Abstract
Heavily melanized gills in crustaceans, often referred to as black gill, have been reported in both wild and cultured marine species. Tissue melanization is generally the result of a response of the crustacean innate immune system to the presence of an irritant or pathogen. While black gill can be caused by a variety of abiotic stressors and nutritional deficiencies, biotic agents are the cause of most reported black gill cases in crustaceans. In high density culture systems, fungi are identified as the most common causes of black gill outbreaks. In the wild, epidemic-scale outbreaks of black gill appear largely to be caused by ciliate rather than fungal infections. Black gill epidemics caused by ciliates have recently been reported in two commercially important fishery species including penaeid shrimp in the South Atlantic Bight USA (Western North Atlantic) and the Gulf of Mexico, and in pandalid shrimp in the Gulf of Maine, USA. Here we review the reports of the occurrence, causative agents, biology, ecology, and impacts of black gill on wild crustacean species of black gill with special focus on the pandalid shrimp species Pandalus borealis parasitized by the apostome ciliate Synophrya sp. and penaeid shrimp in the Western North Atlantic and Gulf of Mexico parasitized by a newly described apostome ciliate species Hyalophysa lynni. A review of the literature reveals large knowledge gaps with respect to black gill in both commercially exploited and other keystone crustacean species. Recommendations for future research include improved surveillance and identification of causative agents of black gill, improved understanding of their interactions with crustacean hosts including distribution, transmission, morbidity, and mortality, epidemiology, molecular biology, and relationship with climate.
Introduction
Heavily pigmented gills, a condition referred to as black gill, black spot, black necrotic disease, black spot gill syndrome, brown gill, or black death, has been observed in a variety of crustaceans, with epidemics in some penaeid and pandalid shrimp populations. In this review we refer to the condition as black gill. The dark black or brown color of the gills is due to melanin production that is an important component of the crustacean immune response (Jiravanichpaisal et al. Citation2006). There are reports of the condition in both cultured and wild crustaceans. In culture systems, black gill is most commonly due to exposure to both pathogens and abiotic stressors associated with high-density low health systems (Pramanik and Mohanty, Citation2015; Smolowitz et al. Citation1992). Much less is known about black gill in wild populations and is therefore the primary focus of this review.
In wild populations, mechanisms of pathogenesis and environmental drivers of black gill have been reported in amphipods, penaeid shrimp, pandalid shrimp and portunid crabs (Comely and Ansell Citation1989; Frischer et al. Citation2017, Citation2018; Haefner and Spacher Citation1985; Lee et al. Citation2019; Rio-Rodriguez et al. Citation2013; Spicer Citation2013). The cause of black gill in wild populations is often not known, but when determined it is often due to the presence of ciliated protozoans. Black gill outbreaks have been associated with significant fishery declines in crustaceans from the South Atlantic Bight (SAB), Middle Atlantic Shelf of USA, Gulf of Mexico, Gulf of Maine, maritime provinces, Canada, Greenland, Scotland, and coastal France (). Recent reports of black gill outbreaks involve Pandalus borealis, Litopenaeus setiferus and Farfantepenaeus aztecus.
Figure 1. Geographic distribution of different species of penaeid and pandalid shrimp. Worldwide reports of black gill from various shrimp and crab species indicated with red dots.
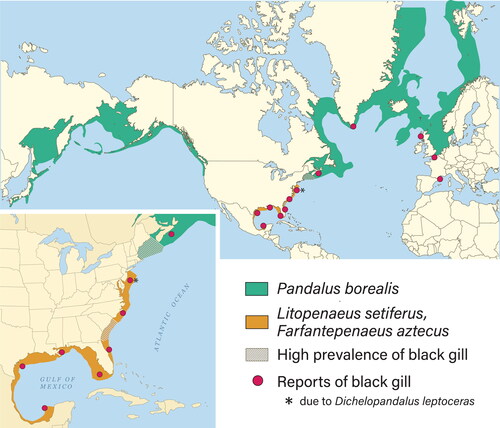
While this review focuses on black gill in marine crustaceans, the condition also affects freshwater species including for example, the freshwater prawn Macrobrachium rosenbergii, the crayfish, Austropotamobius pallipes, the freshwater crab, Eriocheir sinensis and many others (Alderman and Polglase Citation1985; Burns et al. Citation1979; Lester and Paynter Citation1989; Zhang and Bonami Citation2007). In addition to gills, heavy melanization has also been noted in other tissues (Hibbits and Sparks Citation1983; Johnson Citation1985). Another example of heavy melanization, not discussed in this review, is melanosis or “black spot”. This condition refers to the development of dark pigments in the exoskeletons of post-capture shrimp and lobsters and is associated with postmortem enzymatic processes rather than disease (Bartolo and Birk Citation1998; Goncalves and Menezes de Oliveira Citation2016; Ogawa et al. Citation1984; Stentiford and Neil Citation2011).
The organization of this review includes a description of the melanization process associated with black gill, causes of black gill in both wild and cultured crustaceans, life history of ciliates linked to black gill, transmission of black gill ciliates, seasonal changes in black gill, macroscopic and microscopic pathology of black gill, the effects of black gill on morbidity and mortality, and recommended future studies.
Melanin production associated with the crustacean immune response
The production of melanin is associated with the response of the crustacean innate immune system. The immune response generally involves phagocytosis, encapsulation and nodule formation and is often accompanied by intense melanization (Cerenius et al. Citation2010; Hauton Citation2012; Jiravanichpaisal et al. Citation2006). The key enzyme in the synthesis of melanin is phenoloxidase, present in hemocytes as an inactive pro-enzyme prophenoloxidase (proPO). Molecular patterns on the surface of the invading pathogens are recognized by circulating pattern recognition proteins that trigger a cascade of downstream molecular events (Cerenius and Söderhall Citation2018). These events lead to an activating enzyme which converts proPO to the active phenoloxidase which then oxidizes tyrosine to quinones that polymerize to form the insoluble black pigment, melanin (Amparyup et al. Citation2013; Holmblad and Söderhäll Citation1999; Radcliffe Citation1991, Sritunyalucksana and Söderhäll Citation2000). The free radicals and quinones, produced during the polymerization process, can be toxic to an invader (Vaseeharan et al. Citation2016). A wall of melanized fused hemocytes can encapsulate and isolate an invader or eliminate foreign abiotic materials from the host (Martin et al. Citation2000). Because the production of melanin is associated with a nonspecific immune response, the presence of melanized tissue is indicative of an active immune reaction but is not itself a specific diagnosis. Visible black gill is therefore not a disease but a generalized symptom that can be associated with a disease process if a pathogen responsible for tissue pathology is the cause.
Abiotic causes of black gill
While the main function of the crustacean immune system is to destroy foreign biological invaders, it can also be activated by a variety of abiotic stressors including injury, exposure to heavy metals and other toxins, nutritional deficiencies, and non-biological foreign bodies. For example, high ammonia, high nitrite, or a diet deficiency resulted in black gill in pond raised penaeid shrimp (Lightner Citation1985; Lightner and Redman Citation1977; Magarelli et al. Citation1979). Ink particles injected into the hemolymph of lobster, Homarus americanus, resulted in formation of nodules containing melanized ink particles (Martin et al. Citation2000). Exposure to high concentrations of metals (copper, cadmium, nickel, zinc) can cause penaeid and palaemonid shrimp to develop black gill (Denton and Campbell Citation1990; Frias-Espericueta et al. Citation2008: Fontaine and Lightner Citation1975; Lightner and Redman Citation1977; Nimmo et al. Citation1977; Rao and Doughtie Citation1984; Rao et al. Citation1982; Wu et al. Citation2009). Black gill was noted in banana shrimp (Fenneropenaeus merguiensis), pink shrimp (Farfantepenaeus duorarum) and Pacific white shrimp (Litopenaeus vannamei), exposed to between 0.5 and 0.7 mg/L of cadmium (Couch Citation1977; Frias-Espericueta et al. Citation2008; Nimmo et al. Citation1977). Some melanization could have been the result of secondary infection by the fungus, Fusarium sp., which was found in some of the cadmium exposed individuals. High concentrations of some organic toxicants (e.g. pentachlorophenol, dithiocarbamates), produced black gill and gill necrosis in grass shrimp, Palaemonetes pugio (Rao and Doughtie Citation1984). It should be noted, however, that most studies involving abiotic stressors causing gill melanization involved captive crustaceans maintained at high densities and exposed to unrealistically high concentrations of organic and inorganic toxicants not representative of environmental concentrations. It is therefore unclear whether these observations are relevant to wild populations where population densities and toxicant concentrations are generally lower. There have been, however, reports of crustaceans with black gill reported from highly polluted environments. Sinderman (Citation1989), for example, reported black gill in brachyuran crabs collected from sewage sludge dumping areas. The molecular mechanisms that trigger melanin deposition in crustaceans exposed to high concentrations of metals and other toxicants has yet to be determined and needs further investigation.
Biotic causes of black gill
A large diversity of biotic agents are also known to cause black gill. Bacteria, viruses, fungi, and ciliated protists are the most commonly reported black gill-causing infectious agents in crustaceans. In culture, fungal infections are the most common cause of black gill but have also been reported in wild species. For example, black gill in the pandalid shrimp, Dichelopandalus leptocerus, from the east coast of Canada and northeastern United States was found to be caused by a chytrid-like phycomycete (Uzmann and Haynes Citation1968).
While there are a limited number of reports of fungal-caused black gill in wild crustacean populations, there is an extensive body of literature on fungal infections causing black gill in pond-raised shrimp and other captive-reared crustaceans (Bian and Egusa Citation1981; Dewangan et al., Citation2015; Overstreet Citation1973; Egusa and Ueda Citation1972, Chun Citation1980; Khoa et al. Citation2004; Hatai Citation2012; Karthikeyan et al., Citation2015; Lightner et al. Citation1975; Lightner and Redman Citation1977; Nha et al. Citation2009: Rhoobunjongde et al. Citation1991), tank-raised lobsters (Fisher et al. Citation1978; Lightner and Fontaine Citation1975) and tank-raised hermit crabs (Smolowitz et al. Citation1992). The fungi responsible for the black gill in these cultured species included Aspergillus flavus, Aspergillus awamori, Fusarium moniliforme, Fusarium solani and Fusarium incarnatum. Fusarium spp. are common in land and aquatic biota throughout the world and there are numerous reports of Fusarium disease in cultured penaeid shrimp (Brock and Lightner Citation1990; Cruz da Silva et al. Citation2011).
