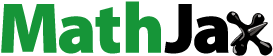
Abstract
In the present study, ventilation coefficient (VC) was estimated in order to understand the concentration of pollutants over the urban region of Hyderabad during 2009–2011. Nocturnal boundary layer height (BLH) was calculated using the accurate (0.2% for Refractivity Profiles) high vertical resolution global positioning system radio occultation (GPSRO), Constellation Observing System for Meteorology Ionosphere and Climate (COSMIC)-derived one-dimensional variational (1-DVAR) refractivity profiles, and observations of boundary layer LIDAR (BLL). The statistical results revealed that the coefficient of determination (R2) between LIDAR- and GPSRO-estimated BLH is 0.51, whereas that for VC is 0.68. The maximum BLH observed during the study period was maximum in the summer season and the minimum was observed in monsoon season, whereas the maximum VC was observed during summer and the minimum in winter. When the BLH and VC are low, the pollutant concentration of black carbon (BC) is higher and vice versa. This means that the dispersion of pollutants in the lower atmosphere is due to convective mixing. It was found that high VC values could dilute air pollutants whereas low values leads to more pollution.
Public Interest Statement
The present research is very useful to the society/public, as nowadays the pollution levels are increasing day by day and it is useful to generate public awareness on pollution levels over metropolitan city. This kind of research is useful for policy-makers to take decision on pollution levels for taking further action.
1. Introduction
Air pollution is one of the most important serious issues in urban regions (Motesaddi Zarandi, Khajevandi, DamezFontaine, & Ardestani, Citation2008) resulting in serious health issues (Goss et al., Citation2014). This is particularly due to the variations in physical–chemical properties of aerosols in urban regions as compared to rural/remote areas (Latha & Badarinath, Citation2004). The environmental effects of atmospheric aerosols are the hot topics in global science community, and their radiative properties are one of the important parameters in assessing climate change (Houghton et al., Citation1996). The atmospheric boundary layer (ABL) is the lowest part of the troposphere, where air is influenced by the earth’s surface and responds to the surface forcing such as frictional drag, evapotranspiration, heat transfer, pollutant emission, and topography (Cooper & Eichinger, Citation1994).
The boundary layer height (BLH) is one of the important parameter that determines the ability of pollutants to disperse and about the structure of turbulence in the boundary layer (Seibert et al., Citation2000). It is also one of the crucial parameter in weather phenomena and climate models that is directly influenced by the land surface processes. The surface ABL plays a vital role in exchange of heat, momentum, moisture, as well as the pollutants transfer. It varies both in time and space from hundreds of meters to a few kilometers.
The BLH alone cannot explain about the concentrations of pollutants. Thus, it is important to obtain estimates of the ventilation coefficient (VC) for the air pollution monitoring and assessment. During the last few decades, there are many studies related to the BLH and VC in order to understand the air quality (e.g. Devara & Raj, Citation1993; Krishnan & Kunhikrishnan, Citation2004).
Black carbon (BC) is one of the major pollutants in the urban area. It is relatively inert (Bodhaine, Citation1995; Hansen et al., Citation1989; Sciare et al., Citation2009) in nature due to its predominant submicron size and hence can be transported over great distances. It has a major impact not only in human health (Grahame, Klemm, & Schlesinger, Citation2014; Janssen et al., Citation2011, Citation2012) and visibility, but also on global and regional climate (Babu, Satheesh, & Moorthy, Citation2002; Drinovec et al., Citation2015). Burning of biomass and fossil fuels, automobiles exhaust, forest fires are the major sources of BC (Badarinath, Kharol, Kaskaoutis, & Kambezidis, Citation2007). BC and particulate matter (PM) emissions over the study region were analyzed by Mahalakshmi, Sujatha, Naidu, and Chowdary (Citation2014) during public transport halt and they found enormous drop in BC and PM when compared to the normal days.
Concentration of the pollutants at any given location is governed by boundary layer dynamics and wind strength. In addition, local emissions (Mahalakshmi et al., Citation2014; Sharma, Kharol, & Badarinath, Citation2010) also contribute to the pollution levels. Hence, the quantity of dispersion of the particles depends on VC (Iyer & Raj, Citation2013; Mahalakshmi, Badrinath, & Naidu, Citation2011). Many researchers recently observed that VC (combination of BLH and wind speed) plays a major role in the dispersion of aerosols.
