Abstract
The recent literature describing polycyclic aromatic hydrocarbons (PAHs) in air, water, soil and sediment, waste sludge, biomonitoring, toxicity, are reviewed. Aspects of sampling, sample preparations such as extraction of PAHs are discussed and analytical methods used are also reviewed. Developments on direct measurement techniques, such as ultraviolet absorption spectrometry and synchronous luminescence, are noted. This review also discusses the microbial PAH-remediation and PAH-degradation with emphasis on biological and physico-chemical factors influencing the biodegradation.
Public Interest Statement
The world has recently witnessed rapid growth in the awareness of PAHs in petroleum products, food, air, sediment, coal, slurge and waste. In recent times various bioremediation and biodegradation processes are now emerging to transform PAHs to less hazardous or non-hazardous compound. These processes are approaches to remove or reduce pollutant in an eco-friendly manner. They also reduce the carcinogenicity, mutagenicity and teratogenicity of PAH in contaminated soil, Water, slurge and food. Sensitive analytical techniques are thus required to adequately assess PAH human health impacts.
1. Introduction
Polycyclic aromatic hydrocarbons (PAHs) are aromatic hydrocarbons with two or more fused benzene rings in various structural configurations (Blumer, Blumer et al. Citation1977, Vichi, Pizzale et al. Citation2005, Haritash and Kaushik Citation2009, Kim, Jahan et al. 2013, Jiang, Yang et al. Citation2014, Masih and Lal Citation2014, Nguyen, Loganathan et al. Citation2014, Sharma Citation2014) and do not contain hetero atom or carry substituents. PAHs containing up to four rings are refer to as light PAHs and those that contain more than four rings are heavy PAHs. Heavy PAHs are more stable and more toxic than the light PAHs (Kuppusamy, Thavamani et al. Citation2016, Li, Wang et al. Citation2016). PAHs only consist of carbon and hydrogen bound in simple to complex ring systems, their benzene ring arrangements have a wide diversity of physical, chemical and toxicological characteristics (Cerniglia Citation1992) Table .
Table 1. Abbreviations and atomic mass units of common PAHs (Wenzl, Simon et al., Citation2006)
Ring linkage patterns in PAHs may occur such that the tertiary carbon atoms are centres of two or three interlinked rings, as in the linear kata-annelated PAH anthracene or the pericondensed PAHpyrene. However, most PAHs occur as hybrids encompassing various structural components, such as naphthalene, acenaphthylene, acenaphthene, fluorine, phenanthrene, anthracene, fluoranthene, pyrene, benz(a)anthracene, chrysene, benzo(b)fluoranthene, benzo(k)fluoranthene, benzo(a)pyrene, benzo(ghi)perylene dibenz(a,h)anthracene and indeno(1,2,3-cd)pyrene. Generally, an increase in the size and angularity of a PAH molecule results in a concomitant increase in hydrophobicity and electrochemical stability (Zander Citation1983, Harvey Citation1997). PAHs are harmful persistent organic pollutants, while the high-molecular-weight (HMW) PAHs are even more detrimental to the environment and human health. The two primary factors which contribute to the persistence of HMW PAHs in the environment are PAHs molecule stability and hydrophobicity. Ring linkage patterns in PAHs may occur such that the tertiary carbon atoms are centres of two or three interlinked rings, as in the linear kata-annelated PAH anthracene and condensed PAH pyrene. Concentrations ratios of specific pairs of PAHs are widely used for the qualitative determination of the PAHs sources. These ratios are called PAHs molecular diagnostic ratios and are commonly used for PAHs concentrations in air, soils and sediments. Some scientists have extended the use of these ratios also for sewage sludge, suggesting that calculation of these ratios by individual PAHs concentrations can be as effective as in soils or sediments. PAH diagnostic ratios may provide an important tool for the identification of PAHs pollution emission sources (Mauro Citation2004, Katsoyiannis, Terzi et al., Citation2007, Perra, Pozo et al. Citation2011, Chen, Teng et al. Citation2012, Tobiszewski and Namieśnik Citation2012, Sun and Zang Citation2013, Huang, Chernyak et al. Citation2014, Liu, Chen et al. Citation2014, Soliman, Al Ansari et al. Citation2014, Varnosfaderany, Bakhtiari et al. Citation2014, Sazakli, Siavalas et al. Citation2015, Di Vaio, Cocozziello et al. Citation2016, Dudhagara, Rajpara et al. Citation2016, He, Meng et al. Citation2016, Hu, Liu et al. Citation2016, Zheng, Xing et al. Citation2016). The application of PAH diagnostic ratios have been used by environmental researchers for identification of PAHs sources. PAH diagnostic ratios have been reviewed, criticised, commented on their use and many researchers have specified their limitations (Mauro Citation2004, Galarneau, Makar et al. Citation2014, Yunker, Macdonald et al. Citation2015) (Commendatore, Nievas et al. Citation2012, Tobiszewski and Namieśnik, Citation2012, Liu, Chen et al., Citation2014, Clément, Muresan et al. Citation2015, Johnston, Lineman et al. Citation2015, Khatisashvili, Matchavariani et al. Citation2015). Katsoyiannis, Terzi et al. (Citation2007) described the reasons why the PAH molecular ratios calculated from sewage sludge concentrations should not be used for the understanding of the PAH sources. Tobiszewski (Tobiszewski and Namieśnik, Citation2012) stated how PAH diagnostic ratios was commonly used as a tool for identifying and assessing pollution emission sources. Some diagnostic ratios are based on parent PAHs, others on the proportions of alkyl-substituted to non-substituted molecules. The ratios are applicable to PAHs determined in different environmental media: air (gas + particle phase), water, sediment, soil, as well as biomonitor organisms such as leaves or coniferous needles, and mussels. PAH diagnostic ratios distinguish PAHs pollution originating from petroleum products, petroleum combustion and biomass or coal burning Table . The compounds involved in each ratio have the same molar mass, so it is assumed they have similar physicochemical properties. Numerous studies show that diagnostic ratios change in value to different extents during phase transfers and environmental degradation. Ratio calculations usually are restricted to PAHs within a given molecular mass (McVeety and Hites Citation1988). The chemical structures of some commonly studied PAHs which are among the16 EPA PAHs are shown in Figure and their symbols in Table .
Table 2. Some PAH ratios (Veyrand, Brosseaud et al. 2007, Tobiszewski and Namieśnik, Citation2012)
Figure 1. Chemical structures of some commonly studied PAH(Haritash and Kaushik Citation2009).
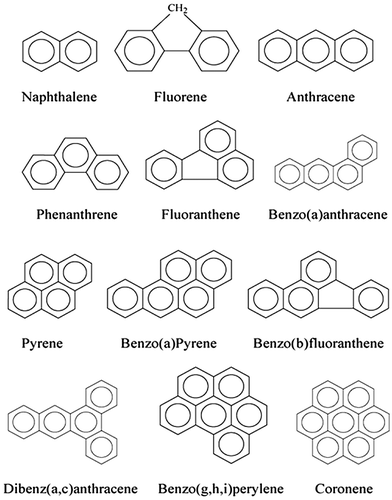
1.1. Human exposure to PAHs
The major route of exposure to PAHs in the general population is from breathing ambient indoor air, eating food containing PAHs, smoking cigarettes, or breathing smoke from open fireplaces, from the fossil fuels that we use to drive our cars, cook our food (Zhang, Cui et al. Citation2015), warm our home, and fuel our industry. PAHs in agricultural crop leaves, contributes to the exposure of organisms to these chemicals through the dietary pathway(Sun, Wang et al. Citation2016). Occupational exposure of workers to PAHs, e.g., during coke production, roofing using bituminous products, oil refining, and coal gasification. Occupational exposure to PAHs may also occur from workers breathing exhaust fumes such as mechanics, street vendors, or motor vehicle and those involved in mining, metal working. The major route of exposure is consumption of food and for smokers the contribution from smoking can be great. Food can be contaminated from environmental sources (natural and mostly anthropogenic), from industrial food processing, and from some domestic cooking practices. PAHs can enter the food chain by deposition from air, or by deposition and transfer from soil and water (Harvey Citation1997).
Human exposure to PAHs also occurs through inhalation of cigarette smoke, exposure to industrial emissions, automobile exhausts, hazardous waste sites, jet fuel, and burn pits, and consumption of barbecued food. Human beings are also exposed to PAHs via air, water and contaminated food they ingest. PAHs are frequently measured in the atmosphere for air quality assessment, in biological tissues for health-effects monitoring, in sediments and molluscs for environmental monitoring, and in foodstuffs for safety reasons. Exclusive sources for Benzol(a)pyrene (BaP) contamination of the environment and exposure to humans include industrial and automobile emissions, hazardous waste sites, cigarette smoke, biomass burning, waste burning, municipal incinerators, volcanic eruptions, home heating and consumption of charcoal broiled and smoked food.
Other exposure comes from tobacco smoke (Castro, Slezakova et al. Citation2011, Zhong, Carmella et al. Citation2011, Appenzeller, Mathon et al. Citation2012, Gao, Du et al. Citation2015, Orisakwe, Igweze et al. Citation2015), Vehicular traffic (Dubowsky, Wallace et al. Citation1999, Kingham, Briggs et al. Citation2000, Hu, Bai et al. Citation2007, Agarwal Citation2009, Slezakova, Castro et al. Citation2013, Agarwal, Bothra et al. Citation2014, Nascimbene, Tretiach et al. Citation2014, Patil, Chetlapally et al. Citation2014, Scheurer, Danysh et al. Citation2014, Gong, Zhu et al. Citation2015, Liu, Gao et al. Citation2015, Cui, Chen et al. Citation2016, Mordukhovich, Beyea et al. Citation2016), e-waste and medical waste (Tang, Shen et al. Citation2010, Wang, Tian et al. Citation2012, Chen, Zhao et al. Citation2013, Feldt, Fobil et al. Citation2014, Liu, Liao et al. Citation2014, Leung, Cheung et al. Citation2015, Luo, Bao et al. Citation2015), charcoals (Nguyen, Hlangothi et al. Citation2013), landfill (Melnyk, Dettlaff et al. Citation2015), wild land fire (Adetona, Simpson et al. Citation2015, Ahad, Jautzy et al. Citation2015), decorative candles (Orecchio Citation2011) oil spills (Lindén and Pålsson Citation2013) (Tansel, Lee et al. Citation2013, Fritt-Rasmussen, Wegeberg et al. Citation2015). PAHs are widespread environmental pollutants, so widespread that it is impossible for anyone to avoid exposure to them. Routes of exposure include ingestion, inhalation, and dermal contact in both occupational and non-occupational settings (Ravindra K, Sokhi R et al. Citation2008). Some exposures may simultaneously involve multiple routes such as dermal and inhalation exposures from contaminated air, affecting the total dose of absorption.
1.2. Effect of PAHs exposure
Exposures from various occupations and high levels of pollutant mixtures containing PAHs are known to result in symptoms such as eye irritation, nausea, vomiting and diaorrhea. Mixtures of PAHs are also known to cause skin irritation and inflammation. Anthracene, benzo(a)pyrene, and naphthalene are direct skin irritants while anthracene and benzo(a)pyrene are reported to be skin sensitizers, i.e. as cause of an allergic skin response in animals and humans (Unwin J, Cocker J et al. Citation2006). A series of health problems (an increased risk of skin, lung, bladder, and gastrointestinal cancers) for workers exposed to mixtures of PAHs and other work place exposure of PAH chemicals have been reported (Armstrong BG, Hutchinson E et al. Citation2004) (Boffetta P, Jourenkova N et al. 1997, Bach PB, Kelley MJ et al. Citation2003, Olsson AC, Fevotte J et al. Citation2010, Diggs DL, Huderson AC et al. Citation2011), PAH eg BaP and Pyrene has been identified as the cause of cancer in laboratory animals. Repeated skin contact to the PAH naphthalene can result in redness and inflammation of the skin (K. 2007). DNA damage induced by PAH exposure has been demonstrated by numerous authors (Gunter MJ, Divi RL et al., Kuo CY, Hsu YW et al. Citation2003, John K, Ragavan N et al. Citation2009, Garcia-Suastegui WA, Huerta-Chagoya A et al. Citation2011). Long-term exposure to PAHs is suspected to raise the risks of cell damage via gene mutation and cardiopulmonary mortality. Figure shows effects of long-term and short-term exposure of PAHs on human.
1.3. Sources
PAHs have natural as well as anthropogenic sources: coal and wood burning (Li, Ma et al. Citation2006, Stumpe-Viksna, Bartkevičs et al. Citation2008, Orecchio Citation2011, Barreca, Bastone et al. Citation2014, Adetona, Simpson et al., Citation2015, Bruns, Krapf et al. Citation2015, Nordin, Uski et al. Citation2015, Pozo, Estellano et al. Citation2015, Fernando, Shaw et al. Citation2016, Orecchio, Amorello et al. Citation2016, p. 32)], petrol and diesel oil combustion (Martins, Bícego et al. Citation2010, Martins, Bícego et al. Citation2011, Sun, Zhang et al. 2016) and industrial processes (Sunner, Gahm et al. Citation1988, Mastral, Callén et al. Citation2002, Wang, Miao et al. Citation2013, Eriksson, Nordin et al. Citation2014, Ren, Wu et al. Citation2014).
PAHs can be formed naturally and many of them are recalcitrant and have toxic properties (Haritash and Kaushik Citation2009, Na, Liu et al. Citation2011, Hanedar, Alp et al. Citation2014, Kamal, Cincinelli et al. Citation2015, Li, Huijbregts et al. Citation2015, Wang, Dao et al. Citation2015). Forest fires (Baxter, Hoffman et al. Citation2014, Choi Citation2014, Obrist, Zielinska et al. Citation2015, Wang, Thai et al. Citation2016), waste burning (Ortun, tild et al. Citation2014, Pongpiachan Citation2015), volcanoes and hydrothermal processes are natural emission sources of PAHs (Chrysikou, Gemenetzis et al. Citation2008, Choi, Citation2014, Domingos, Bulbovas et al. Citation2015, Kong, Li et al. Citation2015). Anthropogenic and natural sources of PAHs in combination with global transport phenomena contributed to their worldwide distribution. They are formed mainly as result of the burning of fossil fuels during heating processes, waste incinerators and from automobile exhausts. They are widely distributed environmental contaminants that have detrimental biological effects, toxicity, mutagenicity and carcinogenicity. PAH concentrations in the environment vary widely, depending on the proximity of the contaminated site to the production source, the level of industrial development, and the mode(s) of PAH transport. PAHs are present in high concentrations in products of fossil fuel, vehicular trafic émission and refining (Marr, Kirchstetter et al. Citation1999, Wickramasinghe, Karunaratne et al. Citation2011, Argyropoulos, Christolis et al. Citation2012, Slezakova, Castro et al., Citation2013, Fan, Li et al. Citation2014, Atagana Citation2015, Bayat, Hashemi et al. Citation2015, Pham, Tang et al. Citation2015, Singh and Gupta Citation2016), wood (Guillen, Sopelana et al. Citation2000, Bhargava, Dlugogorski et al. Citation2002, Stumpe-Viksna, Bartkevičs et al., Citation2008, Bruns, Krapf et al., Citation2015, Nordin, Uski et al., Citation2015, Orecchio, Amorello et al., Citation2016), biomass burning (Luo, Gao et al. Citation2016, Saxena, Sharma et al. Citation2016),charcoals (Nguyen, Hlangothi et al., Citation2013), tobacco (Castro, Slezakova et al., Citation2011, Appenzeller, Mathon et al., Citation2012, Orisakwe, Igweze et al., Citation2015),wood smoke(Bhargava, Dlugogorski et al., Citation2002, Bruns, Krapf et al., Citation2015, Pozo, Estellano et al. Citation2015, Fernando, Shaw et al., Citation2016) and garbage (Marr, Dzepina et al. Citation2006, Fadiel, Epperson et al. Citation2013). Petroleum refining (Caruso, Zhang et al. Citation2015, Wang, Wang et al. Citation2015, Oyo-Ita, Oyo-Ita et al. Citation2016) and transport activities (Fan, Yang et al. Citation2010, Li, Xue et al. Citation2015, Li, Wang et al. 2015, Mulder, Heil et al. Citation2015) are major contributors to localised loadings of PAHs into the environment. Loadings may occur through discharge of industrial effluents and through accidental release of raw and refined products. However, PAHs released into the environment may originate from many other sources; including gasoline (An, Pei et al. Citation2016, Hallare, Bolinao et al. Citation2016, Wang, Rong et al. Citation2016) and diesel (Chiang, Lai et al. Citation2012, Bangia, Symanski et al. Citation2015, Vieira de Souza and Corrêa Citation2015, Vojtisek-Lom, Pechout et al. Citation2015, Zhang and Balasubramanian Citation2016) fuel combustion and tobacco smoke (Appenzeller, Mathon et al., Citation2012, Wilk, Waligórski et al. Citation2013, CitationGao, Du et al. 2015). PAHs are detected in air (Mastral, Callén et al. Citation1998, Mastral, Callén et al. Citation1999, Nøst, Halse et al. Citation2015), soil and sediment (Blumer, Blumer et al. Citation1977, Baltrons, López-Mesas et al. Citation2013, Albanese, Fontaine et al. Citation2014, Adam, Miltner et al. Citation2015, Anyanwu and Semple Citation2015), surface water, groundwater, and road runoff (Bentzen and Larsen Citation2009, Tai, Zhou et al. Citation2009, Zheng, Luo et al. Citation2012, Luo, Zheng et al. Citation2013, Mahler, Van Metre et al. Citation2014, Nekhavhambe, van Ree et al. Citation2014, Sakulthaew, Comfort et al. Citation2014, Azah, Kim et al. Citation2015, Sakulthaew, Comfort et al. Citation2015, Hijosa-Valsero, Bécares et al. Citation2016). PAHs are dispersed from the atmosphere to vegetation (Simonich and Hites 1994, Simonich and Hites 1994, Simonich and Hites Citation1995, Wagrowski and R.A Citation1997, Nadal, Schuhmacher et al. Citation2004, Chrysikou, Gemenetzis et al., Citation2008, Wang, Tian et al., Citation2012, Noth, Katharine Hammond et al. 2013, Terzaghi, Scacchi et al. Citation2015), medicinal herbs (Tripathy, Basak et al. Citation2015) and contaminated foods (Guillén, Sopelana et al. Citation1997, Nieva-Cano, Rubio-Barroso et al. Citation2001, Fontcuberta, Arqués et al. Citation2006, Wenzl, Simon et al. Citation2006, Plaza-Bolaños, Frenich et al. Citation2010, Alomirah, Al-Zenki et al. Citation2011, Duedahl-Olesen Citation2013, Jimenez, Adisa et al. Citation2014, Rose, Holland et al. Citation2015). In soil and sediment PAH concentrations at contaminated and uncontaminated sites ranging from 1 mg/kg to over 300 g/kg have been reported (Chrysikou, Gemenetzis et al., Citation2008, de la Rosa, Paneque et al. Citation2015, Duan, Shen et al. Citation2015).
