Mak, A. S., Brown, P. M., & Wadey, D. (2014). Contact and attitudes toward international students in Australia: Intergroup anxiety and intercultural communication emotions as mediators. Journal of Cross-Cultural Psychology, 45(3), 491–504. https://doi.org/10.1177/0022022113509883
- Maldjian, J. A., Laurienti, P. J., Kraft, R. A., & Burdette, J. H. (2003). An automated method for neuroanatomic and cytoarchitectonic atlas-based interrogation of fMRI data sets. Neuroimage, 19(3), 1233–1239. https://doi.org/10.1016/S1053-8119(03)00169-1
- Mechelli, A., Crinion, J. T., Noppeney, U., O'Doherty, J., Ashburner, J., Frackowiak, R. S., & Price, C. J. (2004). Structural plasticity in the bilingual brain. Nature, 431(7010), 757–757. https://doi.org/10.1038/431757a
- Nakic, M., Smith, B. W., Busis, S., Vythilingam, M., & Blair, R. J. R. (2006). The impact of affect and frequency on lexical decision: The role of the amygdala and inferior frontal cortex. Neuroimage, 31(4), 1752–1761. https://doi.org/10.1016/j.neuroimage.2006.02.022
- Nishizawa, H., Isbell, D. R., & Suzuki, Y. (2022). Review of the Japanese-language proficiency test. Language Testing, Early View. https://doi.org/10.1177/02655322221080898
- Ochsner, K. N., Bunge, S. A., Gross, J. J., & Gabrieli, J. D. (2002). Rethinking feelings: An FMRI study of the cognitive regulation of emotion. Journal of Cognitive Neuroscience, 14(8), 1215–1229. https://doi.org/10.1162/089892902760807212
- Ogasawara, N. (2003). Practical guides of Japanese expressions for daily life. Senmon Kyouiku Publishing.
- Oldfield, R. C. (1971). The assessment and analysis of handedness: The Edinburgh inventory. Neuropsychologia, 9(1), 97–113. https://doi.org/10.1016/0028-3932(71)90067-4
- Pavlenko, A. (2008). Emotion and emotion-laden words in the bilingual lexicon. Bilingualism: Language and Cognition, 11(2), 147–164. https://doi.org/10.1017/S1366728908003283
- Pavlenko, A. (2012). Affective processing in bilingual speakers: Disembodied cognition? International Journal of Psychology, 47(6), 405–428. https://doi.org/10.1080/00207594.2012.743665
- Pellegrini, A. D., Melhuish, E., Jones, I., Trojanowska, L., & Gilden, R. (2002). Social contexts of learning literate language: The role of varied, familiar, and close peer relationships. Learning and Individual Differences, 12(4), 375–389. https://doi.org/10.1016/S1041-6080(02)00044-4
- Perani, D., & Abutalebi, J. (2005). The neural basis of first and second language processing. Current Opinion in Neurobiology, 15(2), 202206. https://doi.org/10.1016/j.conb.2005.03.007
- Perani, D., Abutalebi, J., Paulesu, E., Brambati, S., Scifo, P., Cappa, S. F., & Fazio, F. (2003). The role of age of acquisition and language usage in early, high-proficient bilinguals: An fMRI study during verbal fluency. Human Brain Mapping, 19(3), 170–182. https://doi.org/10.1002/hbm.10110
- Piai, V., Roelofs, A., Acheson, D. J., & Takashima, A. (2013). Attention for speaking: Domain-general control from the anterior cingulate cortex in spoken word production. Frontiers in Human Neuroscience, 7, 832. https://doi.org/10.3389/fnhum.2013.00832
- Planalp, S., & Benson, A. (1992). Friends’ and acquaintances’ conversations I: Perceived differences. Journal of Social and Personal Relationships, 9(4), 483–506. https://doi.org/10.1177/0265407592094002
- Rintell, E. M. (1984). But how did you FEEL about that?: The learner’s perception of emotion in speech. Applied Linguistics, 5(3), 255–264. https://doi.org/10.1093/applin/5.3.255
- Rosselli, M., Vélez-Uribe, I., & Ardila, A. (2017). Emotional associations of words in L1 and L2 in bilinguals. In A. Ardila, A. Cieślicka, R. Heredia, & M. Rosselli (Eds.), Psychology of bilingualism (pp. 39–72). Springer. https://doi.org/10.1007/978-3-319-64099-0_3.
