Abstract
Atlantic salmon (Salmo salar), brown trout (Salmo trutta), and steelhead trout (Oncorhynchus mykiss) kelts have important conservation value for population resilience. Nonetheless, relative to other salmonid life-stages, knowledge on their behavior and survival is poor. This is especially true for kelt downstream migration in rivers fragmented by hydropower plants (HPP), even though the physiological and survival consequences are severe when functional connectivity is not provided. Here, the existing information about kelt downstream migration past HPP was summarized, beginning with an overview of iteroparous salmonid lifecycles. Then, the importance of kelts for conservation and the threats they encounter while migrating was discussed. Finally, the current corpus of peer-reviewed literature and reports focused on kelt migration in these systems was presented. Kelt studies have focused on five main themes: (1) postspawning survival, (2) migration delays before dam passage, (3) passage selection and guidance efficiency, (4) passage mortality, and (5) the swimming behaviors of kelts during migration. Overall, there was a paucity of information about kelts and several information gaps and needs for future research. This work should inform scientists and river managers on the conservation of salmonids in regulated rivers, including the development of passage solutions for safe downstream migration.
1. Introduction
There is an urgent need to transition from a global fossil fuel-based economy to a global green economy to avert the worst climate change scenarios (IPCC Citation2022). Therefore, sources of renewable energy are likely to become ever more vital as nations transition and transform their economies (International Energy Agency Citation2021; Al-Shetwi Citation2022). Hydropower is one of the most important sources of renewable energy. Approximately 71% of global renewable energy is derived from hydropower projects and many new large and small-scale projects are under development (Zarfl et al. Citation2015; Couto and Olden Citation2018; Moran et al. Citation2018). For this reason, it is often considered a “green” source of energy (Geist Citation2021). Nevertheless, hydropower can dramatically alter lotic ecosystems, negatively impact river connectivity, and alter, or limit, volitional migratory movements of aquatic biota (Oliveira et al. Citation2018; Moran et al. Citation2018; Belletti et al. Citation2020; Wang et al. Citation2022). Moreover, fish passage through turbines often involves severe injuries or mortality (Pracheil et al. Citation2016; Silva et al. Citation2018; Bevelhimer et al. Citation2019; Mueller et al. Citation2020). Though attempts have been made to design innovative “fish-friendly” turbines, such technology still results in severe injuries and fatality for fishes (Mueller et al. Citation2022). Bypasses designed to divert downstream-migrating fish from turbines have often targeted juvenile salmonids, with little development undertaken to aid larger adult fish. For these reasons, opponents of hydropower development often consider the industry to be a provider of “red” energy, rather than “green” (Geist Citation2021).
For some recreationally and commercially important salmonid species from the genera Salmo and Oncorhynchus, the effects of hydropower facilities on downstream migrating juveniles (“smolts”) and upstream migrating adults have been studied across species and geographic regions (Ruckelshaus et al. Citation2002; Young et al. Citation2011; Thorstad et al. Citation2012; Moore et al. Citation2018; Heggenes et al. Citation2021). Yet, there is limited knowledge of the impacts of hydropower facilities on the downstream migration of post-spawned salmonid adults (“kelts”). This is surprising, as kelts are a fundamental life stage in many populations of iteroparous salmonid species (Keefer et al. Citation2008; Birnie-Gauvin, Thorstad, et al. Citation2019; Birnie-Gauvin et al. Citation2023). Kelts are important for population viability and stability, as they can return to their natal rivers as repeat spawners one or more times throughout their lifespan (Seamons and Quinn Citation2010; Moore et al. Citation2014; Birnie-Gauvin, Thorstad, et al. Citation2019). Though often only a small proportion of the overall population become kelts, these individuals carry huge reproductive potential. For example, steelhead repeat spawners have twice (for females) or three times (for males) the lifetime reproductive success of maiden spawners (Seamons and Quinn Citation2010). Thus, kelts and repeat spawners can be crucial for the resilience and growth of populations (Fleming Citation1996; Seamons and Quinn Citation2010). Developing a better understanding of kelt behaviors and the threats they face as they migrate in regulated rivers is essential, as these fish can suffer higher rates of injury and mortality when passing turbines than migrating juvenile salmonids (Vikström et al. Citation2020). Increased knowledge is needed to develop mitigative measures against such threats, including fish passage solutions that allow kelts to avoid turbines entirely.
To help highlight this crucial information gap about kelt behavior and survival in regulated rivers, a review of the available literature about salmonid kelt migration in the vicinity of hydropower plants (hereafter “HPP”) is provided, with special attention to Atlantic salmon (Salmo salar), brown trout/sea trout (Salmo trutta), and anadromous rainbow trout (Oncorhynchus mykiss) kelts (hereafter referred to as “salmon,” “brown trout,” or “steelhead”). First, some key aspects of salmonid life cycles and the population resilience value of kelts are outlined, as the lifecycles of these species are often impaired by HPP development (Smialek et al. Citation2021). Then a summary of the threats posed by HPP to kelts, a review of the available literature about kelt swimming behaviors through such facilities, a synopsis of existent knowledge gaps, and guidelines for the development of future research in this topic are provided.
2. Kelts and repeat-spawners
Atlantic salmon, steelhead, and brown trout are all considered iteroparous, meaning they can undertake multiple rounds of reproduction throughout their lives (Birnie-Gauvin et al. Citation2023). Rates of iteroparity vary by species and population. Among Atlantic salmon, up to 30% of maiden spawners can survive to become repeat spawners, though rates as high as 30% are rare (Klemetsen et al. Citation2003; Chaput and Benoît Citation2012; Bordeleau et al. Citation2018; Persson et al. Citation2022). For example, a recent study found that across 179 populations of Atlantic salmon, the mean proportion of repeat spawners was 3.6% (Persson et al. Citation2022). Notably, the mean size and sea age of salmon has decreased greatly during the last century. As most of the literature available on iteroparity for the species is from the late 20th and 21st centuries, historic rates of salmon iteroparity may have been greater than those reported here. Among anadromous brown trout, 30–60% of spawners live to become repeat spawners. Rates of iteroparity among steelhead vary by ecotype. Among steelhead that reenter freshwater during summer or autumn to finish maturing, iteroparity rates are typically less than 5%, while rates of iteroparity may be as high as 17% for steelhead that mature at sea and return to freshwater during winter (Keefer et al. Citation2008; Moore et al. Citation2014; Copeland et al. Citation2019). Rates of steelhead iteroparity also vary with migration distance, where rates are lowest for populations that spawn furthest inland (Keefer et al. Citation2008).
Many individuals of these species are anadromous; they are born in freshwater, but will spend one or more winters at sea, and then return to freshwater to spawn. Atlantic salmon and anadromous brown trout typically return to freshwater between May and October, with notable variability in migration timing among population (Klemetsen et al. Citation2003). They spawn in the autumn and then either migrate to sea immediately after spawning or overwinter in freshwater and migrate in the spring. The downstream-migrating post-spawners are typically referred to as “kelts.” In these species, male kelts often migrate shortly after spawning while female kelts wait until the spring. This is believed to be due to differences in energy reserves postspawning, as males use more energy to reproduce and therefore must return to sea to begin reconditioning earlier than females. By contrast, steelhead spawn in the spring. Some steelhead return to freshwater in the summer or autumn before reproduction and finish maturing there. Other steelhead return shortly before reproducing, having mostly matured at sea. The steelhead kelts then commence their downstream migrations in spring soon after spawning. Typically, there are more female steelhead kelts then male, again likely because males expend more energy while spawning than females (Evans et al. Citation2004; Keefer et al. Citation2008; Copeland et al. Citation2019).
In all three species, kelts are known to have poor condition following their spawning migration and subsequent reproduction. In some studies, “condition” is recorded as a ratio between body length and body weight (i.e. “condition factor”), while in other studies condition also considers physical characteristics including injuries, fungal infections, fin erosion, and activity level (Penney and Moffitt Citation2014). For most, this leads to substantial weight loss and depleted energy levels at the end of spawning, though the extent of energy depletion can vary by sex (Baum and Meister Citation1971; Jonsson, Hansen, et al. 1991). For this reason, species that spawn in the autumn (salmon and brown trout) have two different kelt migration periods. First, lower condition kelts (typically males) migrate in the autumn soon after spawning. Second, kelts with better condition overwinter in freshwater and commence their downstream migration in the spring (typically females). Steelhead kelts are also energy-depleted after spawning. This is particularly the case for summer steelhead, which reenter freshwater the summer before their spring-time spawning. Several studies conducted on summer steelhead have shown that lipid content in muscles and liver decline substantially between freshwater reentry and kelt emigration (Penney and Moffitt Citation2014, Citation2015). Energy depletion and the consequent loss of muscle mass can have serious consequences for fitness. Summer steelhead with the lowest condition are least likely to survive another marine migration and return as repeat spawners (Keefer et al. Citation2008). This may be partly because lower condition kelts are less likely to feed during migration than conspecifics in good condition (Penney and Moffitt Citation2014).