1.4. Extraction
Generally, sample pre-treatment comprises: (1) solvent extraction of PAHs from filters and sorbents; (2) extract concentration; (3) some form of liquid, or solid, chromatography clean-up; (4) eluent concentration; and (5) injection into a gas chromatography (GC) for analysis.
PAHs in air (Szulejko, Kim et al. Citation2014),water (Zhou and Gao Citation2014), food, soil and waste sludge are usually extracted into suitable conventional organic solvent before their introduction into separating or analytical equipments. Analysis by GC, high performance thin layer chromatography (HPTLC) and Fourier transform infrared spectroscopy (FTIR) (Das, Mukherjee et al. Citation2008) showed that the biosurfactant could effectively entrap and solubilize PAH. Water is an alternative solvent and reaction medium to many conventional organic solvents. Other alternative extraction agents that have been studied, including surfactants, biosurfactants, microemulsions, natural surfactants, cyclodextrins, vegetable oil and solution with solid phase particles. These extraction agents have been found to remove PAHs from soil at percentages ranging from 47 to 100% for various PAHs (Lau, Gan et al. Citation2014).
Wilson, Hewitt et al. (Wilson, Hewitt et al. Citation2014) extracted sixteen environmental protection agency-polycyclic aromatic hydrocarbons via solid-phase nanoextraction-gas chromatography/mass spectrometry. Xu, Zhao et al. (CitationXu, Zhao et al. 2014( determined PAHs in environmental water based on magnetite nanoparticles/polypyrrole magnetic solid-phase extraction.
Zhang, Zang et al. (Zhang, Zang et al. Citation2014) used polydimethylsiloxane/metal-organic frameworks coated fibre for solid-phase micro extraction of polycyclic aromatic hydrocarbons in river and lake water samples. Corrotea, Sánchez et al. (Corrotea, Sánchez et al. Citation2014) extracted PAH from water samples into a rotating-disk microextractor and the subsequent determination by gas chromatography-mass spectrometry.
Shamsipur, Gholivand et al. (Shamsipur, Gholivand et al. Citation2015) prepared and evaluated a novel solid-phase microextraction fibre based on functionalized nanoporous silica coating for extraction of PAHs from water samples followed by GC–MS detection. Messer and Taylor (Citation1995)developed a method for the quantitation of trace PAHs from water via solid-phase extraction with supercritical fluid elution. Saitoh, Matsushima et al. (2005) Concentrated PAHs in water to sodium dodecyl sulphate-[gamma]-alumina admicelle. Zhou and Gao Kronholm, Desbands et al. (Citation2002), Zhou and Gao (Citation2014) determined PAHs in water samples by temperature-controlled ionic liquid dispersive liquid-liquid microextraction combined with high performance liquid chromatography (HPLC).
Other extraction methods followed by GC-MS, HPLC-FLD or other analytical instrument include: Supercritical carbon dioxide solvent extraction (Laitinen, Michaux et al. Citation1994), thermal extraction coupled with GC/TOF/MS (Liu, Heltsley et al. Citation2002, sonication extraction (Nieva-Cano, Rubio-Barroso et al., Citation2001), supercritical fluids extraction (Amezcua-Allieri, Ávila-Chávez et al. Citation2012), Hot water extraction (Dadkhah and Akgerman Citation2006), superheated water extraction(Khanjari, Eikani et al. Citation2016),subcritical water extraction (Islam, Jo et al. Citation2013), solid-phase extraction (Kayali-Sayadi Citation1998, Xu, Tang et al. Citation2015, Sørensen, Silva et al. Citation2016, Zheng, Ding et al. Citation2016), suspended droplet micro extraction (Pena-Pereira, Costas-Mora et al. Citation2012), Supercritical fluid extraction (Schlemitz and Pfannhauser, Schlemitz and Pfannhauser Citation1997), sorptive extraction (Mollahosseini, Rokue et al. Citation2016), magnetic solid-phase extraction(Shi, Wu et al. Citation2016) Ultrasound-Assisted Liquid–Liquid Extraction (Taghvaee, Piravivanak et al. Citation2015, CitationGuo, Tan et al. 2016), headspace solid-phase microextraction (Vichi, Pizzale et al., Citation2005), Soxhlet extraction Tanabe, Suzuki et al. 2000), Tenax extraction (Hong, Yim et al. Citation2016), ultrasound-assisted emulsification microextraction (Cacho, Campillo et al. Citation2015), solid phase extraction with Tenax-TA and two non-exhaustive/mild-solvent extraction techniques (Oleszczuk Citation2009) and Stir bar sorptive extraction (Zuin, Montero et al. Citation2005), Tenax extraction(Hong, Yim et al. Citation2016), ultrasonic centrifuge solid-phase extraction (Khan, Latif et al. Citation2015, Hashemi, Ebrahimi et al. Citation2016), dispersive liquid-liquid microextraction(Sadeghi, Kobarfard et al. Citation2016) and in-tube solid-phase microextraction (SPME)(Feng, Sun et al. Citation2016). Recent developments in these areas include: cold-fibre solid-phase micro extraction of PAH extracts; the “Quick, Easy, Cheap, Effective, Rugged, and Safe” (QuEChERS) extraction technique; and, using vehicle-engine air-intake filters to collect airborne particulate matter.
1.5. Analytical methods
Samples are usually extracted and “cleaned up” with organic solvents and analysed for PAHs content by chromatographic techniques coupled with variety of sensitive detectors. Ultraviolet absorption and fluorescence spectroscopy are the most frequently used detectors in HPLC systems, while flame ionization, electron capture and nitrogen detectors and mass spectrometers have been coupled to GLC instruments. Some of these measurement methods are very sensitive and can identify and quantify individual PAH compounds, however, information on exposure is usually available only several days after sampling, instrumentation is very expensive and sample workup is manpower-intensive and therefore very costly. Although this approach offers both good sensitivity and good quality data, the results can rarely be used to provide rapid response to potentially harmful exposures. For PAHs, there is currently an unsatisfied requirement for methods that can accurately estimate exposures in real-time or at least within minutes of an exposure. Indeed, this has been the thrust of some research in the past 10 years and has led to the development of some new techniques of direct importance to the monitoring of toxic compounds in the working environment. The most promising of the new techniques (some listed in Table ) use the principle of synchronous luminescence, room temperature phosphorescence, and ultraviolet absorption. These instrumental techniques are very sensitive, ensuring detection of very low concentrations and, in addition, may provide information on sample PAHs content.
Table 3. Common analytical methods used in analysis of PAHs
2. PAH in Air
PAHs in the ambient air exist in vapour phase or adsorb into airborne particulate matter depending on the atmospheric conditions (ambient temperature, relative humidity, etc.), the nature (i.e., origin and properties) of the aerosol, and the properties of the individual PAH (Ravindra K, Sokhi R et al., Citation2008).
PAHs are complex hazardous organic compounds that are introduced into the atmosphere as by-products of partial combustion processes. Atmospheric emissions of airborne PAHs can originate from heat and power generation (e.g. coal, gas, wood, oil, charcoals), industrial processes (e.g., coke production) (Liberti, Notarnicola et al. Citation2006, Ivan, Hudák et al. Citation2011, Li, Feng et al. Citation2012, CitationDeng, Dai et al. 2014, Talaska, Thoroman et al. Citation2014, Kozielska and Konieczyński Citation2015, Xu, Peng et al. Citation2016), refuse burning and vehicle emissions (Chiang, Lai et al., Citation2012, Li, Lang et al. 2014, Liu, Gao et al. Citation2015, Wei, Guangbin et al. Citation2015, Bayraktar, Paloluoğlu et al. Citation2016, Bi, Luo et al. Citation2016) as shown in Figure . Human exposure to airborne PAHs can result from emissions from other sources, such as cooking, smoking(White, Bradshaw et al. Citation2016), incense, mosquito coil (Verma, Patel et al. Citation2015) and materials containing PAH (e.g., petroleum products, synthetic fuels). Urban road dusts are carriers of polycyclic aromatic compounds (PACs) and are therefore considered to be a major source of contamination of other environmental compartments and a source of exposure to PACs for urban populations. Street dust is an indicator of PAHs pollution status of a city area (Hussain, Rahman et al. Citation2015, Bandowe and Nkansah Citation2016). PAH exposure from solid fuel burning represents an important public health issue for the majority of the global population. Yet, understanding of individual-level exposures remains limited. Indoor air pollution from use of indoor stoves and/or fireplaces is an important source of ambient PAH exposure (Ruokojärvi, Ruuskanen et al. Citation1995, Ruokojärvi, Aatamila et al. Citation2000, Celeiro, Lamas et al. Citation2014, Patelarou and Kelly Citation2014, Shen, Zhang et al. Citation2014, Han, Bai et al. Citation2015, Hassanvand, Naddafi et al. Citation2015, Kong, Li et al., Citation2015, Mugica-Alvarez, Santiago-de la Rosa et al. Citation2015, White, Teitelbaum et al. Citation2015, Verma, Patel et al. Citation2016).
Vehicular traffic is a major source of outdoor air pollution such as PAHs in urban areas, and studies have shown that air pollution is worse during hours of commuting to and from work and school. However, it is unclear to what extent different commuting behaviors are a source of air pollution compared to non-commuters, and if air pollution exposure actually differs by the mode of commuting (Pham, Kameda et al. Citation2013, Patil, Chetlapally et al., Citation2014, Xu, Guo et al. Citation2014, Liu, Gao et al. Citation2015, Pham, Tang et al., Citation2015, Sarigiannis, Karakitsios et al. Citation2015, Afshar-Mohajer, Wilson et al. Citation2016, Chen, Chen et al. Citation2016, Mordukhovich, Beyea et al., Citation2016, Zhai, Li et al. Citation2016). Parkıng Garages is another source of PAHs emission in urban area(Kara and Aydin Citation2015) . Kitchen is also a main source of PAHs emission and urinary PAH metabolites in kitchen workers can be associated with higher concentrations of PAHs in kitchen indoor air (Enault, Robert et al. Citation2015, Gao, Jian et al. Citation2015, Singh, Chandrasekharan Nair et al. Citation2016). Indoor residential carpet dust, indoor residential dust and road /street dust are also major sources of PAHs (Qi, Li et al. Citation2014, Azah, Kim et al., Citation2015, Clément, Muresan et al., Citation2015, Hussain, Rahman et al. Citation2015, Kumar, Kothiyal et al. Citation2015, Soltani, Keshavarzi et al. Citation2015, Yang, Li et al. Citation2015, Chen, Zhang et al. Citation2016, DellaValle, Deziel et al. Citation2016, Li, Han et al. Citation2016, Nagpure, Gurjar et al. Citation2016, Wang, Wang et al. Citation2016).
PAHs are detected in air and monitoring of atmospheric organic pollutants (i.e PAHs) is usually conducted by means of active or passive air samplers (Bartkow, Huckins et al. Citation2004, Bartkow, Kennedy et al. Citation2006, Komarova, Bartkow et al. 2006, Choi, Baek et al. Citation2007, Blahová, Schandlová et al. Citation2011, Yates, Pollard et al. Citation2011, Apostolopoulou, Monteyne et al. Citation2014, Apostolopoulou, Monteyne et al., Citation2014, Jin, Cong et al. Citation2014, Jariyasopit, Liu et al. Citation2015, Loppi, Pozo et al. Citation2015, Terzaghi, Scacchi et al., Citation2015, Domínguez-Morueco, Schuhmacher et al. Citation2016, Parnis, Eng et al. Citation2016).
Alboquegi et al.(Albuquerque, Coutinho et al. Citation2016) reported the analytical results of PAHs concentrations in ambient air obtained in the PM10 and gas-phase, from 2004 to 2014, in Porto, Portugal. As part of a monitoring programme conducted by IDAD — Institute of Environment and Development and supported by the regional municipal solid waste (MSW) management authorities, an extensive database of PAH concentrations in ambient air was collected in Porto’s metropolitan area. During this period a total of 201 samples were collected in two sites classified as suburban. Analytical results showed a clear decreasing trend of total PAHs (∑ PAH) and benzo[a]pyrene (BaP) levels during the period of study, especially in the first years of monitoring. The average annual concentrations of BaP were, throughout the monitoring period, lower than the target value for the annual average (1 ng m−3) defined in the European legislation. PAHs levels showed a strong seasonality, with higher concentrations values during the colder months. The winter/summer ratio of ∑ PAH for the eleven years of study was 5, revealing the seasonal variation of PAHs in the studied area. The estimated toxic equivalency factors (TEFs) used to assess the contribution of the carcinogenic potential, confirmed a significant presence of the moderately active carcinogenic BaP and dibenz[ah]anthracene (DahA) in the samples collected in Porto. The ratio values of individual PAHs concentrations were used as diagnostic tool to identify the possible origin of PAH in the ambient air of Porto. Based on diagnostic ratios, it may be concluded that automobile traffic emissions, mainly related to diesel vehicles, were the major contribution of PAH levels in the ambient air, although some others contributions, such as coal and wood combustion, were identified.
Romagnoli et al.(Romagnoli, Balducci et al. Citation2014) monitored Indoor and outdoor concentrations of PAHs associated with PM2.5 particles in three microenvironments (schools, homes and offices) in the city of Rome, Italy, between winter 2011 and summer 2012. Molecular signatures and indoor/outdoor concentration ratios of PAHs were investigated, with special emphasis on carcinogenic congeners. At indoor locations, total PAHs ranged, on average, from 1.8 to 8.4 ng/m3 in winter and from 0.30 to 1.35 ng/m3 in spring/summer. Outdoors, total PAH concentrations were found to reach 6.3–17.9 ng/m3 in winter and 0.42–1.74 ng/m3 in spring-summer. Indoors, the concentration of benzo[a]pyrene (BaP) was as high as 1.1 ng/m3 in winter and below 0.1 ng/m3 in the warm season, independently of site type; the yearly average remained below the European guideline value. The indoor/outdoor concentration ratios of individual compounds were lower than one for most of congeners, suggesting that outdoor sources were predominant. Nonetheless, the percentages of PAH compounds changed with sites and seasons; in particular, in spring/summer, the concentration of BaP at our sites was more than twice that recorded at the regional network stations.
Jin et al. (Jin, Cong et al. Citation2014) developed a simple, conventional, economic and fast sampling method for volatile and semi volatile compounds that is based on a liquid-phase organic solvent. Laboratory tests showed the feasibility of the method, and the major parameters of the method, such as temperature, organic solvent, carrier gas and air flow rate, were preliminarily optimized. For 16 kinds PAHs, the recovery, relative standard deviation and detection limit were ranged from 87.0 to 104.4%, 2.6 to 15.6% and 0.08 to 0.45 ng mL−1, respectively. Field application of the new method also showed good sampling efficiency for PAHs in real air samples, comparable to the results with an XAD-2 resin-based method. The similarity was closed to 1, suggesting that the new method is suitable for sampling of volatile and semi volatile organic pollutants in air and has great potential for air-pollution monitoring. 29 parent- and alkyl-polycyclic aromatic hydrocarbons (PAHs), 15 oxygenated-PAHs (OPAHs), 11 nitrated-PAHs (NPAHs) and 4 azaarenes (AZAs) in both the gaseous and particulate phases, as well as the particulate-bound carbon fractions (organic carbon, elemental carbon, char, and soot) in ambient air sampled in March and September 2012 from an urban site in Xi’an, central China were extracted and analysed. The average concentrations (gaseous + particulate) of ∑ 29PAHs, ∑ 15OPAHs, ∑ 11NPAHs and ∑ 4AZAs were 1267.0 ± 307.5, 113.8 ± 46.1, 11.8 ± 4.8 and 26.5 ± 11.8 ng m−3 in March and 784.7 ± 165.1, 67.2 ± 9.8, 9.0 ± 1.5 and 21.6 ± 5.1 ng m−3 in September, respectively. Concentrations of ∑ 29PAHs, ∑ 15OPAHs and ∑ 11NPAHs in particulates were significantly correlated with those of the carbon fractions (OC, EC, char and soot). Both absorption into organic matter in particles and adsorption onto the surface of particles were important for PAHs and OPAHs in both sampling periods, with more absorption occurring in September, while absorption was always the most important process for NPAHs. The total carcinogenic risk of PAHs plus the NPAHs was higher in March. Gaseous compounds, which were not considered in most previous studies, contributed 29 to 44% of the total health risk in March and September, respectively.
Choi et al. (Choi, Zdeb et al. Citation2015) developed regionally adaptable chronic personal exposure model to pro-carcinogenic PAH (c-PAH) for the population in Kraków, Poland. They checked the assumption of spatial uniformity in eight c-PAH using the coefficients of divergence (COD), a marker of absolute concentration differences. Upon successful validation, they developed personal exposure models for eight pro-carcinogenic PAH by integrating individual-level data with area-level meteorological or pollutant data. They checked the resulting model for accuracy and precision against home outdoor monitoring data. Results: During winter, COD of 0.1 for Kraków suggest overall spatial uniformity in the ambient concentration of the eight c-PAH. The three models that were developed were associated with index of agreement approximately equal to 0.9, root mean square error<2.6ng/m3, and 90th percentile of absolute difference ≤4ng/m3 for the predicted and the observed concentrations for eight pro-carcinogenic PAH. They concluded that inexpensive and logistically feasible information could be used to estimate chronic personal exposure to PAH profiles, in lieu of costly and labour-intensive personal air monitoring at wide scale. At the same time, thorough validation through direct personal monitoring and assumption checking are critical for successful model development.
Ahmed. (Ahmed, Ahmed et al. Citation2015) reported concentrations of 43 PAHs and 4 oxygenated PAHs (OPAHs) for the first time in particulate matter (PM) sampled in the air of the city of Sulaimaniyah in Iraq. The total PAH concentration at the different sampling sites varied between 9.3 and 114ng/m3. The corresponding values of the human carcinogen benzo[a]pyrene were between 0.3 and 6.9ng/m3, with most samples exceeding the EU annual target value of 1ng/m3. The highly carcinogenic dibenzopyrene isomers dibenzo[a,l]pyrene, dibenzo[a,e]pyrene, dibenzo[a,i]pyrene and dibenzo[a,h]pyrene constituted 0.1-0.4% of the total PAH concentration. However, when scaling for relative cancer potencies using toxic equivalency factors, a benzo[a]pyrene equivalent concentration of dibenzo[a,l]pyrene equal to that of benzo[a]pyrene was obtained, indicating that the contribution of dibenzo[a,l]pyrene to the carcinogenicity of the PAHs could be similar to that of benzo[a]pyrene. A high correlation between the determined concentrations of the dibenzopyrene isomers and benzo[a]pyrene was found, which supported the use of benzo[a]pyrene as an indicator for the carcinogenicity of PAHs in ambient air. The total concentrations of the four OPAHs, 9, 10-anthraquinone, 4H-cyclopenta[def]phenanthren-4-one, benzanthrone, and 7,12-benz[a]anthraquinone, varied between 0.6 and 8.1ng/m3, with 9,10-anthraquinone being the most abundant OPAH in all of the samples.
