Abstract
The 4 Mountain Test (4MT) is a test of allocentric spatial working memory and has been proposed as an earlier marker of predementia Alzheimer’s disease (AD) than episodic verbal memory. We here compare the 4MT to the CERAD word list memory recall in both cognitively normal (CN) and mild cognitive impairment (MCI) cases with or without cerebrospinal fluid markers (CSF) of Alzheimer’s disease pathology. Linear regression was used to assess the influence of CSF determined Aβ-plaque (Aβ-/+) or neurofibrillary tau tangles (Tau-/+) on 4MT and CERAD recall performance. Analyses were performed in the full sample and the CN and MCI sub-samples. Pearson correlations were calculated to examine the relationship between 4MT and tests of psychomotor speed, verbal memory, cognitive flexibility, verbal fluency, and visuo-spatial perception. Analyses showed no significant differences in 4MT scores between Aβ-/Aβ+, nor Tau-/Tau + participants, irrespective of cognitive status. In contrast, CERAD recall scores were lower in both Aβ+ compared to Aβ- (p<.01), and Tau + compared to Tau- participants (p<.01) in the full sample analyses. There were no significant differences in CERAD recall performance between Aβ- vs. Aβ+ and Tau- vs. to Tau + in the in CN/MCI sub-samples. 4MT scores were significantly correlated with tests of psychomotor speed, cognitive flexibility, and visuo-spatial perception in the full sample analyses. In conclusion, the CERAD recall outperformed the 4MT as a cognitive marker of CSF determined AD pathology. This suggests that allocentric working memory, as measured by the 4MT, may not be used as an early marker of predementia AD.
Alzheimer’s disease (AD) is a neurodegenerative disease, where symptoms commonly emerge around 65–75 years of age (2022 Alzheimer’s Disease Facts and Figures, Alzheimer’s Association, Citation2022). The disease is hallmarked by pathophysiological changes in the brain, namely the deposition of amyloid-beta (Aβ) plaques, accumulation of neurofibrillary tau tangles and ensuing neurodegeneration. These markers of pathology can be determined by measuring cerebrospinal fluid (CSF) concentrations of Aβ42/40 ratio (Aβ), phosphorylated tau (p-tau) and total-tau (t-tau), or through neuroimaging using PET scans (Jack et al., Citation2018). While CSF-samples and PET scans are reliable and accurate in identifying early AD pathology, the procedures are invasive, time consuming, costly, and inaccessible for many health care professionals and care givers. The expected increase in AD cases highlight the need for accessible and affordable ways to differentiate between AD, normal aging, and other etiologies (Alzheimer’s Disease International, Citation2015).
AD is considered a continuum where initial brain changes may begin as early as 10–15 years before any clinical manifestation of cognitive decline (Sperling et al., Citation2011). By discovering AD in preclinical or prodromal phases, potential disease-modifying therapies can be implemented more effectively. The preclinical stage is thought to be a phase where different brain mechanisms compensate for early Aβ-plaque deposition and tau tangle formation. In this stage, cognitive functions are largely intact and daily functioning is unimpaired (Sperling et al., Citation2011). The subsequent prodromal phase is marked by mild cognitive impairment (MCI), where individuals show objective cognitive impairment on neuropsychological tests (Albert et al., Citation2011).
While considerable efforts have been made to discover novel CSF-, imaging- or blood markers to identify individuals in preclinical phases of AD, little progress has been achieved in identifying novel cognitive markers for AD. Episodic memory deficits are commonly considered the best early clinical marker of AD (Dubois et al., Citation2014). However, by the time memory deficits can be measured by cognitive tests, the entorhinal cortex (EC) and hippocampi already show signs of tau tangle pathology and accumulation of Aβ-plaques on PET scans (Therriault et al., Citation2022). Thus, memory deficits might represent the middle phase of the predementia AD disease trajectory, rather than an early phase (Dubois et al., Citation2014; Hadjichrysanthou et al., Citation2020). Furthermore, deficits in episodic memory are also present in other diseases, such as ALS, depression, frontotemporal dementia, and in healthy aging (Burt et al., Citation1995; Maass et al., Citation2018; Siafarikas et al., Citation2021; Yamanami-Irioka et al., Citation2011). Memory tests might therefore not be sensitive to the earliest phases of AD, nor specific to AD pathology.
Recently, spatial memory deficits have been suggested as possible markers of early AD pathology. Spatial encoding and navigation have been linked to specialized cells called “grid-cells” in the EC (Fyhn et al., Citation2004), and associated “place-cells” located in the hippocampi (Brun et al., Citation2002). Grid-cells are highly specialized in encoding spatial orientation and distance (Hafting et al., Citation2005), whilst hippocampal place-cells allow us to encode locations and develop cognitive maps (McNaughton et al., Citation2006). These cells enable us to orient ourselves in space, as well as develop and navigate routes. The EC is also one of the first brain regions to be affected in AD. Studies by Braak and Del Tredici (Citation2015) and Arendt et al. (Citation2015) have shown early neuronal loss and accumulation of neurofibrillary tau tangles in the EC of patients who develop AD. Moreover, Kunz et al. (Citation2015) examined grid-cell representation and entorhinal activation in healthy APOE-ε4 allele carriers and non-carriers. APOE-ε4 carriers, who have an increased genetic risk of AD, had reduced representations of grid-cells on fMRI scans. Reductions in grid-cell representations are thought to be related to worse performance on spatial memory tasks. These participants also showed an increased activation in the EC, hypothesized to be caused by compensatory activation due to entorhinal dysfunction (Kunz et al., Citation2015). Cognitive tests that target region specific cognitive abilities associated with the EC might therefore be promising early markers of AD pathophysiology, putatively emerging prior to episodic memory deficits.
The 4 Mountain Test (4MT) is a test of allocentric spatial working memory and has been proposed as an early marker of AD pathology (Moodley et al., Citation2015). Spatial strategies are commonly defined as either allocentric or egocentric. Allocentric strategies use visual cues and objects to encode spatial location irrespective of one’s own viewpoint. In contrast, egocentric strategies use the individuals own viewpoint to encode spatial locations (Derbie et al., Citation2021). The 4MT requires test takers to memorize a computer-generated mountain landscape, and then identify the same landscape from a different viewpoint. The 4MT’s combination of spatial reasoning and working memory has been linked to hippocampal function (Hartley et al., Citation2007). Notably, patients with hippocampal injury have been shown to perform worse on the 4MT compared to uninjured controls. The same participants performed worse on the 4MT than on tests targeting non-spatial working memory or spatial perception (Hartley et al., Citation2007). This could point to spatial working memory being more dependent on the hippocampi and possibly also the EC, compared to working memory or spatial perception alone. In support of this idea, Moodley et al. (Citation2015) showed that the 4MT differentiated between MCI participants with and without the presence of Aβ-plaques (Aβ+/-), whilst no differences in verbal episodic memory were demonstrated. This suggests that the 4MT might outperform episodic memory tests in detecting MCI due to AD.
The present study assesses the 4MT’s ability to detect subtle differences between participants with CSF determined presence or absence of Aβ pathology (Aβ+/-) and phosphorylated tau tangle pathology (p-tau) (Tau+/-) in both cognitively normal (CN) and MCI participants. Considering previous research, we hypothesize that 1) Adjusted for differences in demographical variables, 4MT performance will be significantly lower in Aβ+ compared to Aβ- participants, and lower Tau + compared to Tau- participants, regardless of cognitive status. 2) Episodic memory (measured by CERAD word list memory recall) will be worse in both Aβ+ compared to Aβ-, and in Tau + compared to Tau- participants with MCI, but equal among CN participants. As the 4MT is a novel test, we also examine the influence of demographics on test scores and compute correlations between the 4MT and tests from other cognitive domains. This includes tests of episodic verbal memory, phonemic verbal fluency, visuospatial perception, psychomotor speed, and cognitive flexibility.