Although there are few reports of the extent of fungal infections in wild crustacean species, a recent examination of the gill eukaryotic microbiome from a small number of wild-caught white shrimp (L. setiferus) in the Wassaw Sound estuary in Georgia, USA indicated the presence of 10 different potentially pathogenic fungal taxa (Frischer et al. Citation2017). It therefore can be speculated that under stressful conditions, normally benign fungal associates may become problematic resulting in the activation of the crustacean immune system and black gill. This likely explains the prevalence of fungal-caused black gill from high density commercial shrimp ponds. For example, Egusa and Ueda (Citation1972) reported that Penaeus japonicus held at high densities in a pond with low oxygen concentrations had high Fusarium infection rates. Thus, the treatment for confined crustaceans that exhibit signs of fungal infections is to lower the densities and provide better diet and sanitation conditions (Brock and Lightner Citation1990; Lightner Citation1985; Lightner et al. Citation1975). Additional investigation of potentially pathogenic fungal species and more targeted treatment is warranted.
There are fewer reports of black gill in crustacean species caused by viruses. Viruses are, however, known to be capable of triggering the crustacean immune system (Hauton Citation2012) and thus, theoretically may result in black gill symptoms. Viral-induced black gill has been reported in at least one crab species in culture. Cultured Chinese midden crabs (Eriocheir sinensis) can develop gills with gray or black coloration, referred to as black gill syndrome. This disease is due to an infection by the Eriocheir sinensis ronivirus, a member of the emerging Nidovirus group (Kumar et al. Citation2020; Bateman and Stentiford Citation2017; Zhang and Bonami Citation2007). In general, however, the melanization response is generally not initiated or is actively suppressed by viral infections in decapod crustaceans (Kulkarni et al. Citation2021; Vogt Citation2020).
Gill infestations of ciliated protists (ciliates) are commonly associated with both wild and cultured crustaceans. Peritrich and apostome ciliates are most commonly reported and, whereas peritrich ciliates are generally benign and are not associated with pathology, several apostome ciliates are well known crustacean pathogens (Chatton and Lwoff Citation1935; Johnson and Bradbury Citation1976). Various peritrich ciliates, including Zoothamnium sp., are commonly attached to the gills of decapods in the wild but there is generally no response by the host to their presence () (Couch Citation1966; Foster et al. Citation1978; Ma and Overstreet Citation2006; Overstreet Citation1987). Shrimp kept in dense, confined conditions, however, can exhibit melanized gills due to intense gill infestation by peritrichs, including Lagenophrys sp. and Zoothamnium sp. (Couch Citation1978; Fontaine Citation1985; Foster et al. Citation1978; Johnson et al. Citation2009; Lightner et al. Citation1975).
Figure 2. Peritrich ciliates (arrows) attached to the gill via stalks to their white shrimp (Litopenaeus setiferus) host. Peritrich ciliates typically do not invade host tissue nor elicit a host cellular response. Collected in coastal Georgia (USA). Hematoxylin and Eosin (H & E). Scale bar 50 µm.
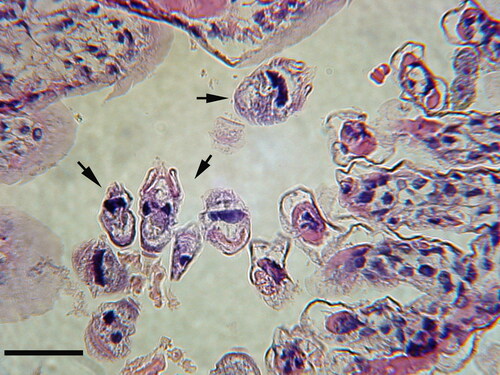
Some ciliate taxa invade the gills of crustacean but do not cause the host to produce melanin. These ciliates include those that are pathogens, as well as species which are harmless to the host. For example, the ciliate, Anophryoides haemophila, after entrance into the gills of the American lobster, H. americanus, produces lesions but does not result in gill melanization (Athanassopoulou et al. Citation2004). Similarly, melanin deposits were not associated with the presence of the pathogenic ciliate, Mesanophrys chesapeakensis, later identified as Orchitophrya stellarum (Small et al. Citation2013), in the gills of the crab, Callinectes sapidus, even though nodules were present in the gill hemolymph (Messick and Small Citation1996). The ciliate Paranophrys sp. invades and damages the gills of Dungeness crab, Cancer magister, but histological examination did not find melanization in the gills (Sparks et al. Citation1982). Various apostome ciliates, including species of Gymnodinioides and Hyalophysa, are often found in high numbers attached to the gills of crustaceans. They are clearly attached to the gills but do not harm their hosts () (Bradbury Citation1966, Citation1994; Bradbury and Clamp Citation1973; Grimes Citation1976; Landers Citation1991; Ohtsuka et al., Citation2015). Thus, commensal ciliates attached to the gills do not elicit an immune response, while some pathogenic ciliates may evade or suppress the crustacean immune system so that melanization does not occur. The last situation may be especially true in animals challenged by other stressors.
Figure 3. Hyalophysa chattoni (arrows) attached to the gills of grass shrimp, Palaemonetes pugio, collected in coastal Georgia (USA). Ciliates have not invaded host tissue nor elicited a host cellular response. H & E. Scale bar 50 µm.
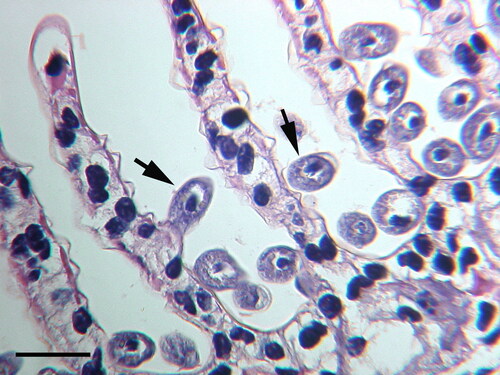
Alternatively, some apostome species, particularly species that are capable of histotrophic feeding on live tissue, have been associated with black gill. Apostome ciliates exhibit complex life histories, including an encysted phoretic stage and an active feeding trophont stage. Apostome ciliates are known to parasitize a wide variety of crustaceans (Chatton and Lwoff Citation1935; Johnson and Bradbury Citation1976). Chatton and Lwoff (Citation1935) published a monograph on this protozoan group and separated the group according to their life history and diet. Early studies of apostome ciliate infections in penaeid shrimp with black gill reported shrimp heavily infected with phoronts of an unidentified apostome ciliate. (Couch Citation1978; Lagniappe Citation2000). More recent work, however, identified these ciliates as encysted trophonts of a newly described apostome ciliate species, Hyalophysa lynni, the agent responsible for black gill in white and brown shrimp (L. setiferus and F. aztecus), in the SAB (Frischer et al. Citation2017, Citation2018; Landers et al. Citation2020; Patel and Landers Citation2019). In these more recent studies, actively growing and dividing H. lynni encysted trophonts were commonly associated with shrimp gill tissues suggestive of active histotrophic feeding (Landers et al. Citation2020). Although questions regarding the life history of H. lynni remain, histotrophic feeding is likely the cause of black gill symptoms in infected penaeid shrimp.
Black gill caused by H. lynni has been extensively reported in coastal Georgia, South Carolina, and Texas USA in L. setiferus and F. aztecus where it can reach epidemic proportions. Black gill caused by H. lynni has been confirmed in all penaeid shrimp species that have been examined throughout the Gulf of Mexico and in the Western North Atlantic coastal ocean and estuaries from the Florida Keys north to the Chesapeake Bay including the commercial important species the White shrimp (L. setiferus), Brown shrimp (F. aztecus), Pink shrimp (Farfantepenaeus duorarum), Tiger shrimp (Penaeus monodon), and Atlantic seabob shrimp (Xiphopenaeus kroyeri) (Frischer unpublished observations). Infections of H. lynni were confirmed by DNA sequencing and microscopy as previously described (Frischer et al. Citation2017).
Epizootics (epidemics) of black gill in the northern shrimp, P. borealis occur in the Gulf of Maine. Initial studies reported the causative agent as an unidentified ciliate (Rinaldo and Yevich Citation1974). More recent studies identified the ciliate as the apostome ciliate, Synophrya sp. (Lee et al. Citation2019). A similar ciliate, likely Synophrya sp., has been reported in P. borealis from Greenland and the Canadian Maritime provinces () (Orr et al. Citation2011; Rinaldo and Yevich Citation1974). A high incidence of black gill caused by Synophrya sp. was also reported in the dipandalid shrimp, D. leptoceras, collected from the middle Atlantic shelf of USA (Ruddell Citation1977). More work needs to be carried out to determine the prevalence of black gill in other ocean areas where pandalid shrimp occur. Besides its presence in pandalid shrimp, Synophrya sp. has also been detected in portunid crabs off the coast of France, Spain, Tunisia, in the SAB, and in the Gulf of Mexico (Chatton and Lwoff Citation1935; Sprague and Couch Citation1971, Johnson and Bradbury Citation1976; Haefner and Spacher Citation1985; Landers Citation2010; Taylor and Landers Citation2019). A survey of decapods in the SAB reported that Synophrya sp. infected 24 of the 54 species collected (Johnson and Bradbury Citation1976). The highest infection rate (95%) was found in the coarsehand lady crab, Ovalipes stephensoni. While Synophrya sp. is common in the swimming crabs of the SAB (), a microscopic examination of several hundred white shrimp (L. setiferus) in the same area detected only one specimen presumptively infected by Synophrya sp. ().
Life history of ciliates linked to black gill
Apostome ciliates, responsible for black gill in wild crustaceans, have a complex polymorphic life cycle that is closely tied to the molting cycle of their crustacean hosts (Bradbury Citation1966; Chatton and Lwoff Citation1935). The apostome life cycle includes four functionally different stages including 1) phoront (attached, pre-feeding stage), 2) trophont (feeding stage), 3) tomont (division stage), and 4) tomite (infective stage).