VC (m2s−1) was estimated as product of the BLH and the mean wind speed (WS) in ms−1 within BLH (m) as shown in Equation (1) (Ashrafi, Shafie-Pour, & Kamalan, Citation2009):(1)
(1)
Here Zi is the BLH and U is the mean wind speed within the BLH.
The conventional approach to calculate BLH is to use the radiosonde observations that are very sparse both in space and time for the BLH and the VC calculations. It can overcome by model simulations. But, the model-simulated temperature profiles are not as good as observations (Thorne et al., Citation2011). In view of the importance of these parameters, the monthly and seasonal variations in VC associated with air pollutants over the urban region of Hyderabad were analyzed in this study.
1.1. Experimental site
The continuous and synchronous measurements were made in the premises of National Remote Sensing Centre (NRSC) at Balanagar (Hyderabad) during the period from 2009 to 2011. Hyderabad is an urban city, situated at Telangana state of India. It is one of the metropolitan cities in Southern Peninsular India with a population of 6,809,970 (as per 2011 census). It has been classified as an A-1 (Categories of cities in India) in terms of development priorities, due to its size, population, and impact. The city is not only influenced by vehicular and anthropogenic, but also long-range transport of biomass burning, dust storms, and forest fire emissions.
2. Data-sets and methodology
Global positioning system radio occultation (GPSRO, hereafter RO), Constellation Observing System for Meteorology Ionosphere and Climate (COSMIC)-derived 1-DVAR refractivity profiles, obtained from COSMIC Data Analysis and Archive Center (CDAAC) website: http://tacccub.gov.tw, were used for the estimation of BLH during 2009–2011. It is in operational mode since April 2006. The vertical and spatial resolutions of RO were 100 m and 300 km, respectively. The errors of RO were 0.2% between 10 and 20 km altitude for refractivity and better than 1 Kelvin for temperature in upper tropospheric–lower stratospheric region (Kursinski & Hajj, Citation2001; Kursinski, Hajj, Schofield, Linfield, & Hardy, Citation1997; Kuo et al., Citation2004; Neiman et al., Citation2008; Schreiner, Rocken, Sokolovskiy, Syndergaard, & Hunt, Citation2007; Sokolovskiy et al., Citation2006; Yunck et al., Citation2009). COSMIC 1-DVAR refractive profiles collocated with LIDAR instrument (48 points) were used during the study period.
Continuous, near real-time measurements of the vertical profile of backscatter coefficient is carried out using nighttime portable Micro Pulse LIDAR (MPL) system at 532 nm fabricated by National Atmospheric Research Laboratory (Kumar, Citation2006). The output pulse energy is 2–20 μJ depending on the repetition rate. It was operated at 2,500-Hz repetition rate and 10-μJ output pulse energy. The raw data profile was integrated every 10-min interval. The laser system bin width was set at 200 ns, corresponding to a height resolution of 30 m. The backscatter intensity measured by LIDAR is proportional to the signal back scattering by particles and air molecules present in the atmosphere. Further specifications of the LIDAR can be found in Kumar (Citation2006).
Furthermore, wind speed and direction play a crucial role in the air pollutants dispersion. Thus, wind speed (WS) profile data were used in the present study associated with BLH. It was taken from radiosonde observations of National Oceanic and Atmospheric Administration (NOAA)/Earth System Research Laboratory (ESRL). The seasonal wind back trajectories ending at Hyderabad (up to 500 m) were considered in this study to understand the transportation of pollutants with the aid of Hybrid Single-Particle Lagrangian Trajectory (HYSPLIT) model (Draxler & Rolph, Citation2003).