3. The value of kelts and repeat spawners for population resilience
Iteroparous reproductive strategies increase reproductive success when environmental conditions vary strongly among years and strengthen population stability via portfolio effects (Schindler et al. Citation2010; Moore et al. Citation2014). For example, populations with several cohorts of spawning adults (maiden and repeat spawners from various age classes) can protect against years of poor recruitment or reproduction among maiden cohorts (Bordeleau et al. Citation2019). Additionally, iteroparity can enhance population persistence in the face of anthropogenic stressors. For example, salmonid populations with kelts and repeat spawners are more likely to persist in fragmented rivers than populations without these life stages (Lawrence et al. Citation2016).
Repeat spawners make important genetic contributions to salmonid populations by playing a role in maintaining genetic diversity (Birnie-Gauvin, Thorstad et al. 2019; Birnie-Gauvin et al. Citation2023). Multiple cohorts of spawners may also increase genetic diversity within a population (Narum et al. Citation2008; Birnie-Gauvin et al. Citation2019). Furthermore, salmonid female body size is linked to fecundity, where larger females produce more eggs than smaller conspecifics (Thorpe et al. Citation1984; Fleming Citation1996; Heinimaa and Heinimaa Citation2004; Moffett et al. Citation2006; Quinn et al. Citation2011; Reid and Chaput Citation2012). Although repeat spawners make up only a fraction of the reproductive adults, they tend to have a larger body size than maiden fish (Chaput and Benoît Citation2012), and thus their contribution in terms of number of eggs are higher (Seamons and Quinn Citation2010; Bordeleau et al. Citation2019).
4. Kelts and hydropower: current knowledge
Many salmonid populations are in decline throughout their native ranges (Limburg and Waldman Citation2009; Kendall et al. Citation2017; Bull et al. Citation2022). Though the causes of these declines are multifaceted, hydropower development has been identified as a major problem for migratory salmonids. Historically, hydropower was considered to primarily affect upstream migrating adult salmonids, and solutions were devised to facilitate the upstream migration of adult spawners past barriers (Birnie-Gauvin, Franklin, et al. 2019). Next, such barriers were also found to negatively impact downstream migrating smolts, and researchers began to study smolt downstream migration in regulated rivers. Though there have been several studies about steelhead passage of hydropower dams on the Columbia River (see Appendix A), the focus on downstream passage of Atlantic salmon and brown trout kelts has been low and little information for these species exists (Calles and Greenberg Citation2009).
A literature review was undertaken to obtain an overview of the current knowledge on the downstream migration of kelts in regulated rivers and their interaction with HPP. As the number of studies on the topic was limited (n = 25), both technical reports and peer-reviewed papers were included. Though this was not a systematic review (due to the small number of peer-reviewed papers published on this topic), all that were relevant were collected. Web of Science and Google Scholar were searched for studies about salmon, brown trout, or steelhead kelts, combined with the terms “hydropower,” “turbines,” “downstream migration,” “migration delays,” “passage mortality,” “passage success,” and “passage solutions.” Of the 25 studies identified, 25 were published as peer-reviewed articless and five were published as technical reports. Eight studies focused on only salmon, four on only brown trout, and ten on only steelhead. An additional three studies were about both salmon and brown trout. All studies were conducted in one of four countries, with ten conducted in the USA, nine in Sweden, and three each in Canada and Norway. From these studies, five main themes were identified as the most relevant for downstream migration of kelts in regulated rivers ():
Figure 1. A summary of the five main themes and main focuses within each theme from the literature review, illustrated for a HPP (top view) with: a forebay (A), a nature-like fishway (B), a guidance structure (C), a dam with three turbines (D), and a technical fishway (E).
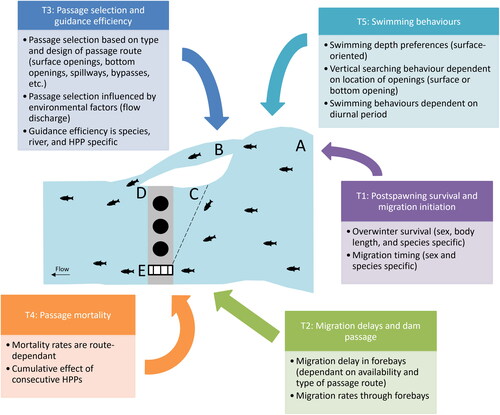
Postspawning survival and migration initiation.
Migration delays before dam passage.
Passage selection and fish guidance efficiency.
Passage mortality.
Swimming behaviors.
An overview of the 25 studies, including information about the research objectives, the main findings, and the characteristics of the study sites (such as guidance structures, passage routes, and turbine types) are provided in Appendix A.
4.1. Postspawning survival and migration initiation
Studies have shown that survival rates between spawning and the initiation of migration are likely site, species, year, and sex specific. Some salmon and brown trout may remain in freshwater for several months after spawning. For example, survival between autumn spawning and the initiation of springtime migration for salmon was 49% in River Klarälven, Sweden, and 56–75% in Saint John River, Canada (Nyqvist et al. Citation2016; Babin et al. Citation2021). In both studies, survival rates were highest for females and smaller fish (Nyqvist et al. Citation2016; Babin et al. Citation2021). Survival rates for brown trout that overwinter in freshwater after spawning can be as high as 88% or 100% (rivers Vindelälven and Piteälven; Östergren and Rivinoja Citation2008). It is possible that interannual variation in water temperature can drive differences in survival rates between autumn spawning and springtime migration in salmon (Nyqvist, Citation2017b). Steelhead kelts begin migration shortly after spawning. Often, female steelhead are more likely to survive spawning than males (Keefer et al. Citation2018; Fuchs et al. Citation2021). Furthermore, wild steelhead have been found to be more likely to survive spawning than hatchery-reared conspecifics (Fuchs et al. Citation2021). Fuchs et al. (Citation2021) found that approximately 56% of steelhead spawners survived reproduction and were able to initiate downstream migration as kelts in several tributaries of the Columbia River.
Several studies reported variation in migratory timing for the species that can overwinter in freshwater after spawning. For example, Kraabøl et al. (Citation2008) found that just over half of brown trout kelts initiated downstream migration 1-3 days after the end of spawning with very little interannual variation. Conversely, Nyqvist et al. (Citation2016) found that the dates for both autumn and spring migration varied markedly between years for salmon. Sex-based differences in migration timing have been documented for salmonid kelts (Arnekleiv et al. Citation2007; Kraabøl et al. Citation2008; Nyqvist et al. Citation2016). One study suggested that the closer to an HPP reservoir a salmon kelt overwintered, the earlier they initiated their downstream migration (Babin et al. Citation2021), though the mechanisms driving this pattern are unclear. The same study also recorded that before beginning their downstream migration, kelts often exhibited nondirected movements, involving an increase in swimming activity in both upstream and downstream directions (Babin et al. Citation2021).
Though the cues that cause salmonid smolts to migrate downstream are well known (e.g., Thorstad et al. Citation2012; Aldvén et al. Citation2015; Simmons et al. Citation2021; Vollset et al. Citation2021), the cues that cause kelts to initiate their downstream migration are less known. There is some evidence from an unregulated river in southern England that diel period, river discharge, and water temperature can cue brown trout kelt migration (Bendall et al. Citation2005). Among the studies conducted in hydropower-regulated rivers that were reviewed, there was little done to examine migration cues, and some of those results were inconclusive. For example, Babin et al. (Citation2021) reported that initiation was not related to water temperature in the Saint John River. Calles and Greenberg (Citation2009), however, noted that brown trout kelt migration in the River Emån, Sweden, occurred at roughly the same temperatures in both the autumn and spring (9.9 ± 0.8 °C vs 10.0 ± 0.4 °C), but at different discharges (23.8 ± 1.6 m3s−1 vs 16.4 ± 1.7 m3s−1). Nonetheless, migratory timing is important, as among steelhead kelts early migrants are more likely to become repeat spawners than later migrants (Evans et al. Citation2008). Whether water temperature and discharge are important for cueing migration, and whether the influence of these conditions on phenology might vary seasonally, is not yet fully known, but this information is crucial for predicting migration timing. The effects of temperature on migratory timing are also important to better understand as the climate continues to change, leading to increases in river temperatures, and because HPP can alter the natural thermal regimes of regulated rivers (Kuriqi et al. Citation2021). Such knowledge is important for defining operational schedules at HPP, including scheduling spill over spillways, which are often an important passage route for kelts (see Section 4.3).
4.2. Migration delays before dam passage
Fish passage times and migration delays associated with the presence of HPP emerged as one of the most frequent topics in the literature review. Migration delays are of particular interest, as kelts often have poor somatic condition and high levels of energy depletion from spawning (Jonsson, Jonsson, et al. Citation1991; Penney and Moffitt Citation2014; Baktoft et al. Citation2020). Delays caused by dams can further increase energy depletion, which could have negative consequences for subsequent fitness and survival (Baktoft et al. Citation2020).