Methods and materials
Cohort description and data collection
The sample comprised data from the Dementia Disease Initiation (DDI) cohort study. DDI is a Norwegian multi-center prospective cohort study focused on early detection and treatment of dementia and neurodegenerative diseases. While DDI collaborates with university hospitals across all Norwegian health regions, the current study used data collected at three sites: Akershus University Hospital, the University Hospital in North Norway, and Betanien Hospital in Bergen. The cohort comprises non-demented participants with symptoms of cognitive decline, as well as healthy controls. Participants with cognitive symptoms were recruited through self-referrals following advertisements in the media, or from GP referrals to memory clinics. Healthy controls were recruited from spouses of symptomatic participants, or through advertisements in the media. All participants underwent a standardized neurological examination, a clinical interview, neuropsychological testing, blood sampling, and a lumbar puncture. Inclusion criteria were ages 40–80, and a native language of Norwegian, Danish, or Swedish. Exclusion criteria were brain disorders or injuries, including clinical stroke and dementia, serious neurological, somatic, or psychiatric disease, intellectual disability, or other conditions that could affect test scores. All included participants fulfilled the inclusion criteria and none of the exclusion criteria. All participants in the current study had Norwegian as their native language.
For the current study, participants were defined as either cognitively normal (CN) or diagnosed with mild cognitive impairment (MCI). Cognitive classifications were based on the published criteria by Albert et al. (Citation2011) with some modification. The Albert et al. (Citation2011) criteria define MCI based on both objective impairment on neuropsychological tests and the presence of subjective cognitive decline. However, Bondi et al. (Citation2014) indicate that basing MCI classification on neuropsychological test scores alone, and not subjective symptoms, improve diagnostic accuracy. Heeding this, we based MCI classification on neuropsychological test results, and not on participants’ subjective experience of symptoms. The neuropsychological test battery comprised tests of cognitive flexibility and psychomotor speed (Trail Making Test A and B, (Reitan & Wolfson, Citation1985)), the CERAD wordlist memory test (Fillenbaum et al., Citation2008), the FAS phonemic verbal fluency test (Spreen & Benton, Citation1969), and the silhouettes test from the Visual Object and Spatial Perception (VOSP) battery (Warrington & James, Citation1991). Participants who attained test scores 1.5 standard deviation (SD) below the expected normative mean on either the Trail Making Test B, CERAD wordlist memory test (recall trial), VOSP silhouettes test, or the FAS phonemic verbal fluency test, were diagnosed with mild cognitive impairment (MCI). Demographically adjusted test norms based on Scandinavian samples were used to determine the expected normative mean for each test (Eliassen et al., Citation2020; Espenes et al., Citation2020; Kirsebom et al., Citation2019; Lorentzen et al., Citation2021). Participants who performed above the 1.5 SD limit were classified as cognitively normal (CN). For the sake of transparency, the percentages of participants in CN and the MCI samples who reported subjective symptoms of cognitive decline are reported in .
Table 1. Descriptive Statistics for Aβ-/+ and Tau-/+ participants in CN and MCI samples.
CSF sampling and biomarker classification
In addition to cognitive classification, participants were also grouped based on Aβ-status determined from CSF-samples. CSF-samples were collected through lumbar punctures performed by neurologists. All CSF-samples were frozen at −80 °C and sent to Akershus University Hospital to be analyzed (see Fladby et al. (Citation2017) for details). Ptau was measured at the Department of Interdisciplinary Laboratory Medicine and Medical Biochemistry, with either Elecsys (n = 3) or Innotest phospho-tau (181 P) assays (n = 92) (Fujirebio, Ghent, Belgium). CSF-concentrations of Aβ1-42 and Aβ1-40 were measured at the Department of Clinical Molecular Biology (EpiGen) and determined using ELISA Quickplex SQ120 from MesoScale Discovery. The V-plex Ab peptide panel 1 (6E10) kit (K15200E-1) was used to measure Aβ1-42 and Aβ1-40 in a multiplex setup. Participants were classified as either Aβ- or Aβ+, based on their CSF-derived concentration of Aβ42/40 ratio. The Aβ42/40 ratio has better diagnostic accuracy in distinguishing between AD and non-AD pathology compared to Aβ42 concentration alone (Hansson et al., Citation2019; Siafarikas et al., Citation2021). In line with published lab-specific cutoffs, participants with an Aβ42/40 ratio ≤0.077 were classified as Aβ+ (Siafarikas et al., Citation2021). Furthermore, participants were classified as Tau+ or Tau- based to their CSF-concentrations of p-tau. In accordance with a recently submitted manuscript from the DDI group (Knudtzon et al., Citation2023), participants with Innotest phospho-tau levels >62.5 were considered Tau+. For samples analyzed using Elecsys assays, p-tau concentrations >19 were classified as Tau+. Participants below these cutoffs were classified as Tau-. Cutoffs for the Elecsys assay were based on unpublished lab-specific cutoffs following receiver operating curve analyses within the DDI cohort on n = 22 Aβ- healthy controls versus n = 49 Aβ+ cognitively impaired cases (MCI, n = 43, dementia, n = 6).
Eighty-seven participants underwent lumbar punctures within three months of completing the 4MT. However, eight participants had a one to three-year delay between CSF-sampling and 4MT completion. Four of these participants completed the 4MT two to three years after undergoing CSF sampling. These participants were classified as Aβ+ and Tau + due to abnormal Aβ42/40 ratios and ptau levels. As Aβ-plaques and tau tangles are considered chronic, these participants likely remained Aβ+/Tau+ in the interim between the lumbar puncture and 4MT completion. Four participants who completed the 4MT one to two years after undergoing lumbar punctures were classified as Aβ- and Tau-. These participants had CSF-concentrations well into the normal range and performed normally on cognitive screening tests across examinations. Thus, they were likely Aβ-/Tau- when completing the 4MT. However, there is more uncertainty surrounding the biomarker classification of these individuals as CSF-concentrations of Aβ and p-tau can become pathological over time.
The current study sample
Our total sample comprised 95 Norwegian adults. The CN sample comprised 61 participants, while 34 were diagnosed with MCI. In the CN sample 39 participants were classified as Aβ- and 22 as Aβ+, while 44 were Tau- and 17 were Tau+. The MCI sample comprised 11 Aβ- and 23 Aβ+ participants, while 13 were Tau- and 21 were Tau+. For a visual representation of the sample, see . Before data collection, we performed power estimations using G*Power version 3.1 (Faul et al., Citation2007), based on effect sizes from Moodley et al. (Citation2015), who compared healthy controls, Aβ- MCI, Aβ+ MCI, and subjects with AD dementia. These estimations indicated that ten participants per group would be sufficient to detect between-group differences on the 4MT with a statistical power of at least .90 (unpublished data). Data collection was conducted between 2019 and 2021. The current sample is comprised of all participants with available 4MT scores, scores on all cognitive screening tests, demographic data, and CSF biomarker status (Aβ and Tau-status). Descriptive statistics are reported in .
Figure 1. Flowchart of participants grouped by cognitive status and Aβ and Tau status.
The flowchart illustrates how many Aβ-/ Aβ+ and Tau-/Tau + participants were included in the CN and MCI sub-samples. N = sample size; CN = cognitively normal; MCI = mild cognitive impairment; Aβ-/+ = CSF Aβ42/40 ratio above/below cutoff; Tau-/+ = CSF p-tau concentration below/above cutoff.
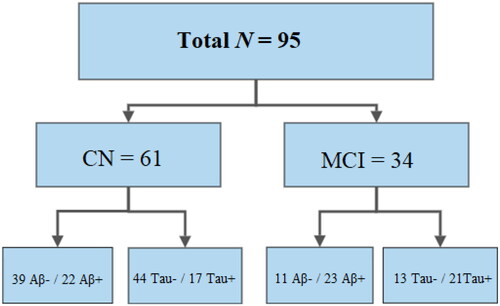
The 4 mountains test procedure (4MT)
The 4MT is a test of allocentric spatial working memory (Chan et al., Citation2016). The test was conducted on apple iPads (Model number: MR7F2KN/A) at each data collection site. Instructions were given verbally by a test administrator. The test required participants to use both working memory and spatial perception to identify a previously shown target image among three similar dummy images. The test consisted of a practice session and the main task. During the practice session, participants familiarized themselves with the task and received feedback from the test administrator. During the practice session, all responses were verbally confirmed or corrected, and a brief explanation of the correct image was given (see Supplementary Materials for the full Norwegian instruction). During the main task, participants were shown a computer-generated landscape consisting of four mountains. They were then told to memorize the landscape and the positions of the mountains shown in the target image. The target image was shown for eight seconds and followed by a two-second delay featuring a fixation cross on a blank white screen. After the delay, participants were shown four test images of landscapes featuring four mountains. One of the images showed the same landscape as the target image, but from a different angle, under different weather conditions, or at a different time of day. The three other images were dummy images portraying similar landscapes as the target image, but where the positions of the mountains relative to each other were changed. Participants responded by tapping their chosen image on the iPad. The test comprised 15 trials in total. If participants did not respond within 20 seconds the test automatically continued to the next trial. Though the test was automated, test administrators supervised test completion. The 4MT total score is comprised of the total number of correctly identified landscapes, with the highest possible score being 15. Test administrators were licensed psychologists, medical doctors, study nurses, and trained psychology students. All test administrators were trained in 4MT administration by an experienced licensed psychologist. For more information regarding the 4MT procedure and example images, see Hartley et al. (Citation2007).