The BLH was estimated from refractivity profiles (dN/dz) of COSMIC GPSRO and the LIDAR instrument operated at Hyderabad. The refractivity profiles are differentiated with respect to the height and then minimum gradient is considered as the BLH, following Sokolovskiy et al. (Citation2006), Basha and Ratnam (Citation2009). Recent improvements in retrieval technique and instrumentation, the quality of data in the lower troposphere along with high vertical resolution leads to BLH estimates. BLH from LIDAR profiles is estimated as the minimum vertical gradient of the backscatter profiles based on the method presented by Flamant, Pelon, Flamant, and Durand (Citation1997) was used in this study. Typical BLH estimation using LIDAR and COSMIC GPSRO respectively on 14 February 2011 was shown in Figure . The BLH was 2.5 km from the LIDAR as well as COSMIC RO. Further, VC has been estimated using the BLH and WS as discussed above.
In addition, continuous and near real-time BC aerosol measurements were made with Aethalometer (AE-31 model, Magee Scientific, USA) during the study period with a time resolution of 5 min. It aspires ambient air from an altitude of 3 m above the ground using inlet tube and its pump. The instrument uses quartz fiber filter tape through which air is passed with fixed flow rate of 2.9 liter per minute (LPM). At the end of the each measurement cycle, changes in the filter transmission at seven wavelengths (370, 470, 520, 590, 660, 880, and 950 nm) are recorded. BC at 880 nm channel was used in the present study since it is considered as the standard channel for BC measurement (Weigarnter et al., Citation2003) as other aerosol particles absorb very less and absorption at this wavelength can be attributed to BC alone. The typical uncertainties associated with the BC concentrations retrieved from the Aethalometer were around 10% as given by the manufacturer.
3. Results and discussion
In general, ground-based LIDAR measurements are limited in space and time. An alternative is to use remote sensing techniques. Global positioning system radio occultation (GPSRO) technique provides a very accurate and high-resolution refractivity profiles in all weather conditions (Hajj et al., Citation2000; Kursinski, Hajj, Leroy, & Herman, Citation2000; Kursinski et al., Citation1997; Steiner, Kirchengast, & Ladreiter, Citation1999; Yunck, Liu, & Ware, Citation2000). As discussed in above section, RO observations provide an opportunity to calculate BLH. In view of this, LIDAR and COSMIC GPSRO estimates of BLH and VC were compared. The BLH and VC estimated using COSMIC GPSRO observations were correlated well with LIDAR estimates (Figure (a) & (b)). The coefficient of determination (R2) for boundary layer height is 0.51 and for VC is 0.68.
Figure 2. Scatter between estimated (a) BLH & (b) VC using LIDAR & RO observations during 2009–2011.
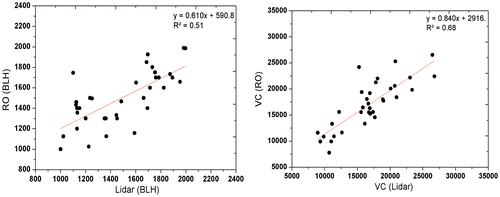
The monthly variations of wind speed are shown in Figure (a), respectively, during the period from 2009–2011 at study site. WS is observed to be high during July (southwest monsoon season) and low in January (winter season). Similarly, the monthly variations of BLH can be seen from the figures (Figure (b)) with gradual rise from January to May and then sudden drop in June during the study period at urban region of Hyderabad. It was noticed that higher values in May as a result of high thermal convection processes during summer/pre-monsoon season and lower values are in July (southwest monsoon).
The monthly variations of VC are shown in Figure (c). The highest value of VC is observed during May (i.e. in summer) due to high BLH obtained from LIDAR (RO) 19,735 m2s−1 (19,568 m2s−1). The lowest value of VC is observed during January (i.e. in winter) with mean value of 13,331 m2s−1 (11,773 m2s−1). The lowest value during winter is associated with low BLH and WS. It shows that the dispersion of pollutants in the lower atmosphere is due to the convection and mixing. Thus, higher the VC value leads to low pollution, and low pollution values lead to more pollution.