Szulejko et al. (Szulejko, Kim et al., Citation2014) reviewed sample-pretreatment options with the main emphasis on all aspects of solvent extraction prior to GC-based analysis of airborne PAHs. They also discussed some aspects of “green chromatography”, used in recent years to reduce solvent usage. Kauneliene et al. (Kauneliene, Krugly et al. Citation2015) determined Spatial and temporal variation of vapour and particle-phase PAHs in six urban and sub-urban locations in Kaunas, Lithuania during heating and non-heating seasons. Two different sampling methodologies were used: passive (based on semipermeable membrane device, SPMD) and active (based on collection on filter and sorption). Sixteen priority PAHs as well as methylated PAHs were quantified in the collected samples. The sampled total amount of 16 PAHs investigated in SPMDs ranged from 10–138 ng/day outdoors, from 5–59 ng/day indoors during the winter sampling campaign. In summer these amounts varied from 11–19 ng/day outdoors and from 19–27 ng/day indoors. The total concentrations of vapor and particle-phase PAH in winter in actively taken samples varied from 49–286 ng/m3 outdoors and from 28–83 ng/m3 indoors. Seasonal differences as well as the influence of the fuel burning for domestic heating purposes on the PAH concentration outdoors were well reflected by the data obtained using the SPMD methodology.
Wei et al. (Wei, Bandowe et al. Citation2015) extracted and analysed 29 parent- and alkyl-PAHs, 15 oxygenated-PAHs (OPAHs), 11 nitrated-PAHs (NPAHs) and 4 azaarenes (AZAs) in both the gaseous and particulate phases, as well as the particulate-bound carbon fractions (organic carbon, elemental carbon, char, and soot) in ambient air sampled in March and September 2012 from an urban site in Xi’an, central China. The average concentrations (gaseous + particulate) of ∑ 29PAHs, ∑ 15OPAHs, ∑ 11NPAHs and ∑ 4AZAs were 1267.0 ± 307.5, 113.8 ± 46.1, 11.8 ± 4.8 and 26.5 ± 11.8 ng m−3 in March and 784.7 ± 165.1, 67.2 ± 9.8, 9.0 ± 1.5 and 21.6 ± 5.1 ng m−3 in September, respectively. Concentrations of ∑ 29PAHs, ∑ 15OPAHs and ∑ 11NPAHs in particulates were significantly correlated with those of the carbon fractions (OC, EC, char and soot). Both absorption into organic matter in particles and adsorption onto the surface of particles were important for PAHs and OPAHs in both sampling periods, with more absorption occurring in September, while absorption was always the most important process for NPAHs. The total carcinogenic risk of PAHs plus the NPAHs was higher in March. Gaseous compounds, which were not considered in most previous studies, contributed 29 to 44% of the total health risk in March and September, respectively.
Yan et al. (Yan, Zheng et al. Citation2015) examined exposure to fine and ultrafine particles as well as particulate PAHs by commuters in three transportation modes (walking, subway and bus) in December 2011 in Beijing, China. During the study period, real-time measured median PM mass concentration (PMC) for walking, riding buses and taking the subway were 26.7, 32.9 and 56.9 μg m−3, respectively, and particle number concentrations (PNC) were 1.1 × 104, 1.0 × 104 and 2.2 × 104 cm−3. Commuters were exposed to higher PNC in air-conditioned buses and aboveground-railway, but higher PMC in underground-subway compared to aboveground-railway. PNC in roadway modes (bus and walking) peaked at noon, but was lower during traffic rush hours, negatively correlated with PMC. Toxic potential of particulate-PAHs estimated based on benzo(a)pyrene toxic equivalents (BaP TEQs) showed that walking pedestrians were subjected to higher BaP TEQs than bus (2.7-fold) and subway (3.6-fold) commuters, though the highest PMC and PNC were observed in subway.
Yagisital et al. (Yagishita, Kageyama et al. Citation2015) measured the atmospheric concentrations of both gas-phase and particulate-phase PAHs including 16 US Environmental Protection Agency priority PAHs (16 PAHs) were measured in eleven cities across Japan. Using the measured average concentrations and toxic equivalency factors (TEF) of the 16 PAHs, the benzo[a]pyrene (BaP)-toxic equivalent for eight major PAHs was obtained: the ratio of BaP to the eight major PAH toxicities ranged from 0.23 to 0.47. Among the target PAHs, from the viewpoint of carcinogenicity, they focused on benzo[. c]fluorene (BcFE), which was detected in both the gas and particulate phase in contrast to BaP. The relative potency factor (RPF) of BcFE was evaluated as 6.46 based on its cancer slope factor relative to that of BaP determined in the benchmark dose calculations for mice. The relative carcinogenic risk of BcFE to BaP was obtained by multiplying the averaged concentrations of BaP and BcFE by the RPF value: the risk of BcFE was 6.8 and 5.1 times higher than that of BaP in summer and winter, respectively. These results show that the collection of atmospheric samples including the gas phase is important when assessing the carcinogenic risk of atmospheric PAHs.
White et al. (White, Teitelbaum et al., Citation2015) examined indoor air pollution from use of indoor stoves and/or fireplaces as an important source of ambient PAHs exposure. However, the association between indoor stove/fireplace use and breast cancer risk is unknown. They hypothesized that indoor stove/fireplace use in a Long Island, New York study population would be positively associated with breast cancer and differ by material burned, and the duration and timing of exposure. They also hypothesized that the association would vary by breast cancer subtype defined by p53 mutation status, and interact with glutathione S-transferases GSTM1, T1, A1 and P1 polymorphisms. Methods: Population-based, case-control resources (1,508 cases/1,556 controls) were used to conduct unconditional logistic regression to estimate adjusted odds ratios (OR) and 95% confidence intervals (CI). Results: Breast cancer risk was increased among women reporting ever burning synthetic logs (which may also contain wood) in their homes (OR = 1.42, 95% CI 1.11, 1.84), but not for ever burning wood alone (OR = 0.93, 95% CI 0.77, 1.12). For synthetic log use, longer duration >7 years, older age at exposure (>20 years; OR = 1.65, 95% CI 1.02, 2.67) and 2 or more variants in GSTM1, T1, A1 or P1 (OR = 1.71, 95% CI 1.09, 2.69) were associated with increased risk.
In Wei et al. (Wei, Guangbin et al., Citation2015)’s studies, most toxic PAHs in the vehicle exhaust were characterized and quantified carried on a chassis dynamometer test. The vehicle exhaust samples were collected under different driving cycles of vehicles such as acceleration, deceleration, uniform speed and idle conditions. The results show that the concentration of lower molecular weight PAHs (2–3 rings) in gaseous phase was higher than that in solid phase, while the concentration of high molecular weight PAHs (5–6 rings) was abundant in solid phase. The sum of two-ring, three-ring and four-ring PAHs accounted for ~87% of the total gaseous PAH concentrations, while about 10 and 4% of gaseous-phase PAHs were five rings and six rings, respectively. By comparison, the percentage of two-ring and three-ring PAHs were decreased in the solid-phase PAHs. Three-, four-, and five-ring PAHs had an absolute advantage in solid phase, which accounted for 89 and 81% of the total PAHs in diesel and gasoline vehicle exhaust, respectively. The Benzo(a)pyrene-equivalent carcinogenic power (BaPE) value of PAHs ranged from 0.558 to 5.684 μg m−3 in vehicle exhaust, and the ratio of BaPE·BaP−1 was between 2.029 and 8.413. In other words, the PAHs contributed 102.9–741.3% of the BaP equivalent carcinogenic toxicity except for sole BaP.
Patil, et al. (Patil, Chetlapally et al., Citation2014) reviewed the impact of occupational health hazards on the health of traffic police personnel. They published, accessed and reviewed research papers on traffic police reporting occupational health issue. Attempts were made to access papers that reported negative associations in order to present a balanced review. The majority of the studies have reported a decrease in the lung function and increased respiratory morbidity. The research on the cytogenetic abnormalities or genotoxic effect of vehicular emissions arising due to long-term exposure to benzene and other PAHs has provided conflicting results, since more or less equal numbers of studies have given evidence for and against the causal association. There is a vast accumulation of epidemiological evidence on the casual association between vehicular pollution and its carcinogenic effect. Multiple studies have concluded that traffic police are highly stressed. A number of occupational factors have been attributed to stress among traffic police. Occupational health studies help us to understand the effects of vehicular pollution and its adverse influence on workers. They also provide opportunity for defined exposures measurements and precise risk assessment. The findings from these studies are easily generalisable and can help us understand the impact of air pollution on the general population.
Wei C et al. (Wei, Bandowe et al., Citation2015) determined the occurrence, composition pattern and sources of several PACs (29 alkyl- and parent-PAHs, 15 oxygenated-PAHs (OPAHs), 4 azaarenes (AZAs), and 11 nitrated-PAHs (NPAHs) in twenty urban road dusts and six suburban surface soils (0-5cm) from Xi’an, central China. The average concentrations of ∑29PAHs, ∑4AZAs, ∑15O PAHs, and ∑11NPAHs were 15767, 673, 4754, and 885 ng g-1 in road dusts and 2067, 784, 854, and 118 ng g-1 in surface soils, respectively. The concentrations of most individual PACs were higher in Streets dusts than suburban soils, particularly for PACs with molecular weight>192 g mol-1. The enrichment factors of individual PACs were significantly positively correlated with log K, indicating an increasing deposition and co-sorption of the PACs in urban dusts with decreasing volatility and increasing hydrophobicity. Significant correlations between the concentrations of individual and sum of PACs, carbon fractions (soot and char), and source-characteristic PACs (combustion-derived PAHs and retene, etc.), indicated that PAHs, OPAHs and AZAs were mostly directly emitted from combustion activities and had similar post-emission fates, but NPAHs were possibly more intensely photolyzed after deposition as well as being emitted from vehicle exhaust sources. The incremental lifetime cancer risk (ILCR) resulting from exposure to urban dust bound-PACs was higher than 10-6, indicating a non-negligible cancer risk to residents of Xi’an.
Yu et al. (Yu, Yang et al. Citation2015) studied high lung cancer prevalence in females in Taiwan with a relatively low number of smokers. Exposure to air pollution from cooking (APC) has been suspected as one of the major risk factors for lung cancer since cooking is one of the major sources of particulate matter (PM) and (PAHs) in household buildings. Many studies have demonstrated that occupational exposure (40h per week) to APC in commercial kitchens and restaurants could cause a cancer risk higher than 10-6. However, currently the data of exposure to APC in household kitchens are relatively few in Taiwan. Therefore, they conducted a health risk assessment for exposure to APC in five household kitchens in northern Taiwan during 2010-2011.Twenty-four-hour monitoring of the particle concentration shows that particle number concentrations during non-cooking hours ranged from 1220 to 6200particles/cm3 and increased to 1,400,000particles/cm3 during cooking hours, and most of these particles are ultrafine ones. Some of the measurement results (PM80μg/m3 at 3 kitchens) exceed the current indoor air quality standards of Taiwan. The gas-particle partitioning of PAHs in household APC indicates that most of the low molecular PAHs (2 and 3 rings) are in the gas phase, while the high molecular PAHs (more than 4 rings) are particle-bound. The particulate-phase PAHs contribute more than 95% of the benzo[a]pyrene equivalent concentration. The incremental lifetime cancer risk resulting from exposure to the household APC could be higher than the acceptable level of 10−6, and thus of health concerns.
Terzaghi, et al.(Terzaghi, Scacchi et al., Citation2015) investigated the temporal variability (weekly) in PAH concentrations and the fingerprint of films developed on window surfaces. Moreover, films and leaves of two tree species (Acer pseudoplatanus and Cornus mas) collected at the same time were used to derive PAH air concentrations and investigated their short-term variability. In general, the most abundant chemicals found in films were phenanthrene and pyrene (22%), followed by perylene (21%) and fluoranthene (16%), but the fingerprint (in contrast to leaves and air) changed over time. Leaf derived air concentrations were within a factor of 2 to 9 from measured values, while air concentrations back-calculated from films were within a factor of 2 to 53. This happened because predicted air concentrations using films and vegetation samplers (especially for low K chemicals) generally reflect only the last few hours (due to the fast equilibrium) of the weekly integrated samples obtained employing the high-volume sampler. This means that films and leaves can be usefully employed for predicting the short-term variability of low K organic contaminant air concentrations.
Paulik et al. (Paulik, Donald et al. Citation2015) developed and deployed passive air samplers in a rural community heavily affected by the natural gas boom. Samplers were analysed for 62 PAHs. Results were grouped based on distance from each sampler to the nearest active well. PAH levels were highest when samplers were closest to active wells. Additionally, PAH levels closest to natural gas activity were an order of magnitude higher than levels previously reported in rural areas. Sourcing ratios indicate that PAHs were predominantly petrogenic, suggesting that elevated PAH levels were influenced by direct releases from the earth. Quantitative human health risk assessment estimated the excess lifetime cancer risks associated with exposure to the measured PAHs. Closest to active wells, the risk estimated for maximum residential exposure was 2.9 in 10 000, which is above the U.S. EPAs acceptable risk level. Overall, risk estimates decreased 30% when comparing results from samplers closest to active wells to those farthest. This work suggested that natural gas extraction may be contributing significantly to PAHs in air, at levels that are relevant to human health.
Miao et al. (Miao, Bouchard et al. Citation2015) examined the relationships between commuting behaviors and air pollution exposure levels measured by urinary 1-OHP (1-hydroxypyrene), a biomarker of exposure to PAHs. A cross-sectional study of 174 volunteers living in Montreal, 92 females and 82 males, aged 20 to 53 years was conducted in 2011. Each participant completed a questionnaire regarding demographic factors, commuting behaviors, home and workplace addresses, and potential sources of PAH exposure, and provided a complete first morning void urine sample for 1-OHP analysis. Multivariable general linear regression models were used to examine the relationships between different types of commuting and urinary 1-OHP levels. Compared to non-commuters, commuters traveling by foot or bicycle and by car or truck had a significantly higher urinary 1-OHP concentration in urine (p. = 0.01 for foot or bicycle vs. non-commuters; p. = 0.02 for car or truck vs. non-commuters); those traveling with public transportation and combinations of two or more types of modes tended to have an increased 1-OHP level in urine (p. = 0.06 for public transportation vs. non-commuters; p. = 0.05 for commuters with combinations of two or more types of modes vs. non-commuters). No significant difference in urinary 1-OHP variation was found by mode of commuting. This preliminary study suggested that despite the mode of commuting, all types of commuting during rush hours increase exposure to air pollution as measured by a sensitive PAH metabolite biomarker, and mode of commuting did not explain exposure variation.
Verma (Verma, Patel et al., Citation2015) described the concentration and emission fluxes of PAHs emitted during burning of commonly used indoor materials, i.e., 15 fuels (i.e., biomass (BM), coal (C), cow dung (CD), kerosene (K), 4 incense (IS) and mosquito coil (MC) in Raipur district, Chhattisgarh, central India. The samples were taken in September 2013 in indoor environments and respective smoke emitted were collected using high volume United State of America (USA) air sampler on quartz fiber filters. The concentration of total 13 PAHs (∑PAH13) (i.e., phenanthrene, anthracene, fluoranthene, pyrene, benz(a)anthracene, chrysene, benzo(b)fluoranthene, benzo(k)fluoranthene, benzo(a)-pyrene, dibenz(ah)anthracene, benzo(ghi) perylene, indeno1,2,3-(cd)pyrene, and coronene) in particulate matter (PM10) in the indoor air during burning of the fuels, IS and MC materials ranged from 367–92052 ng m−3, 4089–14047 ng m−3, and 66–103 ng m−3 with mean values of 7767 ± 11809 ng m−3, 9977 ± 4137 ng m−3, and 74 ± 20 ng m−3, respectively. The mean concentration of the ∑PAH13 present in indoor environment is much higher than the WHO limit value of 1.0 ng m−3. The sources and toxicities of PAHs were discussed.
Lim et al. (Lim, Mattsson et al. Citation2015) identified Benz[j]aceanthrylene (B[j]A) a cyclopenta-fused PAH with strong mutagenic and carcinogenic effects in air PM in samples collected in Stockholm, Sweden and in Limeira, Brazil using LC-GC/MS analysis. They determined concentrations ranged between 1.57 and 12.7 and 19.6-30.2 pg/m3 in Stockholm and Limeira, respectively, which was 11-30 times less than benzo[a]pyrene (B[a]P) concentrations. Activation of the DNA damage response was evaluated after exposure to B[j]A in HepG2 cells in comparison to B[a]P. We found that significantly lower concentrations of B[j]A were needed for an effect on cell viability compared to B[a]P, and equimolar exposure resulted in significant more DNA damage with B[j]A. Additionally, levels of γH2AX, pChk1, p53, pp53, and p21 proteins were higher in response to B[j]A than B[a]P. On the basis of dose response induction of pChk1 and γH2AX, B[j]A potency was 12.5- and 33.3-fold higher than B[a]P, respectively. Although B[j]A levels in air were low, including B[j]A in the estimation of excess lifetime cancer risk increased the risk up to 2-fold depending on which potency factor for B[j]A was applied. Together, their results show that B[j]A could be an important contributor to the cancer risk of air PM.
Li et al.(Li, Wang et al. 2015) measured twelve nitro-PAHs (nPAHs) and four oxy-PAHs (oPAHs) in air samples for 12 months at 18 sites in urban settings, rural villages, or rural fields in northern China. The nPAH concentrations were higher in urban areas (1.3 ± 1.3 ng/m3), and nPAH/parent PAH ratios were higher (suggesting important contributions from motor vehicles and secondary formation) in urban sites than in rural villages. oPAHs are primarily emitted from solid fuel combustion and motor vehicles, and similar oPAH concentrations were found in urban areas (23 ± 20 ng/m3) and rural villages (29 ± 24 ng/m3). The high numbers of motor vehicles in Beijing and intensive industrial activity in Taiyuan and Dezhou caused higher nPAH concentrations. No spatial trend in oPAH concentrations was found in the rural villages, because similar oPAH mixtures are emitted from solild fuel combustion. The nPAH and oPAH concentrations were higher in the winter, and correlated with residential energy consumption and precipitation.