In Synophrya sp. the life cycle includes two distinct phases, an invasive histotrophic phase and an exuviotrophic phase (). There are two feeding stages, the invasive hypertrophont that feeds on host tissues before molting and the benign exuviotrophic trophont that feeds on exuvial fluid after the host molts. The cycle begins with the swimming tomite settling on a crab or shrimp exoskeleton to form the attached phoront stage. The phoront invades the gill lamellae and metamorphoses into the large hypertrophont. At premolt the hypertrophont divides to produce numerous swimming hypertomites which feed on exuvial fluid (referred to as exuviotrophic trophonts) and then encyst as the tomont stage. The tomont divides by palintomy (repeated divisions without intervening growth) and the smaller daughter cells, tomites, are released to begin the cycle again. Studies of the life cycle of Synophrya sp. have primarily been described in portunid crabs (Chatton and Lwoff Citation1935; Landers Citation2010). Studies of Synophrya sp. in the northern shrimp, P. borealis, suggest a similar life cycle with the identification of large feeding hypertrophonts, small hypertomites, settled phoronts and hypertomonts in these hosts ( and ). Comparative taxonomy based on ribosomal RNA sequences have suggested that Synophrya in portunid crabs and pandalid shrimp are two different species (Lee et al. Citation2019).
Figure 6. Different stages in the life cycle of Synophrya sp. which includes the invasive histotrophic phase on the gill and an exuviotrophic phase in the exuvial fluid after the molt. Based on studies by Chatton and Lwoff (Citation1935) and Landers (Citation2010). Photo inserts used with permission from Lee et al. Citation2019.
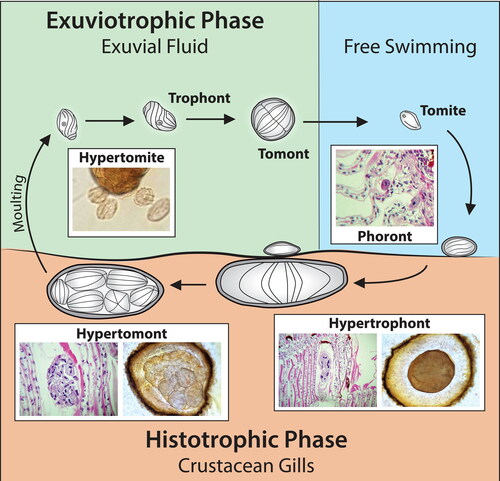
Figure 7. Synophrya whole mount life cycle stages from Achelous spinimanus (A-D) and Pandalus borealis (E-H) from the Atlantic coast. A. A hypertrophont with a healthy macronuclear reticulum (dark cords at arrow). Inset: cyst filled with hypertomites, revealing solid macronuclei (arrowhead). B. Degenerating macronucleus in a hypertrophont isolated by a melanin reaction. C. Final stages of macronuclear degeneration. D. Whole mount gill lamella with a wildly shaped hypertrophont, surrounded by the host’s reaction. E. Undivided hypertrophont stage revealing dark thin lines of the sparce ciliary rows (arrowheads). F. Divisional stages showing ciliary rows (arrowheads). G. Divisional stages with large macronuclear cords (arrow). H. Final stages of division with many divisional products, from a dissected specimen. The smallest cells are the hypertomites (arrow). A-C. Hematoxylin. D. Unstained. E-H. Silver nitrate. Scale bars 100 µm (A-C, E-H), 500 µm (D). Figure H used with permission from Lee et al. Citation2019. The remaining figures are new.
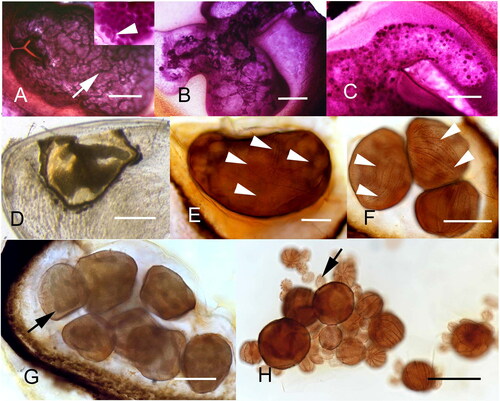
Figure 8. Thick and thin section microscopy of Synophrya. A. Synophrya hypertrophonts within the gill lamellae of Pandalus borealis (arrowheads). Plastic section. B. Synophrya hypertrophonts within the gill lamellae of P. borealis. Arrowhead indicates the peripheral chromatin of the macronuclear reticulum. Paraffin section. C. Synophrya cyst from P. borealis gills filled with multiple daughter cells, the hypertomite stage, which forms in premolt hosts (arrowhead). The dark structures within each cell are the macronuclei. Paraffin section. D-E. TEM of Synophrya from Achelous spinicarpus from the Gulf of Mexico. The hypertrophont cyst wall (arrowhead) is indicated. This very large stage has sparsely distributed ciliary rows. One is indicated by the arrows in D. The arrow in E indicates chromatin from the macronuclear reticulum. Inset: a rare section through the micronucleus. A. Toluidine blue. B-C. H & E. D-E uranyl acetate/lead citrate. Scale bars 200 µm (A, C), 500 µm (B), 3 µm (D, E), 5 µm (inset). Figures A, D and E used with permission from Lee et al. Citation2019 and Landers Citation2010. The remaining figures are new.
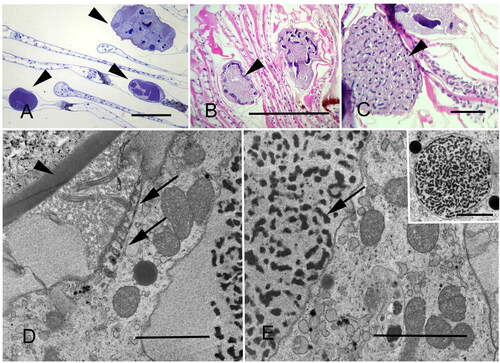
At least one species in the genus Hyalophysa is now known to cause black gill. Based on ciliature and 18S rRNA gene sequences, H. lynni is closely related to the nonpathogenic marine species H. chattoni and the freshwater species H. bradburyae and H. lwoffi (Landers et al. Citation2020). In addition to being pathogenic, H. lynni has a unique morphology and life cycle. Based on a study of life cycle forms Landers et al. (Citation2020) proposed a life cycle of H. lynni () that includes histotrophic feeding. Feeding on living tissues is believed to be the cause of the pathology associated with H. lynni infections where swimming tomites settle on the shrimp gill lamellae and encyst as phoront stages. Another unusual feature, not found in other Hyalophysa species, is the production of tomites while encysted on the host. An attached divisional stage is known only for one other apostome ciliate, Phoretophrya nebaliae, an exuviotroph found on the crustacean Nebalia geoffroyi, which attaches to its host as an enlarged tomite stage, and then divides (Chatton and Lwoff Citation1935). In addition to the attached encysted stages of H. lynni, invasive stages have been observed, but the link between these invasive stages and the external life cycle stages is unresolved. Although there is supportive indirect evidence that H. lynni feeds histotrophically on shrimp gill tissue, this has not been conclusively proven. Circumstantial evidence supports the hypothesis that H. lynni trophonts are also able to feed exuviotrophically, as swimming trophont stages with a ciliature similar to the encysted trophonts were observed in molts of infected L. setiferus (Landers et al. Citation2020). The phoront stage, assuming it exists, has not been confirmed although smaller phoronts have been observed on infected shrimp and are assumed to be H. lynni phoronts.
Figure 9. Proposed life cycle of Hyalophysa lynni where swimming tomites settle on gill lamellae and encyst as phoronts. The encysted phoront enlarges to become an encysted trophont which later becomes a tomont, the divisional stage that forms tomites. Photo inserts used with permission from Landers et al. (Citation2020).
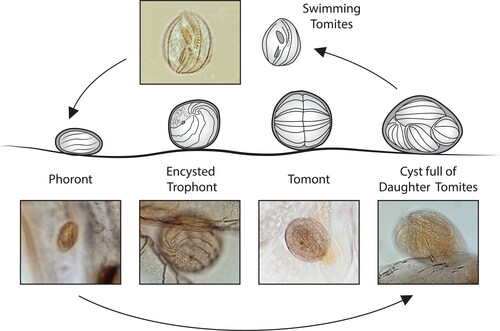
Transmission
Little is known about the transmission and infectivity of H. lynni, but based on the theorized life cycle (Landers et al. Citation2020), infection is understood to be due to the production of a free-living swimming tomite. Infectivity via direct contact and waterborne routes have both been reported (Frischer et al. Citation2017). Sentinel studies involving ciliate-free shrimp exposed to natural waters in aquaria with flow-through natural sea water or in cages placed in a SAB estuary resulted in significant transmission (O’Hare et al. Citation2019, Tomamichel et al. unpublished observations). These results are supportive of the hypothesis that H. lynni infections are transmitted via the water resulting in epidemic-scale outbreaks. An extensive search of the literature suggests that transmission of Synophrya sp. has not been directly studied. Further investigation is required in both H. lynni and Synophrya sp. to confirm waterborne transmission and to understand the factors that regulate the infection cycle.
Seasonality of Hyalophysa lynni and Synophrya sp. infections
The prevalence of symptomatic black gill infections in both penaeid and pandalid shrimp due to H. lynni and Synophrya sp., respectively, varies seasonally. This seasonality suggests an important role of environmental conditions as a driver of black gill infections. Since the first official reports of shrimp black gill in Georgia’s wild penaeid shrimp fishery in the SAB in 1996 () (Page et al. 2012), visible black gill has regularly appeared in the late summer when water temperatures reach their maximum and oxygen levels are at their lowest (Verity et al. Citation2006) (). Symptomatic shrimp typically persist through December but the timing and severity of the outbreak varies interannually (Kendrick et al. Citation2021). More recently, however, shrimp with visible black gill infections have been observed earlier in the season. For example, in 2016, 2019, and 2020, shrimp with visible black gill were observed as early as late May and early June in Georgia, South Carolina, and Texas (Kendrick et al. Citation2021; Swinford and Anderson Citation2021, Frischer unpublished observations). Before 2016 symptomatic black gill had not been observed in Georgia before August since it was first reported in 1996. Although visible findings of shrimp black gill generally are not apparent until the late summer, microscopic and PCR diagnostics indicate the presence of H. lynni beginning in April or May and persisting generally into December (). Even using the most sensitive PCR-based molecular diagnostic techniques, H. lynni has not been detected in SAB shrimp populations in the winter although occasionally an encysted ciliate resembling H. lynni has been observed. It is currently not understood where H. lynni goes in the winter and how it returns the following year.