As both the 4MT program and the test instruction were developed in English, we translated the instruction into Norwegian. The instruction was translated by clinical psychologist Bjørn-Eivind Kirsebom (Department of Neurology, University Hospital of North Norway, Tromsø Norway) (see Supplementary Materials).
CERAD word list memory test procedure
The Consortium to Establish a Registry for Alzheimer’s Disease (CERAD) word list memory test is a 10-word verbal memory test (Fillenbaum et al., Citation2008). The test comprises three learning trials where a list of ten words is presented verbally and visually (in writing) by the test administrator. After each learning trial participants are told to name as many words as possible. The third learning trial is followed by a 10-minute delay, after which participants are told to freely recall as many words as possible (recall trial). The CERAD recall score comprised the total number of words recalled after the 10-minute delay.
Ethical considerations
The DDI study was approved by the regional ethics committee for health research (REK sør-øst) from 2013–2025. The study was conducted in compliance with the Norwegian Health Research Act of 2009 and the Helsinki Declaration of 1964, revised in 2013. All participants were informed of the potential risks and benefits associated with study participation and signed written informed consent forms. They were also informed of their right to withdraw from the study. Data collection and storage were evaluated and approved by the data protection officer at the University Hospital of North Norway, and by the Norwegian Data Protection Authority (Datatilsynet).
Statistical analyses
Estimating the effects of Aβ-status, Tau-status, and demographics on 4MT and CERAD recall performance
Statistical analyses were performed in Rstudio using R version 4.2.1 and packages dplyr (Wickham, François, et al., Citation2022), tidyverse (Wickham et al., Citation2019), rsq (Zhang, Citation2022), car (Weisberg & Fox, Citation2019) and haven (Wickham, Miller, et al., Citation2022). Figures were made using ggplot2 (Wickham et al., Citation2016). To investigate if there were differences in demographics between biomarker groups, we performed independent sample t-tests. T-tests compared mean age and years of education in Aβ- vs. Aβ+, and Tau- vs. Tau + participants in the CN and MCI sub-samples, respectively. To investigate if the distribution of sex differed between Aβ-/Aβ+, or Tau-/Tau + participants, we performed Chi-square tests.
The influence of Aβ-status on 4MT and CERAD recall scores was examined through multiple regression analyses. Before analyses were performed, we ensured that assumptions for regression (linearity, homoscedasticity, normality) were fulfilled. To investigate the general effects of Aβ -status, Tau-status, and demographics on 4MT and CERAD recall performance analyses were first conducted in the full sample. Afterwards analyses were repeated in the cognitive subsamples (CN sample and MCI sample). Aβ-status (0 = Aβ-, 1 = Aβ+) was included as the predictor of interest, and age, years of education and sex (0 = male, 1 = female) were included as covariates. Analyses were first performed with 4MT as the outcome variable and then repeated with CERAD recall as the outcome variable.
To assess the effect of Tau-status on 4MT and CERAD recall performance, we repeated the same statistical procedure as described above, but with Tau-status (Tau- = 0, Tau+ = 1) as the predictor of interest. Like before, analyses were first performed in the full sample and then repeated separately in the CN and MCI sub-samples. As all analyses were based on specific hypotheses, we did not correct for multiple comparisons. The significance level was set to the conventional alpha level of .05, and all p-values <.05 were deemed significant.
Correlations between the 4MT and cognitive screening tests
The 4MT is a novel cognitive test and is rarely used in clinical settings. Therefore, we wanted to further examine the relationship between the 4MT and tests of verbal fluency, visuo-spatial perception, psychomotor speed, cognitive flexibility, and episodic verbal memory. We calculated Pearson correlation coefficients between 4MT scores and test scores from the DDI cognitive screening battery in the full sample. The screening battery included CERAD word list memory recall, the FAS phonemic fluency test, Trail Making tests A and B, and the VOSP silhouettes test.
Results
The effects of Aβ-status and Tau-status on 4MT and CERAD recall scores
Descriptive statistics and results from t-tests are reported in . T-tests showed small demographic differences between Aβ- and Aβ+, and Tau- and Tau + participants in both the CN and MCI sample (see ). Thus, regression analyses investigating differences in 4MT and CERAD recall performance between Aβ- and Aβ+, and Tau- and Tau+ participants were adjusted for demographic factors age, years of education and sex.
illustrates 4MT and CERAD recall performance in Aβ- and Aβ+ and Tau- and Tau+ participants in the full sample and cognitive sub-samples. 4MT performance did not differ between Aβ-/Aβ+ nor Tau-/Tau+ participants in the full sample analyses, nor in the CN or MCI sub-samples (see ).
Figure 2. 4MT and CERAD recall scores in Aβ-/Aβ+ and Tau- /Tau + participants across All samples. The box plots show differences in 4MT and CERAD recall scores between Aβ- (blue) and Aβ+ (red), and between Tau- (green) and Tau+ (yellow) participants in the full sample (A, B, C, D), the CN sample (E, F, G, H), and the MCI sample (I, J, K, L). All analyses are adjusted for differences in age, years of education, and sex. Black dots indicate the adjusted mean for each group. The only significant difference was found in the full sample (B and D), where Aβ+ and Tau + participants performed worse on the CERAD recall compared to Aβ- and Tau- participants. p = p-value; Aβ-/+ = CSF Aβ42/40 ratio above/below cutoff; Tau-/+ = CSF p-tau concentration below/above cutoff; 4MT score = 4 Mountain Test total score; CERAD recall = CERAD word list memory test recall score.
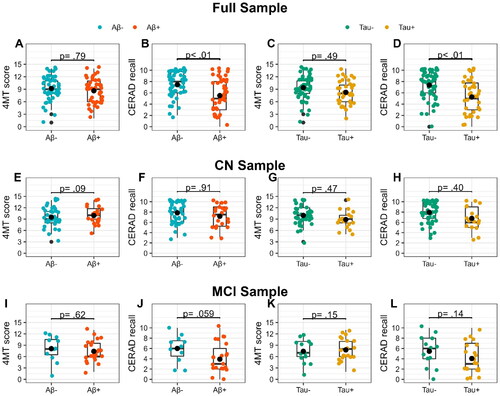
Table 2. Regression models for 4MT and CERAD recall across samples.
In the full sample analyses, CERAD recall performance was significantly worse among Aβ+ compared to Aβ- (b = −1.86, p <.01) (R2 = 0.14, F(4,90) = 3.7, p <.01) and Tau + compared to Tau- participants (b = −2.1, p <. 001)(R2 = 0.14, F(4, 90) = 3.9, p <.001). No demographic variable influenced CERAD recall scores in the full sample analyses. The CN and MCI sub-sample analyses showed no difference in CERAD recall performance between Aβ- vs. Aβ+ or Tau- vs. Tau + participants. However, in the MCI sample the effect of Aβ-status on CERAD recall approached the threshold for statistical significance (b = −2.1, p = .059).
Effects of age, years of education and sex on 4MT and CERAD recall performance
In analyses with Aβ-status as the predictor, both in the full sample and the CN sub-sample, lower scores on the 4MT were associated with higher age. Age accounted for 9% of the variance in the full sample (b = −0.10, p <.01) (R2 = 0.12, F(4, 90) = 3.0, p <.05) and 14% of the variance in the CN sample (b = −0.12, p <.001)(R2 = 0.18, F(4, 56) = 3.0, p <.05). In analyses with Tau-status as the predictor of interest, age was also the only significant demographic factor. Age accounted for 8% of the variance in the full sample (b = −0.09, p <.01) (R2 = 0.12, F(4, 90) = 3.1, p <.05) and 9% in the CN sample (b = −0.09, p <.05) (R2 = 0.14, F(4, 56) =2.3, p =.07). No other demographic variable significantly influenced 4MT scores.