Kong et al. (Kong, Li et al., Citation2015) collected PM2.5 samples at a suburban site of Nanjing around 2014 Chinese Spring Festival (SF) and analysed for 18 kinds of PAHs by GC-MS. Comparison of PAH concentrations during different periods, with different air mass origins and under different pollution situations were done. Sources were analysed by diagnostics ratios and principal component analysis (PCA). The threat of PAHs was assessed by BaP equivalent concentrations (BaPeq) and incremental lifetime cancer risk (ILCR). The averaged PAHs for pre-SF, SF and after SF periods were 50.6, 17.2 and 29ngm−3, indicating the variations of PAH sources, with reduced traffic, industrial and construction activities during SF and gradually re-starting of them after-SF. According to PAH mass concentrations, their relative abundance to particles, ratio of PAHs (3-ring+4-ring)/PAHs(5-ring+6-ring), mass concentrations of combustion-derived and carcinogenic PAHs, fireworks burning is an important source for PAHs during SF. The ILCR values for Chinese New Year day were 0.68 and 3.3 per 100,000 exposed children and adults. It suggested the necessity of controlling fireworks burning during Chinese SF period which was always companied with serious regional haze pollution. PAH concentrations exhibited decreasing trend when air masses coming from the following directions as North China Plain (63.9ngm-3); Central China (53.0ngm-3); Shandong Peninsula (46.6ngm-3); Northwest China (18.8ngm-3); Sea (15.8ngm-3). For different pollution situations, they decreased as haze (44.5ngm-3); fog-haze (28.4ngm-3); clear (12.2ngm-3); fog day (9.2ng m-3). Coal combustion, traffic emission, industrial processes and petroleum (only for non-SF holiday periods) were the main sources of PM2.5 associated PAHs. Fireworks burning contributed 14.0% of PAHs during SF period. Directly measurement of PAHs from fireworks burning is urgently needed for source apportionment studies in the future.
Hsu et al. (Hsu, Harner et al. Citation2015) conducted PAHs measurements in collaboration with Wood Buffalo Environmental Association (WBEA) at four community ambient Air quality Monitoring Stations (AMS) in the Athabasca Oil Sands Region (AOSR) in Northeastern Alberta, Canada. The 2012 and 2013 mean concentrations of a subset of the 22 PAH species were 9.5, 8.4, 8.8, and 32 ng m-3 at AMS 1 (Fort McKay), AMS 6 (residential Fort McMurray), AMS 7 (downtown Fort McMurray), and AMS 14 (Anzac), respectively. The average PAH concentrations in Fort McKay and Fort McMurray were in the range of rural and semirural areas, but peak values reflect an industrial emission influence. At these stations, PAHs were generally associated with NO, NO, PM, and SO indicating the emissions were from the combustion sources such as industrial stacks, vehicles, residential heating, and forest fires, whereas the PAH concentrations at AMS 14 (35 km south of Fort McMurray) were more characteristic of urban areas with a unique pattern: eight of the lower molecular weight PAHs exhibited strong seasonality with higher levels during the warmer months. Enthalpies calculated from Clausius-Clapeyron plots for these eight PAHs suggest that atmospheric emissions were dominated by temperature-dependent processes such as volatilization at warm temperatures. These findings point to the potential importance of localized water-air and/or surface-air transfer on observed PAH concentrations in air.
Vuković et al.(Vuković, Aničić Uroševic et al. Citation2014) performed a study in four parking garages in downtown of Belgrade with the aim to provide multi-pollutant assessment. Concentrations of 16 US EPA priority PAHs were determined in PM10 samples. The carcinogenic health risk of employees’ occupational exposure to PAHs (B[a]A, Cry, B[b]F, B[k]F, B[a]P and DB[ah]A) was estimated. A possibility of using Sphagnum girgensohnii moss bags for monitoring of trace element air pollution in semi-enclosed spaces was evaluated as well. The results showed that concentrations of PM10, B[a]P exceeded the EU Directive target values. Concentration of Zn, Ba and Cu were two orders of magnitude higher than those measured at different urban sites in European cities. Cumulative cancer risk obtained for heavy metals and PAHs was 4.51×10-5 and 3.75×10-5 in M and PP, respectively; upper limit of the acceptable US EPA range is 10-4. In the moss, higher post-exposure than pre-exposure (background) element concentrations was observed. In comparison with instrumental monitoring data, similar order of abundances of the most elements in PM10 and moss samples was found. However, using of the S.girgensohnii moss bag technique in indoor environments needs further justification. Padula, A. M Background: Preterm birth is an important marker of health and has a prevalence of 12-13% in the U.S. Studies suggested that exposure to PAHs during pregnancy is related to adverse birth outcomes. The aim of thier study was to evaluate the association between exposure to PAHs during the pregnancy and preterm birth. Methods: The study population included births from year’s 2001 to 2006 of women whose maternal residence was within 20. km of the primary monitoring site in Fresno, California. Data in the Fresno area were used to form a spatio-temporal model to assign daily exposure to PAHs with 4, 5, or 6 rings at the maternal residence throughout pregnancy of all of the births in the study area. Gestational age at birth and relevant covariates were extracted from the birth certificate. Results: We found an association between PAHs during the last 6 weeks of pregnancy and birth at 20-27 weeks (OR=2.74; 95% CI: 2.24-3.34) comparing the highest quartile to the lower three. The association was consistent when each quartile was compared to the lowest (OR2nd=1.49, 95% CI: 1.08-2.06; OR3rd=2.63, 95% CI:1.93-3.59; OR4th=3.94, 95% CI:3.03-5.12). Inverse associations were also observed for exposure to PAHs during the entire pregnancy and the first trimester and birth at 28-31 weeks and 20-27 weeks. Conclusion: An association between PAH exposure during the 6 weeks before delivery and early preterm birth was observed. However, the inverse association with early preterm birth offers an unclear, and potentially complex, inference of these associations.
Wang et al.(Wang, Geng et al. Citation2014) collected and analysed for 18 kinds of PAHs in daily PM2.5 samples at a suburban site of Nanjing around 2014 Chinese Spring Festival (SF) and analysed for 18 kinds of PAHs by GC-MS. Comparison of PAH concentrations during different periods, with different air mass origins and under different pollution situations was done. Sources were analysed by diagnostics ratios and principal component analysis (PCA). The threat of PAHs was assessed by BaP equivalent concentrations (BaPeq) and incremental lifetime cancer risk (ILCR). The averaged PAHs for pre-SF, SF and after SF periods were 50.6, 17.2 and 29ngm-3, indicating the variations of PAH sources, with reduced traffic, industrial and construction activities during SF and gradually re-starting of them after-SF. According to PAH mass concentrations, their relative abundance to particles, ratio of PAHs (3-ring+4-ring)/PAHs(5-ring+6-ring), mass concentrations of combustion-derived and carcinogenic PAHs, fireworks burning is an important source for PAHs during SF. The ILCR values for Chinese New Year day were 0.68 and 3.3 per 100,000 exposed children and adults. It suggested the necessity of controlling fireworks burning during Chinese SF period which was always companied with serious regional haze pollution. PAH concentrations exhibited decreasing trend when air masses coming from the following directions as North China Plain (63.9ngm-3); Central China (53.0ngm-3); Shandong Peninsula (46.6ngm-3); Northwest China (18.8ngm-3); Sea (15.8ngm-3). For different pollution situations, they decreased as haze (44.5ngm-3); fog-haze (28.4ngm-3); clear (12.2ngm-3); fog day (9.2ng m-3). Coal combustion, traffic emission, industrial processes and petroleum (only for non-SF holiday periodss) were the main sources of PM2.5 associated PAHs. Fireworks burning contributed 14.0% of PAHs during SF period. Directly measurement of PAHs from fireworks burning is urgently needed for source apportionment studies in the future. Assessment of PAH in air (indoor and outdoor) has been accomplished in Changbai (also known as “Baekdu”) Mountain China/North Korea (Zhao, Kim et al. Citation2015), kitchen air, China (Zhu and Wang Citation2003), Hangzhou, China(Liu, Zhu et al. Citation2001), Tokyo and Beijing supercities (Ando, Katagiri et al. Citation1996), Metropolitan Santiago, Chile(Adonis and Gil Citation2001), Krakow, Poland (Jedrychowski, Perera et al. Citation2015), industrial region in Turkey (Aydin, Kara et al. Citation2014), London and Potugal (Kendall, Duarte et al. Citation2002), Seoul, Korea(Kim, Lee et al. Citation2016), Huddersfield, England(Kingham, Briggs et al., Citation2000), Taranto, Italy(Liberti, Notarnicola et al., Citation2006), Chicargo (Odabasi, Vardar et al. Citation1999), Pearl River Delta (Wang, Huang et al. Citation2013), Beijing–Tianjin region, North China (Wang, Simonich et al. Citation2011, Wang, Yu et al. Citation2014), Qingyang China (Wang, Wu et al. Citation2013), wood-smoke-polluted cities in Chile(Pozo, Estellano et al., Citation2015), industrial Lanzhou City, China (Wang, Zhao et al. Citation2015), polluted air in Korean peninsula(Kim, Lee et al. Citation2016), Beijing air pollution (Liu, Baumgartner et al. Citation2016) air toxics emissions from on-road vehicles in Delhi(Nagpure, Gurjar et al., Citation2016),Semiurban Area of Dourados, Brazil (Ré, Kataoka et al. Citation2015) and PAHs in air in Europe (Garrido, Jiménez-Guerrero et al. Citation2014).
3. PAH in water
PAHs, one of the major groups of anthropogenic environmental pollutants, were firstly identified and measured in coastal waters, native mussels and fish of an industrialized South American estuary.
Zhi et al. (Zhi, Zhao et al. Citation2015) studied the fate of PAHs in the water columns from Poyang Lake. The predominance PAHs are of lower molecular weight congeners demonstrated that petroleum and the combustion products of fuel oil, as well as other pyrogenic sources, contributed to the main input of PAHs in the Poyang region. The vehicle emissions were mostly from diesel engines.
Qin et al. (Qin, He et al. Citation2014) used GC-MS to measure the residual levels of PAHs in the water, suspended particular matter (SPM) and sediment from Lake Chaohu .The spatial–temporal distributions and the SPM–water partition of PAHs and their influencing factors were investigated. The potential sources and contributions of PAHs in the sediment were estimated by positive matrix factorization (PMF) and probabilistic stable isotopic analysis (PSIA). The results showed that the average residual levels of total PAHs (PAH16) in the water, SPM and sediment were 170.7 ± 70.8 ng/L, 210.7 ± 160.7 ng/L and 908.5 ± 1878.1 ng/g dry weights, respectively. The same spatial distribution trend of PAH16 in the water, SPM and sediment was found from high to low: river inflows; western lake; eastern lake; water source area. There was an obvious seasonal trend of PAH16 in the water, while no obvious seasonal trend was found in the SPM. The residues and distributions of PAHs in the water, SPM and sediment relied heavily on carbon content. Significant Pearson correlations were found between LogKoc and LogKow as well as some hydro-meteorological factors. Three major sources of PAHs including coal and biomass combustions, and vehicle emissions were identified.
Penezic et al.(Penezić, Gašparović et al. Citation2014) developed a new sensing system for PAHs in waters. The system consists of a wafer-based device with chip-based mercury on platinum microelectrode as a working electrode and a platinum auxiliary electrode, incorporated into a flow cell system with an external reference electrode. The Hg microelectrode was coated with a phospholipid-triglyceride mixed layer and interactions between anthracene, phenanthrene, pyrene and fluoranthene and the layer were monitored using rapid cyclic voltammetry. The layer proved sensitive to interactions with PAHs in ‘organic matter free’ seawater, with respective detection limits of 0.33, 0.35, 0.15 and 0.32μgL-1 for phenanthrene, pyrene, anthracene and fluoranthene. Tested interferences, such as sodium humate, dextran T-500 and bovine serum albumin, representing humic substances, polysaccharides and proteins, did not have an influence on the layer response. The system was also tested with a river water sample where concentrations of PAHs were determined using the standard addition method and compared with the results obtained by using gas chromatography-mass spectrometry (GC-MS). The concentration of total PAHs obtained by the standard addition method is ∼80% lower compared with the results obtained by GC-MS analysis. The difference is explained by the fact that the electrochemical method measures water-soluble and free PAHs whereas the chromatographic method measures both dissolved and particulate-organic PAHs.
Zhi et al. (Zhi, Zhao et al., Citation2015) studied the fate PAHs and organochlorine pesticides (OCPs) in the water columns from Poyang Lake. The total concentrations of OCPs and PAHs were 19.10–111.78 ng L−1 and 5.56–266.1 ng L−1, respectively. Among OCPs, endosulfans, chlordanes, and HCHs accounted for 21.96%, 24.6% and 24.65%, and were the predominant pollutants. Results suggested that the main sources of DDTs were residue from technical DDTs and dicofol, as well as antifouling paints for ships, while for HCHs, the main sources included long-distance transmission, agriculture activities and the combination of industrial products with separate lindane in use. As for PAHs, the predominance of lower molecular weight congeners demonstrated that petroleum and the combustion products of fuel oil, as well as other pyrogenic sources, contributed to the main input of PAHs in the Poyang region. The vehicle emissions were mostly from diesel engines. Moreover, HCH, DDT and BaP concentrations in water of Poyang Lake were all below the threshold values.
Wang et al. (Wang, Chen et al. Citation2014) used a combination of multiple molecular markers to improve the identification of pollution sources in sediment samples collected from Huaihe River, China. No significant spatial variation of aliphatic hydrocarbons (normal alkanes, n-alkanes) was observed, whereas, relatively high concentrations of 28 PAHs (Σ28PAH) and 20 linear alkylbenzenes (Σ20LAB) in urban sediments and low concentrations of Σ28PAH and Σ20LAB in farm areas were determined. Overall, sediment samples collected from urban areas contained high concentration of Σ20LAB with low concentration of Σ28PAH which mostly originated from pyrolysis, while rural sediments had opposite trends, reflecting the significant input of domestic sewage in urban areas. Residual n-alkanes were mostly from natural sources with relatively low proportion of petrogenic input. For PAHs, the concentrations of diagentic perylene and pyrolytic PAHs from farm areas to urban areas tend to decrease and increase, respectively. Likely origins of pyrolytic PAHs were considered combustion of coal and petroleum related. In the village areas, pyrolytic PAHs were mostly contributed from coal and diesel combustion. Poor domestic wastewater treatment in rural areas caused low ratio of I/E. On the other hand, the results of total toxic benzo[a]pyrene (BaP) equivalent reveal the ecological risk by PAHs was negligible in Huaihe River. PAH assessment were also carried out in the reverine,dams, wastewater, rivers and coastal area of the following countries Malaysia (Tavakoly Sany, Hashim et al. Citation2014), Erjen River in Taiwan (Wang, Liu et al. Citation2015), Qingdao, China (Zhao, Cao et al. Citation2015) niger delta Nigeria (Anyakora and Coker Citation2006), Lagos Lagoon, Nigeria (Benson, Essien et al. Citation2014), France (Bertrand, Mondamert et al. Citation2015), NW Gulf of Mexico (Botello, Soto et al. Citation2015), Seine River (Bourgeault and Gourlay-Francé Citation2013), River Ganga in India (Chakraborty, Sakthivel et al. Citation2014), Niger Delta region Nigeria (Duke Citation2008), Barigui River in Curitiba, Brazil (Froehner, MacHado et al. Citation2012), Taihu Lake (Guo and Fang 2012), Lu Cbbock, Texas. USA (Heintzman, Anderson et al. Citation2015) Pearl River Estuary (Huang, He et al. Citation2015 ), Soltan Abad River, Iran (Kafilzadeh Citation2015), Huangjueya town China (Lan, Sun et al. Citation2014), Pearl River Delta (Li, Duan et al. 2014, Yang, Yu et al. Citation2016), Liaohe River Basin, northeast China (Lv, Xu et al. Citation2014), Songhua River Basin, China(Ma, Liu et al. Citation2013), terai belt of North India (Masih and Lal Citation2014), Europe (Menchaca, Rodríguez et al. Citation2014) Danube River and its tributaries, Hungary, Somme River water, France (Net, Dumoulin et al. Citation2014), suburban area of Japan (Ozaki, Takamura et al. Citation2015) Lake Chaohu, China (Qin, He et al., Citation2014), PeruSaramuro and Trompeteros sites (Reátegui-Zirena, Stewart et al. Citation2014), Almendares River, Cuba (Santana, Massone et al. Citation2015), Taizi River, Northeast of China (Song, Hu et al. Citation2013), Three Gorges Reservoir, China (Wolf, Bergmann et al. Citation2013), Jiulong River Estuary, China:(Wu, Wang et al. Citation2015), Huaihe River, China (Zhang, Liu et al. Citation2014), Beijing, China (Qi, Liu et al. Citation2013), Sydney Basin, Nova Scotia, Canada:Xichuan China (Tai, Zhang et al. Citation2012) (Wangyang River), North China (Zhang, Yu et al. Citation2015), Soan River, Pakistan (Aziz, Syed et al. Citation2014), major rivers in the Czech Republic (Blahova, Leontovycova et al. Citation2013), Daliao River (China) (Chen, Teng et al. Citation2013), Pearl River Delta region (China) (Chen, Teng et al., Citation2013, Wu, Teng et al. Citation2014), Jura region (France) (Chiffre, Degiorgi et al. Citation2015), Southern Taiwan (Chou, Liu et al. Citation2014), Black River and the Ashtabula River, Ohio(Christensen and Bzdusek Citation2005), Columbia River Estuary (Counihan, Waite et al. Citation2014), mountainous river in coastal california (Conaway, Draut et al. Citation2013), Shenzhen River, South China (Deng, Yang et al. Citation2014), Guan River Estuary, China (He, Pang et al. Citation2014), River Brahmaputra in Guwahati, India (Hussain, Balachandran et al. Citation2015), (Berre lagoon), France (Kanzari, Asia et al. Citation2015), urban river (Huveaune), France (Kanzari, Syakti et al. Citation2014), Karoon River, Khuzestan Province, Iran (Keshavarzi, Mokhtarzadeh et al. Citation2015), Prai and Malacca Rivers, Peninsular Malaysia (Keshavarzifard, Zakaria et al. Citation2014), Laolongdong underground river, (Lan, Sun et al. Citation2015), (CitationLiu, Liu et al. 2012), Haihe river basin China (Liu, Zhang et al. 2014), Hun River, northeast China (CitationLiu, He et al. 2015), Liao River Delta, Northeast China (Ma, Ye et al. Citation2014), Barigui River Brazil (Machado, Figueira et al. Citation2014), Neuquen River, Argentine Patagonia (Monza, Loewy et al. Citation2013), Ovia River, Niger Delta region Nigeria (Obinaju, Graf et al. Citation2015), Calabar River, SE Niger Delta, Nigeria (Oyo-Ita, Offem et al. Citation2013), River Elbe Estuary, Germany (Otte, Keiter et al. Citation2013), main river estuaries of Chaohu Lake China (Ren, Wu et al. Citation2015), upper Po River basin Europe (Squadrone, Favaro et al. Citation2014), Yangtze River Estuary (Wang, Feng et al. Citation2013), Yangtze River, China (Yu, Liu et al. 2015), Deep Bay, South China (Qiu, Zhang et al. Citation2009), River Tiber (Italy) (Patrolecco, Ademollo et al. Citation2010), North Sea oil field (Sundt, Pampanin et al. Citation2011), Mediterranean Sea (Castro-Jiménez, Berrojalbiz et al. Citation2012), Kaohsiung Harbor lagoon, Taiwan, North Sea oil field:(Sundt, Ruus et al. Citation2012), marine protected areas (Western Mediterranean) (Marrucci, Marras et al. Citation2013), estuarine water (Chang, Fang et al. Citation2014, Rojo-Nieto, Oliva et al. Citation2014), Tunisian soil irrigated with treated wastewater (Haddaoui, Mahjoub et al. Citation2016), shallow lakes in the Mediterranean region (NW Spain) (Villanueva, Tapia et al. Citation2015, Hijosa-Valsero, Bécares et al., Citation2016), Dalian, Northeast China (Hong, Jia et al. Citation2016), Colombian Cauca River (Sarria-Villa, Ocampo-Duque et al. Citation2016) and Lake Chaohu, China (Qin, He et al. Citation2013).