Figure 10. Prevalence of black gill in coastal Georgia, USA. A. Average annual (August-December) of visible black gill prevalence since its first appearance in penaeid shrimp in coastal Georgia, USA. Collections were carried out monthly from 42 stations representative of the coastal, sounds, and creeks in Georgia by the Georgia Department of Natural Resources Environmental Trawl Survey program. Data from August-November period when visible black gill is most prevalent is shown. Data not available from 2017. Data used with permission from the Georgia DNR (Coastal Resources Division). B. Prevalence of Hyalophysa lynni detected by PCR and visual prevalence of black gill in coastal Georgia. Average monthly prevalence of black gill in penaeid shrimp based on visible observations of melanized gills (gray bars) and a PCR based diagnostic test (circles). Figure updated and used with permission from Frischer et al. (Citation2017).
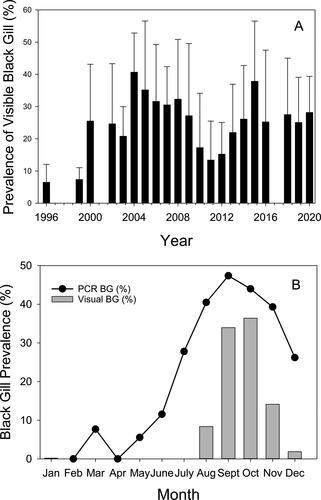
Synophrya sp. infections also vary seasonally in portunid crabs and pandalid shrimp. Bradbury (Citation1996) and Chatton and Lwoff (Citation1935) noted that Synophrya appears on the gills of portunid crabs in the summer months but was absent for the rest of the year. In contrast, symptomatic black gill in P. borealis in the Gulf of Maine peaks in the winter months (Rinaldo and Yevich Citation1974). In the fall months there was a low incidence of black gill in pandalid shrimp off the coasts of Maine, Labrador and Newfoundland (Orr et al. Citation2011; Rinaldo and Yevich Citation1974). Another pandalid shrimp, D. leptoceras, collected from the continental shelf of the middle Atlantic region of USA had a high incidence of black gill, caused by Synophrya, in the fall and winter (Ruddell Citation1977).
Pathology
The gross appearance of black gill in different crustacean species varies depending on the species and the pathogen (). The pathogen responsible for black gill is unknown in some cases, such as Black Necrotic Disease in crabs from Scotland which exhibit gills with brown or black bands (Comely and Ansell Citation1989) and the blackened gills in the amphipod, Traskorchestia traskiana, from western Canada beaches (Spicer Citation2013). Penaeus japonicus in culture, infected with the fungus, Fusarium sp., have been described as having black spots in the gill (Egusa and Ueda (Citation1972), while Brock and Lightner (Citation1990) described Penaeus californiensis with Fusarium disease as having white gills with a dark black color. Overstreet (Citation1973) noted black spots on the gills of hatchery grown shrimp infected with an unidentified fungus.
Table 1. Gross morphology of black gill in crustaceans from the wild caused by various agents.
Macroscopically, the appearance of the lesions produced by the apostome Synophrya sp. presents as black spots (). Microscopically, infections from both portunid crabs and pandalid shrimp reveal a circle of melanin deposition with a hypertrophont within (). Portunid crabs from the South Atlantic Bight with Synophrya sp. are described as having gills with black lesions with clear centers (Bradbury Citation1996; Johnson and Bradbury Citation1976) while Chatton and Lwoff (Citation1935) describe crabs (Achelous and Carcinus) from coastal France with black gill as having melanized scars on the gills and carapace. The visible findings of white or brown shrimp (L. setiferus and F. aztecus) infected with H. lynni are gill tissues with a diffuse light brown to black color (). Some shrimp exhibit asymmetric coloration between the right and left laterals. A correlation was noted between ciliate numbers and the color of the gills, ranging from light brown to dark black (Frischer et al. Citation2017, ).
Figure 11. Black gill in Pandalus borealis collected in the Gulf of Maine (USA). Used with permission from Lee et al. (Citation2019).
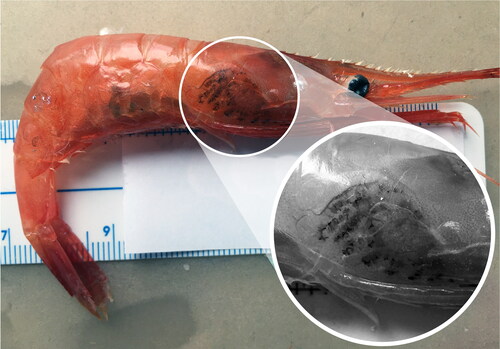
Figure 12. A. Black gill in white shrimp, Litopenaeus setiferus, B. Gills removed from white shrimp with severe black gill.
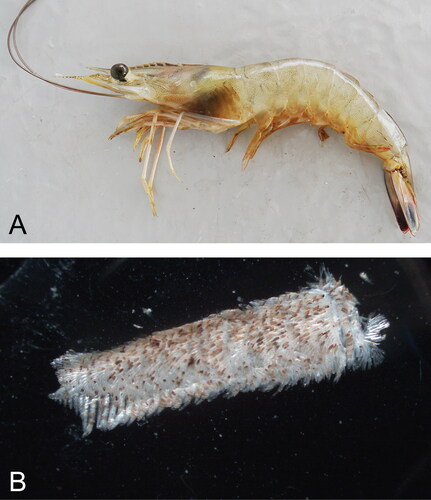
Figure 13. Correlation between the visible severity of black gill symptoms (gill color appearing clean, light brown, brown, black) in Litopenaeus setiferus and Hyalophysa lynni abundance. Data was estimated from 329 shrimp collected from August 2013 thru October 2014. Bar indicates one standard deviation. Dashed line is a linear regression (r2 = 0.99). Figure updated from Frischer et al. Citation2017.
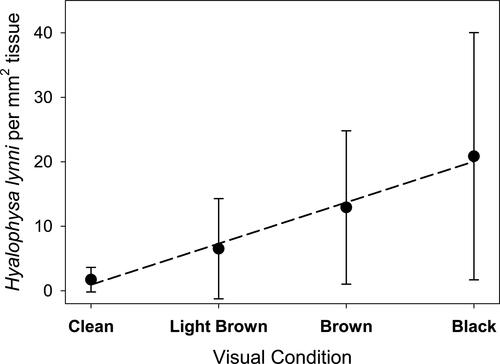
Fungal infections elicit a typical hemocyte modulated immune response. Fungal hyphae in gills of penaeid shrimp with Fusarium disease are typically encapsulated by layers of hemocytes and heavily melanized tissue (Brock and Lightner Citation1990; Lightner Citation1996; Bian and Egusa Citation1981). In laboratory studies, introduced foreign particles also elicit a hemocytic response resulting in tissue melanization. For example, after ink particles were injected into the lobster, H. americanus, nodules formed which later became melanized masses (Martin et al. Citation2000).
Prior to the development of black gill visible to the naked eye in penaeid shrimp infected with H. lynni, microscopic examination of gills often revealed low numbers of ciliates and melanized nodules without exhibiting severe pathology (). The prevalence of visibly symptomatic shrimp is therefore likely to be lower than the prevalence of infection. During peak infection periods in severely affected animals, however, marked melanization, cyst wall scars, encapsulation of the ciliate by melanized nodules, formation of isolating walls or membranes, damage to the exoskeleton, and necrosis of gill lamellae are typical features (). Both invasive and enlarged domed noninvasive divisional stages are associated with gill melanization. Interestingly, melanized nodules are observed in the absence of a ciliate in direct proximity ( and ), raising the possibility that the host response was effective in destroying the ciliate that elicited the formation of a particular nodule. Ciliates and remnants of melanized nodules are present in molted exoskeletons suggesting the role of molting in removing infections (). Scanning electron microscopy clearly reveals the extent of damage to gill lamellae as a result of black gill in L. setiferus (). Transmission electron microscopy reveals changes to the host’s exoskeleton underneath an encysted H. lynni parasite, and has shown that invading cells that have penetrated the host’s exoskeleton are enclosed in a cyst wall during the process (). These results suggest that the cyst wall is semipermeable, a characteristic known for the cyst wall of Synophrya (Chatton and Lwoff Citation1935). This semi-permeability is also known for the Hyalophysa cyst wall, in which encysted phoronts can respond to external chemicals in their environment, and can exhibit contractile vacuole activity, thus demonstrating water movement across the cyst wall (Bradbury Citation1966, Bradbury and Trager Citation1967, Landers Citation1986). This semipermeable nature may allow the attached and invading ciliate to release enzymes or other molecules used for exoskeleton penetration, and may allow the ciliate to absorb materials through the cyst wall.
Figure 14. Gill tissue from Litopenaeus setiferus collected before visible black gill occurred in the population. Arrow indicates a ciliate associated with small amounts of tissue necrosis and melanization. Arrowheads indicate small ciliates which have not induced a host cellular response. H & E. Scale bar 100 µm.