Generally, most studies of salmonid kelts reported migration delays in reservoirs and forebays (Wertheimer and Evans Citation2005; Arnekleiv et al. Citation2007; Östergren and Rivinoja Citation2008; Calles et al. Citation2012; Colotelo et al. Citation2013, Citation2014; Nyqvist, Nilsson, et al. Citation2017; Babin et al. Citation2021). In some cases, delays in forebays can be long, such as 50 days for salmon in the forebay of one HPP (Nyqvist et al. Citation2016), or 29 days for brown trout in the forebay of another HPP (Östergren and Rivinoja Citation2008). The amount of time that kelts are delayed in a forebay depends on the types of fish passages available. For example, Nyqvist, Nilsson, et al. (Citation2017) studied passage delay at a dam before and after remedial measures were taken, where a simple bypass entrance/trash gate for downstream migrating fish was replaced by an angled rack with a bypass and a nature-like fishway. They found the median delay prior to the construction of the nature-like bypass was 220 min (interquartile range: 18 min − 10 days) while the median delay post-construction was 34 min (interquartile range: 20 min − 202 min) after the nature-like fishway was installed. Similarly, steelhead kelts in the Columbia River experienced longer migration delays at the Bonneville Dam prior to the installation of a surface bypass than after a new bypass was put in place (Wertheimer Citation2007). The extent of the migratory delay is likely also a function of river size compared to the size of the HPP and the passage routes available. For example, the size of the Columbia River, relative to the passage routes at its dams, may make it challenging for steelhead to locate passage. In such a large river, the discharge through the dam passage routes is likely to be relatively small, which can hinder kelts from finding safe passage.
Migration delays and passage selection are likely to be species-specific. For example, brown trout kelts can take longer than salmon kelts to pass the same dams in the same rivers (Calles et al. Citation2012). Furthermore, delays may be related to hydropower operation schedules. This was observed among brown trout kelts in southern Norway, that typically migrate in the autumn after spawning (Arnekleiv et al. Citation2007). Unfortunately, these kelts were then delayed upstream of a dam and could not pass until the spring when the spillways in the dam were opened (Arnekleiv et al. Citation2007). Since salmon and brown trout kelts with low condition factors are most likely to migrate in autumn (Halttunen et al. Citation2013), an overwinter delay means they are also delayed from beginning to recondition at sea, where they can access larger and more energetic prey items than in freshwater. Even if kelts can pass before spillways are opened, residence times in forebays are typically shorter during spill (Wertheimer and Evans Citation2005). Additionally, the delay experienced by individual kelts can be related to the passage route that they use, where delays vary depending on whether kelts pass through bypasses or via turbines (Scruton et al. Citation2007).
Some authors have compared migration rates in river reaches upstream and downstream of reservoirs to rates within reservoirs and have found that kelts generally migrate faster through the free-flowing reaches of rivers compared to reservoirs (Wertheimer and Evans Citation2005; Colotelo et al. Citation2013; Babin et al. Citation2021). Some authors have also noted that migration rates can be fast in tailraces, sometimes even faster than through non-impounded river reaches (Colotelo et al. Citation2013, Citation2014).
4.3. Passage selection and fish guidance efficiency
To facilitate bidirectional fish passage, HPP are often equipped with guidance structures, fishways, and bypasses (Silva et al. Citation2018). Nevertheless, spillways and turbines are commonly used by fish during downstream migration. Thus, passage selection was a common theme in studies analyzing kelt migration in regulated rivers. Kelt passage selection varied based on the available passage facilities, with most studies demonstrating that they prefer surface-oriented routes (surface spillways, bypasses, etc.) over submerged routes or passage via turbines (Hatch et al. Citation2003; Wertheimer and Evans Citation2005; Wertheimer Citation2007; Arnekleiv et al. Citation2007; Scruton et al. Citation2007; Colotelo et al. Citation2013, Citation2014; Nyqvist et al. Citation2016). Nevertheless, kelts will pass via bottom spill spillways and/or turbines when no other options are available. This was recorded in the River Klarälven, where thirteen tagged salmon kelts passed the dam via the turbines, while only two tagged salmon kelts passed via the spillways (Nyqvist, Bergman, et al. Citation2017). In this study, the open spillway was an upward-opening gate (“bottom spill”), which may have been unattractive to kelts that are often considered to be surface-oriented (see Section 4.5).
As with salmonid smolts, several studies indicated that flow discharge greatly impacted route selection among kelts (Hatch et al. Citation2003; Wertheimer and Evans Citation2005). For example, both Hatch et al. (Citation2003) and Wertheimer and Evans (Citation2005) found that steelhead kelts increase their passage via spillways rather than turbines as spill rates increased. Moreover, brown trout kelts in the River Emån were more likely to use the nature-like fishway when discharge to the turbines was low (Calles and Greenberg Citation2009). The magnitude of the effect of discharge on passage rates varies depending on the configuration of the spill gates at a given dam. For example, Arnekleiv et al. (Citation2007) demonstrated that increasing discharge through spillways had no effect on brown trout kelt passage rates when the spillways were at opposing ends of a dam, but when discharge was instead increased at neighboring spillways, brown trout kelt passage rate greatly increased. It is challenging to disentangle the effect of flow as an attraction cue for downstream navigation and the effect of strong flows that compel kelts toward a given route. Nonetheless, these findings suggest that kelts could potentially be guided by manipulating both flow discharge as well as release points through a dam.
Fish guidance efficiency is the ratio or percentage of fish that use an existing guidance structure in the river to find non-turbine passage routes (Scruton et al. Citation2008). There are many different types of guidance structures, which fall into two categories: physical structures, including bar racks and louvers, and behavioral structures, including bubble screens, electric barriers, acoustic barriers, water jet curtains, and lights (Noatch and Suski Citation2012; Meister et al. Citation2022). Physical guidance structures block fish from entering turbine intakes while behavioral guidance structures aim to repel or attract fish based on their known behaviors. The guidance efficiency of any given guidance structure varies between species. For example, Calles et al. (Citation2012) showed that brown trout kelts had a higher guidance efficiency than salmon kelts when using a bypass system at the Herting HPP in the River Ätran, Sweden. Guidance efficiency is also strongly dependent on the site, hydropower facility, and existing fish passage facilities (e.g., bypasses, fishways, spillways, etc.). For example, Scruton et al. (Citation2007) reported a 92% mean guidance efficiency for salmon to a surface-spill bypass in Newfoundland, Canada. The angled rack with a bypass and a nature-like fishway reported by Nyqvist, Nilsson, et al. (Citation2017) had an even higher guidance efficiency for salmon kelts, with 96% of the kelts successfully directed to the bypass. In steelhead kelts, Wertheimer (Citation2007) recorded an 80% guidance efficiency to surface bypasses at two dams in the Columbia River. A technical report synthesizing results across several studies of steelhead kelt in the Columbia River demonstrated that, like for salmon, guidance efficiency for steelhead kelts varies substantially by the dam and bypass type (Ferguson et al. Citation2005). Moreover, guidance efficiency can vary interannually. Scruton et al. (Citation2008) showed that guidance efficiency varied substantially between consecutive years for salmon in the Exploits River, Canada. There appears to be less research conducted on the use of behavioral barriers for salmonid kelts, though one recent study suggests that bubble screens may be a cost-effective and efficient guidance structure for salmon and brown trout kelts (Leander et al. Citation2024).
4.4. Passage mortality
Injury and mortality from HPP passage is a well-known problem for migratory fishes, with blade strikes, barotrauma, and shear forces among the main causes (Mueller et al. Citation2017, Citation2020). There appears to be little information about injury among salmonid kelts in HPP-regulated rivers; however, numerous studies report kelt mortality. Thus, mortality rates are one of the main topics addressed in this review.
In every study where kelts passed HPP via turbines, kelt mortality was recorded. In Sweden, two studies recorded a 62%-66% mortality rate for salmon kelts that passed through the Kaplan turbines at a HPP on the River Klarälven (Nyqvist et al. Citation2016; Nyqvist, Bergman, et al. Citation2017). Similar results were found for brown trout kelts migrating past the Stornorrfors power station in the River Umeälven, Sweden, (mortality = 69%), though mortality was lower at the Sikfors power station in the neighboring River Piteälven (mortality = 25%) (Östergren and Rivinoja Citation2008). High rates of mortality have also been recorded for steelhead in the Columbia River basin (Wertheimer and Evans Citation2005). The combined mortality rates for salmon and brown trout passing through turbines in Sweden (rivers Umeälven, Dalälven, and Piteälven) ranged from 25-56% (Ferguson et al. Citation2008; Vikström et al. Citation2020). Mortality rates in all these studies were estimated from several rivers with different types of turbines (either Francis or Kaplan turbines; Appendix A) with different hydraulic heads. A single study reported mortality from a non-turbine route; one kelt was observed to die while passing a spillway in the River Piteälven (Östergren and Rivinoja Citation2008). The authors of that study did not speculate on the exact cause of death, which may have been related to poor condition or another biological factor in conjunction with passing the spillway. Mortality during downstream passage is lower in good condition and wild kelts than their poor condition or hatchery-reared conspecifics (Hatch et al. Citation2003; Evans et al. Citation2008; Fuchs et al. Citation2021). Downstream mortality can be decreased when kelts are collected during their migration and transported (either by truck or barge) past HPP. This may be particularly useful in rivers with multiple high-head HPP (Evans et al. Citation2008; Trammell et al. Citation2016). When coupled with kelt reconditioning programs, 3.2% of steelhead that were transported past HPP returned as repeat spawners while only 0.9% of kelts that were transported but not reconditioned returned as repeat spawners (Trammell et al. Citation2016).