4. PAH in soil and sediment
Pollution pressures are always placed in the coastal and estuarine ecosystems of any developing and developed countries because of the elevated pollutants discharged from various sources such as industries, waste and human activities. PAHs in the environment were closely linked to human activities, which have been intensively studied for their geochemical interest as markers. Riverine inputs (Boonyatumanond, Wattayakorn et al. Citation2006) are the most important sources of PAHs in the coastal sediments and soil has been identified as the primary reservoir for PAHs in the United Kingdom (Beriro, Cave et al. Citation2016). The waste generated from industrial processes and operations including domestic wastes when treated partially and disposed in soil–water environment enter to lakes, streams, rivers, oceans and other water bodies. The pollutants get dissolved or lie suspended in water or get deposited on soil sediment beds.
Wang et al. (Zhang, Liu et al., Citation2014) collected surface water and sediments from eleven locations on the middle region of Huaihe River to evaluate the environmental effects of urban and industrial activities. The concentrations of sixteen PAHs in water and sediments were between 1.2-5.1 μg/L and 72-139 ng/g, respectively. High levels of PAHs in water were recorded in comparison to those of other countries, while the concentrations in sediments were low to moderate levels. A weak positive correlation was observed (R2 = 0.49, P = 0.13) between PAHs and total organic carbon in water. However, a significant positive correlation (R2 = 0.79, P = 0.004) was found in sediments, which indicated that total organic carbon was a factor in PAH levels in sediments but not in water. The parent ratios were studied by principal component analysis that showed the PAHs from water were predominantly derived from coal combustion (34%), oil spills (49%), and vehicular emissions (17%), while the major PAH sources to sediments were coal combustion. Using the ecological toxic equivalency factor index, it was concluded that the PAHs levels may provide a potential risk in water but not in sediments.
Li et al. (Li and Duan Citation2015) reviewed the status of PAH contamination in China Sea and assessed the concentrations, sources, and fates of PAHs in sediments of China Sea. PAH concentrations in China Sea sediments decreased from north to south due to the higher emissions in North China. Atmosphere was probably the main carrier of PAHs in the north due to the higher contents of atmospheric fine particles and higher wind speeds. However, riverine inputs were probably the most important sources of PAHs in the coastal sediments of South China due to higher rainfall.
Aly et al. (Aly Salem, Morsy et al. Citation2014) analysed PAHs and aliphatic hydrocarbon in sediments collected from the Suez Gulf, Aqaba Gulf and the Red Sea proper stations Egypt. A geological study demonstrated that the sediments in the studied area have a nature from very fine sand to very coarse sand, mainly biogenic fragments, and carbonate aggregates. Total aliphatic hydrocarbon concentrations (C14–C32) ranged from 33.97 to 553.48 ng/g with a mean value of 174.8 ± 167.06. The highest and lowest alkane concentrations are recorded at El-Tour and Ras Mohamed, respectively. Accordingly, the variation in n-alkanes content may refer to the anthropogenic sources (sewage, industrial discharges, and shipping activities) and natural inputs (submerged/floating macrophytes and emergent terrestrial plants and microbial activity). Meanwhile, ΣPAH concentrations were in the range of 0.74–456.91 ng/g, with the mean value of 32.94 ng/g. The highest concentration of total PAHs is recorded in sediments collected from El-Quseir (456.91 ng/g), followed by that in Sharm El Mayaa (100.05 ng/g) and Suez 10 (97.19 ng/g); while lower concentrations are detected in Sheraton (0.74 ng/g), Ras Mohamed and Na’ama Bay (0.74, 6.86 and 11.1 ng/g, respectively). In this context, ratio of low molecular weight of PAHs (2- and 3-rings) to high (4- to 6-rings) has been used to differentiate between the pyrogenic and petrogenic sources of PAHs in the studied samples. In all studied stations, ΣLPAHs/ΣHPAHs ratios were 1, revealing their pyrogenic sources. The concentration levels of PAHs in the current study were compared to the effect range low (ERL) and the effect range medium (ERM) values; the average concentration of level of PAHs for all investigated stations was below the ERL except El-Quseir station which recorded PAHs higher than the ERL but still lower than the ERM. This finding indicated that PAHs in surface sediments of the studied area have no adverse biological effects except at El-Quseir which may cause mild adverse biological effects but not acute effects. To assess the potential health risk of PAHs; the BaP equivalent (BaPE) is used. High levels of BaPE values were found at El-Quseir only, indicating that PAHs in this site showed relatively high toxicity among all sites. Toxic equivalency factors (TEFs) of seven carcinogenic PAHs (BaA, BbF, BkF, BaP, Chr, DBA and InP), were used to quantitatively assess the potential toxicological significance to human health. In this study, the toxic equivalent (TEQcarc) values of sediment samples varied from not detected (ND) to 72.27 ng TEQ g−1, with the mean value of 2.94 ng TEQ g−1. The higher total TEQcarc values were found at El-Quseir Station 72.27 ng TEQ g−1.
Ohura et al. (Ohura, Sakakibara et al. Citation2015) analysed the sedimentary halogenated (chlorinated and brominated) PAHs (Cl/BrPAHs) and investigated contamination processes and sources. Assessments were conducted in sediments from three sites: surface sediments from the Yellow Sea and sediment cores from Kandy Lake and Negombo Lagoon, Sri Lanka. Most of ClPAHs targeted were detected in all sediments. Spatial distributions of total ClPAH concentrations in the Yellow Sea showed the presence of multiple hot spots that differed from those of total PAHs. In Kandy and Negombo sediments, total ClPAH concentrations were slightly higher in surface layers than in bottom layers; the opposite trend was observed for PAHs. Principal component analysis showed that the clusters of most ClPAHs were similar to those of anthropogenically derived elements, but were far from those of PAHs. Consequently, ClPAHs in sediments appear to be persistent contaminants, which may make them appropriate as indicators of anthropogenic sources.
Apostoloupolus et al. (Apostolopoulou, Monteyne et al., Citation2014) investigated the concentrations of 22 PAHs in Posidonia oceanica seagrass, sediments, and seawater from the Alexandroupolis Gulf in the Aegean Sea, from 2007 to 2011. Temporal trends of total PAH contents in P. oceanica and sediments were similar. PAH levels in seawater, sediments, and seagrasses generally decreased with increasing distance from Alexandroupolis Port. Leaves and sheaths of P. oceanica had higher PAH levels than roots and rhizomes. P. oceanica accumulates PAHs and has good potential as a bioindicator of spatiotemporal pollution trends. PAH concentrations were also examined using in situ passive seawater sampling and were compared to results of passive sampling in the laboratory using local sediments and seawater. Levels of high molecular weight PAHs assessed using passive samplers confirmed the decreasing gradient of pollution away from Alexandroupolis Port. Passive sampling also proved useful for investigating sources of PAHs in P. oceanica meadows.
Bacosa and Inoue (Bacosa and Inoue Citation2015) studied the Great East Japan Earthquake caused tsunamis and resulted in widespread damage to human life and infrastructure. The disaster also resulted in contamination of the environment by chemicals such as PAHs. They investigated the degradation potential and describe the PAH-degrading microbial communities from tsunami sediments in Miyagi, Japan. PAH-degrading bacteria were cultured by enrichment using PAH mixture or pyrene alone as carbon and energy sources. Among the ten consortia tested for PAH mixture, seven completely degraded fluorene and more than 95% of phenanthrene in 10 days, while only four consortia partially degraded pyrene. Six consortia partially degraded pyrene as a single substrate. Polymerase chain reaction-denaturing gradient gel electrophoresis (PCR-DGGE) revealed that each sample was dominated by unique microbial populations, regardless of sampling location. The consortia were dominated by known PAHs degraders including Sphingomonas, Pseudomonas, and Sphingobium; and previously unknown degraders such as Dokdonella and Luteimonas. A potentially novel and PAH-degrading Dokdonella was detected for the first time. PAH-ring hydroxylating dioxygenase (PAH-RHDα) gene was shown to be more effective than nidA in estimating pyrene-degrading bacteria in the enriched consortia. The consortia obtained in this study are potential candidates for remediation of PAHs contaminated soils.
Huang et al. (Huang, Chernyak et al., Citation2014) studied the PAHs in the Great Lakes basin because they are of concern due to their toxicity and persistence in bottom sediments. Their nitro derivatives, nitro-PAHs (NPAHs), which can have stronger carcinogenic and mutagenic activity than parent PAHs, may follow similar transport routes and also are accumulated in sediments. Limited information exists regarding the current distribution, trends and loadings of these compounds, especially NPAHs, in Lake Michigan sediments. This study characterizes PAHs, NPAHs, and biomarkers steranes and hopanes in surface sediments collected at 24 offshore sites in southern Lake Michigan. The ΣPAH14 (sum of 14 compounds) ranged from 213 to 1291 ng/g dry weight (dw) across the sites, levels that are 2 to 10 times lower than those reported 20 to 30 years earlier. Compared to consensus-based sediment quality guidelines, PAH concentrations suggest very low risk to benthic organisms. The ΣNPAH5 concentration ranged from 2.9 to 18.6 ng/g dw, and included carcinogenic compounds 1-nitropyrene and 6-nitrochrysene. ΣSterane6 and ΣHopane5 concentrations ranged from 6.2 to 36 and 98 to 355 ng/g dw, respectively. Based on these concentrations, Lake Michigan is approximately receiving 11, 0.16, 0.25 and 3.6 metric tons per year (t/yr) of ΣPAH14, ΣNPAH5, ΣSterane6 and ΣHopane5, respectively. Maps of OC-adjusted concentrations display that concentrations decline with increasing off-shore distance. The major sources of PAHs and NPAHs are pyrogenic in nature, based on diagnostic ratios. Using chemical mass balance models, sources were apportioned to emissions from diesel engines (56 ± 18%), coal power plants (27 ± 14%), coal-tar pavement sealants (16 ± 11%), and coke ovens (7 ± 12%). The biomarkers identify a combination of petrogenic and biogenic sources, with the southern end of the lake more impacted by petroleum. This first report of NPAH levels in sediments of Lake Michigan reveals several carcinogenic compounds at modest concentrations, and a need for further work to assess potential risks to aquatic organisms.
Wang et al.(Wang, Chen et al. Citation2014) studied the identification of pollution sources in sediment samples collected from Huaihe River, China. No significant spatial variation of aliphatic hydrocarbons (normal alkanes, n-alkanes) was observed, whereas, relatively high concentrations of 28 PAHs (Σ28PAH) and 20 linear alkylbenzenes (Σ20LAB) in urban sediments and low concentrations of Σ28PAH and Σ20LAB in farm areas were determined. Overall, sediment samples collected from urban areas contained high concentration of Σ20LAB with low concentration of Σ28PAH which mostly originated from pyrolysis, while rural sediments had opposite trends, reflecting the significant input of domestic sewage in urban areas. Residual n-alkanes were mostly from natural sources with relatively low proportion of petrogenic input. For PAHs, the concentrations of diagentic perylene and pyrolytic PAHs from farm areas to urban areas tend to decrease and increase, respectively. Likely origins of pyrolytic PAHs were considered combustion of coal and petroleum related. In the village areas, pyrolytic PAHs were mostly contributed from coal and diesel combustion. Poor domestic wastewater treatment in rural areas caused low ratio of I/E. On the other hand, the results of total toxic benzo[a]pyrene (BaP) equivalent reveal the ecological risk by PAHs was negligible in Huaihe River Zhang et al. also studied PAH in this river (Zhang, Liu et al., Citation2014, Zhang, Liu et al. Citation2015). Other countries where PAH investigations in soil and sediment actively took place include: Lake Michigan. USA (Huang and Batterman Citation2014, Huang, Chernyak et al., Citation2014), Caspian Sea and Anzali Wetland (Yancheshmeh, Bakhtiari et al. Citation2014), Gulf of Mexico (Tansel, Fuentes et al. Citation2011), Chaohu Lake (Ren, Wu et al., Citation2014), Austin, Texas (Van Metre and Mahler Citation2014), Spain (Veses, Mosteo et al. Citation2014), Erjen River in Taiwan (Wang, Liu et al., Citation2015), Yangtze River Estuary in China (Wang, Liu et al. Citation2014), Brighton, UK (Zhou, Siddiqui et al. Citation2014) Akaki River, Lake Awassa, and Lake Ziway, Ethiopia (Mekonnen, Chandravanshi et al. Citation2015), Yellow River, China (Feng, Li et al. Citation2014, Yuan, Li et al. Citation2014) Yelloe sea China (Zhou, Wang et al. Citation2016), Imam Khomeini Port, Persian Gulf, Iran (Abdollahi, Raoufi et al. Citation2013), Gorgan Bay, Caspian Sea (Araghi, Bastami et al. Citation2014), Mediterranean coastal environment of Egypt (Barakat, Mostafa et al. Citation2011), Mediterranean Sea (Baumard, Budzinski et al. Citation1998), Mar Piccolo in Taranto (Ionian Sea, Southern Italy (Cardellicchio, Buccolieri et al. Citation2007), Chukchi Plateau to the Makarov Basin (Dong, Bai et al. Citation2015), Northern Adriatic Sea (Mediterranean Sea) (Frapiccini and Marini Citation2015), nador lagoon (Morocco) (Giuliani, Piazza et al. Citation2015), southeast China(Leung, Cheung et al., Citation2015) deep sea sediments (Louvado, Gomes et al. Citation2015), San Francisco Bay, CA, USA, (Nilsen, Rosenbauer et al. Citation2015), multi-industrial city, South Korea (Kwon and Choi Citation2014), Sydney Harbour, Nova Scotia, Canada (Walker, Willis et al. Citation2015), British Columbia, Canada (Yunker, Macdonald et al., Citation2015), Subei Grand Canal, China (Zheng, Han et al. Citation2015), Yellow River, China (Feng, Hu et al. Citation2016), Swedish National Monitoring Program (Löf, Sundelin et al. Citation2016) Imo River, SE Nigeria (Oyo-Ita, Oyo-Ita et al. Citation2016), Coatzacoalcos River discharge area (Gulf of Mexico) (Ruiz-Fernández, Betancourt Portela et al. Citation2016), mixed-use reservoir (Sun, Zhang et al. 2016), Luan River Estuary, China (Zhang, Liu et al. Citation2016), Shilianghe Reservoir, eastern China (Zhang, Zhang et al. Citation2016), (Usman, Chaudhary et al. Citation2015), agricultural soil from Southern Spain (De la Rosa, Paneque et al. Citation2016), Indian Himalayan Region (Devi, Yadav et al. Citation2016), soils of an industrial city, Lanzhou, China (Jiang, Yves et al. Citation2016), Soils from Bursa, Turkey (Karaca Citation2016) and Dongjiang River Basin (Zheng, Ran et al. Citation2014), New Brunswick fish farm (Sather, Ikonomou et al. Citation2006) New York City community garden soils (Marquez-Bravo, Briggs et al. Citation2016), sediments in Yangtze River Estuary, China (Yu, Liu et al. 2015), coastal sediment of Klang Strait, Malaysia (Tavakoly Sany, Hashim et al., Citation2014), Japanese remote background site, Noto peninsula (Tang, Hakamata et al. Citation2015) and eggs of a coastal bird (Vidal, Domínguez et al. Citation2011).
5. PAH in sewage sludge
Waste and sewage sludge both industrial and domestic have recently increased as a result of rapid industrialisation and overpopulation. Waste water of these waste and sewage sludge is often use to irrigate farmland and the application of sewage sludge as a fertilizer is a common method used to improve soil properties. However, sewage sludge and waste water may contain various organic pollutants including PAHs. The presence of contaminants considerably restricts the application of sewage sludge for the fertilisation and reclamation of soils. PAHs are ubiquitous in the environment and vary widely in sewage sludge depending on the input of industrial effluents. PAHs may be present as contaminants in wastewater sewage sludge. Due to their toxicity and persistence in the solid phase, information are gathered relating to their presence in sewage sludge in order to determine their contamination risks after land application (Khadhar, Higashi et al. Citation2010, Ning, Lin et al. Citation2014). Wastewater sewage sludge increased the removal of PAHs from soils but the effect is controlled by the physical, chemical and microbial soil properties, the contaminant and microorganisms in wastewater sludge (Ji, Yang et al. Citation2015, Fernández-Luqueño, López-Valdez et al. Citation2016). Lin et al.(Lin, Ning et al. Citation2016) established an efficient oxidation process for the degradation of PAHs in textile dyeing sludge, which increased the removal of PAHs in the sludge.
Recent reports show that as much as 10 - 106 t of sewage sludge was produced in the member countries of the European Union between 2003 and 2006; 8.7106 t in the EU-1 and additional 1.2 - 106 t for the 12 new Member States, and significant amounts of this total have been applied to land, 37% (DGEnv, 2010).
Furthermore, in Europe, the use of sewage sludge in agriculture is regulated under the so-called Sewage Sludge Directive, Directive 86/278/EEC, (EC, 1986) which was adopted with the view to encouraging its application in agriculture and to regulate its use, to prevent harmful effects on soil, vegetation, animals and humans.
Aiemi et al. (Aemig, Chéron et al. Citation2016) studied the fate of organic matter during anaerobic digestion of sewage sludge in batch systems thanks to a sequential chemical fractionation of the particulate phase coupled to fluorescence spectroscopy. Polycyclic Aromatic Hydrocarbons (PAHs) distribution within the organic pools was characterized from their analysis in the residual fraction after each extraction. Both methods were combined to understand the link between PAHs presence in organic pools and their spectral characterization after extraction. Two batch systems (sludge and inoculum mixture) were set up to study the impact of PAHs spiking on their fate and distribution. The sequential fractionation allowed us to extract and characterize about 50% of total Chemical Oxygen Demand. Moreover, fluorescence spectroscopy helped us to understand the organic pools evolution: the most easily extracted pools composed of protein-like molecules were highly degraded meaning that chemical accessibility mimics the bioaccessibility to degrading microorganisms. PAHs were present in all pools of organic matter but native PAHs were mainly present in low accessible (hardly extractable) fractions and during anaerobic digestion, they accumulated in the non-accessible (non extractable) fraction. Spiked PAHs were more dissipated during anaerobic digestion since spiking made them present in more accessible fractions. During the anaerobic digestion, contrary to native PAHs, spiked ones relocated toward less accessible organic fractions confirming the ageing phenomenon. PCA analysis showed that, in spiked mixture, PAHs presence in organic pools is linked to both PAHs physical–chemical properties and quality/quantity of the associated organic pools.