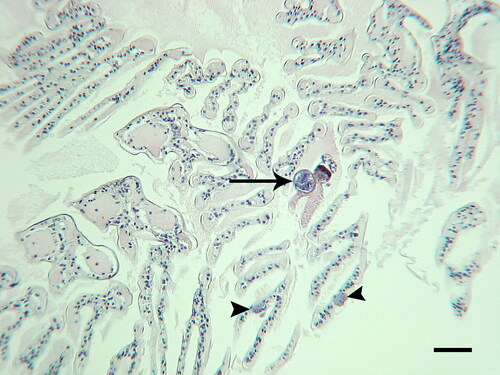
Figure 15. Light microscopy of Litopenaeus setiferus gills with Hyalophysa lynni infection. A. Low magnification of gills with no infection. B. H. lynni cyst with multiple tomites. Adjacent lamellae have undergone melanization and necrosis of the distal regions. C-D. Heavily infected gill regions with melanized areas (brown) and attached ciliates (arrows). E. Unsectioned area of gills with attached spherical ciliate cysts. F. Single gill lamella with melanization area (left) and a cyst scar from a previously attached ciliate (arrow). A-D. H & E. E-F. Silver nitrate. Scale bars 50 µm (A-D, F), 100 µm (E). Figure E used with permission from Landers et al. (Citation2020). Remaining figures are new.
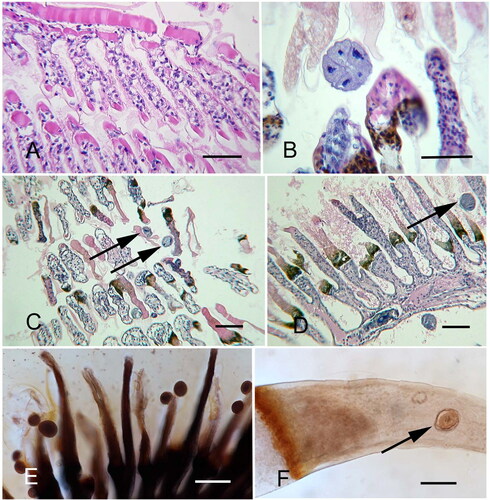
Figure 16. Light microscopy of Litopenaeus setiferus gills with Hyalophysa lynni trophonts. A. Noninvasive form, plastic section. The elongated macronucleus with round chromatin masses is visible. B-D. Paraffin sections of invasive forms, revealing the cell (within the cyst) extending into host tissue. E-F. Whole mount preparations with a trophont cyst nestled within a melanization scar (E) and an actively invading trophont stage (F). A. Toluidine blue. B-D. H & E. E. Silver nitrate stain. F. Hematoxylin. Scale bar 50 µm for each. Figure F used with permission from Landers et al. (Citation2020). Remaining figures are new.
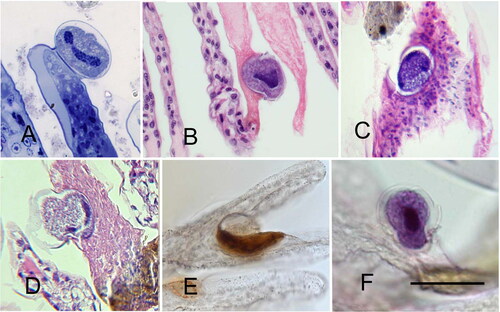
Figure 17. Scanning electron microscopy of Hyalophysa lynni attached to Litopenaeus setiferus gills. A. A cyst containing multiple tomites. The wall has collapsed upon the daughter cells. The secreted attachment peduncle is indicated (arrowhead). B. Two cysts. The cyst on the right has a collapsed wall, revealing the spiraling ciliary rows of the encysted trophont stage. Attachment peduncles, arrowheads. Scale bars 25 µm (A), 50 µm (B). Figures are new.
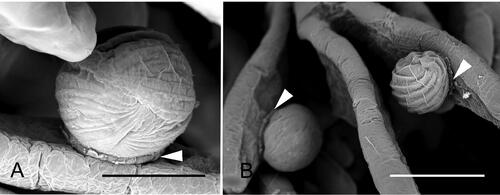
Figure 18. Exoskeleton of a recently molted white shrimp (Litopenaeus setiferus) with black gill, showing the presence of Hyalophysa lynni (arrowheads) and nodules (arrows). H & E. Scale bar: approx. 50 µm. Used with permission from Frischer et al. (Citation2018).
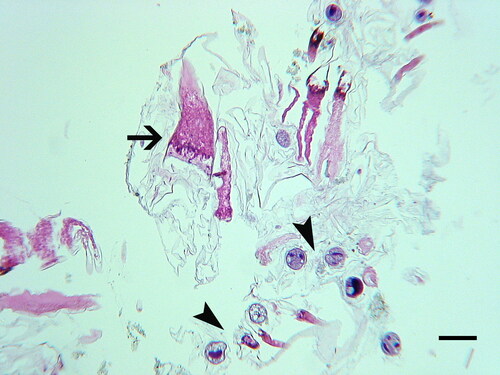
Figure 19. Electron microscopy of Hyalophysa lynni. A. Scanning EM of Litopenaeus setiferus gill tissue. B. Gill tissue exhibiting necrosis due to black gill disease. Necrotic lamellae are indicated with arrows, and an attached Hyalophysa lynni cyst is indicated with an arrowhead. C. TEM of H. lynni attached trophont stage (shrimp black gill ciliate, or sBG). The outer exoskeleton (Ex) of the host shrimp normally has a darkened appearance after fixation and staining (arrowheads). This outer layer is disrupted under the attached apostome cyst (white arrows). The black arrow indicates the inner layer of the ciliate cyst. D. TEM of a ciliate (sBG) during gill invasion. The location where the ciliate has invaded the exoskeleton (Ex) and entered the host tissue is to the right of “X”. The invasive cell is surrounded by a cyst wall (arrows), suggesting that feeding can take place through this layer. Scale bars 200 µm (A,B) and 2 µm (C,D). Used with permission from Landers et al. (Citation2020).
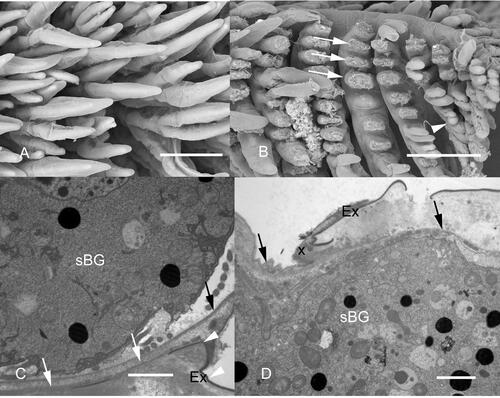
Synophrya sp. infections appear to elicit responses in pandalid shrimp that are similar to those observed in the penaeid shrimp infected with H. lynni, including extensive melanization, nodule formation and necrosis ( and ). One of the differences between black gill due to H. lynni and Synophrya sp. is the presence of large cytoplasmic masses, hypertrophonts, within the gill lamellae in pandalid shrimp infected by Synophrya sp. (). These hypertrophonts are separated from adjacent host tissues by an accumulation of necrotic debris and melanin with the melanin typically encircling the parasite (). The host response, including melanin deposition, is thought to isolate the parasite from its food source, the hemolymph of its crustacean host (Taylor and Landers Citation2019). Over time, the parasite will degenerate if the host does not molt before the ciliate’s energy reserves are depleted. Molting releases the parasite and leads to the hypertomite stage. The degeneration of the ciliate is observable in the macronucleus, which changes from a delicate reticulum to isolated islands of nuclear material (). Synophrya hypertrophonts have been observed invading the gill lamellae of the crab, A. spinimanus, A. gibbesii and A. ordwayi collected in the SAB (), P. borealis from the Gulf of Maine ( and ) and in A. spinicarpus from the Gulf of Mexico (). It should be noted that P. borealis occurs only in cold high latitude waters while portunid crabs with black gill are found in the warm waters of the SAB and Gulf of Mexico (). Adult Carcinus maenas from the northern coast of France seemed unaffected by Synophrya infections but young juvenile crabs were heavily infected and expected to die (Chatton and Lwoff Citation1935).
Morbidity and mortality
A primary question concerning black gill remains its contribution to morbidity, mortality, and the sustainability of shrimp fisheries and aquaculture operations. As described above, abiotic agents, fungi and ciliates responsible for black gill in pandalid and penaeid shrimp can produce necrosis, lesions, and melanized hemocytic nodules in gill tissues that interfere with critical gill functions including respiration and ion regulation that rely on unimpeded flow of hemolymph through the gill vasculature (White et al. Citation1985, Martin et al. Citation2000, Burnett & Burnett Citation2015). It has been speculated that impaired respiratory capacity can lead to increased morbidity and mortality either directly if the damage is great enough or indirectly if reduced respiratory capacity and endurance due to gill tissue damage associated with black gill leads to increased mortality due to predation (Frischer et al. Citation2018).
In high density culture systems mortality due to Fusarium sp. infections has been documented (Brock and Lightner Citation1990). Significant mortality due to black gill in culture systems, however, is rare. In wild populations it has been even more difficult to determine the impact of black gill on shrimp populations. For example, in the ongoing black gill epidemic in penaeid shrimp in the SAB, identifying a direct relationship between black gill and shrimp mortality has been challenging. Comparison between fishery performance (landings) and the prevalence of black gill are suggestive of cause and effect but are not conclusive. Fishery independent data has so far not revealed statistically significant relationships between shrimp abundance or health metrics and the prevalence of black gill (Kendrick et al. Citation2021). Shrimpers, however, report that shrimp with black gill are lethargic and have thin shells. Shrimpers have also described the sudden disappearance of shrimp in late summer at the beginning of the fall season when the prevalence of black gill typically peaks. George McKenzie, a recently retired Georgia shrimper, in an interview captured on film said “When that black gill gets in em, it kills em before we get to em. You go out there one day and be tearing the shrimp up, two days later they’ll be gone. They just die out, they get weak” (Sullivan Citation2015). Georgia shrimper captain Wynn Gale (F/V Big Cobb) described that in the late summer when black gill emerges, “it’s like someone turns the [shrimp] spigot off” (unpublished interview, Frischer). These observations suggest a causal relationship between black gill and fishery performance but, in the absence of directly observed mortality and controlled experimental studies, it has not been possible to disentangle and quantify the effect of black gill from the myriad of other natural and socio-economic factors that influence fishery performance. In the Gulf of Maine P. borealis fishery that experiences high levels of black gill due to Synophrya sp., the fishery has been closed since 2014 through at least 2021 due to low population assessments (Berger Citation2018). The role of black gill, however, has not been determined.