In the CN sample analyses with Aβ-status as the predictor of interest, better CERAD recall performance was associated with lower age (b = −0.8, p <.05), more years of education (b = 0.17, p <.05) and female sex (b = 1.09, p <.05) (R2 = 0.28, F(4, 56) = 5.5, p <.001). In models with Tau-status as the predictor of interest, better CERAD recall performance was again associated with lower age (b = −0.07, p <.05) and female sex (b = 1.1, p <.05) in the CN sample (R2 = 0.29, F(4, 56) = 5.7, p <.001). CERAD recall scores were unaffected by demographics in the MCI sample, irrespective of Aβ and Tau-status. See for detailed regression models and effect sizes.
Correlational analyses between 4MT scores and cognitive screening tests
Better 4MT performance was associated with faster TMT-A and TMT-B completion time, as well as higher VOSP silhouette scores. There were no significant correlations between 4MT scores and CERAD recall or FAS verbal fluency scores. Correlation plots with r-values and corresponding p-values are presented in .
Figure 3. Plots of correlations between 4MT scores and cognitive screening tests in the full sample. The plots show correlations between 4MT performance and the VOSP silhouettes test (a), Trail Making Test-A (B), Trail Making Test-B (C), FAS verbal fluency test (D) and CERAD recall scores. Only correlations in plots A, B and C were significant. r = Pearson correlation coefficient; p = p-value.
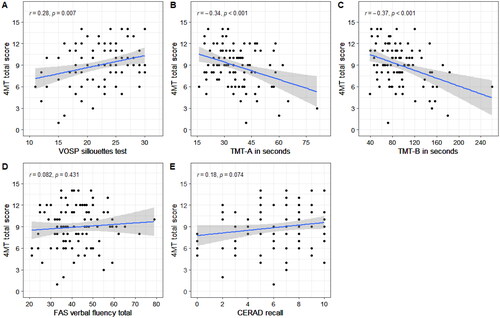
Discussion
In contrast to our main hypotheses, 4MT scores did not differentiate between Aβ-/Aβ+ nor between Tau-/Tau + participants, irrespective of cognitive status. CERAD recall performance was worse in Aβ+ compared to Aβ- participants, and Tau + compared to Tau- participants in the full sample analyses, but not in the sub-samples stratified by cognitive status. In the full sample, correlational analyses showed significant associations between 4MT scores and VOSP, TMT-A, and TMT-B performance, but not with CERAD recall.
Previously, the 4MT has been shown to differentiate between Aβ- and Aβ+ participants with MCI (Moodley et al., Citation2015). Moodley et al. (Citation2015) found significantly lower 4MT scores in Aβ+ compared to Aβ- MCI participants, while other neuropsychological test scores remained equal between groups. Despite the overall similarities between our samples (similar mean age, years of education, sex distribution) we did not reproduce the Moodley et al. (Citation2015) results. The differing results between these studies may be driven by sample characteristics and differences in biomarker classification. The 4MT is a novel test and has mostly been used in studies with small sample sizes. The study by Moodley et al. (Citation2015) includes only ten Aβ+ and nine Aβ- MCI participants. In contrast, our study includes twice as many Aβ+ MCI participants (n = 23). Smaller samples are often more prone to sample characteristics influencing results. Based on sample size alone one would expect our current study to be more sensitive to between-group differences compared to Moodley et al. (Citation2015). One other study have shown that poor 4MT performance predicts dementia risk scores (Ritchie et al., Citation2018). However, few large-scale studies have used the 4MT to compare Aβ+ and Aβ- MCI participants.
Furthermore, biomarker classification differs between our study and the Moodley et al. (Citation2015) study. Moodley et al. (Citation2015) defined biomarker status based on CSF-derived Aβ42 concentration, p-tau concentration, and the p-tau/Aβ42 ratio. In comparison, our participants were stratified according to their ratio of Aβ42/40, while Tau-status was examined in separate analyses. By defining biomarker status based on both Aβ and p-tau markers, one could argue that the Moodley et al. (Citation2015) sample may comprise individuals with more AD-specific brain pathology. Studies have shown that the accumulation of tau tangles in the EC is related to local atrophy in the EC (Berron et al., Citation2021), as well as lower synaptic density in the hippocampus (Mecca et al., Citation2022). In mouse models, tau tangle pathology has been shown to impair spatial memory and grid-cell function in aging mice, but not younger mice (Fu et al., Citation2017). Tau tangles might therefore contribute to impaired spatial memory in the Moodley et al. (Citation2015) study. However, descriptive analyses of our MCI sample showed that of 78% of Aβ+ participants were also Tau+ (see ). PET-imaging studies have shown increased neuronal loss in hippocampal areas in Aβ+ individuals compared to Aβ- controls (Bastin et al., Citation2020). Moreover, the Aβ42/40 ratio is considered more specific to AD pathology compared to Aβ42 concentration alone (Hansson et al., Citation2019), a finding that was recently confirmed in the DDI cohort (Siafarikas et al., Citation2021). In our analyses we investigated the effects of Tau-status on 4MT performance but found no significant differences between Tau- and Tau + participants. We thus find it unlikely that the results from Moodley et al. (Citation2015) are driven by more AD-specific pathology and tau tangle deposition, compared to our sample.
In contrast to the 4MT, CERAD recall scores differentiated between Aβ- and Aβ+ participants in the full sample analyses. Verbal episodic memory deficits are a well-known clinical feature of AD and have been shown in both prodromal and late-stage AD (Dubois et al., Citation2007; Ribeiro et al., Citation2007; Tromp et al., Citation2015). These findings somewhat contrast those of Moodley et al. (Citation2015), who did not find any differences between Aβ- and Aβ+ participants on the Rey Auditory Verbal Learning Test (RAVLT). The Moodley et al. (Citation2015) MCI participants showed low verbal memory performance, as they recalled 2.8 words on average (out of 15 possible). Given participants’ average education and age in the Moodley et al. (Citation2015) sample, such low scores indicate existing memory impairments in both Aβ- and Aβ+ MCI participants. The low RAVLT scores support the possibility that their Aβ+ MCI participants are further along the AD predementia trajectory. Curiously, their Aβ- MCI showed equally low verbal memory performance. Though our study also failed to find significant differences between Aβ- and Aβ+ MCI participants, the difference was apparent in the full sample analyses. Moreover, our MCI participants recalled more words in both Aβ- and Aβ+ groups on the shorter CERAD recall compared to Moodley et al. (Citation2015) (see for average raw scores between groups). Thus, the Moodley et al. (Citation2015) MCI sample might comprise more impaired participants than our sample, irrespective of Aβ-status.
Several studies have found deficits in tests of spatial navigation (Coughlan et al., Citation2018; Howett et al., Citation2019) and wayfinding (Allison et al., Citation2016) in MCI due to AD. Grid-cell dysfunction in the EC has been proposed as a possible mechanism driving early AD spatial deficits. The 4MT is hypothesized to be supported by hippocampal and entorhinal function (Hartley et al., Citation2007; Moodley et al., Citation2015), and 4MT scores have been shown to correlate with hippocampal volume and cortical thickness in the precuneus (Moodley et al., Citation2015). However, it is unknown if grid cell function is directly involved in 4MT completion. Many studies utilize other spatial tests, such as maze tests (Hort et al., Citation2007; Laczó et al., Citation2009; Tangen et al., Citation2022) or virtual reality (VR) tests (Allison et al., Citation2016; Howett et al., Citation2019). Howett et al. (Citation2019) found that MCI participants with AD biomarkers performed worse on a VR test of path integration compared to non-AD MCI. The same study found no differences in 4MT performance between groups. VR and maze tests commonly require participants to physically orient themselves and navigate, either in a virtual environment or in the lab setting. In contrast, the 4MT is conducted on an iPad, and does not require real-life navigation or physical movement. Spatial perspective taking is the ability to create cognitive representations of viewpoints other than your own, and has been shown to predict performance on navigational tasks (Kozhevnikov et al., Citation2006). Though the 4MT is thought to facilitate an allocentric spatial perspective and strategy, a study by Brucato et al. (Citation2023) reported that the 4MT showed a low correlation with other tests of spatial perspective taking. In the present study, we found significant relationships between 4MT scores and VOSP silhouette test scores, TMT-A and TMT-B performance. All of these tests have visuo-spatial components, but differ in tempo, visuo-motor, and visual perception demands. Performance on the TMT-B has also been linked to AD pathology, as it has been shown to predict conversion from MCI to Alzheimer’s Dementia (Rye et al., Citation2022). Taken together, our findings and the current literature indicate that the 4MT has visuo-spatial components. However, the precise neural underpinnings of the 4MT and its putative relation to grid-cell associated cognition remain unclear.