The monthly variation of BC is shown in Figure . It is obvious from the figure that low values during the monsoon season are as a result of strong westerly winds and that is air is mainly transported from cleaner marine region (Figure (b)) during the monsoon seasons with poor BC concentrations (Figure ). In order to understand the possible sources of high-aerosol loading during the study period, we have analyzed NOAA-HYSPLIT backward trajectories as shown in Figure ((a)–(d)). It was found that the concentration of BC is much higher in winter season than any other seasons due to longer residence time of particulates in the atmosphere associated with low VC. Similar results were reported earlier (Krishnan & Kunhikrishnan, Citation2004; Mahalakshmi et al., Citation2011). Figure explains about the correlation between BC concentration and VC and these both are inversely related to each other as shown in earlier studies.
Figure 5. HYSPLIT wind back trajectories at 500 m. (a) Premonsoon (b) Monsoon (c) Postmonsoon, and (d) Winter
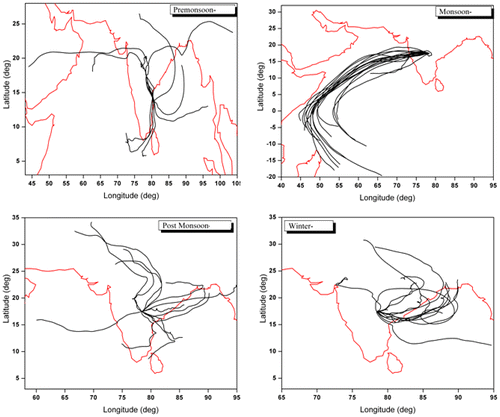
Biomass burning due to either forest fires or agricultural residue burning is an important source of anthropogenic emissions in several regions of India (Badarinath, Kiran Chand, & Prasad, Citation2006; Badarinath, Kharol, & Sharma, Citation2009). For example, agricultural crop residues burnt in the Indo Gangetic Plains (IGP) during the months of October and November each year has significant impact (Badarinath et al., Citation2006; Verma, Venkataraman, & Boucher, Citation2008). The long-range transport of aerosols (Figure (a), (c)) from the adjoining regions is associated with remarkable changes in the concentration of the BC aerosols (e.g. Beegum et al., Citation2008; Moorthy, Babu, & Satheesh, Citation2003, Citation2005) over the study region during pre- and post-monsoon seasons. The emissions from the intense forest fire activities result in higher BC aerosols over the region (Figure ). It was also reported by Badarinath et al. (Citation2007) that each year intense forest fire activities take place in the northeast region of India during February–May with a peak in March and April.
Dispersion of pollutants is dependent upon many meteorological parameters (Collier et al., Citation2005), most significant being wind speed and BLH that defines the volume of air through which the pollution is mixed. The results shows that higher VC value leads to low concentrations of BC, less pollution, whereas low values leads to more concentration of BC, more pollution. The results mentioned show that the VC significantly influences the BC concentrations.
4. Summary and conclusions
The highest value of VC observed was during summer compared to the other seasons. This could be due to the higher values of BLH. However, lower values of BLH and WS result in lower values of VC during winter period. The stronger winds carry the pollutants away from the region which disperses and dilute them. Pollutant particles are generally suspended up to the height of BLH. Above the boundary layer concentration of the pollutants are very less.
Acknowledgments
We would like to thank our Director NRSC for his support and encouragement to carry out this work. The authors gratefully acknowledge Biswadip Gharai and Subin Jose for providing LIDAR and BC data. The authors also thank CDAAC for providing RO profiles and NOAA for providing HYSPLIT Model.
Additional information
Funding
Notes on contributors
P. Sujatha
The author joined NRSC in November, 2011 as a research scholar. Recently, she submitted her PhD degree in the Meteorology and Oceanography department from Andhra University, Visakhapatnam. Her doctoral work focused on “Characterization of Troposphere over Indian region using GPSRO technique.” Her research interest includes atmospheric aerosols and trace gases, satellite remote sensing using multi sensors data.
References
- Ashrafi, K., Shafie-Pour, M., & Kamalan, H. (2009). Estimating temporal and seasonal variation of ventilation coefficients. International Journal of Environmental Research, 3, 637–644.
- Babu, S. S., Satheesh, S. K., & Moorthy, K. K. (2002). Aerosol radiative forcing due to enhances black carbon at an urban site in India. Geophysical Research Letters, 18, 27-1–27-4.