During pyrolysis of sewage a great number of carcinogenic PAHs are emitted into the atmospher and there are some dissipation of PAHs in soil amended with sewage sludge compost.
Oleczuk et al.(Oleszczuk, Zielińska et al. Citation2014) identified the effect of various biochars on the content of freely dissolved (Cfree) PAHs in sewage sludge. Apart from the evaluation of biochars obtained from various materials, the study also included the determination of the effects of biochar particle sizes and biochar production temperature on their ability to bind PAHs in sewage sludge. Increase in biochar dose caused a gradual reduction of C free PAHs content, but only up to the biochar dose of 5%. Depending on the kind of initial material from which the biochar was produced, the reduction of C free PAHs content in sewage sludge varied from 17.4% to 58.0%. Both the temperature and the particle size of biochar had an effect on PAH free concentration reduction. Biochars characterised by a low polarity index (O/C or (O + N)/C) reduced the level of C free PAHs better than biochars with a higher polarity index value.
Zielińska (Zielińska and Oleszczuk Citation2015) investigated whether the pyrolysis affect (reduces or adds) the total quantity of PAHs in sewage sludge-derived biochars and whether the pyrolysis changes the PAHs spectrum in terms of relative contributions of more hazardous components. Additionally, the trace metal content was determined before and after pyrolysis as well as the ecotoxicological parameters test towards plant (Lepidium sativum), bacteria (Vibrio fischeri) and crustacean (Daphnia magna). Sewage sludges conversion to biochar significantly reduced the content of PAHs (from 8- to 25-fold depending on pyrolysis temperature and kind of sludge). The exception was the content of naphthalene. Naphthalene was predominant in sewage sludge-derived biochars. However the concentration of the most hazardous 5- and 6-rings PAHs in sewage sludge-derived biochars was much lower compared to sewage sludge. The pyrolysis of sewage sludges caused also a significant reduction of their toxicity towards the test organisms. Only in the case of crustacean it was observed that the extracts from some biochars, obtained at higher temperatures (600°C and 700°C) were more toxic to D. magna than extracts from sewage sludge.
Sebileska et al. (Siebielska Citation2014) determined changes in naphthalene (Naph), phenanthrene (Phe), pyrene (Pyr), benzo(a)pyrene (BaP) and benzo(ghi)perylene (BgP) concentrations in a mixture of sewage sludge and the organic fraction of municipal waste during composting and anaerobic digestion. The processes were carried out on a laboratory scale. The selected polycyclic aromatic hydrocarbons (PAHs) were analysed in the samples using gas chromatography-mass spectrometry. The rates at which the PAHs concentrations decreased were evaluated during composting and anaerobic digestion. The selected PAHs degradation kinetics were compared during these processes. The most important conclusion of this work is that composting is much more effective than anaerobic digestion in removing five PAHs from a mixture of sewage sludge and the organic fraction of municipal waste.
Karaca et al. (Karaca and Tasdemir Citation2015) investigated the removal of PAHs from municipal treatment sludge,while Shih et al. investicated the removal of PAHs from marine sediment using Fenton process (Shih, Binh et al. Citation2016). Karaca et al, (Karaca, G. and Y. Tasdemir Citation2015) determined the effects of temperature, sunlight, H2O2 and FeSO4·7H2O concentrations on the PAH removal. A UV apparatus was designed for the PAH removal experiments. All experiments were conducted in triplicate. The highest ∑11 PAH removal ratio (71%) was obtained with 4.9M H2O2 addition to sludge (without FeSO4·7H2O addition) at 17°C. It was concluded that ferrous (Fe2+) and ferric (Fe3+) addition was unnecessary because ferrous and ferric contents of the sludge acted as catalysts. ∑11 PAH concentration was reduced 23% with acid cracking. PAH removal efficiency reached about 63% using the Fenton process. Sixty percent PAH was removed from the sludge with using sunlight in ambient air applications. PAH removal was hindered due to an increase in average temperature (17-36°C) during Fenton and Fenton-like processes. It was determined that 3-rings compounds were removed from sludge easier than the heavier compounds. It could also be seen that phenanthrene and anthracene (3-ring compounds) were removed from sludge with evaporation as 20 and 35%, respectively. It was concluded that the evaporation mechanism became more effective with increasing temperature. A special-designed UV apparatus was used in the PAH removal studies and effects of chemicals, temperature, and UV light were investigated. The evaporation mechanism became more effective in PAH removal with increasing temperature. The highest PAH removal ratio was obtained during H2O2 application. It was concluded that iron content in the sludge reacted with H2O2 and contributed to removal of PAHs during H2O2 application.
Hung et al. (Hung, Cam et al. Citation2014) analysed PAHs in sludge from Kim Nguu River, Hanoi, Vietnam to understand the contamination levels, distribution and accumulation pattern of municipal sludge from a highly urbanized area that receive direct discharge of wastewater. Their result also indicated increased levels of PAHs, which are among the first data on PAHs accumulation in municipal sludge from metropolitan area in Vietnam. PAH concentrations ranged from 218 to 751 mg/kg dry wt. (mean: 456 mg/kg dry wt.), which were greater than those reported in sewage sludge from other countries as well as in sediments and soils collected from the same area. Accumulation pattern revealed the predominant of higher-ringed PAH compounds. Indicator ratios suggest the sources of PAHs were probably derived from biomass (wood and coal) and fossil fuel combustion and petroleum emissions. Most of the sludge samples contain PAHs concentrations exceeding various international guidelines values for sludge and sediment, such as probable effect levels, suggesting the possible risk for adverse biological effects in the study area and in the landfill sites where dredged sludge was dumped.
Włodarczyk-Makuła, (Włodarczyk-Makuła Citation2014) determined the changes in the concentration of PAHs in sewage sludge stored under various light conditions. The sewage sludge samples were stored under aerobic conditions in dark, in laboratory conditions and exposed to UV rays. The changes in the concentration of PAHs were analysed in three series: in sludge samples taken from treatment plant, in sludge with the addition of a standard PAH mixture and in sewage sludge with the addition both a standard mixture and with added sodium azide (abiotic samples). Changes in 10 PAHs concentration in sewage sludge samples were studied at seven-day intervals for four weeks. The concentration of PAHs was determination using GC-MS. The losses of 10 hydrocarbons after four weeks of storing were in the range of 12–99%. The half-life of hydrocarbons was in the range of 5–953 d in sewage sludge taken from treatment plant. In sewage sludge amended with standard mixture, half-life of PAHs was not exceeding 137d.
Hu et al. (Hu, Li et al. Citation2014) investigated the distributions of 16 US EPA priority controlled PAHs in seven kinds of different wastewater sewage sludges and bio-oils from the sludge pyrolysis. A lab-scale tube furnace was used to simulate sludge pyrolysis and retrieve condensed oils. PAH determination was conducted with the extraction, concentration, and purification of PAHs in sludge samples and the resulting bio-oils, and then GC-MS analysis. Then, the factors influencing the distributions of different rings of PAHs in pyrolysis bio-oil, such as the chemical characteristics of raw sewage sludge and pyrolysis condition, were analysed. It was noted that the total amount of PAHs in raw sludge is evidently varied with the sludge resource, with values ranging between 9.19 and 23.68 mg/kg. The middle molar weight (MMW) PAH distribution is dominant. PAH concentrations in sludge pyrolysis bio-oil were ranged from 13.72 to 48.9 mg/kg. The most abundant PAHs were the low molar weight (LMW) PAHs. It could be found that the concentration of LMW PAHs in bio-oil is correlated with MMW PAHs in raw sewage sludge at best, which the correlation coefficient is 0.607. For MMW and HMW (high molar weight) PAHs, they are significantly correlated with HMW PAHs in raw sewage sludge, which the correlation coefficients are 0.672 and 0.580, respectively. The concentration of LMW PAHs in bio-oil is also relatively significant and correlated with the volatile matter content of raw sludge. In addition, it was proved that final temperature and residence time have important influences on PAH generations during the pyrolysis of sewage sludge.
Aggresive investigation and monitoring sewage sluge has been pursured in severel locations in many countries of the world including but not the least: Swedish sewage treatment plants (Pettersson, Adolfsson-Erici et al. Citation2006), Xiaodian sewage irrigation area, North of China (Li, Wang et al. 2014), Europe (Suciu, Lamastra et al. Citation2015), Wastewater Treatment Plants in Zhejiang Province, (Hua, Wu et al. Citation2008), sewage sludge incinerators in Korea (Park, Lee et al. Citation2009), Guadalete wastewater treatment Spain (Villar, Callejón et al. Citation2006), Luddington and Lee Valley, in the UK. (Wild, Berrow et al. Citation1991) sludge treatment in Korea (Ju, Lee et al. Citation2009) and sewage treatment plant situated beside an urban river, East China (Wang, Xi et al. Citation2013), e-waste recycling sites (Shi, Zheng et al. Citation2016) specific locations in India (Subramanian, Kunisue et al. Citation2015).
6. PAHs in food
Food consumption is an important pathway for human exposure to environmental contaminants such as PAHs. The exposure to PAHs from food sources is ubiquitous and dietary intake is one of the major exposure pathways of PAHs (Duan, Shen et al. Citation2016) .
The occurrence of PAHs in food is due to either food preparation or processing techniques or entry into the food chain when either crops or plants are grown in contaminated soil or marine life or fish live in contaminated water. Dietary intake of PAHs is the major route of human exposure (Xia, Duan et al. Citation2010). Food can be contaminated by PAHs that are present in air, soil, or water, or during food processing and cooking. The presence of PAHs in food implies a potential risk to human health. Their presence in the environment is reflected in their presence at detectable levels in many types of uncooked food. In addition, cooking processes can generate PAHs in food. PAHs can also be formed during the curing and processing of raw food prior to cooking. Several studies have been carried out to determine the levels of exposure to PAHs from representative human diets, and the proportion of the overall burden of environmental exposure to PAHs that is attributable to the diet. In most cases, it is concluded that diet is the major source of human exposure to PAHs. The major dietary sources of PAHs are cereals and vegetables, rather than meat, except where there is high consumption of meat cooked over an open flame. Cereals and cereal products were identified as a major contributor to the intake of PAHs, owing to their high consumption. PAHs might occur in fats and oils such as sunflower, olive, soybean, palm, and rapeseed oils. Vegetable fats and oils have higher concentration of PAHs in their food group. The concentrations of PAHs are generally greater on the plant surface (outer leaves, peel) than in internal tissue. Seafood and fish can be exposed to PAHs present in water and sediments due to atmospheric pollution or oil spills. PAHs are also formed as a result of some domestic food preparation methods. When food, particularly meat and fish, are cooked over an open flame, PAHs are formed. If the grilled food is in direct contact with the flame, pyrolysis of the drippings from meat or fish generates PAHs that can be deposited on its surface (Lee, Kim et al. Citation2016). Even if not in direct contact, fat dripping onto the flame or hot coals generates these compounds that are carried back onto the surface of the food (Lijinsky and Shubik 1965, Lijinsky and Shubik 1965, Lijinsky and Ross Citation1967). PAH formation during charcoal grilling was shown to be dependent upon the fat content of the meat, duration of cooking and the temperature used.
Smoked and grilled food may contribute significantly to the intake of PAHs if such foods are a large part of the usual diet. Simple practices such as selecting preferentially lean meat and fish and avoiding contact of foods with flames for barbecuing, using less fat for grilling, and cooking at lower temperature forlonger time, results in a significant reduction of food contamination by PAHs (Lijinsky and Ross Citation1967).
Roasting and drying of coffee beans, cocoa beans and tea leaves may increase the PAH content (Pincemaille, Schummer et al., Citation2014) mainly of those which undergo an inappropriate drying process. Rose et al. (Rose, Holland et al., Citation2015) investigated the effects of frying, grilling, barbecuing, toasting and roasting on the formation of 27 different PAHs in foods. A total of 256 samples from in-house cooking experiments were produced. There was little evidence of PAH formation during the grilling, frying, roasting and toasting experiments. Comparison with the raw materials used in the experiments showed little or no increase in PAH concentrations for all of the sample types, regardless of distances from the heat source, cooking mediums and intensity of cooking conditions. Barbecuing with charcoal plus wood chips however resulted in the formation of benzo[a]pyrene in most foods; for beef burgers only, barbecuing over charcoal (without the use of wood chips) gave the highest levels. In general PAH levels increased when the food was barbequed closer to the heat source. For sausages cooked over briquettes, and for beef burgers, beef and salmon cooked over charcoal, the concentration of PAHs was lower when the food was closer to the heat source. Cooking time may result in a moderate increase of PAHs in some foods, although concentrations in beef burgers appeared to fall when cooking time was extended by 50–100%.
Li et al. (Li, Wu et al. Citation2016) determined the concentration of PAH in Youtiao, or oil stick. Youtiao is typical, traditional and widely-consumed fried food in China. The concentration of PAHs in youtiao from different origins was determined. The dietary exposure and cancer risk associated with benzo[a]pyrene equivalents from youtiao consumption were estimated using Monte Carlo simulation. Analysis of 16 PAHs in youtiao was completed by GC-MS. Concentrations of the sum of 16 PAHs were between 9.90 and 89.97μg/kg. The sum concentrations of PAH4, including benzo[a]anthracene (BaA), chrysene (Chr), benzo[. b]fluoranthene (BbF) and benzo[. a]pyrene (BaP), ranged from 1.41 to 26.56μg/kg. The median dietary exposure of BaPeq concentrations from youtiao for children, adolescents, adults and seniors in China, were 0.0147, 0.0101, 0.0561 and 0.0106ng/(kg·day), respectively. Health risk estimates expressed as the 95th percentile incremental lifetime cancer risks (ILCRs) with respect to PAHs indicated a slight potential carcinogenic risk for children in northern China and adults in both the north and south.
Pissinatti, Nunes et al. (Pissinatti, Nunes et al. Citation2015) studied the presence of PAHs in food and concluded that they are potential risk to human health. Although the coffee has been appointed as an important matrix to be monitored for contamination by PAHs, there is still a gap in the literature regarding to the methods of analysis appropriately validated for this scope and also related to the occurrence of contamination in Brazilian commercial samples. They determined ten priority PAHs in roasted coffee by isotope dilution gas chromatography coupled to mass spectrometry was optimized, validated and applied to an exploratory study with 24 commercial samples. The samples were extracted with simultaneous clean-up using pressurized liquid extraction (PLE), followed by liquid–liquid extraction (LLE) and clean-up using silica columns. Linearity was obtained in the concentration range from 0.25 to 4.00 μg kg−1. Matrix effects were not significant. The precision was demonstrated with relative standard deviations varying from 3.3 to 24% under repeatability and from 3.3 to 33% under intermediate precision conditions. The average recovery values ranged between 87 and 111%. These values, as well as the limits of detection, limits of quantification and uncertainties of measurements, met the performance criteria established by European Union regulations. The contamination of the commercial samples ranged from 1.00 ± 0.35 to 11.29 ± 2.33 μg kg−1, indicating the need of further occurrence studies and establishment of monitoring programs in Brazil.
Duedahl-Olesen et al. (Duedahl-Olesen, Navaratnam et al. Citation2015) investigated the presence of 25 PAHs in tea and coffee with focus on four PAHs (PAH4), classified by the European Food Safety Authority (EFSA) as suitable indicators; benz[a]anthracene (BaA), chrysene (CHR), benzo[b]fluoranthene (BbF) and benzo[a]pyrene (BaP). PAH4 from samples of 18 brands of tea leaves and 13 brands of coffee were extracted by pressurized liquid extraction (PLE) followed by highly automated clean up steps for gel permeation chromatography (SX-3) and solid phase extraction (500mg silica). GC-MS were applied for detection of PAH4. The limit of detection (LOD) ranged from 0.1–0.3 μg/kg with recoveries from 94–106% for PAH4. Concentrations of PAH4 followed the pattern of the total sum of 25 PAHs with higher concentrations with a maximum of 115 μg/kg in tea leaves compared to 5.1 μg/kg in coffee. The highest PAH4 levels were found in black tea leaves. An additional 18 samples were used for estimation of transfer from solids to ready-to-drink preparations. Only up to 2% PAH4 were detected in the ready-to-drink tea, while for coffee the PAH4 transfer was up to 14%. The estimated exposure to PAH4 from tea infusions and coffee brew for the average Danish consumption is 29% of the total exposure to PAH4 for Danish consumers.
Adisa et al. (Adisa, Jimenez et al. Citation2015) evaluated twenty-eight different tea samples sold in the United States using HPLC with fluorescence detection (FLD) for their contamination with PAHs. Many PAHs exhibit carcinogenic, mutagenic, and teratogenic properties and have been related to several kinds of cancer in man and experimental animals. The presence of PAHs in environmental samples such as water, sediments, and particulate air has been extensively studied, but food samples have received little attention. Eighteen PAHs congeners were analysed, with percentage recovery higher than 85%. Contamination expressed as the sum of the 18 analysed PAHs was between 101 and 1337 μg/kg on dry mass and the average contents in all of the 28 examined samples was 300 μg/kg on dry mass. Seven of the congeners were found in all samples with wide ranges of concentrations as follows: fluorene (7–48 μg/kg), anthracene (1–31 μg/kg), pyrene (1–970 μg/kg), benzo(a)anthracene (1–18 μg/kg) chrysene (17–365 μg/kg), benzo(a)pyrene (1–29 μg/kg), and indeno(1,2,3-cd)pyrene (4–119 μg/kg). The two most toxic congeners benzo(a)pyrene and dibenzo(a,h)anthracene were found at high concentrations only in Earl Grey Twinnings, Earl Grey Harney& Sons Fine Teas, and Chai Ultra Spice Black Tea Twinnings. Six PAH congeners are considered as suspected carcinogens (U.S.EPA), formed the basis of the estimation of the toxic equivalent (TEQ), Chai Ultra-Spice Black Tea Twinnings had the highest TEQ (110.9) followed by two grey tea samples, Earl Grey Harney & Sons Fine Tea (57.7) and Earl Grey Twinnings (54.5). Decaffeinated grey teas had the lowest TEQs, decaffeinated Earl Grey Bigelow (9.4) and Green Tea Honey Lemon Decaffeinated Lipton.