In cognitively normal participants we found no significant differences in the 4MT nor the CERAD recall, regardless of Aβ and Tau status. The Aβ+ CN participants are in the early preclinical stage of AD, where cognitive functions are thought to remain intact. Brain plasticity and cognitive flexibility might be compensating for increasing brain pathology in this sub-sample. Furthermore, Aβ-plaques and tau tangles do not immediately impair cognitive functions in the affected brain regions. AD biomarkers typically emerge 10 to 15 years before any clinical manifestation of cognitive impairment (Sperling et al., Citation2011). Pathophysiological changes in the early stages of the disease might not affect cognitive processes in a way that is detectable by cognitive testing. Indeed, several studies have found no cognitive impairment in individuals with confirmed AD biomarkers (Bennett et al., Citation2006; Besson et al., Citation2015; Price & Morris, Citation1999). While both Aβ-plaques and tau tangles are considerable risk factors for developing cognitive impairment and dementia, complex factors like cognitive reserve, genetic vulnerability, and cardiovascular health also influence disease mechanisms and cognitive outcomes (Dubois et al., Citation2010).
As the 4MT is a novel test, few studies have examined how demographic factors affect test scores. Our sample showed an effect of age on 4MT scores in the CN sample, favoring younger participants. These findings are in line with previous studies of spatial cognition in aging populations, where performance on spatial tasks change with age (Driscoll et al., Citation2005; Gazova et al., Citation2013; Moffat et al., Citation2006; Rodgers et al., Citation2012). These changes have been observed in healthy elderly, where older individuals prefer egocentric over allocentric navigation strategies (Colombo et al., Citation2017; Rodgers et al., Citation2012). Older individuals generally show slower processing speed on cognitive tests (Salthouse, Citation2000), and slower response time on spatial tasks (Colombo et al., Citation2017). While we did not analyze response time in the present study, the 4MT requires participants to respond within 20 seconds. The timed aspects of the task might therefore contribute to the age effect. Moreover, the 4MT landscapes are constructed digitally and the task is administered on an iPad. For older participants, the computerized imagery might be unfamiliar and increase test difficulty. This was supported by clinical observations during test administration. Though the test was well tolerated among participants, older participants were often unfamiliar with the test format and needed a thorough explanation of the testing procedure. Descriptive analyses of the 4MT total score revealed that the variable was normally distributed (data not shown), but that no participant obtained the maximum possible score. Thus, both test demands and the test format could have contributed to the age effect in the CN sample.
Interestingly, we did not find the effects of sex nor education on 4MT scores in the CN, nor the MCI sample. Some studies report a male advantage on visuo-spatial tests, including spatial tasks involving working memory (Coluccia & Louse, Citation2004; Voyer et al., Citation2017). Brucato et al. (Citation2023) reported a significant difference in 4MT performance favoring males in a sample of healthy younger adults. Furthermore, tests of mental rotation are known to be influenced by sex (Collins & Kimura, Citation1997). Though the 4MT is a test of spatial working memory, the test is comprised of visual images where the landscapes have been rotated or the viewpoint has been changed. For some trials, mental rotation would be a plausible cognitive strategy for task completion. As there was no effect of sex, the task might place higher demands on other cognitive processes, like working memory, rather than mental rotation. The present study did not collect data regarding which strategy participants used to complete the test. Thus, we do not know whether participants used mental rotation or other strategies during the 4MT.
In contrast to the 4MT, CERAD recall showed larger influences of demographics on test performance. Verbal memory tests are known to be affected by demographic factors. Indeed, a previous study based on DDI data by Kirsebom et al. (Citation2019) demonstrated that CERAD recall scores were influenced by age, sex, and years of education. Thus, the effects of demographics on CERAD recall performance in the CN sample are expected. However, in the MCI sample demographic factors no longer influenced performance on CERAD recall. MCI participants had objective impairment on at least one test from the DDI cognitive screening battery. The MCI participants recalled fewer words compared to CN participants, as shown by the difference between intercepts in . The participants’ cognitive impairment has possibly canceled out the effects of demographics on CERAD recall performance in the MCI sample. Interestingly, a similar change happens to the effect of age on 4MT scores in the MCI sample. MCI participants generally performed worse on the 4MT compared to CN participants, also shown through the different intercepts in . Thus, cognitive impairment possibly attenuates the demographic influence on both 4MT and CERAD recall scores.
The present study has some limitations. Though we fulfilled the initial sample size requirement calculated in the power analysis, our sample might be somewhat limited. For a more powerful comparison, we would have liked to include more Aβ+ CN participants and Aβ- MCI participants. A larger sample size would facilitate more sophisticated statistical analyses and allow us to examine possible interaction effects between Aβ and tau-tangle pathology. Furthermore, the 4MT was developed in English and has no normative data that we know of. The test was translated into Norwegian for the purpose of this study, but on-screen test instructions remained in English. While the test was translated by a researcher with Norwegian as their native language, it was not back-translated into English. Finally, at the time of analyses, we only had MRI imaging data for a small subset of the participants, thus we could not include hippocampal volume in the analyses. Imaging data could have provided further insight into the relationship between AD-related brain pathology and 4MT performance.
In conclusion, the 4MT did not differentiate between Aβ- and Aβ+, nor between Tau- and Tau + participants, irrespective of cognitive status. In contradiction to previous literature (Moodley et al., Citation2015), the 4MT was outperformed as a marker of early AD pathology by a short verbal memory test. Consequently, the proposal that the 4MT is an early marker of predementia AD was not supported. More immersive tests with larger demands on spatial navigation might prove to be more sensitive to early AD pathology. Future research on the 4MT would benefit from larger samples, and possibly in-depth imaging data. Research into the precise neural mechanisms involved in the 4MT could bring further insight into its specificity and sensitivity to AD pathology, and its potential clinical usefulness in other conditions than AD.
Supplementary Materials
Download MS Word (17.3 KB)Acknowledgements
We would like to thank study nurse Erna Utnes (Akershus University hospital) for assistance with data collection and handling, and neurologist Grit Richter (University hospital of North-Norway) for essential help with clinical examinations during the data collection period.
Disclosure statement
No potential conflict of interest was reported by the author(s).
Data availability statement
The data used in this study is not openly available due to patient confidentiality. The data supporting these findings may be made available upon reasonable request from the corresponding author, pending ethical approval by the regional ethical committee (Helse Sør-Øst). The data is stored at a secure server (Tjenester for sensitive data, TSD) at the University of Oslo in compliance with the General Data Protection Regulation (GDPR).