- Badarinath, K. V. S., Kharol, S. K., Kaskaoutis, D. G., & Kambezidis, H. D. (2007). Case study of a dust storm over Hyderabad area, India: Its impact on solar radiation using satellite data and ground measurements. Science of The Total Environment, 384, 316–332.10.1016/j.scitotenv.2007.05.031
- Badarinath, K. V. S., Kharol, S. K., & Sharma, A. R. (2009). Long-range transport of aerosols from agriculture crop residue burning in Indo-Gangetic Plains-A study using LIDAR, ground measurements and satellite data. Journal of Atmospheric and Solar-Terrestrial Physics, 71, 112–120.10.1016/j.jastp.2008.09.035
- Badarinath, K. V. S., Kiran Chand, T. R., & Prasad, K. V. (2006). Agricultural crop residue burning in the Indo-Gangetic Plains-a study using IRS-P6 AWiFS satellite data. Current Science, 91, 1085–1089.
- Basha, G., & Ratnam, M. V. (2009). Identification of atmospheric boundary layer height over a tropical station using high-resolution radiosonde refractivity profiles: Comparison with GPS radio occultation measurements. Journal of Geophysical Research, 114, D16101. doi:10.1029/2008JD011692
- Beegum, S. N., Moorthy, K. K., Nair, V. S., Babu, S. S., Satheesh, S. K., Vinoj, V., … Singh, D. (2008). Characteristics of spectral aerosol optical depths over India during ICARB. Journal of Earth System Science, 117, 303–313.10.1007/s12040-008-0033-y
- Bodhaine, B. A. (1995). Aerosol absorption measurements at Barrow, Mauna Loa and the south pole. Journal of Geophysical Research, 100, 8967–8975.10.1029/95JD00513
- Collier, C. G., Davies, F., Bozier, K. E., Holt, A. R., Middleton, D. R., Pearson, G. N., … Young, R. I. (2005). Dual-Doppler lidar measurements for improving dispersion models. Bulletin of the American Meteorological Society, 86, 825–838.10.1175/BAMS-86-6-825
- Cooper, D. I. & Eichinger, W. E. (1994). Structure of the atmosphere in an urban planetary boundary layer from lidar and radiosonde observations. Journal of Geophysical Research, 99, 22937–22948.10.1029/94JD01944
- Devara, P. C. S., & Raj, P. E. (1993). Lidar measurements of aerosols in the tropical atmosphere. Advances in Atmospheric Sciences, 10, 365–378.10.1007/BF02658142
- Draxler, R. R., & Rolph, G. D. (2003). HYSPLIT (Hybrid Single-Particle Lagrangian Integrated Trajectory) model. Silver Spring, MD: NOAA Air Resource Lab.
- Drinovec, L., Močnik, G., Zotter, P., Prévôt, A. S. H., Ruckstuhl, C., Coz, E., … Hansen, A. D. A. (2015). The “dual-spot” aethalometer: An improved measurement of aerosol black carbon with real-time loading compensation. Atmospheric Measurement Techniques Discussions, 8, 1965–1979. doi:10.5194/amt-8-1965-2015
- Flamant, C., Pelon, J., Flamant, P. H., & Durand, P. (1997). Lidar determination of the entrainment zone thickness at the top of the unstable marine atmospheric boundary layer. Boundary Layer Meteorology, 83, 247–284.10.1023/A:1000258318944
- Goss, P. E., Strasser-Weippl, K., Lee-Bychkovsky, B. L., Fan, L., Li, J., Chavarri-Guerra, Y., … Chan, A. (2014). Challenges to effective cancer control in China, India, and Russia. The Lancet Oncology, 15, 489–538.10.1016/S1470-2045(14)70029-4
- Grahame, T. J., Klemm, R., & Schlesinger, R. B. (2014). Public health and components of particulate matter: The changing assessment of black carbon. Journal of the Air & Waste Management Association, 64, 620–660.10.1080/10962247.2014.912692
- Hajj, G., Xiaoqing, P., Romans, L., Schreiner, W. S., & Straus, P, & Wang, C. (2000). COSMIC gps ionospheric sensing and space weather. Terrestrial Atmospheric and Oceanic Science, 11, 235–272.