Garcia et al. (Garcia, Tild et al. Citation2015) described PAHs occurrence in fifty-four samples of tea marketed in the main supermarkets from Argentina. A HPLC method was applied with FLD and UV-VIS diodes array detector (DAD) for the analysis of sixteen PAHs in tea (camellia sinensis), with percentages recoveries higher than 84.5%. Contamination expressed as the sum of sixteen analysed PAHs was between 509.7 and 2746.5 μg kg-1 on dry mass and the mean was 930.4 μg kg-1 on dry mass.
Bansal et al. (Bansal and Kim Citation2015) described the status of PAH pollution among different food types, the route of dietary intake, measures for its reduction, and legislative approaches to control PAH. To this end, a comprehensive review is outlined to evaluate the status of PAH contamination in many important food categories along with dietary recommendations. Our discussion is also extended to describe preventive measures to reduce PAH in food products to help reduce the risks associated with human intake.
Huang and Penning (Huang and Penning Citation2014) conducted the analysis of PAHs in food using HPLC coupled with UV or FD, or by GC/MS. PAH metabolites or PAH–deoxyribonucleic acid adducts are generally detected by LC/MS or diverse immunoassays. Cancer risk has been widely accepted as the most significant health concern associated with PAH-contaminated food. Toxicological studies show that certain PAHs can also produce mutagenic/genotoxic, reproductive and developmental, immunotoxic, cardiovascular, and neurologic effects. It is hard to effectively reduce the levels of PAHs in food and their associated health risks due to their ubiquitous nature. Measures such as instruction and education of manufacturers and consumers, continuous control of PAHs emissions into the environment, and enforcement of safe-limits of PAHs in foods can minimize their intake and health risk.
Shao et al. (Zhao, Zhang et al. Citation2014) studied the residual levels, tissue distribution and human health risk assessment of PAHs in edible fishes, bighead carp (Aristichthys nobilis) and silver carp (Hypophthalmichthys molitrix), from the largest freshwater lake in China, Poyang Lake, were studied. PAHs concentrations ranged from 105 to 513 ng g−1 ww and from 53.9 to 401 ng g−1 ww in different tissues of bighead carp and silver carp, respectively. Low molecular weights (LMW) PAHs were the predominant compounds, suggesting the gill-water transfer might be the major exposure route for PAHs in the studied fish species. Tissue distribution indicated that the hepatobiliary system accumulated higher concentrations of PAHs than the extrahepatic tissues with bile being the most predominant tissue for both species. Composition analysis demonstrated that PAHs were from the combined petrogenic and pyrogenic origin, and the gasoline combustion might be the main source. A preliminary evaluation of human health risk using benzo[a]pyrene (BaP) potency equivalent concentration (PEC) as well as the incremental lifetime cancer risk (ILCR) indicated that PAHs in fish would induce potential carcinogenic effects.
Benzo[a]pyrene is the most commonly formed PAHs by the incomplete combustion of organic matter.PAHs are widely distributed in the environment and human exposure to them is unavoidable. A number of them, such as benzo[a]pyrene, are carcinogenic and mutagenic, and they are widely believed to make a substantial contribution to the overall burden of cancer in humans (White, Chen et al. Citation2016). Their presence in the environment is reflected in their presence at detectable levels in many types of uncooked food. In addition, cooking processes can generate PAHs in food. PAHs can also be formed during the curing and processing of raw food prior to cooking. Several studies have been carried out to determine the levels of exposure to PAHs from representative human diets, and the proportion of the overall burden of environmental exposure to PAHs that is attributable to the diet. In most cases, it is concluded that diet is the major source of human exposure to PAHs. More recently, biomonitoring procedures have been developed to assess human exposure to PAHs and these have also indicated that diet is a major source of exposure. Exposure to nitro-PAHs through food consumption appears to be very low.
Soceanu, et al. (Soceanu, Dobrinas et al. Citation2014) Vegetables are an important part of a healthy diet because of their nutritional value. Contamination in vegetable may prove hazardous for population. PAHs are compounds widespread in the environment, many of them showing carcinogenic effects. These compounds can reach the food chain by different ways and, therefore, the analysis of PAHs in food is a matter of concern. They provided data regarding levels of PAHs: acenaphthene (Ace), acenaphthylene (Acy), fluorene (F), naphthalene (Np), anthracene (An), fluoranthene (Fl), phenanthrene (Ph), benzo[α]anthracene (B[α]An), benzo[k]fluoranthene (B[k]Fl), chrysene (Chry), pyrene (Py), benzo[ghi]perylene (B[ghi]Pe), benzo[α]pyrene (B[α]Py), dibenzo[α,h]anthracene dB[α,h]An, indeno[1,2,3–cd]pyrene (I[1,2,3–cd]Py) in different vegetables (potato, celery, dill, parsley, carrot, cucumber, onion, garlic, cabbage, spinach). Bulb, stem, leaves and fruit were taking into account. Samples were collected from rural and urban areas from Romania, belonging to Dobrogea region. The RSD values were less than 4.7%, indicating that the GC-MS method is precise. The calculated R2 values were above 0.998, indicating the linear relationship between targeted spiking levels and mean introduced concentrations, within the working range of concentrations. LOD and LOQ values were determined using calibration standards. An analytical procedure was used, based on extraction and clean-up step, followed by the injection of concentrated extracts in gas chromatograph Hewlett- Packard 5890. A look at total PAH contents reveals that leafy vegetables showed higher values while chrysene was below the limit of quantification for most studied samples. Sample location appears to be one important factor affecting vegetables PAH contents. The obtained results show that PAHs were detected at higher concentrations in urban areas than in rural area.
Abramsson-Zetterberg et al. (Abramsson-Zetterberg, Darnerud et al. Citation2014) analysed content of PAHs, benzo(a)pyrene (B(a)P) and PAH4 (B(a)P, chrysene, benzo(b)fluoranthene, and benz(a)anthracene) in food i.e. B(a)P and PAH4 levels originating from a home-barbecue-study on sausages and loin of pork. The calculated total mean intake of B(a)P and PAH4 was 50 ng/person and day 276 ng/person and day, respectively. Sugar and sweets, cereal products, meat, and dairy products contributed most to the total intake. In case of PAH concentrations below LOD, 0.03 μg/kg, 1/2 LOD was used in the intake calculations. The highest mean level of B(a)P and PAH4 were found in the barbecued products, but since the estimated consumption in Sweden is low, the contribution to the total food intake is almost negligible, about 2%. The calculated B(a)P levels in food has decreased during the last 10 years and indicates a low cancer risk for the Swedish population.
Bansal and Kim (Bansal and Kim Citation2015) reviewed the status of PAHs pollution among different food types, the route of dietary intake, measures for its reduction, and legislative approaches to control PAH. They evaluated the status of PAH contamination in many important food categories along with dietary recommendations. They described preventive measures to reduce PAH in food products to help reduce the risks associated with human intake. They described the status of PAHs pollution among different food types, the route of dietary intake, measures for its reduction, and legislative approaches to control PAH.
Wickilife et al. (Wickliffe, Overton et al. Citation2014) discussed the increasing ability of contemporary analytical methods to distinguish not only different chemical structures among PAHs but also their concentrations in environmental media. Using seafood contamination following the Deepwater Horizon accident as an example, they identified issues that are emerging in the PAH risk assessment process because of increasing analytical sensitivity for individual PAHs, and they described the paucity of toxicologBackground: PAHs are abundant and widespread environmental chemicals. They are produced naturally and through man-made processes, and they are common in organic media, including petroleum. PAHs, including the large variety of chemically modified or substituted PAHs, are naturally occurring and may constitute health risks if human populations are exposed to hazardous levels. However, toxicity evaluations have not kept pace with modern analytic methods and their increased ability to detect substituted PAHs. Therefore, although it is possible to measure these compounds in seafood and other media, we do not have sufficient information on the potential toxicity of these compounds to incorporate them into human health risk assessments and characterizations. Conclusions: Future research efforts should strategically attempt to fill this toxicological knowledge gap so human health risk assessments of PAHs in environmental media or food can be better determined. This is especially important in the aftermath of petroleum spills.
Some of the food that PAHs have been found in them are : seafood (Cheng, Huang et al. Citation2013, Nwaichi and Ntorgbo Citation2016), baby food (Çolak, Hampikyan et al. Citation2013),smoked food (Gomaa, Gray et al. Citation1993, Karl and Leinemann Citation1996)charcoal-broiled foods (Kangsadalampai, Butryee et al. Citation1997, Chaemsai, Kunanopparat et al. Citation2016), smoked shrimp (Kpoclou, Anihouvi et al. Citation2014),smoked fish (Adeyeye, Oyewole et al. 2016, Adeyeye, Oyewole et al. 2016), grilled food (Larsson, Sahlberg et al. Citation1983, Alomirah, Al-Zenki et al., Citation2011), beverages(Plaza-Bolaños, Frenich et al., Citation2010), smoked meat(Alonge Citation1988, Chen and Lin Citation1997, Chen and Chen Citation2005, Djinovic, Popovic et al. Citation2008, Janoszka Citation2011, Chen, Shen et al. Citation2014, Sikorski and Sinkiewicz Citation2014) and meat product (Potthast and Eigner Citation1975, Binnemann Citation1979, Lawrence and Weber Citation1984, Tóth and Potthast Citation1984, Roda, Simoni et al. Citation1999, Šimko Citation2002, Šimko Citation2005, Kim, Hwang et al. Citation2014, Ledesma, Rendueles et al. Citation2014, Nisha, Dinesh Kumar et al. Citation2015, Li, Dong et al. Citation2016, Singh, Varshney et al. Citation2016), home-smoked food in Iceland (Thorsteinsson Citation1969), shrimp and fish (Steinig Citation1976, Larsson Citation1982, Simko Citation1991, Al-Yakoob, Saeed et al. Citation1994, Moret, Conte et al. Citation1999, Liang, Tse et al. Citation2007, Levengood and Schaeffer Citation2011, Bandowe, Bigalke et al. Citation2014, Motorykin, Santiago-Delgado et al. Citation2015, Pena, Ridley et al. Citation2015, Vignet, Joassard et al. Citation2015, Zhang, Pan et al. Citation2015), food samples collected in Barcelona, Spain (Fontcuberta, Arqués et al.), tea (Schlemitz and Pfannhauser Citation1997, Zongmao and Haibin Citation1997, Kayali-Sayadi Citation1998, Fiedler, Cheung et al. Citation2002, Lin and Zhu Citation2004, Lin, Tu et al. Citation2005, Zuin, Montero et al., Citation2005, Lin, Zhu et al. Citation2006, Viñas, Campillo et al. Citation2007, Ziegenhals, Jira et al. Citation2008, Ishizaki, Sito et al. Citation2011, Li, Li et al. Citation2011, Singh, Vashishth et al. Citation2011, Drabova, Pulkrabova et al. Citation2012, Grover, Singh et al. 2013, Pincemaille, Schummer et al. Citation2014, Duedahl-Olesen, Navaratnam et al., Citation2015, Garcia London, Renoso et al. Citation2015, Chen, Gao et al. Citation2016), coffee (Duedahl-Olesen, Navaratnam et al., Citation2015)and grilled meat (Fretheim Citation1983, Mottier, Parisod et al. Citation2000, Roseiro, Gomes et al. Citation2012, Lee, Kim et al., Citation2016), yerba maté (Thea, Ferreira et al. Citation2016), vegitable (Banerjee, Utture et al. Citation2012, Nwaichi, Wegwu et al. Citation2014, Wang, Xu et al. Citation2016) edible oils (Kang, Lee et al. Citation2014, Akdoğan, Buttinger et al. Citation2016, Hao, Li et al. Citation2016), food crops (Yin, Liang et al. Citation2014), Fish oils(Yebra-Pimentel, Fernández-González et al. Citation2014), vegitable oil (Mocek and Ciemniak Citation2016, Shi, Zhang et al. Citation2016),Syrian olive oils(Krajian and Odeh Citation2014), soybean and rapeseed oils (Hua, Zhao et al. Citation2016), milk (Iwegbue and Bassey Citation2013),Canned fish (Iwegbue, Overah et al. Citation2014), Sausage (Gomes, Santos et al. Citation2013), buffalo mozzarella cheese (Fasano, Esposito et al. Citation2015, Fasano, Esposito et al. Citation2016), Circassian cheese(Gul, Dervisoglu et al. Citation2015), Cashew nut(de Oliveira Galvão, de Melo Cabral et al. Citation2014), honey(Ciemniak, Witczak et al. Citation2013), pork(Xia, Duan et al., Citation2010), wheat grain (Wu, Wang et al. Citation2016) and Cephalopods(Morais, Gomes et al. Citation2013).
7. PAH in coal and petroleum products
The ever-growing energy demand for industrial development in an eco-friendly manner necessitates the evaluation of coal and its combustion residues. In management of coal combustion and its residues on sustainable basis, characterization of PAHs is imperative and essential. A high amount of PAHs are emitted from processing coal, during incomplete combustion of organic matter (Achten and Hofmann Citation2009). Coke oven battery is one of the main sources of fugitive total suspend particles (TSP) and PAHs in coke plants(Kozielska and Konieczyński, Citation2015). The petroleum oil contamination has damaged the environment according to the different decomposed level of compounds which exist in diverse oil pollutants (Lindén and Pålsson Citation2013, Mirvakili and Hadjizadeh Zaker Citation2014, Zhao, Cao et al., Citation2015).
Verma et al. (Verma, Masto et al. Citation2015) evaluated the feed coal, fly ash, and bottom ashes from a power plant (Unchahar, India). Feed coal is low grade high volatile sub-bituminous. Quartz and kaolinite are the predominant minerals in the coal, whereas quartz, mullite, and anhydrite in the ashes. The total PAHs content was much higher in coal (4542 μg kg−1) than fly ash (32.4 μg kg−1) and bottom ash (10.1 μg kg−1). High molecular weight PAHs were predominant in coal and fly ash, low molecular weight PAHs in bottom ash.
Kozielska and Konieczyński (Kozielska and Konieczyński, Citation2015) studied the content of selected PAHs associated with the TSP in the immediate vicinity of the coke oven battery Radlin (Poland) was investigated. TSP collection places were located on battery wall, battery roof, pusher machine and coal transfer car. Revealed TSP concentrations were within the range of 0.50–5.15 mg/m3, the total content of PAHs within wide range of 216.6–28018.9 μg/g. Regardless of the concentration level of PAHs connected with the TSP in the coke oven battery surrounding, it was found that four rings PAHs are the main fraction (50–70%). The high average concentrations of benzo[a]pyrene (BaP) and Toxicity Equivalent BaP (BaPeq) reaching 1.29 and 2.63 μg/m3 respectively in the immediate vicinity of coke oven battery, could pose a serious threat to the health of a coking plant workers. Calculated diagnostic ratios BaA/ (BaA + Ch), Fl/ (Fl + Py), BaP/ (BaP + Ch), BbF/BkF, BaP/BghiP, BaA/Ch are characteristic for the coking process Table .
Chen et al. (Chen, Zhi et al. Citation2015) found that both China and UNEP recommend replacing raw coal chunks with coal briquettes in household sector as clean coal technology (CCT), which has been confirmed by the decreased emissions of particulate matter and black carbon. However, the clean effect has never been systematically checked by other pollutants like PAHs. In this study, 5 coals with different geological maturities were processed as both chunks and briquettes and burned in 3 typical coal stoves for the measurement of emission factors (EFs) of particle-bound PAHs. It was found that the EFs of 16 parent PAHs, 26 nitrated PAHs, 6 oxygenated PAHs, and 8 alkylated PAHs for coal briquettes were 6.90 ± 7.89, 0.04 ± 0.03, 0.65 ± 0.40, and 72.78 ± 18.23 mg/kg, respectively, which were approximately 3.1, 3.7, 1.9, and 171 times those for coal chunks, respectively. Such significant increases in PAH emissions increased human health risk and challenged the policy of CCT,
Gargulio et al. (Gargiulo, Apicella et al. Citation2015) found that the different thermal behaviors and solubilities of large and structurally different PAH mixtures featuring coal tar pitch (CP) and naphthalene synthetic pitch (NP) samples could be read in light of their different molecular weight (MW) distribution and spectroscopic features. The number-average MW obtained by mass spectrometry for CP (417 Da) and NP (691 Da) resulted to be lower in comparison to the values evaluated by size-exclusion chromatography (SEC) (796 and 824 Da for CP and NP, respectively) because of the different response of the detector of mass spectrometry to low- and high-MW components. Hence, SEC showed to be more suitable for the analysis of PAH mixtures overlapping and covering a higher mass range in comparison to mass spectrometry. Insights into structural PAH features were given by means of spectroscopic analysis [infrared (IR), ultraviolet-visible (UV-vis), and fluorescence], allowing for the discrimination between different families of PAHs as ortho-fused PAHs and rylenes interspersed with aliphatic (mainly naphthenic) groups, mainly featuring CP and NP, respectively. Besides showing the different aromaticity and aliphatic/aromatic hydrogen distribution, the improvement of Fourier transform infrared (FTIR) and UV-vis absorption analysis put also in evidence the contribution of carbon-rich particle impurities and PAH aggregates in CP and NP, respectively.
Pergal et al.(Pergal, Relić et al. Citation2014) investigated the threat PAHs from deposited coal ash, obtained by coal combustion in this power plant, can represent for the surrounding environment, samples of coal ash were submitted to extraction with river water used for transport of coal ash to the dump, as well as with water of different ionic strength and acidity. It was found that, out of 16 EPA priority PAHs, only naphthalene, acenaphthylene, fluorene, phenantrene, fluoranthene, and pyrene were found in measurable concentrations in the different extracts. Their combined concentration was around 0.1 μg/L, so they do not, in terms of leached concentrations, represent serious danger for the surrounding environment. In all cases of established (and leached) PAH compounds, changes of ionic strength, acidity, or the presence of organic compounds in river water may to some extent influence the leached concentrations. However, under the examined conditions, similar to those present in the environment, leached concentrations were not more than 50% greater than the concentrations leached by distilled water. Therefore, water desorption is likely the most important mechanism responsible for leaching of PAH compounds from filter coal ash.
Ruiz-Fernadace et al. (Ruiz-Fernández, Betancourt Portela et al., Citation2016) studied to assess the contamination impact by the oil industry in the southern Gulf of Mexico. The sedimentary record showed the prevalence of petrogenic PAHs between 1950s and 1970s, a period during which waste discharges from the oil industry were not regulated. Later on, sediments exhibited higher contents of pyrogenic PAHs, attributed to the incineration of petrochemical industry wastes and recurrent wildfires in open dumpsites at the nearby swamps. The total concentration of the 16 EPA-priority PAHs indicated low levels of contamination (100 ng g−1), except a peak value (1000 ng g−1) during the late 1970s, most likely due to the major oil spill produced by the blowout of the Ixtoc-I offshore oil rig in deep waters of the southwestern Gulf of Mexico.