Additional information
Funding
References
- Albert, M. S., DeKosky, S. T., Dickson, D., Dubois, B., Feldman, H. H., Fox, N. C., Gamst, A., Holtzman, D. M., Jagust, W. J., Petersen, R. C., Snyder, P. J., Carrillo, M. C., Thies, B., & Phelps, C. H. (2011). The diagnosis of mild cognitive impairment due to Alzheimer’s disease: Recommendations from the national institute on aging-Alzheimer’s association workgroups on diagnostic guidelines for Alzheimer’s disease. Alzheimer’s & Dementia : The Journal of the Alzheimer’s Association, 7(3), 270–279. https://doi.org/10.1016/j.jalz.2011.03.008
- Allison, S. L., Fagan, A. M., Morris, J. C., & Head, D. (2016). Spatial navigation in preclinical Alzheimer’s disease. Journal of Alzheimer’s Disease , 52(1), 77–90. https://doi.org/10.3233/JAD-150855
- Alzheimer’s Association. (2022). 2022 Alzheimer's disease facts and figures. Alzheimer's & Dementia.18 (3): 700–789. https://doi.org/10.1002/alz.12638
- Alzheimer’s Disease International. (2015). World Alzheimer Report 2015- The Global Impact of Dementia. https://www.alzint.org/u/WorldAlzheimerReport2015.pdf
- Arendt, T., Brückner, M. K., Morawski, M., Jäger, C., & Gertz, H.-J. (2015). Early neurone loss in Alzheimer’s disease: cortical or subcortical? Acta Neuropathologica Communications, 3(1), 10. https://doi.org/10.1186/s40478-015-0187-1
- Bastin, C., Bahri, M. A., Meyer, F., Manard, M., Delhaye, E., Plenevaux, A., Becker, G., Seret, A., Mella, C., Giacomelli, F., Degueldre, C., Balteau, E., Luxen, A., & Salmon, E. (2020). In vivo imaging of synaptic loss in Alzheimer’s disease with [18F]UCB-H positron emission tomography. European Journal of Nuclear Medicine and Molecular Imaging, 47(2), 390–402. https://doi.org/10.1007/s00259-019-04461-x
- Bennett, D. A., Schneider, J. A., Arvanitakis, Z., Kelly, J. F., Aggarwal, N. T., Shah, R. C., & Wilson, R. S. (2006). Neuropathology of older persons without cognitive impairment from two community-based studies. Neurology, 66(12), 1837–1844. https://doi.org/10.1212/01.wnl.0000219668.47116.e6
- Berron, D., Vogel, J. W., Insel, P. S., Pereira, J. B., Xie, L., Wisse, L. E. M., Yushkevich, P. A., Palmqvist, S., Mattsson-Carlgren, N., Stomrud, E., Smith, R., Strandberg, O., & Hansson, O. (2021). Early stages of tau pathology and its associations with functional connectivity, atrophy and memory. Brain : A Journal of Neurology, 144(9), 2771–2783. https://doi.org/10.1093/brain/awab114
- Besson, F. L., La Joie, R., Doeuvre, L., Gaubert, M., Mézenge, F., Egret, S., Landeau, B., Barré, L., Abbas, A., Ibazizene, M., de La Sayette, V., Desgranges, B., Eustache, F., & Chételat, G. (2015). Cognitive and brain profiles associated with current neuroimaging biomarkers of preclinical Alzheimer’s disease. The Journal of Neuroscience: The Official Journal of the Society for Neuroscience, 35(29), 10402–10411. https://doi.org/10.1523/jneurosci.0150-15.2015
- Bondi, M. W., Edmonds, E. C., Jak, A. J., Clark, L. R., Delano-Wood, L., McDonald, C. R., Nation, D. A., Libon, D. J., Au, R., Galasko, D., & Salmon, D. P. (2014). Neuropsychological criteria for mild cognitive impairment improves diagnostic precision, biomarker associations, and progression rates. Journal of Alzheimer’s Disease, 42(1), 275–289. https://doi.org/10.3233/jad-140276
- Braak, H., & Del Tredici, K. (2015). The preclinical phase of the pathological process underlying sporadic Alzheimer’s disease. Brain: A Journal of Neurology, 138(Pt 10), 2814–2833. https://doi.org/10.1093/brain/awv236
- Brucato, M., Frick, A., Pichelmann, S., Nazareth, A., & Newcombe, N. S. (2023). Measuring spatial perspective taking: Analysis of four measures using item response theory. Topics in Cognitive Science, 15(1), 46–74. https://doi.org/10.1111/tops.12597
- Brun, V. H., Otnass, M. K., Molden, S., Steffenach, H.-A., Witter, M. P., Moser, M.-B., & Moser, E. I. (2002). Place cells and place recognition maintained by direct entorhinal-hippocampal circuitry. Science, 296(5576), 2243–2246. https://doi.org/10.1126/science.1071089
- Burt, D. B., Zembar, M. J., & Niederehe, G. (1995). Depression and memory impairment: A meta-analysis of the association, its pattern, and specificity. Psychological Bulletin, 117(2), 285–305. https://doi.org/10.1037/0033-2909.117.2.285
- Chan, D., Gallaher, L. M., Moodley, K., Minati, L., Burgess, N., & Hartley, T. (2016). The 4 mountains test: A short test of spatial memory with high sensitivity for the diagnosis of pre-dementia Alzheimer’s disease. Journal of Visualized Experiments, (116), 54454. https://doi.org/10.3791/54454
- Collins, D. W., & Kimura, D. (1997). A large sex difference on a two-dimensional mental rotation task. Behavioral Neuroscience, 111(4), 845–849. https://doi.org/10.1037//0735-7044.111.4.845
- Colombo, D., Serino, S., Tuena, C., Pedroli, E., Dakanalis, A., Cipresso, P., & Riva, G. (2017). Egocentric and allocentric spatial reference frames in aging: A systematic review. Neuroscience and Biobehavioral Reviews, 80, 605–621. https://doi.org/10.1016/j.neubiorev.2017.07.012
- Coluccia, E., & Louse, G. (2004). Gender differences in spatial orientation: A review. Journal of Environmental Psychology, 24(3), 329–340. https://doi.org/10.1016/j.jenvp.2004.08.006
- Coughlan, G., Laczó, J., Hort, J., Minihane, A.-M., & Hornberger, M. (2018). Spatial navigation deficits—overlooked cognitive marker for preclinical Alzheimer disease? Nature Reviews. Neurology, 14(8), 496–506. https://doi.org/10.1038/s41582-018-0031-x
- Derbie, A. Y., Chau, B. K. H., Wong, C. H. Y., Chen, L.-D., Ting, K.-H., Lam, B. Y. H., Lee, T. M. C., Chan, C. C. H., & Smith, Y. (2021). Common and distinct neural trends of allocentric and egocentric spatial coding: An ALE meta-analysis []. The European Journal of Neuroscience, 53(11), 3672–3687. https://doi.org/10.1111/ejn.15240
- Driscoll, I., Hamilton, D. A., Yeo, R. A., Brooks, W. M., & Sutherland, R. J. (2005). Virtual navigation in humans: The impact of age, sex, and hormones on place learning. Hormones and Behavior, 47(3), 326–335. https://doi.org/10.1016/j.yhbeh.2004.11.013
- Dubois, B., Feldman, H. H., Jacova, C., Cummings, J. L., Dekosky, S. T., Barberger-Gateau, P., Delacourte, A., Frisoni, G., Fox, N. C., Galasko, D., Gauthier, S., Hampel, H., Jicha, G. A., Meguro, K., O’Brien, J., Pasquier, F., Robert, P., Rossor, M., Salloway, S., … Scheltens, P. (2010). Revising the definition of Alzheimer’s disease: A new lexicon. The Lancet. Neurology, 9(11), 1118–1127. https://doi.org/10.1016/S1474-4422(10)70223-4