Respiratory impairment due to black gill has also been hypothesized to lead to reduced endurance and increased losses due to predation (Frischer et al. Citation2018). Compared to shrimp without visible symptoms of black gill, symptomatic shrimp exhibited reduced physical endurance and escape responses. In a follow-up mesocosom study, Gooding et al. (Citation2020) reported that shrimp with symptomatic black gill were significantly more susceptible to predation by three common predator species including red drum (Sciaenops ocellatus), spotted seatrout (Cynoscion nebulosus), and blue crabs (C. sapidus). In these studies, predator species were 1.4 to 3.0 times more likely to consume symptomatic shrimp than asymptomatic shrimp. Thus, both correlative field observations and laboratory studies support the hypothesis that black gill caused by the apostome ciliate H. lynni is negatively impacting the SAB penaeid shrimp fishery. More generally, fishery collapses due to disease have been reported in many invertebrate species and have been increasing in both frequency and intensity around the globe (Sweet and Bateman Citation2015). Hundreds of millions of dollars in losses to commercially valuable wild and cultivated species have been attributed to disease (Shinn et al. Citation2015).
A major focus of this review has been on a group of ciliated protozoans, apostome ciliates, where two genera (Hyalophysa and Synophrya) are agents for black gill epizootics in penaeid shrimp in the SAB region and pandalid shrimp in the Gulf of Maine but, reports of apostome caused disease in crustaceans are not limited to these examples. Apostome ciliates including species belonging to the genera Pseudocollinia and Collinia are commonly associated with euphausiids; crustaceans in the family Euphausiidae. In the North Pacific there are reports of mass mortality of euphausiids in the North Pacific due to infections of Collinia beringensis. (Gómez-Gutiérrez et al. Citation2003). High infection rates of C. beringensis have also been reported in euphausiids in the Bering Sea (Capriulo and Small Citation1986; Capriulo et al. Citation1991). Because apostome ciliates appear to be common crustacean pathogens it can be speculated that future investigations of reports of black gill and mass mortality of pelagic and benthic crustaceans in the world’s ocean will likely find high infection rates due to different species of apostomes.
Summary and recommended future studies
Black gill has been widely reported in both cultured and wild crustaceans. There are only a few reports of fungi causing black gill in wild crustaceans, but in crustaceans held in confined conditions, e.g. ponds, aquaria, black gill is often due to fungal infections. Two apostome ciliates, H. lynni and Synophrya sp., have been shown to be responsible for black gill epidemics in penaeid shrimp in the SAB and in pandalid shrimp in the Gulf of Maine, respectively. More studies are needed to determine the prevalence of black gill in pandalid and penaeid shrimp from the southern hemisphere. Work has been carried out on the transmission, life history, effects, seasonal changes and pathology of shrimp infected with H. lynni, though work remains to determine all of the details of its life history. Studies have been carried out on the pathology and life history of Synophrya but more work needs to be done on the transmission, sublethal effects, and seasonal changes of crustaceans infected with this apostome. A significant relationship was found between the mean fall prevalence of white shrimp black gill and both the Pacific Decadal Oscillation and the El Niño and the Southern Oscillation climatic indices in the SAB (Kendrick et al. Citation2021). Future studies may determine if there is a relationship between climate change, black gill incidence, and shrimp population numbers.
Acknowledgments
Lee Ann Deleo assisted with the preparation of the figures. We thank T. Taylor and M. Patel at Troy University for their laboratory work on shrimp black gill.
Additional information
Funding
References
- Alderman DJ, Polglase D. JL. 1985. Fusarium tabacinum (beyma) gams, as a gill parasite in the crayfish, Austropotamobius pallipes Lereboullet. J Fish Dis. 8(2):249–252. doi:10.1111/j.1365-2761.1985.tb01222.x
- Amparyup P, Charoensapsri W, Tassanakajon A. 2013. Prophenoloxidase system and its role in shrimp immune responses against major pathogens. Fish Shellfish Immunol. 34(4):990–1001. doi:10.1016/j.fsi.2012.08.019
- Athanassopoulou F, Speare D, Cawthorn RJ, Macmillan R, Despres B. 2004. Pathology of Anophryoides haemophila (Scuticociliatida: Orchitophryidae), parasite of American lobster Homarus americanus kept under experimental conditions. Aquaculture. 236(1–4):103–117. doi:10.1016/j.aquaculture.2004.02.023
- Bartolo I, Birk EO. 1998. Some factors affecting Norway lobster (Nephrops norvegicus) cuticle polyphenol oxidase activity and back-spot development. Int J Food Sci Technol. 33(3):329–336. doi:10.1046/j.1365-2621.1998.00168.x
- Bateman KS, Stentiford G. 2017. A taxonomic review of viruses infecting crustaceans with an emphasis on wild hosts. J Invertebr Pathol. 147:86–110. doi:10.1016/j.jip.2017.01.010
- Berger T. 2018. Moratorium on Northern Shrimp Commercial Fishery Maintained through 2021. State of Maine Department of Marine Resources. Atlantic State Marine Fisheries Commission 2018. Asmfc.org/upkoads/file/5bef2fd9pr40NorthernShrimpMoratoium.pdf
- Bian BZ, Egusa S. 1981. Histopathology of black gill disease caused by Fusarium solani (Martius) infection in the Kuruma prawn, Penaeus japonicas Bate. J Fish Dis. 4(3):195–201. doi:10.1111/j.1365-2761.1981.tb01126.x
- Bradbury PC, Trager W. 1967. Excystation of apostome ciliates in relation to molting of their crustacean hosts. II. Effect of Glycogen. Biol Bull. 133(2):310–316. doi:10.2307/1539827
- Bradbury PC. 1966. The life cycle and morphology of the apostomatous ciliate, Hyalophysa chattoni n.g., n. sp. J Protozool. 13(2):209–225. doi:10.1111/j.1550-7408.1966.tb01896.x
- Bradbury PC. 1994. Parasitic protozoa of molluscs and crustacean. In: Krier JP, editor. Parasitic protozoa. San Diego (CA): Academic Press; p. 139–264.
- Bradbury PC. 1996. Pathogenic ciliates. In: Hausmann K, Bradbury PC, editors. Ciliates: cells as organisms. Stuttgart, Germany: Gustav Fisher Verlag; p. 463–477
- Bradbury PC, Clamp JC. 1973. Hyalophysa lwoffi sp.n. from fresh water shrimp Palaemonetes paludosus and revision of the genus Hyalophysa. J Protozool. 20(2):210–213. doi:10.1111/j.1550-7408.1973.tb00865.x
- Brock JA, Lightner DV. 1990. Diseases of crustaceans In: Kinne O, editor. Diseases of marine animals. Hamburg, Germany: Biologische Anstalt Helgoland; p. 245–423.
- Burnett KG, Burnett LE. 2015. Respiratory and metabolic impacts of crustacean immunity: are there implications for the insects? Integr Comp Biol. 55(5):856–868. doi:10.1093/icb/icv094
- Burns CD, Berrigan ME, Henderson GE. 1979. Fusarium sp. infections in the freshwater prawn Macrobrachium rosenbergii (De Man). Aquaculture. 16(3):193–198. doi:10.1016/0044-8486(79)90107-8
- Capriulo GM, Pedone MJ, Small EB. 1991. High apostome ciliate endoparasite infection rates found in the Bering Sea euphausiid Thysanoessa inermis. Mar Ecol Prog Ser. 72:203–204. doi:10.3354/meps072203
- Capriulo GM, Small EB. 1986. Discovery of an apostome ciliate (Collinia beringensis n. sp.) endoparasitic in the Bering Sea euphausiid Thysanoessa inermis. Dis Aquat Org. 1:141–148.
- Cerenius L, Jiravanichpaisal P, Liu H-P, Söderhäll I. 2010. Crustacean immunity In: Söderhäll, K, editor. Invertebrate immunity. Boston (MA): Springer; p. 239–259.
- Cerenius L, Söderhall K. 2018. Arthopoda: pattern recognition proteins in crustacean immunity. In: Cooper EL, editor. Advances in comparative immunology. Heidelberg, Germany: Springer; p. 213–224.
- Chatton É, Lwoff A. 1935. Les ciliés apostomes; morphologie, cytologie, éthologie, évolution, systématique. Première partie. Aperçu historique et général, Étude monographique des genres de des espèces. Arch Zool Exp Gén. 77:1–453.
- Chun SK. 1980. Fungus Leucothrix sp. growing on the gills of cultured shrimp Penaeus japonicas. Bull Korean Fish Soc. 13:121–124.
- Comely CA, Ansell AD. 1989. The occurrence of black necrotic disease in crab species from the west of Scotland. Ophelia. 30(2):95–112. doi:10.1080/00785326.1989.10430838
- Couch JA. 1966. Two peritrichous ciliates from the gills of the blue crab. Chesapeake Sci. 7(3):171–173. doi:10.2307/1351165
- Couch JA. 1977. Ultrastructural study of lesions in gills of a marine shrimp exposed to cadmium. J Invert Pathol. 29(3):267–288. doi:10.1016/S0022-2011(77)80032-3
- Couch JA. 1978. Diseases, parasites, and toxic responses of commercial penaeid shrimp of the Gulf of Mexico and south Atlantic coasts of North America. Fish Bull. 76:1–44.
- Cruz da Silva LR, Camilo de Souza O, Fernandes MJS, Lima DMM, Coelho RRR, Souza-Motta CM. 2011. Culturable fungal diversity of shrimp Litopenaeus vannamei Boone from breeding farms in Brazil. Braz J Microbiol. 42(1):49–56. doi:10.1590/S1517-83822011000100007
- Denton GRW, Campbell RSF. 1990. The pathology of cadmium and nickel toxicity in the banana shrimp (Penaeus merguiensis). Asian Fish Sci. 3:287–297.
- Dewangan NK, Gopalakrishnan A, Kannan D, Shettu N, Singh RRJ. 2015. Black gill disease of Pacific white shrimp (Litopenaeus vannamei) by Aspergillus flavus. J Coast Life Med. 3(10):761–765. doi:10.12980/jclm.3.2015j5-70
- Egusa S, Ueda T. 1972. A Fusarium sp. associated with black gill disease of the Kuruma prawn, Penaeus japonicas Bate. Bull Jap Soc Fish. 38(11):1253–1260. doi:10.2331/suisan.38.1253
- Fisher WS, Nilson EH, Steenbergen JF, Lightner DV. 1978. Microbial diseases of cultured lobsters: a review. Aquaculture. 14(2):115–140. doi:10.1016/0044-8486(78)90025-X
- Fontaine CT, Lightner DV. 1975. Cellular response to injury in penaeid shrimp. Mar Fish Rev. 37:4–10.