Moreover, mortality is cumulative in rivers with multiple HPP. This was clearly documented in the River Klarälven, Sweden, where salmon kelts pass eight dams on their migratory route from their spawning grounds. Nyqvist et al. (Citation2016) reported a mortality rate of 100% for downstream migratory kelts in this river. Evans et al. (Citation2008) tagged two groups of steelhead kelts, one group that subsequently migrated past seven HPP and a second that only migrated past two HPP. In both groups, some kelts were released to migrate downstream volitionally, while others were moved past the dams via barge or truck. They found that there was no statistically significant difference in survival between the kelts that migrated past two dams versus the kelts that were trucked past the two dams. In contrast, there was a statistically significant decrease in survival between the kelts that migrated past seven dams versus those moved downstream via barge without having to swim past the HPP. Thus, in that study mortality past multiple dams can also be considered cumulative, as the difference in survival between kelts that swam past HPP versus those that were moved downstream without swimming past the HPP was significant only when there were many HPP to pass. A later study in the Columbia River confirmed that passage mortality is cumulative in the presence of multiple HPP. In the study by Keefer et al. (Citation2018), steelhead kelts had to pass up to eight HPP. Overall survival to the lowermost HPP varied between 12.5 and 20.3%. Survival decreased notably at each HPP on the way downstream.
Entrainment and impingement are two other important aspects related to injury and mortality when studying the downstream passage of kelts through HPP (Calles et al. Citation2012; Rytwinski et al. Citation2017). Entrainment occurs when fish pass through hydropower structures, while impingement occurs when fish are trapped against hydropower structures (Rytwinski et al. Citation2017). Though Calles et al. (Citation2012) reported the entrainment of three salmon kelts and one brown trout kelt that died on racks at a hydropower plant in the River Ätran, Sweden, entrainment and impingement of salmonid kelts have not been reported in other kelt studies. While kelts, due to their higher swimming capacity than smolts, might be less susceptible to impingement, it is more likely that this phenomenon is underreported in the literature. Though kelts have a higher swimming capacity, their poor condition, including, for example, depleted energy reserves and Saprolegnia infections, could still render them susceptible to impingement (Penney and Moffitt Citation2014, Citation2015; Buelow and Moffitt Citation2015). Additionally, impingement may occur when solutions designed for a given species or life stage do not work for non-target fishes. It is possible that entrainment is underreported because larger-than-expected fish have recently been found to easily pass fish screens that should have been effective (Knott et al. Citation2023). Future research focusing on this theme is imperative and needed to guide the development of solutions for safe downstream passage of kelts.
Though several studies recorded kelt condition, few explicitly recorded kelt injury from HPP passage. The emphasis of these studies was on recording mortality, yet severe injuries could result in delayed mortality. Further information about injuries (types and sources of injury) would be beneficial. Moreover, most studies of kelt mortality rely on data obtained from telemetry. Because kelts typically are in poor condition, it is important to consider whether tag-induced mortality is higher for kelts than would be for other life stages. In studies where only the best condition kelts are tagged, it is possible that mortality is under-reported.
4.5. Swimming behaviors
Understanding kelt swimming behavior and swimming performance in the vicinity of HPP is fundamental for developing future passage solutions that protect and guide kelts past hydropower facilities. The swimming behavior of salmonid smolts has been well-studied (e.g., Enders et al. Citation2009, Citation2012; Silva et al. Citation2020). Yet, this area of research is still in its infancy for salmonid kelts with few studies focusing on their behavior at a high-resolution in regulated rivers (Appendix A).
Fish swimming depth is an adaptive response to feeding opportunities, predator avoidance, and habitat preferences. Fish may change swimming depth to take advantage of areas with prey, to moderate their body temperature, or to take advantage of daylight. At HPP, fish passage solutions should account for such preferences by ensuring passage routes for migrating fishes are at appropriate depths to reflect where fish most often swim. The swimming depth preferences of migrating kelts in regulated rivers have been analyzed in several studies. Kelts are generally considered to be surface-oriented (Wertheimer Citation2007; Weiland et al. Citation2009; Nyqvist et al. Citation2016; Nyqvist, Nilsson, et al. Citation2017; Babin et al. Citation2021) and primarily dive when searching for a passage past a dam (Colotelo et al. Citation2013). Swimming depth preference is species-specific, with salmon displaying a stronger preference for surface-oriented swimming than steelhead. For example, salmon kelts in the Saint John River, Canada, were surface-oriented 96% of the time and only dove to depths greater than 5 meters when swimming through reservoirs (Babin et al. Citation2021). In Sweden, salmon kelts primarily swam at depths between 1-3 meters below the river surface when approaching the turbine intakes (Nyqvist, Bergman, et al. Citation2017). In the Columbia River, USA, steelhead in one study mainly swam within the top 10 meters of the river but could dive down to 25 meters below the surface when searching for a route past the dam (Colotelo et al. Citation2013), while a second study found steelhead kelts were mainly surface-oriented (Wertheimer and Evans Citation2005), and a third study reported that the swimming depth distribution of steelhead kelts was only weakly skewed toward the surface and middle of the water column (Weiland et al. Citation2009). Thus, information regarding the swimming depth preference for steelhead kelts remains ambiguous. Unfortunately, through the literature review it was clear that there is little information on swimming depth preferences of brown trout kelts in regulated rivers.
Swimming behavior can also be influenced by diel period. For example, Colotelo et al. (Citation2014) found that 75% of steelhead kelts swam past the dam during the day, though Nyqvist, Bergman, et al. (Citation2017) and Colotelo et al. (Citation2013) found that diurnal period did not affect whether salmon and steelhead kelts would approach or pass a dam. Conversely, Scruton et al. (Citation2007) found that salmon kelts were most likely to exit the forebay during dusk and night. Thus, there appears to be variation in diel swimming depths across salmonid species and river systems. Furthermore, Nyqvist, Nilsson, et al. (Citation2017) found that diel swimming activity can be linked with passage options at dams, where before the installation of a nature-based fishway 38% of kelts approached and passed the dam during the day but following the installation of the new fishway 59% passed during the day, though when tested statistically the difference between the years was not significant. It should be noted, however, that behavioral data can also be impacted by production schedule in regulated rivers. Though diel period is an important determinant of behavior, passage timing in these studies may have also been influenced by whether the HPP were operating.
In hydropower-regulated rivers, it is important to consider how fish behavior is affected by hydraulic conditions, as HPP infrastructure alters the natural hydrodynamics of the flow. Fish are strongly affected by variations in flow hydrodynamics, exhibiting distinct swimming behaviors that vary by species and between life stages (Liao Citation2007). Two common behaviors are attraction and repulsion to different hydraulic conditions (Enders et al. Citation2012; Smith et al. Citation2014). Hydraulic conditions should be manipulated to attract fish to safe passage routes, thereby minimizing potential delays, and repulse them from risky passage routes like turbines. Few studies have evaluated the effects of hydraulic variables on kelt swimming behavior. In this review, only Babin et al. (Citation2021) attempted to study these relationships, and the results from the study were largely inconclusive. First, Babin et al. (Citation2021) tested the effect of flow velocity on migration rates of salmon kelts, finding that they swam slower in higher velocities, though the results were statistically insignificant. Next, they sought to evaluate the effect of flow direction on kelt rheotaxis (orientation relative to flow), though again the effect was statistically insignificant. It is possible these relationships were found to be statistically insignificant because the hydraulic data was collected at too large a resolution to combine with the kelt swimming speeds. Weiland et al. (Citation2009) observed that steelhead kelts tend to orient tail-first (positive rheotaxis) when passing the Bonneville Dam in the Columbia River, USA, and surmised this might be caused by the relatively high velocities in front of the surface flow outlet and turbine intakes; however, they did not formally test the relationship between flow velocity and kelt rheotaxis. A better understanding of the relationship between hydraulics and swimming behavior is crucial for guiding and protecting fish past hydropower facilities.
5. Discussion: Important knowledge gaps, challenges, and future research priorities
The five main themes identified in this review cover some of the aspects of kelt migration in regulated rivers. Nevertheless, there remains much that is unknown about this valuable life stage. Here, the most important knowledge gaps in the literature and discuss the direction and priorities for future studies are highlighted.
5.1. Knowledge gaps
Though salmonids are some of the most studied fish species in the scientific literature (Birnie-Gauvin, Thorstad, et al. 2019), several substantial knowledge gaps in the field of kelt migration in regulated rivers have been identified. These knowledge gaps include fundamental behavioral, mechanistic, and ecological questions that remain unanswered, yet are crucial for advancing passage solutions for salmonid kelts in regulated rivers.
There remain significant knowledge gaps for baseline data on kelt swimming behaviors in regulated rivers. From the literature review, swimming depth (for which the results were ambiguous) emerged as the main swimming behavior studied for kelts (Wertheimer Citation2007; Weiland et al. Citation2009; Colotelo et al. Citation2013; Nyqvist et al. Citation2016; Nyqvist, Nilsson, et al. Citation2017; Babin et al. Citation2021). Yet, limited knowledge exists on other crucial behavioral characteristics, like swimming speed and rheotaxis, required for designing guidance and passage structures suitable for kelts. The interplay between hydraulic conditions and behavior is vital for designing infrastructure that can repel (e.g., in front of intakes) or attract (e.g., bypasses) kelts. Additionally, there was no research conducted on kelt swimming biomechanics. Understanding the basic principles underlying kelt swimming biomechanics, which are directly related to hydraulic conditions in rivers, can also better enhance the understanding of kelt swimming behaviors (Liao Citation2007).