- Hansen, A. D. A., Conway, T. J., Strele, L. P., Bodhaine, B. A., Thoning, K. W., Tans, P., & Novakov, T. (1989). Correlations among combustion effluent species at Barrow, Alaska: Aerosol black carbon, carbon dioxide, and methane. Journal of Atmospheric Chemistry, 9, 283–299.10.1007/BF00052838
- Houghton, J. T., Meira Filho, L. G., Callander, B. A., Harris, N., Kattenberg, A., & Maskell, K. (Eds.) (1996). Climate change 1995: The science of climate change (pp. XII–572). Cambridge: Cambridge University Press.
- Iyer, U. S., & Raj, P. (2013). Ventilation coefficient trends in the recent decades over four major Indian metropolitan cities. Journal of Earth System Science, 122, 537–549.10.1007/s12040-013-0270-6
- Janssen, N. A. H., Gerlofs-Nijland, M. E., Lanki, T., Salonen, R. O., Cassee, F., Hoek, G., … Krzyzanowski, M. (2012). Health effects of black carbon. Copenhagen: The WHO European Centre for Environment and Health, Bonn, World Health Organisation Regional 30, Office for Europe.
- Janssen, N. A. H., Hoek, G., Simic-Lawson, M., Fischer, P., van Bree, L., ten Brink, H., … Cassee, F.R. (2011). Black carbon as an additional indicator of the adverse health effects of airborne particles compared with PM10 and PM2.5. Environmental Health Perspectives, 119, 1691–1699.10.1289/ehp.1003369
- Krishnan, P., & Kunhikrishnan, P. K. (2004). Temporal variations of ventilation coefficient at a tropical Indian station using UHF wind profiler. Current Science, 86, 447–451.
- Kumar, Y. B. (2006). Portable lidar system for atmospheric boundary layer measurements. Optical Engineering, 45, 076201.10.1117/1.2221555
- Kuo, Y. H., Wee, T. K., Sokolovskiy, S., Rocken, C., Schreiner, W., Hunt, D., & Anthes, R. A. (2004). Inversion and error estimation of GPS radio occultation data. Journal of the Meteorological Society of Japan, 82, 507–531.10.2151/jmsj.2004.507
- Kursinski, E. R., & Hajj, G. A. (2001). A comparison of water vapor derived from GPS occultations and global weather analyses. Journal of Geophysical Research, 106, 1113–1138.10.1029/2000JD900421
- Kursinski, E. R., Hajj, G. A., Schofield, J. T., Linfield, R. P., & Hardy, K. R. (1997). Observing Earth’s atmosphere with radio occultation measurements using the global positioning system. Journal of Geophysical Research, 102, 23429–23465.10.1029/97JD01569
- Kursinski, R., Hajj, G., Leroy, S., & Herman, B. (2000). The GPS radio occultation technique. In Applications of constellation observing system for meteorology. ionosphere and climate (pp. 53–114). Hong Kong: Springer-Verlag.
- Latha, K. M., & Badarinath, K. V. S. (2004). Correlation between black carbon aerosols, carbon monoxide and tropospheric ozone over a tropical urban site. Atmospheric Research, 71, 265–274.10.1016/j.atmosres.2004.06.004
- Mahalakshmi, D. V., Badrinath, K. V. S., & Naidu, C. V. (2011). Influence of boundary layer dynamics on pollutant concentration over urban region-A study using ground based measurements. Indian Journal of Radio & Space physics, 40, 147–152.
- Mahalakshmi, D. V., Sujatha, P., Naidu, C. V., & Chowdary, V. M. (2014). Contribution of vehicular emission on urban air quality: Results from public strike in Hyderabad. Indian Journal of Radio & Space Physics, 43, 340–348.