- Sabatinelli, D., Fortune, E. E., Li, Q., Siddiqui, A., Krafft, C., Oliver, W. T., Back, S., & Jeffries, J. (2011). Emotional perception: Meta-analyses of face and natural scene processing. Neuroimage, 54(3), 2524–2533. https://doi.org/10.1016/j.neuroimage.2010.10.011
- Seeley, W. W., Menon, V., Schatzberg, A. F., Keller, J., Glover, G. H., Kenna, H., Reis, A. L., & Greicius, M. D. (2007). Dissociable intrinsic connectivity networks for salience processing and executive control. The Journal of Neuroscience, 27(9), 2349–2356. https://doi.org/10.1523/JNEUROSCI.5587-06.2007
- Sheikh, N. A., & Titone, D. (2016). The embodiment of emotional words in a second language: An eye-movement study. Cognition and Emotion, 30(3), 488–500. https://doi.org/10.1080/02699931.2015.1018144
- Shin, K. (1990). From a Japanese cloze test to a Japanese modified C-test: Concerning scoring problems. Studies in Language and Culture, 11(2), 213–225.
- Slotnick, S. D. (2017). Cluster success: fMRI inferences for spatial extent have acceptable false-positive rates. Cognitive Neuroscience, 8(3), 150–155. https://doi.org/10.1080/17588928.2017.1319350
- Sulpizio, S., Maschio, N. D., Fedeli, D., & Abutalebi, J. (2019). Bilingual language processing: A meta-analysis of functional neuroimaging studies. Neuroscience & Biobehavioral Reviews, 108, 834–853. https://doi.org/10.1016/j.neubiorev.2019.12.014
- Suzuki, Y. (2015). Self-assessment of Japanese as a second language: The role of experiences in the naturalistic acquisition. Language Testing, 32(1), 63–81. https://doi.org/10.1177/0265532214541885
- Tzourio-Mazoyer, N., Landeau, B., Papathanassiou, D., Crivello, F., Etard, O., Delcroix, N., Mazoyer, B., & Joliot, M. (2002). Automated anatomical labeling of activations in SPM using a macroscopic anatomical parcellation of the MNI MRI single-subject brain. Neuroimage, 15(1), 273–289. https://doi.org/10.1006/nimg.2001.0978
- Vingerhoets, G., Van Borsel, J., Tesink, C., van Den Noort, M., Deblaere, K., Seurinck, R., Vandemaele, P., & Achten, E. (2003). Multilingualism: An fMRI study. Neuroimage, 20(4), 2181–2196. https://doi.org/10.1016/j.neuroimage.2003.07.029
- Wager, T. D., Davidson, M. L., Hughes, B. L., Lindquist, M. A., & Ochsner, K. N. (2008). Prefrontal-subcortical pathways mediating successful emotion regulation. Neuron, 59(6), 1037–1050. https://doi.org/10.1016/j.neuron.2008.09.006
- Wagner, A. D., Paré-Blagoev, E. J., Clark, J., & Poldrack, R. A. (2001). Recovering meaning: Left prefrontal cortex guides controlled semantic retrieval. Neuron, 31(2), 329–338. https://doi.org/10.1016/S0896-6273(01)00359-2
- Wu, Y. J., & Thierry, G. (2012). How reading in a second language protects your heart. The Journal of Neuroscience, 32(19), 6485–6489. https://doi.org/10.1523/JNEUROSCI.6119-11.2012