Few studies examined the cumulative impacts of passing consecutive HPP on kelts, yet many rivers have multiple HPP between the spawning grounds and marine environment. The few studies that have recorded passage success at multiple consecutive HPP have noted that mortality occurs at every dam (i.e. Nyqvist et al. Citation2016), but little work has been undertaken to understand the mechanisms underlying this phenomenon. For example, declining survival rates could be a relic of exhaustion (Penney and Moffitt Citation2014; Baktoft et al. Citation2020), as kelts experience passage delays and exert large amounts of energy to overcome dams, and/or could be cumulative effects of injuries induced from sequential dam passages (Geist Citation2021). There have been several studies in the Columbia River Basin that highlight the low energy reserves and often poor condition of migrating steelhead kelts, which lends credence to the idea that this is a contributing factor (Penney and Moffitt Citation2014, Citation2015; Buelow and Moffitt Citation2015; Keefer et al. Citation2018). Yet these mechanisms remain unexplored for salmon and brown trout kelts.
Furthermore, the long-term effects of passing HPP remains elusive. It is possible that kelts deemed to have survived HPP passage had injuries that they succumbed to after the study period. Alternatively, kelts that were substantially delayed at dams might have been subjected to increasingly warmer waters in the springtime, a potential source of physiological stress (Cunjak et al. Citation1998) or may have had less time to recondition at sea due to late arrival. The consequences of experiencing higher temperatures due to delays upstream of dams might also become more of a challenge under climate change, as springs become warmer over time (IPCC Citation2022). Such long-term effects of HPP passage on kelts represent a pressing gap in the scientific literature.
Injury and mortality caused by dam passage is another understudied subject among salmonid kelts. There can be different causes of injury and mortality among fish that pass turbines at HPP, such as turbine strikes, barotrauma, and shear stress (Pracheil et al. Citation2016; Mueller et al. Citation2017, Citation2020, Citation2022). Yet, studies have not attempted to determine the cause of mortality of kelts deemed to have been unsuccessful in passing a given HPP. Furthermore, only one study reported mortality from using a spillway route (Östergren and Rivinoja Citation2008). Whether this is because kelts are rarely injured from these routes, or whether this simply has not been studied, is unclear. The extent to which kelt characteristics, including energy reserves, migration timing, extent of fungal infections, and previous injury, affect susceptibility to injury and mortality during HPP passage is also unclear. Understanding the mechanisms underlying mortality rates for kelts could have important implications for designing turbines and passage structures for improved HPP passage success.
Environmental variables, such as water temperature and discharge, are understudied for kelt migration in hydropower regulated rivers. This may be particularly important, as HPP production can alter temperature regimes (Kuriqi et al. Citation2021). Few of the reviewed studies included water temperature or discharge as covariates in their analyses, and results were ambiguous (e.g., Calles and Greenberg Citation2009; Babin et al. Citation2021). The proximate, environmental factors that trigger springtime migration of kelts are not well established. Environmental factors could also explain the interannual variation in survival and passage success noted in several studies (Scruton et al. Citation2008; Nyqvist, Nilsson, et al. Citation2017). More focus on such environmental factors is important, as temperature and discharge regimes are forecasted to shift under climate change (IPCC Citation2022). This information could also help HPP operators to better predict the timing of kelt downstream migration now and in the future and ensure that adequate levels of spill are occurring during the migration period.
5.2. Challenges
There are some challenges associated with studying the downstream migration of salmonid kelts in hydropower-regulated rivers. The majority (96%) of the reviewed studies used telemetry methods but tagging fish with poor condition (like kelts) can lead to higher rates of tag-related mortality, which might bias passage survival estimates. To overcome this issue, some authors tagged the fish before they spawned, by capturing them during their upstream migration when their condition was better (e.g., Arnekleiv et al. Citation2007, Calles and Greenberg Citation2009, Nyqvist et al. Citation2016, Keefer et al. Citation2018, or Fuchs et al. Citation2021). Unfortunately, not all upstream migrating adults arrived at their spawning grounds (Ferguson et al. Citation2005), so tagging these fish can result in “wasted” tags. To avoid this and adverse effects of tagging poor condition kelts, researchers might only tag good condition downstream migrating kelts, ensuring just individuals that have spawned are tagged (Wertheimer and Evans Citation2005; Wertheimer Citation2007). It is then imperative to remember this might bias results by inflating estimates of passage success and guidance efficiency, as good condition kelts might be more likely to successfully pass HPP than lower condition conspecifics (Hatch et al. Citation2003; Wertheimer and Evans Citation2005; Evans et al. Citation2008). Thus, it is important to carefully consider when and which kelts are tagged for an experiment to balance tagging success with biases that might arise from targeting fish based on apparent condition.
Secondly, obtaining the hydraulic data necessary for studying kelt behavior in regulated rivers is also challenging. Acquiring in situ measurements requires expensive equipment and can be hampered by topographic hazards. Using computational fluid dynamics models to simulate hydraulic conditions can be a good alternative (Coutant and Whitney Citation2000). Even so, the simulated hydraulic data demands a good validation, which still should be completed via in situ flow measurements.
Finally, it is challenging to compare key metrics, such as passage time, guidance efficiency, and mortality rates, across studies. A lack of standardization of these types of metrics may negatively influence the development of future solutions for downstream passage of kelts. In 2021, the European Committee for Standardization published guidance for assessing important metrics related to fish passage solutions, with the goal of achieving standardized and comparable results (CEN Citation2021). Still, these have not been strongly applied. Information such as the presence and type of guidance structures, types, and number of turbines, etc., should be explicitly included in these studies to facilitate comparison of results. Yet, only 19 and 13 of the studies reviewed provided some description of guidance structures and listed the types of turbines used at the site, respectively (Appendix A).
5.3. Future research priorities
There are two main groups of future priorities of kelt research in regulated rivers: those that will feed into the improved design at HPP and those that will lead to improvements in management practices. Improved design and improved management practices should be considered two of the most important goals for researchers working with kelts, as these support the ultimate goal of protecting salmonid kelts in regulated rivers to maximize their potential to become repeat spawners. Improved designs and management practices are interconnected and are crucial for improving conservation ().
Figure 2. Overview of future research priorities for studies of kelts in regulated rivers. New studies should aim to support one or two important goals: improving design for guidance, protection, and passage at HPP and/or improving river and operational management at HPP (orange). To do so, there are still substantial knowledge gaps within fundamental fields (green). These can begin to be addressed with various tools, including tools used in laboratory and field settings (blue).
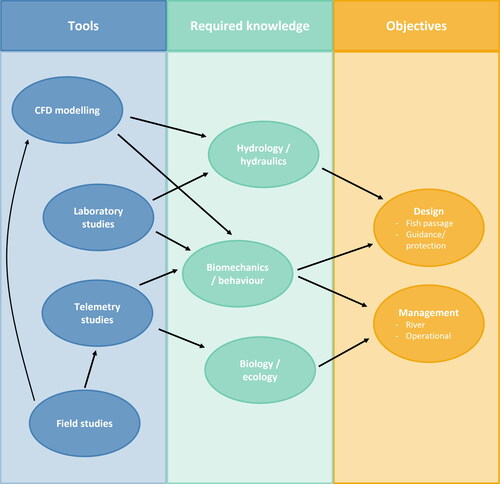
Based on the knowledge gaps identified above, there are four main research topics that should be prioritized by researchers working with kelts in hydropower-regulated rivers. First, better knowledge is needed about the swimming behaviors and biomechanics of kelts in both regulated and unregulated systems. Thus far, the focus has been on swimming depth preferences (Appendix A), with little focus on swimming speeds at HPP or rheotaxis. Future research in this field should also consider how hydraulic conditions affect biomechanics and behaviors, as these hydraulic conditions could then be manipulated to guide kelts. This would help to inform improved design at HPP. Second, the mechanisms driving injury and mortality for kelts migrating past HPP are understudied. These are likely to be site, river, and route dependent, and may include environmental factors like temperature. But better understanding whether injury/mortality arises from turbines strikes, impingement, etc., is crucial for protecting kelts as they migrate and could also lead to improved design at HPP. Third, a better understanding of the migration phenology could allow managers to better predict the arrival of kelts and schedule HPP operations, which could help to improve management practices. Finally, understanding the long-term effects of HPP passage on kelts is critically important, both for improved design and management at HPP.
6. Conclusion
As populations of iteroparous salmonids continue to face stark challenges throughout much of their native ranges, the conservation of kelts is crucial. Ensuring that kelts have safe and efficient downstream migratory routes can have significant ramifications on their ability to recondition and return as a repeat spawner. Repeat spawners, with their high fecundity, can help to bolster flagging populations. Though many threats can compromise the migratory success of kelts, both in rivers and the ocean, one of the major threats is river fragmentation due to the presence of hydropower plants. As demonstrated throughout this review, kelts are generally understudied, but are particularly understudied when considering the effect that hydropower might have on their migratory success. Current research is limited to studies of postspawning survival, migration delays, passage selection, guidance efficiency, passage mortality, and some swimming behaviors. Yet, there remain many behavioral, mechanistic, and ecological questions that are fundamental to advancing solutions to guide and protect kelts past HPP. These questions should be addressed as a matter of priority.
Disclosure statement
The authors report there are no competing interests to declare.