- Moorthy, K. K., Babu, S. S., & Satheesh, S. K. (2003). Aerosol spectral optical depths over the Bay of Bengal: Role of transport. Geophysical Research Letters, 30, 1249.10.1029/2002GL016520
- Moorthy, K. K., Babu, S. S., & Satheesh, S. K. (2005). Aerosol characteristics and radiative impacts over the Arabian Sea during the intermonsoon season: Results from ARMEX field campaign. Journal of the Atmospheric Sciences, 62, 192–206.10.1175/JAS-3378.1
- Motesaddi Zarandi, S., Khajevandi, M., DamezFontaine, D., & Ardestani, M. (2008). Determination of air pollution monitoring stations. International Journal of Environmental Research, 2, 313–318.
- Neiman, P. J., Ralph, F. M., Wick, G. A., Kuo, Y.-H., Wee, T.-K., Ma, Z., Taylor, G. H., & Dettinger, M. D. (2008). Diagnosis of an intense atmospheric river impacting the pacific northwest storm summary and offshore vertical structure observed with COSMIC satellite retrievals. Monthly Weather Review, 136, 4398–4420.10.1175/2008MWR2550.1
- Schreiner, W., Rocken, C., Sokolovskiy, S., Syndergaard, S., & Hunt, D. (2007). Estimates of the precision of GPS radio occultations from the COSMIC/FORMOSAT-3 Mission. Geophysical Research Letters, 34, L04808. doi:10.1029/2006GL027557
- Sciare, J., Favez, O., Oikonomou, K., Sarda-Estève, R., Cachier, R., & Kazan, V. (2009). Long-term observation of carbonaceous aerosols in the Austral Ocean: Evidence of a marine biogenic origin. Journal of Geophysical Research, 114, doi:10.1029/2009JD011998
- Seibert, P., Beyrich, F., Gryning, S. E., Joffre, S., Rasmussen, A., & Tercier, P. (2000). Review and intercomparison of operational methods for the determination of the mixing height. Atmospheric Environment, 34, 1001–1027.10.1016/S1352-2310(99)00349-0
- Sharma, A. R., Kharol, S. K., & Badarinath, K. V. S. (2010). Influence of vehicular traffic on urban air quality—A case study of Hyderabad, India. Transportation Research Part D: Transport and Environment, 15, 154–159.10.1016/j.trd.2009.11.001
- Sokolovskiy, S., Rocken, C., Hunt, D., Schreiner, W., Johnson, J., Masters, D., & Esterhuizen, S. (2006). GPS profiling of the lower troposphere from space: Inversion and demodulation of the open-loop radio occultation signals. Geophysical Research Letters, 33, 2006G. doi:10.1029/L026112
- Steiner, A. K., Kirchengast, G., & Ladreiter, H. P. (1999). Inversion, error analysis, and validation of GPS/MET occultation data. Annales Geophysicae, 17, 122–138.10.1007/s005850050742
- Thorne, P. W., Brohan, P., Titchner, H. A., McCarthy, M. P., Sherwood, S. C., Peterson, T. C., … Kennedy, J. J. (2011). A quantification of uncertainties in historical tropical tropospheric temperature trends from radiosondes. Journal of Geophysical Research, 116, D12116. doi:10.1029/2010JD015487
- Verma, S., Venkataraman, C., & Boucher, O. (2008). Origin of surface and columnar Indian Ocean Experiment (INDOEX) aerosols using source and region tagged emissions transport in a general circulation model. Journal of Geophysical Research, 113, D24211.10.1029/2007JD009538
- Weigarnter, E., Saathoff, H., Schnaiter, M., Streit, N., Bitnar, B., & Baltensperger, U. (2003). Absorption of light by soot particles: Determination of the absorption coefficient by means of aethalometers. Journal of Aerosol Science, 34, 1445–1463.
- Yunck, T., Liu, C. H., & Ware, R. (2000). A history of GPS sounding. Terrestrial, Atmospheric and Oceanic Sciences, 11, 1–20.
- Yunck, T. P., Fetzer, E. J., Mannucci, A. M., Ao, C. O., Irion, F. W., Wilson, B. D., & Manipon, G. J. M. (2009). Use of radio occultation to evaluate atmospheric temperature data from spaceborne infrared sensors. Terrestrial, Atmospheric and Oceanic Sciences, 20, 71–85.10.3319/TAO.2007.12.08.01(F3C)