- Dubois, B., Feldman, H. H., Jacova, C., Dekosky, S. T., Barberger-Gateau, P., Cummings, J., Delacourte, A., Galasko, D., Gauthier, S., Jicha, G., Meguro, K., O’brien, J., Pasquier, F., Robert, P., Rossor, M., Salloway, S., Stern, Y., Visser, P. J., & Scheltens, P. (2007). Research criteria for the diagnosis of Alzheimer’s disease: revising the NINCDS–ADRDA criteria. The Lancet. Neurology, 6(8), 734–746. https://doi.org/10.1016/S1474-4422(07)70178-3
- Dubois, B., Feldman, H. H., Jacova, C., Hampel, H., Molinuevo, J. L., Blennow, K., DeKosky, S. T., Gauthier, S., Selkoe, D., Bateman, R., Cappa, S., Crutch, S., Engelborghs, S., Frisoni, G. B., Fox, N. C., Galasko, D., Habert, M.-O., Jicha, G. A., Nordberg, A., … Cummings, J. L. (2014). Advancing research diagnostic criteria for Alzheimer’s disease: the IWG-2 criteria. The Lancet. Neurology, 13(6), 614–629. https://doi.org/10.1016/S1474-4422(14)70090-0
- Eliassen, I. V., Fladby, T., Kirsebom, B.-E., Waterloo, K., Eckerström, M., Wallin, A., Bråthen, G., Aarsland, D., & Hessen, E. (2020). Predictive and diagnostic utility of brief neuropsychological assessment in detecting Alzheimer’s pathology and progression to dementia. Neuropsychology, 34(8), 851–861. https://doi.org/10.1037/neu0000698
- Espenes, J., Hessen, E., Eliassen, I. V., Waterloo, K., Eckerström, M., Sando, S. B., Timón, S., Wallin, A., Fladby, T., & Kirsebom, B.-E. (2020). Demographically adjusted trail making test norms in a Scandinavian sample from 41 to 84 years. The Clinical Neuropsychologist, 34(sup1), 110–126. https://doi.org/10.1080/13854046.2020.1829068
- Faul, F., Erdfelder, E., Lang, A.-G., & Buchner, A. (2007). G*Power 3: A flexible statistical power analysis program for the social, behavioral, and biomedical sciences. Behavior Research Methods, 39(2), 175–191. https://doi.org/10.3758/BF03193146
- Fillenbaum, G. G., van Belle, G., Morris, J. C., Mohs, R. C., Mirra, S. S., Davis, P. C., Tariot, P. N., Silverman, J. M., Clark, C. M., Welsh-Bohmer, K. A., & Heyman, A. (2008). Consortium to establish a registry for Alzheimer’s disease (CERAD): the first twenty years. Alzheimer’s & Dementia : The Journal of the Alzheimer’s Association, 4(2), 96–109. https://doi.org/10.1016/j.jalz.2007.08.005
- Fladby, T., Pålhaugen, L., Selnes, P., Waterloo, K., Bråthen, G., Hessen, E., Almdahl, I. S., Arntzen, K.-A., Auning, E., Eliassen, C. F., Espenes, R., Grambaite, R., Grøntvedt, G. R., Johansen, K. K., Johnsen, S. H., Kalheim, L. F., Kirsebom, B.-E., Müller, K. I., Nakling, A. E., … Aarsland, D. (2017). Detecting at-risk Alzheimer’s disease cases. Journal of Alzheimer’s Disease, 60(1), 97–105. https://doi.org/10.3233/JAD-170231
- Fu, H., Rodriguez, G. A., Herman, M., Emrani, S., Nahmani, E., Barrett, G., Figueroa, H. Y., Goldberg, E., Hussaini, S. A., & Duff, K. E. (2017). Tau pathology induces excitatory neuron loss, grid cell dysfunction, and spatial memory deficits reminiscent of early Alzheimer’s disease. Neuron, 93(3), 533–541.e535. https://doi.org/10.1016/j.neuron.2016.12.023
- Fyhn, M., Molden, S., Witter, M. P., Moser, E. I., & Moser, M.-B. (2004). Spatial representation in the entorhinal cortex. Science, 305(5688), 1258–1264. https://doi.org/10.1126/science.1099901
- Gazova, I., Laczó, J., Rubinova, E., Mokrisova, I., Hyncicova, E., Andel, R., Vyhnalek, M., Sheardova, K., Coulson, E. J., & Hort, J. (2013). Spatial navigation in young versus older adults [Original Research]. Frontiers in Aging Neuroscience, 5, 94. https://doi.org/10.3389/fnagi.2013.00094
- Hadjichrysanthou, C., Evans, S., Bajaj, S., Siakallis, L. C., McRae-McKee, K., de Wolf, F., … Anderson, R. M, (2020). The dynamics of biomarkers across the clinical spectrum of Alzheimer’s disease. Alzheimer’s Research & Therapy, 12(1), 74. https://doi.org/10.1186/s13195-020-00636-z
- Hafting, T., Fyhn, M., Molden, S., Moser, M. B., & Moser, E. I. (2005). Microstructure of a spatial map in the entorhinal cortex. Nature, 436(7052), 801–806. https://doi.org/10.1038/nature03721
- Hansson, O., Lehmann, S., Otto, M., Zetterberg, H., & Lewczuk, P. (2019). Advantages and disadvantages of the use of the CSF Amyloid β (Aβ) 42/40 ratio in the diagnosis of Alzheimer’s Disease. Alzheimer’s Research & Therapy, 11(1), 34. https://doi.org/10.1186/s13195-019-0485-0
- Hartley, T., Bird, C. M., Chan, D., Cipolotti, L., Husain, M., Vargha-Khadem, F., & Burgess, N. (2007). The hippocampus is required for short-term topographical memory in humans. Hippocampus, 17(1), 34–48. https://doi.org/10.1002/hipo.20240
- Hort, J., Laczó, J., Vyhnálek, M., Bojar, M., Bureš, J., & Vlček, K. (2007). Spatial navigation deficit in amnestic mild cognitive impairment. Proceedings of the National Academy of Sciences of the United States of America, 104(10), 4042–4047. https://doi.org/10.1073/pnas.0611314104
- Howett, D., Castegnaro, A., Krzywicka, K., Hagman, J., Marchment, D., Henson, R., Rio, M., King, J. A., Burgess, N., & Chan, D. (2019). Differentiation of mild cognitive impairment using an entorhinal cortex-based test of virtual reality navigation. Brain : A Journal of Neurology, 142(6), 1751–1766. https://doi.org/10.1093/brain/awz116
- Jack, C. R., Bennett, D. A., Blennow, K., Carrillo, M. C., Dunn, B., Haeberlein, S. B., Holtzman, D. M., Jagust, W., Jessen, F., Karlawish, J., Liu, E., Molinuevo, J. L., Montine, T., Phelps, C., Rankin, K. P., Rowe, C. C., Scheltens, P., Siemers, E., Snyder, H. M., & Sperling, R, (2018). NIA-AA research framework: Toward a biological definition of Alzheimer’s disease. Alzheimer’s & Dementia: The Journal of the Alzheimer’s Association, 14(4), 535–562. https://doi.org/10.1016/j.jalz.2018.02.018
- Kirsebom, B.-E., Espenes, R., Hessen, E., Waterloo, K., Johnsen, S. H., Gundersen, E., Botne Sando, S., Rolfseng Grøntvedt, G., Timón, S., & Fladby, T. (2019). Demographically adjusted CERAD wordlist test norms in a Norwegian sample from 40 to 80 years. The Clinical Neuropsychologist, 33(sup1), 27–39. https://doi.org/10.1080/13854046.2019.1574902
- Knudtzon, S., Nordengen, K., Grøntvedt, G. R., Jarholm, J., Eliassen, I. V., Selnes, P., … Kirsebom, B.-E. (2023). Age-adjusted CSF t-tau and NfL have lower diagnostic accuracy for Alzheimer’s disease. [Manuscript submitted for publication].