Additional information
Funding
Reference list
- Aldvén D, Degerman E, Höjesjö J. 2015. Environmental cues and downstream migration of anadromous brown trout (Salmo trutta) and Atlantic salmon (Salmo salar) smolts. Boreal Environ Res. 20:35–44.
- Al-Shetwi AQ. 2022. Sustainable development of renewable energy integrated power sector: trends, environmental impacts, and recent challenges. Sci Total Environ. 822:153645. doi: 10.1016/j.scitotenv.2022.153645.
- Arnekleiv JV, Kraabøl M, Museth J. 2007. Efforts to aid downstream migrating brown trout (Salmo trutta L.) kelts and smolts passing a hydroelectric dam and a spillway. Hydrobiologia. 582(1):5–15. doi: 10.1007/s10750-006-0547-8.
- Babin AB, Ndong M, Haralampides K, Peake S, Jones R, Curry RA, Linnansaari T. 2021. Overwintering and migration behaviour of post-spawned Atlantic salmon Salmo salar in a large hydropower-regulated river and reservoir. J Fish Biol. 99(3):856–874. doi: 10.1111/jfb.14768.
- Baktoft H, Gjelland KØ, Szabo-Meszaros M, Silva AT, Riha M, Økland F, Alfredsen K, Forseth T. 2020. Can energy depletion of wild Atlantic salmon kelts negotiating hydropower facilities lead to reduced survival? Sustainability. 12(18):7341. doi: 10.3390/su12187341.
- Baum ET, Meister AL. 1971. Fecundity of Atlantic salmon (Salmo salar) from two Maine rivers. J Fish Res Bd Can. 28(5):764–767. doi: 10.1139/f71-106.
- Belletti B, Garcia de Leaniz C, Jones J, Bizzi S, Börger L, Segura G, Castelletti A, van de Bund W, Aarestrup K, Barry J, et al. 2020. More than one million barriers fragment Europe”s rivers. Nature. 588(7838):436–441. doi: 10.1038/s41586-020-3005-2.
- Bendall B, Moore A, Quayle V. 2005. The post-spawning movements of migratory brown trout Salmo trutta L. J Fish Biol. 67(3):809–822. doi: 10.1111/j.0022-1112.2005.00786.x.
- Bevelhimer MS, Pracheil BM, Fortner AM, Saylor R, Deck KL. 2019. Mortality and injury assessment for three species of fish exposed to simulated turbine blade strike. Can J Fish Aquat Sci. 76(12):2350–2363. doi: 10.1139/cjfas-2018-0386.
- Birnie-Gauvin K, Bordeleau X, Eldøy SH, Bøe K, Kristensen ML, Nilsen CI, Lennox RJ. 2023. A review of iteroparity in anadromous salmonids: biology, threats and implications. Rev Fish Biol Fisheries. 33:1005–1025. doi: 10.1007/s11160-023-09773-8.
- Birnie-Gauvin K, Franklin P, Wilkes M, Aarestrup K. 2019. Moving beyond fitting fish into equations: progressing the fish passage debate in the Anthropocene. Aquat Conserv. 29(7):1095–1105. doi: 10.1002/aqc.2946.
- Birnie-Gauvin K, Thorstad EB, Aarestrup K. 2019. Overlooked aspects of the Salmo salar and Salmo trutta lifecycles. Rev Fish Biol Fish. 29(4):749–766. doi: 10.1007/s11160-019-09575-x.
- Bordeleau X, Hatcher BG, Denny S, Fast MD, Whoriskey FG, Patterson DA, Crossin GT. 2018. Consequences of captive breeding: fitness implications for wild-origin, hatchery-spawned Atlantic salmon kelts upon their return to the wild. Biol Conserv. 225:144–153. doi: 10.1016/j.biocon.2018.06.033.
- Bordeleau X, Pardo SA, Chaput G, April J, Dempson B, Robertson M, Levy A, Jones R, Hutchings JA, Whoriskey FG, et al. 2019. Spatio-temporal trends in the importance of iteroparity across Atlantic salmon populations of the Northwest Atlantic. ICES J Mar. Sci. 77(1):326–344. doi: 10.1093/icesjms/fsz188.
- Buelow J, Moffitt CM. 2015. Physiological indices of seawater readiness in postspawning steelhead kelts. Ecol Freshwater Fish. 24(1):112–122. doi: 10.1111/eff.12130.
- Bull CD, Gregory SD, Rivot E, Sheehan TF, Ensing D, Woodward G, Crozier W. 2022. The likely suspects framework: the need for a life cycle approach for managing Atlantic salmon (Salmo salar) stocks across multiple scales. ICES J Mar Sci. 79(5):1445–1456. doi: 10.1093/icesjms/fsac099.
- Calles O, Greenberg L. 2009. Connectivity is a two-way street—the need for a holistic approach to fish passage problems in regulated rivers. River Res Appl. 25(10):1268–1286. doi: 10.1002/rra.1228.
- Calles O, Karlsson S, Hebrand M, Comoglio C. 2012. Evaluating technical improvements for downstream migrating diadromous fish at a hydroelectric plant. Ecol Eng. 48:30–37. doi: 10.1016/j.ecoleng.2011.05.002.
- CEN. 2021. Water quality – Guidance for assessing the efficiency and related metrics of fish passage solutions using telemetry. Brussels (Belgium): The European Committee for Standardization CEN.
- Chaput G, Benoît HP. 2012. Evidence for bottom–up trophic effects on return rates to a second spawning for Atlantic salmon (Salmo salar) from the Miramichi River, Canada. ICES J Mar Sci. 69(9):1656–1667. doi: 10.1093/icesjms/fss055.
- Colotelo A, Harnish R, Jones B, Hanson A, Trott D, Greiner M, McMichael G, Ham K, Deng Z, Brown R, et al. 2014. Passage distribution and federal Columbia River power system survival for steelhead kelts tagged above and at Lower Granite Dam, Year 2. Washington: Pacific Northwest National Laboratory.
- Colotelo A, Jones B, Harnish R, McMichael G, Ham K, Deng Z, Squeochs G, Brown R, Weiland M, Ploskey G, et al. 2013. Passage distribution and federal Columbia River power system survival for steelhead kelts tagged above and at Lower Granite Dam. Washington: Battelle.
- Copeland T, Bowersox BJ, Ackerman MW, Camacho C. 2019. Patterns of iteroparity in wild Snake River steelhead. Trans Am Fish Soc. 148(5):926–937. doi: 10.1002/tafs.10187.
- Coutant CC, Whitney RR. 2000. Fish behavior in relation to passage through hydropower turbines: a review. Trans Am Fish Soc. 129(2):351–380. doi: 10.1577/1548-8659(2000)129<0351:FBIRTP>2.0.CO;2.
- Couto TB, Olden JD. 2018. Global proliferation of small hydropower plants – science and policy. Front Ecol Environ. 16(2):91–100. doi: 10.1002/fee.1746.
- Cunjak RA, Prowse TD, Parrish DL. 1998. Atlantic salmon (Salmo salar) in winter: “the season of parr discontent”? Can J Fish Aquat Sci. 55(S1):161–180. doi: 10.1139/d98-008.
- Ducharme LJA. 1969. Atlantic Salmon returning for their fifth and sixth consecutive spawning trips. J Fish Res Bd Can. 26(6):1661–1664. doi: 10.1139/f69-149.
- Enders EC, Gessel MH, Anderson JJ, Williams JG. 2012. Effects of decelerating and accelerating flows on juvenile salmonid behavior. Trans Am Fish Soc. 141(2):357–364. doi: 10.1080/00028487.2012.664604.
- Enders EC, Gessel MH, Williams JG. 2009. Development of successful fish passage structures for downstream migrants requires knowledge of their behavioural response to accelerating flow. Can J Fish Aquat Sci. 66(12):2109–2117. doi: 10.1139/F09-141.
- Evans AF, Beaty RE, Fitzpatrick MS, Collis K. 2004. Identification and enumeration of steelhead kelts at a Snake River hydroelectric dam. Trans Am Fish Soc. 133(5):1089–1099. doi: 10.1577/T03-121.1.
- Evans AF, Wertheimer RH, Keefer ML, Boggs CT, Peery CA, Collis K. 2008. Transportation of steelhead kelts to increase iteroparity in the Columbia and Snake Rivers. N Am J Fish Manage. 28(6):1818–1827. doi: 10.1577/M08-043.1.
- Ferguson JW, Matthews GM, McComas RL, Absolon RF, Brege DA, Gessel MH, Gilbreath LG. 2005. Passage of adult and juvenile salmonids through federal Columbia River power system dams. Seattle (Washington): U.S. Department of Commerce [accessed 2022 Oct 31]. https://groups.nceas.ucsb.edu/flow-experiments/documents/overviews-and-reviews/Ferguson%20et%20al.2005.fishpassagecolumbiariver.NOAATechMemo64.pdf/at_download/file.
- Ferguson JW, Ploskey GR, Leonardsson K, Zabel RW, Lundqvist H. 2008. Combining turbine blade-strike and life cycle models to assess mitigation strategies for fish passing dams. Can J Fish Aquat Sci. 65(8):1568–1585. doi: 10.1139/F08-078.