- Fontaine CT. 1985. A survey of potential disease-causing organisms in bait shrimp from west Galveston Bay. In NOAA technical memorandum NMFS-SEFC-169. Galveston (TX): US Dept. of Commerce, Southeast Fisheries Center; p. 41.
- Foster CA, Sarphie TG, Hawkins WE. 1978. Fine structure of the peritrichous ectocommensal Zoothamnium sp. with emphasis on its mode of attachment to penaeid shrimp. J Fish Dis. 1(4):321–335. doi:10.1111/j.1365-2761.1978.tb00036.x
- Frias-Espericueta MG, Abad-Rosales S, Nevarez-Velazques AC, Osuna-Lopex I, Paez-Osuna F, Lozano-Olvera R, Voltolina D. 2008. Histological effects of a combination of heavy metals on Pacific white shrimp Litopenaeus vannamei juveniles. Aquat Toxicol. 3:152–157.
- Frischer ME, Fowler AE, Brunson JF, Walker AN, Powell SA, Price AR, Bulski K, Frede RL, Lee RF. 2018. Pathology, effects, and transmission of black gill in commercial penaeid shrimp from the South Atlantic Bight. J Shellfish Res. 37(1):149–158. doi:10.2983/035.037.0113
- Frischer ME, Lee RF, Price AR, Walters TL, Bassette MA, Verdiyev R, Torris MC, Bulski K, Geer PJ, Powell SA, et al. 2017. Causes, diagnostics, and distribution of an ongoing penaeid shrimp black gill epidemic in the U.S. South Atlantic Bight. J. Shellfish Res. 36(2):487–500. doi:10.2983/035.036.0220
- Gómez-Gutiérrez J, Peterson WT, De Robertis A, Brodeur RD. 2003. Mass mortality of krill caused by parasitoid ciliates. Science. 301(5631):339. doi:10.1126/science.1085164
- Goncalves AA, Menezes de Oliveira AR. 2016. Melanosis in crustaceans: a review. Food Sci Technol. 65:791–799. doi:10.1016/j.lwt.2015.09.011
- Gooding EL, Kendrick MR, Brunson JF, Kingsley-Smith PR, Fowler AE, Frischer ME, Byers JE . 2020. Black gill increases the susceptibility of white shrimp, Penaeus setiferus (Linnaeus, 1767), to common estuarine predators. J Exp Mar Biol Ecol. 524:151284. doi:10.1016/j.jembe.2019.151284
- Grimes BB. 1976. Notes on the distribution of Hyalophysa and Gymnodinioides on crustacean hosts in coastal North Carolina and a description of Hyalophysa trageri sp.n. J Protozool. 23(2):246–251. doi:10.1111/j.1550-7408.1976.tb03764.x
- Haefner PA, Spacher PJ. 1985. Gill meristics and branchial infestation of Ovallipes stephensoni (Crustacea, Brachyura) by Synophrya hypertrophica (Ciliata, Apostomida). J Crustacean Biol 5(2):273–280. doi:10.2307/1547874
- Hatai K. 2012. Diseases of fish and shellfish caused by marine fungi. In: Raghukumar C, editor Biology of Marine Fungi. Berlin, Germany: Springer-Verlag; p. 15–52.
- Hauton C. 2012. The scope of the crustacean immune system for disease control. J Invertebr Pathol. 110(2):251–260. doi:10.1016/j.jip.2012.03.005
- Hibbits J, Sparks AK. 1983. Observations on the histopathology caused by a parasitic ciliate (Paranophrys sp.?) in the isopod Gnorimosphaeroma oregonensis. J Invert Pathol. 41(1):51–56. doi:10.1016/0022-2011(83)90235-5
- Holmblad T, Söderhäll K. 1999. Cell adhesion molecules and antioxidative enzymes in a crustacean, possible role in immunity. Aquaculture. 172(1–2):111–123. doi:10.1016/S0044-8486(98)00446-3
- Jiravanichpaisal P, Lee BL, Söderhäll K. 2006. Cell-mediated immunity in arthropods: hematopoiesis, coagulation, melanization and opsonization. Immunobiol. 211(4):213–236. doi:10.1016/j.imbio.2005.10.015
- Johnson CA, Bradbury PC. 1976. Observations on the occurrence of the parasitic ciliate Synophrya in decapods in coastal waters off the southeastern United States. J Protozool. 23(2):252–256. doi:10.1111/j.1550-7408.1976.tb03765.x
- Johnson PT. 1985. Parasites of benthic amphipods: Microsporidans of Ampelisca agassizi (Judd) and some other gammarideans. Fish Bull. 83:497–505.
- Johnson SK, Parker JC, Holcomb HW. 2009. Control of Zoothamnium sp. on penaeid shrimp. Proc World Mariculture Soc. 4(1–4):321–325. doi:10.1111/j.1749-7345.1973.tb00116.x
- Karthikeyan V, Selvakumar P, Gopalakrishnan A. 2015. A novel report of fungal pathogen Aspergillus awamori causing black gill infection on Litopenaeus vannamei (pacific white shrimp). Aquaculture. 444:36–40. doi:10.1016/j.aquaculture.2015.03.021
- Kendrick MR, Brunson JF, Frischer ME, Kingsley-Smith PR. 2021. Climate indices predict black gill prevalence in white shrimp, Penaeus setiferus (Linnaeus, 1767), in South Carolina and Georgia, USA. J Shellfish Res. 40:145–151.
- Khoa LV, Hatai K, Aoki T. 2004. Fusarium incarnatum isolated from black tiger shrimp, Penaeus monodon Fabricius, with black gill disease cultured in Vietnam. J Fish Dis. 27(9):507–515. doi:10.1111/j.1365-2761.2004.00562.x
- Kulkarni A, Krishna S, Anand D, Uthaman SK, Otta SK, Karunasagar I, Valappil RK. 2021. Immune responses and immunoprotection in crustaceans with special reference to shrimp. Rev Aquacult. 13(1):431–459. doi:10.1111/raq.12482
- Kumar M, Rathinan RB, Tripathi G. 2020. Nidoviruses in aquatic organisms: paradigm of a nascent concern. Int J Aquat Fish Sci. 6:82–88.
- Lagniappe. 2000. Louisiana Sea Grant and LSU Agricultural Center Fisheries Newsletter. Black Gill Dis Shrimp. 24:11.
- Landers S, Lee RF, Walters TL, Walker AN, Powell SA, Patel M, Frischer ME. 2020. Hyalophysa lynni n. sp. (Ciliophora, Apostomatida), a new pathogenic ciliate and causative agent of shrimp black gill in penaeid shrimp. Eur J Protistol. 73:125673. doi:10.1016/j.ejop.2020.125673
- Landers SC. 1986. Studies of the phoront of Hyalophysa chattoni (Ciliophora, Apostomatida) encysted on grass shrimp. J Protozool. 33(4):546–552. doi:10.1111/j.1550-7408.1986.tb05660.x
- Landers SC. 1991. The fine structure of secretion in Hyalophysa chattoni: formation of the attachment peduncle and the chitinous phoretic cyst wall. J Protozool. 38(2):148–157. doi:10.1111/j.1550-7408.1991.tb06035.x
- Landers SC. 2010. The fine structure of the hypertrophont of the parasitic apostome Synophrya (Ciliophora, Apostomatida)). Eur J Protistol. 46(3):171–179. doi:10.1016/j.ejop.2010.03.001
- Lee RF, Walker AN, Landers SC, Walters TL, Powell SA, Frischer ME. 2019. Black spot gill syndrome in the northern shrimp, Pandalus borealis, caused by the parasitic ciliate Synophrya sp. J Invertebr Pathol. 161:40–46. doi:10.1016/j.jip.2019.01.003
- Lester RJG, Paynter JL. 1989. Diseases of cultured prawns in Australia. Actes de Colloques Ifremer. 97–101. Tahiti, French Polynesia, 20 Feb–4 March 1989, No. 9, Chap. 11, pp. 97–101. https://www.archimer.ifremer.fr/doc/00000/1458/.
- Lightner DV, Fontaine CT. 1975. A mycosis of the American lobster, Homarus americanus, caused by Fusarium sp. J Invert Pathol. 25:239–245. doi:10.1016/0022-2011(75)90074-9
- Lightner DV, Redman R. 1977. Histochemical demonstration of melanin in cellular inflammatory processes of penaeid shrimp. J Invert Pathol. 30(3):298–302. doi:10.1016/0022-2011(77)90137-9
- Lightner DV. Fontaine CT. Hanks K. 1975. Some Forms of Gill Disease in Penaeid Shrimp. Proceedings of the annual meeting - World Mariculture Society. World Aquacul Soc. 6(1-4):347–365. doi:10.1111/j.1749-7345.1975.tb00030.x.
- Lightner DV. 1985. A review of the diseases of cultured penaeid shrimps and prawns with emphasis on recent discoveries and developments, 79–103. Proceedings of the First International Conference on the Culture of Penaeid Prawns/Shrimps. Asian Fisheries Development Center, Iloilo, Philippines, Southeast Asian Fisheries Development Center.
- Lightner DV. 1996. A handbook of shrimp pathology and diagnostic procedures for disease of cultured penaeid shrimp. Baton Rouge (LA): World Mariculture Society.