- Kozhevnikov, M., Motes, M. A., Rasch, B., & Blajenkova, O. (2006). Perspective-taking vs. mental rotation transformations and how they predict spatial navigation performance []. Applied Cognitive Psychology, 20(3), 397–417. https://doi.org/10.1002/acp.1192
- Kunz, L., Schröder, T. N., Lee, H., Montag, C., Lachmann, B., Sariyska, R., Reuter, M., Stirnberg, R., Stöcker, T., Messing-Floeter, P. C., Fell, J., Doeller, C. F., & Axmacher, N. (2015). Reduced grid-cell like representations in adults at genetic risk for Alzheimer’s disease. Science , 350(6259), 430–433. https://doi.org/10.1126/science.aac8128
- Laczó, J., Vlcek, K., Vyhnálek, M., Vajnerová, O., Ort, M., Holmerová, I., Tolar, M., Andel, R., Bojar, M., & Hort, J. (2009). Spatial navigation testing discriminates two types of amnestic mild cognitive impairment. Behavioural Brain Research, 202(2), 252–259. https://doi.org/10.1016/j.bbr.2009.03.041
- Lorentzen, I. M., Espenes, J., Hessen, E., Waterloo, K., Bråthen, G., Timón, S., … Kirsebom, B.-E. (2021). Regression-based norms for the FAS phonemic fluency test for ages 40–84 based on a Norwegian sample. Applied Neuropsychology: Adult, 30 (2), 159–168. https://doi.org/10.1080/23279095.2021.1918128
- Maass, A., Lockhart, S. N., Harrison, T. M., Bell, R. K., Mellinger, T., Swinnerton, K., Baker, S. L., Rabinovici, G. D., & Jagust, W. J. (2018). Entorhinal tau pathology, episodic memory decline, and neurodegeneration in Aging. The Journal of Neuroscience: The Official Journal of the Society for Neuroscience, 38(3), 530–543. https://doi.org/10.1523/JNEUROSCI.2028-17.2017
- McNaughton, B. L., Battaglia, F. P., Jensen, O., Moser, E. I., & Moser, M.-B. (2006). Path integration and the neural basis of the ‘cognitive map. Nature Reviews. Neuroscience, 7(8), 663–678. https://doi.org/10.1038/nrn1932
- Mecca, A. P., Chen, M.-K., O’Dell, R. S., Naganawa, M., Toyonaga, T., Godek, T. A., Harris, J. E., Bartlett, H. H., Zhao, W., Banks, E. R., Ni, G. S., Rogers, K., Gallezot, J. D., Ropchan, J., Emery, P. R., Nabulsi, N. B., Vander Wyk, B. C., Arnsten, A. F. T., Huang, Y., Carson, R. E., & van Dyck, C. H. (2022). Association of entorhinal cortical tau deposition and hippocampal synaptic density in older individuals with normal cognition and early Alzheimer’s disease. Neurobiology of Aging, 111, 44–53. https://doi.org/10.1016/j.neurobiolaging.2021.11.004
- Moffat, S. D., Elkins, W., & Resnick, S. M. (2006). Age differences in the neural systems supporting human allocentric spatial navigation. Neurobiology of Aging, 27(7), 965–972. https://doi.org/10.1016/j.neurobiolaging.2005.05.011
- Moodley, K., Minati, L., Contarino, V., Prioni, S., Wood, R., Cooper, R., D’Incerti, L., Tagliavini, F., & Chan, D. (2015). Diagnostic differentiation of mild cognitive impairment due to Alzheimer’s disease using a hippocampus-dependent test of spatial memory. Hippocampus, 25(8), 939–951. https://doi.org/10.1002/hipo.22417
- Price, J. L., & Morris, J. C. (1999). Tangles and plaques in nondemented aging and “preclinical” Alzheimer’s disease. Annals of Neurology, 45(3), 358–368. https://doi.org/10.1002/1531-8249(199903)45:3 < 358::AID-ANA12 > 3.0.CO;2-X
- Reitan, R. M., & Wolfson, D. (1985). The Halstead-Reitan Neuropsychological Test Battery. Neuropsychology Press.
- Ribeiro, F., Guerreiro, M., & De Mendonça, A. (2007). Verbal learning and memory deficits in mild cognitive impairment. Journal of Clinical and Experimental Neuropsychology, 29(2), 187–197. https://doi.org/10.1080/13803390600629775
- Ritchie, K., Carrière, I., Howett, D., Su, L., Hornberger, M., O’Brien, J. T., Ritchie, C. W., & Chan, D. (2018). Allocentric and egocentric spatial processing in middle-aged adults at high risk of late-onset Alzheimer’s disease: The PREVENT dementia study. Journal of Alzheimer’s Disease, 65(3), 885–896. https://doi.org/10.3233/JAD-180432
- Rodgers, M. K., Sindone, J. A., & Moffat, S. D. (2012). Effects of age on navigation strategy. Neurobiology of Aging, 33(1), 202.e215–202.e222. https://doi.org/10.1016/j.neurobiolaging.2010.07.021
- Rye, I., Vik, A., Kocinski, M., Lundervold, A. S., & Lundervold, A. J. (2022). Predicting conversion to Alzheimer’s disease in individuals with Mild Cognitive Impairment using clinically transferable features. Scientific Reports, 12(1), 15566. https://doi.org/10.1038/s41598-022-18805-5
- Salthouse, T. A. (2000). Aging and measures of processing speed. Biological Psychology, 54(1–3), 35–54. https://doi.org/10.1016/s0301-0511(00)00052-1
- Siafarikas, N., Kirsebom, B.-E., Srivastava, D. P., Eriksson, C. M., Auning, E., Hessen, E., Selbaek, G., Blennow, K., Aarsland, D., & Fladby, T. (2021). Cerebrospinal fluid markers for synaptic function and Alzheimer type changes in late life depression. Scientific Reports, 11(1), 20375. https://doi.org/10.1038/s41598-021-99794-9
- Sperling, R. A., Aisen, P. S., Beckett, L. A., Bennett, D. A., Craft, S., Fagan, A. M., Iwatsubo, T., Jack, C. R., Kaye, J., Montine, T. J., Park, D. C., Reiman, E. M., Rowe, C. C., Siemers, E., Stern, Y., Yaffe, K., Carrillo, M. C., Thies, B., Morrison-Bogorad, M., Wagster, M. V., & Phelps, C. H. (2011). Toward defining the preclinical stages of Alzheimer’s disease: Recommendations from the national institute on aging-Alzheimer’s association workgroups on diagnostic guidelines for Alzheimer’s disease. Alzheimer’s & Dementia : The Journal of the Alzheimer’s Association, 7(3), 280–292. https://doi.org/10.1016/j.jalz.2011.03.003
- Spreen, O., & Benton, A. L. (1969). Neurosensory Center Comprehensive Examination for Aphasia: Manual of instructions (NECCEA). University of Victoria.
- Tangen, G. G., Nilsson, M. H., Stomrud, E., Palmqvist, S., & Hansson, O. (2022). Spatial navigation and its association with biomarkers and future dementia in memory clinic patients without dementia. Neurology, 99(19), e2081–e2091. https://doi.org/10.1212/wnl.0000000000201106
- Therriault, J., Pascoal, T. A., Lussier, F. Z., Tissot, C., Chamoun, M., Bezgin, G., Servaes, S., Benedet, A. L., Ashton, N. J., Karikari, T. K., Lantero-Rodriguez, J., Kunach, P., Wang, Y.-T., Fernandez-Arias, J., Massarweh, G., Vitali, P., Soucy, J.-P., Saha-Chaudhuri, P., Blennow, K., … Rosa-Neto, P. (2022). Biomarker modeling of Alzheimer’s disease using PET-based braak staging. Nature Aging, 2(6), 526–535. https://doi.org/10.1038/s43587-022-00204-0
- Tromp, D., Dufour, A., Lithfous, S., Pebayle, T., & Després, O. (2015). Episodic memory in normal aging and Alzheimer disease: Insights from imaging and behavioral studies. Ageing Research Reviews, 24(Pt B), 232–262. https://doi.org/10.1016/j.arr.2015.08.006
- Voyer, D., Voyer, S. D., & Saint-Aubin, J. (2017). Sex differences in visual-spatial working memory: A meta-analysis. Psychonomic Bulletin & Review, 24(2), 307–334. https://doi.org/10.3758/s13423-016-1085-7
- Warrington, E., & James, M. (1991). A new test of object decision: 2D silhouettes featuring a minimal view. Cortex, 27(3), 377–383. https://doi.org/10.1016/S0010-9452(13)80033-0
- Weisberg, S., & Fox, J. (2019). An R Companion to Applied Regression. Sage.
- Wickham, H., Averick, M., Bryan, J., Chang, W., McGowan, L., François, R., Grolemund, G., Hayes, A., Henry, L., Hester, J., Kuhn, M., Pedersen, T., Miller, E., Bache, S., Müller, K., Ooms, J., Robinson, D., Seidel, D., Spinu, V., … Yutani, H. (2019). Welcome to the tidyverse. Journal of Open Source Software, 4(43), 1686. https://doi.org/10.21105/joss.01686
- Wickham, H., Chang, W., Henry, L., Pedersen, T. L., Takahashi, K., Wilke, C., … Dunnington, D. (2016). ggplot2: Elegant graphics for data analysis. In Springer-Verlag.
- Wickham, H., François, R., Henry, L., & Müller, K. (2022). dplyr: A grammar of data manipulation. In https://dplyr.tidyverse.org, https://github.com/tidyverse/dplyr
- Wickham, H., Miller, E., & Smith, D. (2022). Haven: Import and export ‘SPSS’, ‘Stata’ and ‘SAS’ Files. In https://haven.tidyverse.org,https://github.com/tidyverse/haven,https://github.com/WizardMac/ReadStat.
- Yamanami-Irioka, A., Uchihara, T., Endo, T., Irioka, T., Watanabe, M., Kitagawa, M., & Mizusawa, H. (2011). Amnesia in frontotemporal dementia with amyotrophic lateral sclerosis, masquerading Alzheimer’s disease. Case Reports in Neurology, 3(3), 242–247. https://doi.org/10.1159/000331859
- Zhang, D. (2022). R-squared and related measures. In (Version 2.5) https://CRAN.R-project.org/package=rsq