- Fleming IA. 1996. Reproductive strategies of Atlantic salmon: ecology and evolution. Rev Fish Biol Fisheries. 6(4):379–416. doi: 10.1007/BF00164323.
- Fuchs NT, Caudill CC, Murdoch AR, Truscott BL. 2021. Overwintering distribution and postspawn survival of steelhead in the upper Columbia River Basin. N Am J Fish Manag. 41(3):757–774. doi: 10.1002/nafm.10585.
- Geist J. 2021. Green or red: challenges for fish and freshwater biodiversity conservation related to hydropower. Aquat Conserv. 31(7):1551–1558. doi: 10.1002/aqc.3597.
- Halttunen E, Jensen JLA, Næsje TF, Davidsen JG, Thorstad EB, Chittenden CM, Hamel S, Primicerio R, Rikardsen AH. 2013. State-dependent migratory timing of postspawned Atlantic salmon (Salmo salar). Can J Fish Aquat Sci. 70(7):1063–1071. doi: 10.1139/cjfas-2012-0525.
- Hatch DR, Branstetter R, Narum S. 2003. Evaluate steelhead (Oncorhynchus mykiss) kelt outmigration from Lower Granite Dam to Bonneville Dam and test the use of transportation to increase returns of repeat spawners. Oregon: Columbia River Inter-Tribal Fish Commission [accessed 2022 Aug 8]. https://critfc.org/wp-content/uploads/2021/08/03_07report.pdf.
- Heggenes J, Stickler M, Alfredsen K, Brittain JE, Adeva-Bustos A, Huusko A. 2021. Hydropower-driven thermal changes, biological responses and mitigating measures in northern river systems. River Res Appl. 37(5):743–765. doi: 10.1002/rra.3788.
- Heinimaa S, Heinimaa P. 2004. Effect of the female size on egg quality and fecundity of the wild Atlantic salmon in the sub-arctic River Teno. Boreal Environ Res 9:55–62.
- International Energy Agency. 2021. Renewables 2021. Paris, France: IEA. https://www.iea.org/reports/renewables-2021.
- IPCC 2022. Climate change 2022: impacts, adaptation and vulnerability. Cambridge (UK) and New York: Cambridge University Press. doi: 10.1017/9781009325844.
- Jonsson N, Hansen LP, Jonsson B. 1991. Variation in age, size and repeat spawning of adult atlantic salmon in relation to river discharge. J Anim Ecol. 60(3):937–947. doi: 10.2307/5423.
- Jonsson N, Jonsson B, Hansen LP. 1991. Energetic cost of spawning in male and female Atlantic salmon (Salmo salar L.). J Fish Biol. 39(5):739–744. doi: 10.1111/j.1095-8649.1991.tb04403.x.
- Keefer ML, Wertheimer RH, Evans AF, Boggs CT, Peery CA. 2008. Iteroparity in Columbia River summer-run steelhead (Oncorhynchus mykiss): implications for conservation. Can J Fish Aquat Sci. 65(12):2592–2605. doi: 10.1139/F08-160.
- Keefer ML, Jepson MA, Clabough TS, Johnson EL, Narum SR, Hess JE, Caudill CC. 2018. Sea-to-sea survival of late-run adult steelhead (Oncorhynchus mykiss) from the Columbia and Snake rivers. Can J Fish Aquat Sci. 75(3):331–341. doi: 10.1139/cjfas-2016-0430.
- Kendall NW, Marston GW, Klungle MM. 2017. Declining patterns of Pacific Northwest steelhead trout (Oncorhynchus mykiss) adult abundance and smolt survival in the ocean. Can J Fish Aquat Sci. 74(8):1275–1290. doi: 10.1139/cjfas-2016-0486.
- Klemetsen A, Amundsen P-A, Dempson JB, Jonsson B, Jonsson N, O”Connell MF, Mortensen E. 2003. Atlantic salmon Salmo salar L., brown trout Salmo trutta L. and Arctic charr Salvelinus alpinus (L.): a review of aspects of their life histories. Ecol Freshw Fish. 12(1):1–59. doi: 10.1034/j.1600-0633.2003.00010.x.
- Knott J, Mueller M, Pander J, Geist J. 2023. Bigger than expected: Species- and size-specific passage of fish through hydropower screens. Ecol Eng. 188:106883. doi: 10.1016/j.ecoleng.2022.106883.
- Kraabøl M, Arnekleiv JV, Museth J. 2008. Emigration patterns among trout, Salmo trutta (L.), kelts and smolts through spillways in a hydroelectric dam. Fish Manag Ecol. 15(5–6):417–423. doi: 10.1111/j.1365-2400.2008.00633.x.
- Kuriqi A, Pinheiro AN, Sordo-Ward A, Bejarano MD, Garrote L. 2021. Ecological impacts of run-of-river hydropower plants—Current status and future prospects on the brink of energy transition. Renew Sustainable Energy Rev. 142:110833. doi: 10.1016/j.rser.2021.110833.
- Lawrence ER, Kuparinen A, Hutchings JA. 2016. Influence of dams on population persistence in Atlantic salmon (Salmo salar). Can J Zool. 94(5):329–338. doi: 10.1139/cjz-2015-0195.
- Leander J, Hellström G, Nordin J, Jonsson M, Klaminder J. 2024. Guiding downstream migrating Atlantic salmon (Salmo salar) and brown trout (Salmo trutta) of different life stages in a large river using bubbles. River Res Appl. 40(1):107–115. doi: 10.1002/rra.4209.
- Liao JC. 2007. A review of fish swimming mechanics and behaviour in altered flows. Philos Trans R Soc Lond B Biol Sci. 362(1487):1973–1993. doi: 10.1098/rstb.2007.2082.
- Limburg KE, Waldman JR. 2009. Dramatic declines in North Atlantic diadromous Fishes. BioScience. 59(11):955–965. doi: 10.1525/bio.2009.59.11.7.
- Meister J, Selz OM, Beck C, Peter A, Albayrak I, Boes RM. 2022. Protection and guidance of downstream moving fish with horizontal bar rack bypass systems. Ecol Eng. 178:106584. doi: 10.1016/j.ecoleng.2022.106584.
- Moffett IJJ, Allen M, Flanagan C, Crozier WW, Kennedy GJA. 2006. Fecundity, egg size and early hatchery survival for wild Atlantic salmon, from the River Bush. Fish Manage Ecol. 13(2):73–79. doi: 10.1111/j.1365-2400.2006.00478.x.
- Moore A, Privitera L, Ives MJ, Uzyczak J, Beaumont WRC. 2018. The effects of a small hydropower scheme on the migratory behaviour of Atlantic salmon Salmo salar smolts. J Fish Biol. 93(3):469–476. doi: 10.1111/jfb.13660.
- Moore JW, Yeakel JD, Peard D, Lough J, Beere M. 2014. Life-history diversity and its importance to population stability and persistence of a migratory fish: steelhead in two large North American watersheds. J Anim Ecol. 83(5):1035–1046. doi: 10.1111/1365-2656.12212.
- Moran EF, Lopez MC, Moore N, Müller N, Hyndman DW. 2018. Sustainable hydropower in the 21st century. Proc Natl Acad Sci USA 115(47):11891–11898. doi: 10.1073/pnas.1809426115.
- Mueller M, Pander J, Geist J. 2017. Evaluation of external fish injury caused by hydropower plants based on a novel field-based protocol. Fish Manage Eco. 24(3):240–255. doi: 10.1111/fme.12229.
- Mueller M, Sternecker K, Milz S, Geist J. 2020. Assessing turbine passage effects on internal fish injury and delayed mortality using X-ray imaging. PeerJ 8:e9977. doi: 10.7717/peerj.9977.
- Mueller M, Knott J, Pander J, Geist J. 2022. Experimental comparison of fish mortality and injuries at innovative and conventional small hydropower plants. J Appl Ecol. 59(9):2360–2372. doi: 10.1111/1365-2664.14236.
- Narum SR, Hatch D, Talbot AJ, Moran P, Powell MS. 2008. Iteroparity in complex mating systems of steelhead Oncorhynchus mykiss (Walbaum). J Fish Biol. 72(1):45–60. doi: 10.1111/j.1095-8649.2007.01649.x.
- Noatch MR, Suski CD. 2012. Non-physical barriers to deter fish movements. Environ Rev. 20(1):71–82. doi: 10.1139/a2012-001.
- Nyqvist D, Bergman E, Calles O, Greenberg L. 2017. Intake approach and dam passage by downstream-migrating Atlantic salmon kelts. River Res Appl. 33(5):697–706. doi: 10.1002/rra.3133.
- Nyqvist D, Calles O, Bergman E, Hagelin A, Greenberg LA. 2016. Post-spawning survival and downstream passage of landlocked Atlantic salmon (Salmo salar) in a regulated river: is there potential for repeat spawning? River Res Appl. 32(5):1008–1017. doi: 10.1002/rra.2926.
- Nyqvist D, Nilsson PA, Alenäs I, Elghagen J, Hebrand M, Karlsson S, Kläppe S, Calles O. 2017. Upstream and downstream passage of migrating adult Atlantic salmon: remedial measures improve passage performance at a hydropower dam. Ecol Eng 102:331–343. doi: 10.1016/j.ecoleng.2017.02.055.