- Ma H, Overstreet RM. 2006. Two new species of Epistylis (Ciliophora: Peritrichida) on the blue crab (Callinectes sapidus) in the Gulf of Mexico. J Eukaryot Microbiol. 53(2):85–95. doi:10.1111/j.1550-7408.2005.00080.x
- Magarelli PC, Hunter B, Lightner DV, Benard Colvin L. 1979. Black death—ascorbic acid deficiency disease in penaeid shrimp. Comp Biochem Physiol. 63(1):103–108. doi:10.1016/0300-9629(79)90634-0
- Martin GG, Quintero M, Quigley M, Khosrovian H. 2000. Elimination of sequestered material from the gills of decapod crustaceans. J Crust Biol. 20(2):209–217. doi:10.1163/20021975-99990032
- Messick GA, Small EB. 1996. Mesanophrys chesapeakensis n. sp., a histophagous ciliate in the blue crab, Callinectes sapidus, and associated histopathology. Invert Biol. 115(1):1–12. doi:10.2307/3226937
- Nha VV, Hoa DT, Khoa LV. 2009. Black gill disease of cage-cultured ornate rock lobster Panulirus ornatus in central Vietnam caused by Fusarium species. Aquaculture Asia. 14:35–37.
- Nimmo DWR, Lightner DV, Bahner LH. 1977. Effects of cadmium on the shrimp, Penaeus duorarum, Palaemonetes pugio and Palaemonetes vulgaris. In Vernberg FJ, Calabrese A, Thurberg FP, Vernberg WB, editors. Physiological responses of marine biota to pollutants. New York (NY): Academic Press; p. 131–183
- O’Hare MK, Womack NC, Raigoza S, Walters TL, Frischer ME. 2019. Development of novel sentinel assay to investigate the waterborne transmission of shrimp black gill. Puerto Rico: Abstract for the Annual Meeting of American Society of Limnology and Oceanography.
- Ogawa ME, Magalhaes-Neto E, Aguiar-Junior O, Kozima TT. 1984. Black discolouration of spiny lobster genus Panulirus White. III. Incidence of melanosis in the integumentary tissues. Nipp Suis Gakk. 50(3):471–475. doi:10.2331/suisan.50.471
- Ohtsuka S, T, SuzakiA, Kanazawa and M, Ando 2015. Biology of symbiotic apostome ciliates: their diversity and importance in the aquatic ecosystems. In Ohtsuka ST, Suzaki T, Horiguchi N, Suzuki F, editors. Marine protists. Tokyo, Japan: Springer; p. 441–463.
- Orr D, Veitch PJ, Sullivan DJ, Skanes K. 2011. The status of the northern shrimp (Pandalus borealis) resource off Labrador and northeastern Newfoundland as of March 2010. In Canadian Science Advisory Secretariat Research. Ottawa (ON): Fisheries and Oceans Canada; p. 175. Document 2011/004, pp.
- Overstreet RM. 1973. Parasites of some penaeid shrimps with emphasis on reared hosts. Aquaculture. 2:105–140. doi:10.1016/0044-8486(73)90140-3
- Overstreet RM. 1987. Solving parasite-related problems in cultured crustacea. Int J Parasitol. 17(2):309–318. doi:10.1016/0020-7519(87)90105-6
- Page J. Ecological Monitoring Surveys. Attachment 3 in Geer, P.J., and C. Belcher. Georgia Marine Research, Surveys, and Development. Annual Report to USFWS Sportfish Restoration Project GA F-79-R-7. July 2011– June 2012. GA DNR Coastal Resources Division, Brunswick, GA. 2012. 17 pp.
- Patel MK, Landers SC. 2019. Staining experiments with the ciliated protozoan known to cause shrimp black gill disease in Litopenaeus setiferus. Southeastern Biol. 66:176–177.
- Pramanik DS, Mohanty SK. 2015. Occurrence of black gill disease in relation to stocking density and some major prevailing hydrological parameters of Penaeus monodon in northern Odisha, India. Eur J Experi Biol. 5:38–43.
- Radcliffe NA. 1991. The prophenoloxidase system and its role in arthropod immunity. In: Warr GW, Cohen N, editors. Phylogenesis of immune functions. Boca Raton (FL): CRC Press; p. 45–71.
- Rao KR, Doughtie DG, Conklin PJ. 1982. Physiological and histopathological evaluation of diothiocarbamate toxicity to the grass shrimp, Palaemonetes pugio. In: Vernberg WB, Calabrese A, Thurberg FP, Vernberg FJ, editors. Physiological mechanisms of marine pollutant toxicity. New York (NY): Academic Press; p. 413–445
- Rao KR, Doughtie DG. 1984. Histopathological changes in grass shrimp exposed to chromium, pentachlorophenol and dithiocarbamates. Mar Environ Res. 14(1–4):371–395. doi:10.1016/0141-1136(84)90089-8
- Rhoobunjongde W, Hatai K, Wada S, Kubota SS. 1991. Fusarium moniliforme (Sheldon) isolated from gills of kuruma prawn Penaeus japonicas (Bate) with black gill disease. Bull Jap Soc Fish. 57(4):629–635. doi:10.2331/suisan.57.629
- Rinaldo RG, Yevich F. 1974. Black spot gill syndrome of the Northern shrimp, Pandalus borealis. J Invert Pathol. 24:204–233.
- Rio-Rodriguez RE, Pech D, Soto-Rodriguez SA, Gomez-Solano MI, Sosa-Lopez A. 2013. A ten-month diseases survey on wild Litopenaeus setiferus (Decapoda:Penaeidae) from southern Gulf of Mexico. Int J Trop Biol. 61:1175–1188.
- Ruddell CL. 1977. Histopathological studies on twelve benthic invertebrates from the middle Atlantic outer continental shelf. Special report in applied marine science and ocean engineering (SRAMSOE) No. 179, Pp10-1 to 10–47. Williamsburg (VA): Virginia Institute of Marine Science, College of William and Mary.
- Shinn AP, Pratoomyot J, Bron JE, Paladini G, Brooker EE, Brooker AJ. 2015. Economic costs of protistan and metazoan parasites to global mariculture. Parasitology. 142(1):196–270. doi:10.1017/S0031182014001437
- Sinderman CJ. 1989. The shell disease syndrome in marine crustaceans. NOAA Technical Memorandum NMFS-F/NEC-64. Woods Hole (MA): National Marine Fisheries Service; p. 35 repository.library.noaa.gov/view/noaa/5848.
- Small HJ, Miller TL, Coffey AH, Delaney KL, Schott E, Shields JD. 2013. Discovery of an opportunistic starfish pathogen, Orchitophrya stellarum, in captive blue crabs, Callinectes sapidus. J Invertebr Pathol. 114(2):178–185. doi:10.1016/j.jip.2013.07.008
- Smolowitz RM, Bullis RA, Abt DA. 1992. Mycotic branchitis in laboratory-maintained hermit crabs (Pagurus spp.). J. Crustacean Biol. 13:163–168.
- Sparks AK, Hibbits J, Fegley JC. 1982. Observations on the histopathology of a systemic ciliate (Paranophrys sp.?) disease in the Dungeness crab, Cancer magister. J Invert Pathol. 39(2):219–228. doi:10.1016/0022-2011(82)90014-3
- Spicer JL. 2013. Physiological changes accompanying the presence of black gill syndrome in the high shore amphipod Traskorchestia traskiana. J Exp Mar Biol Ecol. 446:131–138. doi:10.1016/j.jembe.2013.05.003
- Sprague V, Couch J. 1971. An annotated list of protozoan parasites, hyperparasites and commensals of decapod Crustacea. J Protozool. 18(3):526–537. doi:10.1111/j.1550-7408.1971.tb03367.x
- Sritunyalucksana KK, Söderhäll K. 2000. The proPO and clotting system in crustaceans. Aquaculture. 191(1–3):53–69. doi:10.1016/S0044-8486(00)00411-7
- Stentiford GD, Neil DM. 2011. Diseases of Nephrops and Metanephrops: a review. J Invertebr Pathol. 106(1):92–109. doi:10.1016/j.jip.2010.09.017
- Sullivan MP. 2015. Black gill in Georgia shrimp. Video available for viewing at: https://www.youtube.com/watch?v=xJQkORTHuVE. Accessed 13 March 2021.
- Sweet MJ, Bateman KS. 2015. Diseases in marine invertebrates associated with mariculture and commercial fisheries. J Sea Res. 104:16–32. doi:10.1016/j.seares.2015.06.016
- Swinford JL, Anderson JD. 2021. Prevalence of black gill (Hyalophysa lynni) in white shrimp (Litopenaeus setiferus) and brown shrimp (Farfantepanaeus aztecus) along the Texas Gulf coast. Mar Coast Fish. 13(3):263–274. doi:10.1002/mcf2.10153
- Taylor TA, Landers SC. 2019. Staining experiments with Synophrya, a parasitic ciliate found within the gills of portunid crabs. Southeastern Biol. 66:174.
- Uzmann JR, Haynes EB. 1968. A mycosis of the gills of the pandalid shrimp, Dichelopandalus leptocerus. J Invert Pathol. 12(3):275–277. doi:10.1016/0022-2011(68)90327-3
- Vaseeharan B, Ishwarya R, Malaikozhundan B, Selvaraj D, Chen J-C. 2016. Phenoloxidase an important constituent in crustacean immune system: a review. J Fish Soc Taiwan. 43:215–225.
- Verity PG, Alber M, Bricker SB. 2006. Development of Hypoxia in Well-mixed Subtropical Estuaries in the Southeastern USA. Estuaries Coasts. 29(4):665–673. doi:10.1007/BF02784291
- Vogt G. 2020. Cytopathology and immune response in the hepatopancreas of decapod crustaceans. Dis Aquat Organ. 138:41–48. doi:10.3354/dao03443
- White KN, Ratcliffe NA, Rossa M. 1985. The antibacterial activity of hemocyte clumps in the gills of the shore crab, Carcinus maenas. J Mar Biol Ass. 65(4):857–870. doi:10.1017/S0025315400019366
- Wu J-P, Chen H-C, Huang DJ. 2009. Histopathological alterations in gills of white shrimp, Litopenaeus vannamei (Boone) after acute exposure to cadmium and zinc. Bull Environ Contam Toxicol. 82(1):90–95. doi:10.1007/s00128-008-9582-7
- Zhang S, Bonami JR. 2007. A roni-like virus associated with mortalities of the freshwater crab, Eriocheir sinensis Milne Edwards, cultured in China, exhibiting “‘sighs disease’ and black gill syndrome”. J Fish Dis. 30(3):181–186. doi:10.1111/j.1365-2761.2007.00796.x