- Oliveira AG, Baumgartner MT, Gomes LC, Dias RM, Agostinho AA. 2018. Long-term effects of flow regulation by dams simplify fish functional diversity. Freshw Biol. 63(3):293–305. doi: 10.1111/fwb.13064.
- Östergren J, Rivinoja P. 2008. Overwintering and downstream migration of sea trout (Salmo trutta L.) kelts under regulated flows—Northern Sweden. River Res Appl. 24(5):551–563. doi: 10.1002/rra.1141.
- Penney ZL, Moffitt CM. 2014. Histological assessment of organs in sexually mature and post-spawning steelhead trout and insights into iteroparity. Rev Fish Biol Fish. 24(3):781–801. doi: 10.1007/s11160-013-9338-2.
- Penney ZL, Moffitt CM. 2014. Proximate composition and energy density of stream-maturing adult steelhead during upstream migration, sexual maturity, and kelt emigration. Trans Am Fish Soc. 143(2):399–413. doi: 10.1080/00028487.2013.862184.
- Penney ZL, Moffitt CM. 2015. Fatty-acid profiles of white muscle and liver in stream-maturing steelhead trout Oncorhynchus mykiss from early migration to kelt emigration. J Fish Biol. 86(1):105–120. doi: 10.1111/jfb.12552.
- Quinn TP, Seamons TR, Vøllestad LA, Duffy E. 2011. Effects of growth and reproductive history on the egg size–fecundity trade-off in steelhead. Trans Am Fish Soc. 140(1):45–51. doi: 10.1080/00028487.2010.550244.
- Persson L, Raunsgard A, Thorstad EB, Østborg G, Urdal K, Sægrov H, Ugedal O, Hindar K, Karlsson S, Fiske P, et al. 2022. Iteroparity and its contribution to life-history variation in Atlantic salmon. Can J Fish Aquat Sci. 80(3):577–592. doi: 10.1139/cjfas-2022-0126.
- Pracheil BM, DeRolph CR, Schramm MP, Bevelhimer MS. 2016. A fish-eye view of riverine hydropower systems: the current understanding of the biological response to turbine passage. Rev Fish Biol Fish. 26(2):153–167. doi: 10.1007/s11160-015-9416-8.
- Reid JE, Chaput G. 2012. Spawning history influence on fecundity, egg size, and egg survival of Atlantic salmon (Salmo salar) from the Miramichi River, New Brunswick, Canada. ICES J Mar Sci. 69(9):1678–1685. doi: 10.1093/icesjms/fss091.
- Ruckelshaus MH, Levin P, Johnson JB, Kareiva PM. 2002. The Pacific Salmon Wars: what science brings to the challenge of recovering species. Annu Rev Ecol Syst. 33(1):665–706. doi: 10.1146/annurev.ecolsys.33.010802.150504.
- Rytwinski T, Algera DA, Taylor JJ, Smokorowski KE, Bennett JR, Harrison PM, Cooke SJ. 2017. What are the consequences of fish entrainment and impingement associated with hydroelectric dams on fish productivity? A systematic review protocol. Environ Evid. 6(1):8. doi: 10.1186/s13750-017-0087-x.
- Schindler DE, Hilborn R, Chasco B, Boatright CP, Quinn TP, Rogers LA, Webster MS. 2010. Population diversity and the portfolio effect in an exploited species. Nature. 465(7298):609–612. doi: 10.1038/nature09060.
- Scruton DA, Pennell CJ, Bourgeois CE, Goosney RF, King L, Booth RK, Eddy W, Porter TR, Ollerhead LMN, Clarke KD. 2008. Hydroelectricity and fish: a synopsis of comprehensive studies of upstream and downstream passage of anadromous wild Atlantic salmon, Salmo salar, on the Exploits River, Canada. Hydrobiologia. 609(1):225–239. doi: 10.1007/s10750-008-9410-4.
- Scruton DA, Pennell CJ, Bourgeois CE, Goosney RF, Porter TR, Clarke KD. 2007. Assessment of a retrofitted downstream fish bypass system for wild Atlantic salmon (Salmo salar) smolts and kelts at a hydroelectric facility on the Exploits River, Newfoundland, Canada. Hydrobiologia. 582(1):155–169. doi: 10.1007/s10750-006-0557-6.
- Seamons TR, Quinn TP. 2010. Sex-specific patterns of lifetime reproductive success in single and repeat breeding steelhead trout (Oncorhynchus mykiss). Behav Ecol Sociobiol. 64(4):505–513. doi: 10.1007/s00265-009-0866-7.
- Silva AT, Bærum KM, Hedger RD, Baktoft H, Fjeldstad H-P, Gjelland KØ, Økland F, Forseth T. 2020. The effects of hydrodynamics on the three-dimensional downstream migratory movement of Atlantic salmon. Sci Total Environ. 705:135773. doi: 10.1016/j.scitotenv.2019.135773.
- Silva AT, Lucas MC, Castro-Santos T, Katopodis C, Baumgartner LJ, Thiem JD, Aarestrup K, Pompeu PS, O”Brien GC, Braun DC, et al. 2018. The future of fish passage science, engineering, and practice. Fish Fish. 19(2):340–362. doi: 10.1111/faf.12258.
- Simmons OM, Gregory SD, Gillingham PK, Riley WD, Scott LJ, Britton JR. 2021. Biological and environmental influences on the migration phenology of Atlantic salmon Salmo salar smolts in a chalk stream in southern England. Freshw Biol. 66(8):1581–1594. doi: 10.1111/fwb.13776.
- Smialek N, Pander J, Geist J. 2021. Environmental threats and conservation implications for Atlantic salmon and brown trout during their critical freshwater phases of spawning, egg development and juvenile emergence. Fish Manage Ecol. 28(5):437–467. doi: 10.1111/fme.12507.
- Smith DL, Goodwin RA, Nestler JM. 2014. Relating turbulence and fish habitat: a new approach for management and research. Rev Fish Sci Aquac. 22(2):123–130. doi: 10.1080/10641262.2013.803516.
- Thorpe JE, Miles MS, Keay DS. 1984. Developmental rate, fecundity and egg size in Atlantic salmon, Salmo salar L. Aquaculture 43(1–3):289–305. doi: 10.1016/0044-8486(84)90030-9.
- Thorstad EB, Whoriskey F, Uglem I, Moore A, Rikardsen AH, Finstad B. 2012. A critical life stage of the Atlantic salmon Salmo salar: behaviour and survival during the smolt and initial post-smolt migration. J Fish Biol. 81(2):500–542. doi: 10.1111/j.1095-8649.2012.03370.x.
- Trammell JLJ, Fast DE, Hatch DR, Bosch WJ, Branstetter R, Pierce AL, Blodgett JW, Frederiksen CR. 2016. Evaluating steelhead kelt treatments to increase iteroparous spawners in the Yakima River Basin. N Am J Fish Manage. 36(4):876–887. doi: 10.1080/02755947.2016.1165767.
- Vikström L, Leonardsson K, Leander J, Shry S, Calles O, Hellström G. 2020. Validation of Francis–Kaplan turbine blade strike models for adult and juvenile Atlantic Salmon (Salmo salar, L.) and anadromous brown trout (Salmo trutta, L.) passing high head turbines. Sustainability. 12(16):6384. doi: 10.3390/su12166384.
- Vollset KW, Lennox RJ, Lamberg A, Skaala Ø, Sandvik AD, Sægrov H, Kvingedal E, Kristensen T, Jensen AJ, Haraldstad T, et al. 2021. Predicting the nationwide outmigration timing of Atlantic salmon (Salmo salar) smolts along 12 degrees of latitude in Norway. Divers Distrib. 27(8):1383–1392. doi: 10.1111/ddi.13285.
- Wang B, Yang X, Li S-L, Liang X, Li X-D, Wang F, Yang M, Liu C-Q. 2022. Anthropogenic regulation governs nutrient cycling and biological succession in hydropower reservoirs. Sci Total Environ. 834:155392. doi: 10.1016/j.scitotenv.2022.155392.
- Weiland MA, Kim J, Nagy WT, Johnson GE. 2009. Evaluation of steelhead kelt passage into the Bonneville Dam second Powerhouse corner collector prior to the juvenile migration seasons, 2007 and 2008. Washington: Pacific Northwest National Laboratory [accessed 2022 Oct 31]. doi: 10.2172/1001548.
- Wertheimer RH. 2007. Evaluation of a surface flow bypass system for steelhead kelt passage at Bonneville Dam, Washington. N Am J Fish Manage. 27(1):21–29. doi: 10.1577/M05-216.1.
- Wertheimer RH, Evans AF. 2005. Downstream passage of steelhead kelts through hydroelectric dams on the Lower Snake and Columbia Rivers. Trans Am Fish Soc. 134(4):853–865. doi: 10.1577/T04-219.1.
- Young PS, Cech JJ, Thompson LC. 2011. Hydropower-related pulsed-flow impacts on stream fishes: a brief review, conceptual model, knowledge gaps, and research needs. Rev Fish Biol Fish. 21(4):713–731. doi: 10.1007/s11160-011-9211-0.
- Zarfl C, Lumsdon AE, Berlekamp J, Tydecks L, Tockner K. 2015. A global boom in hydropower dam construction. Aquat Sci. 77(1):161–170. doi: 10.1007/s00027-014-0377-0.
Appendix A
Table A1. An overview of the key aspects of each kelt study included in the review.