Authors who investigated PAHs in coal.coke and petroleum products include (Gray Citation1984, Chu, Villeneuve et al. Citation1994, Adedosu, Sonibare et al. Citation2010) Mastra et al. (Mastral, Callén et al. Citation1995, Mastral, Callén et al. Citation1996), Srivastava et al. (Srivastava, Srivastava et al. Citation1985) Scotts and Stephens (Stout and Emsbo-Mattingly Citation2008), Tiruta-Barna et al. (Tiruta-Barna, Mahjoub et al. Citation2006), Liu et al. (Liu, Niu et al. Citation2008, Liu, Wang et al. Citation2012) and Zhao et al. (Zhao, Zhu et al. Citation2015), Gao et al. (Gao, He et al. Citation2016), Kozielska (Kozielska and Konieczyński, Citation2015).
8. Toxicity, carcinogenicity and mutagenicity of PAHS
The growing rate of industrialization is gradually leading to contamination and deterioration of the environment and pollution is likely to reach disturbing levels in the years ahead. PAHs are major pollutant and public concern over the deleterious effects of PAHs has grown rapidly due to recognition of their toxicity, carcinogenicity, and teratogenicity. Benzo(a)pyrene (BaP) possesses the greatest carcinogenic potential among the various PAHs. PAHs are widespread in various ecosystems and are pollutants of great concern due to their potential toxicity, mutagenicity and carcinogenicity (Wynder and Hoffmann Citation1959, Andrews, Thibault et al. Citation1978, Lowe and Silverman Citation1984, Peng, Xiong et al. Citation2008, Jung, Yan et al. Citation2010, Broyde, Wang et al. Citation2011, Klein Citation2014). Twelve, likely carcinogenic, PAHs (i.e., phenanthrene (Phe), anthracene (An), fluoranthene (Fluo), pyrene (Pyr), benz[a]anthracene (B[a]A), chrysene (Chry), benzo[b]fluoranthene (B[b]F), benzo[k]fluoranthene (B[k]F), benzo[a]pyrene (B[a]P), indeno[1,2,3-cd]pyrene (Ind), dibenz[a,h]anthracene (D[a,h]A), and benzo[g,h,i]perylene (B[g,h]P) (Pongpiachan, Tipmanee et al. Citation2013).
Seven PAH compounds have been classified as probable human carcinogens: benz(a)anthracene, benzo(a)pyrene, benzo(b)fluoranthene, benzo(k) fluoranthene, chrysene, dibenz(ah)anthracene, and indeno(1,2,3-cd) pyrene Table5. When PAHs enter agricultural soils through sewage sludge, they pose an environmental risk to soil organisms, agricultural food grown in that soil and humans that eats from the food grown on PAHs polluted soil. Industries such as petrochemical, pharmaceutical, insecticides and fertilizers generates the hazardous waste comprising of inorganic and organic compounds. Organic compounds mainly composed PAHs, are one of the toxic environmental pollutant. Excessive exposure to PAHs often results in lung cancer, a disease with the highest cancer mortality in the United States. After entry into the lung, PAHs induce phase I metabolic enzymes such as cytochrome P450 (CYP) monooxygenases, i.e. CYP1A1/2 and 1B1, and phase II enzymes such as glutathione S-transferases, UDP glucuronyl transferases, NADPH quinone oxidoreductases (NQOs), aldo-keto reductases (AKRs), and epoxide hydrolases (EHs), via the aryl hydrocarbon receptor (AhR)-dependent and independent pathways. PAHs are among the likely major causative agents for lung cancer in smokers. PAHs require metabolic activation to exert their carcinogenic effects, and one important pathway proceeds through a three-step sequence resulting in the formation of diol epoxides, which react with DNA to produce adducts that can cause mutations and initiate the carcinogenic process. Humans can also be exposed to PAHs through diet, via consumption of charcoal broiled foods. Metabolism of PAHs through the CYP1A1/1B1/EH pathway, CYP peroxidase pathway, and AKR pathway leads to the formation of the active carcinogens diol-epoxides, radical cations, and o-quinones(Moorthy, Chu et al. Citation2015). Exposure to carcinogenic PAHs, such as benzoapyrene (BaP), may have a role in increased esophageal cancer risk (Deziel, Wei et al. Citation2013). With rapid urbanization, the contamination of policy PAHs has become one of the major factors threatening urban soil quality and human health.
PAHs are highly hazardous and can negatively affect health as well as the environment.These health effects include carcinogenesis, localized skin effects, pulmonary and respiratory problems, genetic reproductive and developmental effects, behavioral, neurotoxic, other organ system effects. Human liver toxicity is associated with exposure to PAHs. PAHs are responsible for more cancers, primarily lung cancers, than any other carcinogen (Hakura and Sonoda Citation2013, Jarvis, Dreij et al. Citation2014, Rengarajan, Rajendran et al. Citation2015, White, Teitelbaum et al., Citation2015, Manoli, Kouras et al. Citation2016). PAHs in air caused asthma in children living in developed countries and the leading cause of childhood hospitalization and school absenteeism(Karama, Peters et al. 2015). Exposures to traffic-related air pollutants including PAH have been associated with the development and exacerbation of asthma (Jung, Lovinsky-Desir et al. Citation2015).The most studied PAHs are unsubstituted multi-ring structures. These compounds are not DNA reactive, and must be modified to become carcinogenic. PAHs are environmental carcinogens whose metabolites can react with DNA to form bulky DNA adducts(Broyde, Wang et al., Citation2011).
DNA damage is an important step in carcinogenesis. The Ames assay is a short-term screening of carcinogens that induce DNA damage. Most carcinogens require enzymatic activation through oxidation by cytochrome P450 (CYP450) in the presence of S9 mix. A combination of iron (Fe)(III) porphyrin and an oxidant is also able to oxidize compounds as an alternative metabolic pathway to CYP450. Previously it was reported that a chemical model containing a water-soluble 5,10,15,20-tetrakis(1-methylpyridinium4-yl)porphyrinatoiron(III) chloride (4-MPy) and tert-butyl hydroperoxide (t-BuOOH) activated aromatic amines and amides(Inami, Ishikawa et al. 2010).
PAHs are considered to be important, dangerous and priority pollutants, which cause serious health problems and/or genetic defects in humans, as well as harming the flora and fauna of affected habitats. PAHs are ubiquitous environmental pollutants that are potent mutagens and carcinogens. They are human carcinogens and mutagens; they are toxic to all living organisms. Atmospheric PAHs may cause respiratory problems, impair pulmonary function and cause bronchitis (Tsapakis and Stephanou Citation2005). PAHs are widely distributed in the environment and human exposure to them is unavoidable. A number of them, such as benzo[a]pyrene, are carcinogenic and mutagenic, and they are widely believed to make a substantial contribution to the overall burden of cancer in humans. Due to their carcinogenic, mutagenic and teratogenic potential, the removal of PAHs from aqueous environment using physical, biological and chemical processes has been studied by several researchers. (Kim, Tishbee et al. Citation1981, Kangsadalampai, Butryee et al., Citation1997, Inami, Ishikawa et al. 2010, Kang, Cheung et al. 2011).
Ever since benzo (a) pyrene (BaP)1 was recognized as a carcinogen, at the beginning of this century, the presence of it and of other PAHs in the environment has received continuous attention(Liu, Chen et al., Citation2014, Liu, Pan et al. Citation2015) Table . PAHs are ubiquitous environmental pollutants that are potent mutagens and carcinogens. Researchers have taken advantage of these properties to investigate the mechanisms by which chemicals cause cancer of the skin and other organs. When applied to the skin of mice, several carcinogenic PAHs have also been shown to interact with the immune system, stimulating immune responses and resulting in the development of antigen-specific T-cell- mediated immunity. Due to their carcinogenic, mutagenic and teratogenic potential, the removal of PAHs from aqueous environment using physical, biological and chemical processes has been studied by several researchers. Toxic equivalency factors (TEFs) approach was used for quantitative risk estimate, and incremental lifetime cancer risk (ILCR) was calculated (Hoseini, Yunesian et al. Citation2016).
Table 4. Maximum levels of benzo[a]pyrene in food, specified in Commission Regulation (EC) 208/2005 (Numbering of food groups is identical with numbering in Regulation)(Wenzl, Simon et al., Citation2006)
DNA damage is an important step in carcinogenesis. The Ames assay is a short-term screening of carcinogens that induce DNA damage. Most carcinogens require enzymatic activation through oxidation by cytochrome P450 (CYP450) in the presence of S9 mix. A combination of iron (Fe)(III) porphyrin and an oxidant is also able to oxidize compounds as an alternative metabolic pathway to CYP450. Previously it was reported that a chemical model containing a water-soluble 5,10,15,20-tetrakis(1-methylpyridinium4-yl)porphyrinatoiron(III) chloride (4-MPy) and tert-butyl hydroperoxide (t-BuOOH) activated aromatic amines and amides.
Nam et al (Nam, Jeon et al. Citation2015) determine the toxic effects of PAHs on earthworms. Five PAHs were used: fluorene, anthracene, phenanthrene, fluoranthene, and pyrene. Only fluorene and phenanthrene exhibited toxicity (LC values 394.09 and 114.02 g L−1, respectively) against the earthworm Eisenia fetida. None of the other PAHs tested in this study enhanced the mortality of adult earthworm until the concentrations reached to 1000 g L−1. After exposure to PAHs, acetylcholinesterase (AChE) activity in E. fetida decreased in a concentration-dependent manner, and phenanthrene exhibited the strongest inhibitory effect on AChE, followed by fluorene. Activity of a representative detoxifying enzyme, carboxylesterase, was dramatically reduced in E. fetida exposed to all tested PAHs in comparison with that observed in the control test. The remaining glutathione S-transferase activity significantly decreased in E. fetida after exposure to PAHs. To profile small proteins <20 kDa, SELDI-TOF MS with Q10 ProteinChips was used, and 54 proteins were identified as being significantly different from the control (p = 0.05). Among them, the expressions of three proteins at 4501.8, 4712.4, and 4747.9 m/z were only enhanced in E. fetida exposed to anthracene and pyrene. One protein with 16,174 m/z was selectively expressed in E. fetida exposed to fluorene, phenanthrene, and fluoranthene. These proteins may be potential biomarkers for the five PAHs tested in E. fetida.
Lui et al. (Liu, Pan et al. Citation2015) performed digital gene expression (DGE) to investigate the digestive gland transcriptome profile of the clam Ruditapes philippinarum exposed to BaP. A total of 10,508,312 and 11,414,297 clean reads were generated respectively, from control and BaP exposure DGE libraries. One hundred and forty-five differentially expressed genes were detected after comparing two libraries with 58 up-regulated and 87 down-regulated genes. GO annotation and KEGG pathway analyses were performed on all genes to understand their biological functions and processes. The results showed that numerous enriched differentially expressed genes are related to growth and development, antioxidant metabolism, apoptosis and detoxification metabolism. Quantitative real-time PCR was performed to verify the expressed genes of DGE. Our results provide evidences that RNA-seq is a powerful tool for toxicology and capable of generating novel and valuable information at the transcriptome level for characterizing deleterious effects caused by BaP.
Gao et al. (CitationGao, Wang et al. 2015) collected 24-hPM2.5 samples were at six sites in a subtropical city of South China during November-December, 2009. Particle-phase concentrations of PAHs and organic tracers such as hopanes for vehicular emissions (VE), levoglucosan for biomass burning (BB) and picene for coal combustion (CC) were determined. Meanwhile, their gas-phase concentrations were calculated from gas/particle (G/P) partitioning theory using the particle-phase concentrations. The 4 ring PAHs (fluoranthene to chrysene) had lower particle-phase fractions (10%-79%) than other species. Estimated BaPeq and lifetime cancer risk for particle-only (P-only) vs gas+particle (G+P) data sets showed similar values, indicating PAHs with 5-7 rings dominated the carcinogenicity of PAHs. Positive Matrix Factorization (PMF) was applied on both P-only and G+P data sets to estimate the source contributions to PAHs and their toxicity. Three common sources were identified: VE, BB and CC, with CC as the most significant source for both particulate (58%) and total (G+P, 40%) PAHs. While CC exhibited consistent contributions to BaPeq for P-only (66%) vs G+P (62%) solutions, VE and BB contributions were under- and overestimated by 68% and 47%, respectively by the P-only solution, as compared to the G+P solution. The results provide an insight on the impact of G/P partitioning on the source apportionment of PAHs and their toxicity.
Hafnar et al. (Hafner, Gartiser et al. Citation2015) prepared and dissolved acetonic Soxhlet extracts from three German river sediments from the River Rhine (Altrip and Ehrenbreitstein with moderate contamination) and River Elbe (Veringkanal Hamburg heavily contaminated) were prepared and redissolved in dimethyl sulfoxide (DMSO). These extracts were analysed with a standard bioassay battery with organisms from different trophic levels (bacteria, algae, Daphnia, fish) as well as in the Ames test and the umuC test for bacterial mutagenicity and genotoxicity according to the respective OECD and ISO guidelines. In total, 0.01% (standard) up to 0.25% (only fish embryo test) of the DMSO sediment extract was dosed to the test systems resulting in maximum sediment equivalent concentrations (SEQ) of 2 up to 50 g l−1. The sediment of Veringkanal near Hamburg harbor was significantly more toxic in most tests compared to the sediment extracts from Altrip and Ehrenbreitstein from the River Rhine. The most toxic effect found for Veringkanal was in the algae test with an E (72 h) of 0.00226 g l−1 SEQ. Ehrenbreitstein and Altrip samples were about factor 1,000 less toxic. In the Daphnia, Lemna, and acute fish toxicity tests, no toxicity at all was found at 2 g l−1 SEQ. corresponding to 0.01% DMSO. Only when increasing the DMSO concentration the fish embryo test showed a 22-fold higher toxicity for Veringkanal than for Ehrenbreitstein and Altrip samples, while the toxicity difference was less evident for the Daphnia test due to the overlaying solvent toxicity above 0.05% dimethyl sulfoxide (DMSO). The higher toxicities observed with the Veringkanal sample are supported by the PAH and PCB concentrations analysed in the sediments. The sediment extracts of Altrip and Veringkanal were mutagenic in the Ames tester strain TA98 with metabolic activation (S9-mix). The findings allow a better ecotoxicological characterization of the sediments extensively analysed in all subprojects of the DanTox project (e.g., Garcia-Kaeufer et al. Environ Sci Pollut Res. doi:10.1007/s11356-014-3894-4, 2014; Schiwy et al. Environ Sci Pollut Res. doi:10.1007/s11356-014-3185-0, 2014; Hollert and Keiter 2015). In the absence of agreed limit values for sediment extracts in standard tests, further data with unpolluted reference sediments are required for a quantitative risk assessment of the investigated polluted sediments.
Li et al.(Li, Lang et al. 2014) analysed 16 US EPA priority PAHs in surface soils collected from reed wetland of Liaohe estuary. Total concentrations of the sixteen PAHs ranged from 235 ng g− 1 to 374 ng g− 1, while the total concentrations of seven carcinogenic PAHs (cPAHs) varied from 82.6 ng g− 1 to 109 ng g− 1. Toxicity of PAHs was assessed using toxic equivalent quantity (TEQ). The BaP and DBahA were the major contributors to TEQBaP, although IND showed the highest concentration level. Quantitative source apportionment of PAHs and toxicity (i.e. TEQBaP) were performed, using the CMB–TEQ (chemical mass balance model and TEQ) method. Results showed that, the vehicular sources (gasoline and diesel engine emissions) yielded a higher contribution to TEQBaP (95.7%) than that to PAHs (57.1%), while petrogenic source and biomass burning, two important contributors for total PAHs (21.6% and 21.3%, respectively), contributed a little to TEQBaP (3.6% and 0.7%, respectively). Hanedar et al.(Hanedar, Alp et al., Citation2014) and Fadiel et al. (Fadiel, Epperson et al., Citation2013) have demonstrated that intrauterine growth restriction (IUGR) has been associated with exposure to PAHs which are released in the combustion of oil, fuel, gas, garbage, and tobacco. Pregnant women exposed to PAHs are at risk of the effects of these environmental toxins; for example, benzo-α-pyrene (BαP) is able to enter the blood stream and could contribute to IUGR or other developmental abnormalities via effects on the placental cells. Since IUGR has been associated with decreased cord blood concentrations of immunoreactive insulin-like growth factor 1 (ir-IGF-1) and IUGR has been associated with disordered development and fetal programming, they tested the effects of BαP on human placental trophoblast cells in culture. Experimental: IGF-1 expression and activation was studied using an immortalized human placental trophoblast cell line (HTR-8). The cells were treated with vehicle control or 1 μmol/L BαP, or 5 μmol/L BαP for 12 hours. RNA was extracted and the exons of IGF-1 were amplified using reverse transcriptase-polymerase chain reaction (RT-PCR). The ir-IGF-1 expression levels were compared using gle lectrophoresis. The PCR products were sequenced, and levels of mutation were measured with comparative sequence analysis. A computational protein analysis (computer simulation) was performed in order to assess the potential impact of BaP-associated mutation on IGF-1 protein function. Results: The IGF-1 pression decreased considerably in BαP-treated cells relative to untreated controls (P <.05), also in a dose-dependent manner. Comparative sequence analysis indicated that the level of BαP exposure correlated with the percentage of base pair mutations in IGF-1 nucleotide sequences for both treatment groups (P <05). Shifts were observed in the open reading frame, indicating a possible change in the IGF-1 start codon. Protein folding simulation analysis indicated that the base pair changes induced by BαP weakened IGF-1-IGF binding protein (IGFBP) interaction. They concluded that the exposure of human placental trophoblast cells to BαP exposure results in reduction of IGF-1 expression and base pair mutations. The direct action of BαP on the placenta indicates that it may not be necessary for BαP to access other maternal tissues in order for gene abnormalities to occur. Given that PAHs are known to work through aryl hydrocarbon hydrolase (AHH), these results are likely due to the presence of AHH in HTR cells. Computational modeling of BαP actions on IGF1, substrate-ligand binding, supports the biological premise of this work and underlines the need to determine actual biological effects rather than equating immune to bioactivity of IGF1.
Meyer (Meyer, Seiler et al. Citation2014) studied a German lignite extract that exhibited toxicity to Danio rerio and Caenorhabditis elegans and was shown to have mutagenic and dioxin-like activity. Besides the comparatively low content of known toxic PAH, highly intensive peaks of m/z 274 and m/z 324 were observed during the chromatographic analysis. These compounds are assumed to be alkylated chrysenes and picenes (3,3,7-trimethyl-1,2,3,4-tetrahydrochrysene, 1,2-(1’-isopropylpropano)-7-methylchrysene and an isomer of the latter, 1,2,9-trimethyl-1,2,3,4-tetrahydropicene and 2,2,9-trimethyl-1,2,3,4-tetrahydropicene). These compounds are intermediates in the diagenetic formation of chrysene and picene from triterpenoids. Due to their general high abundance in lignites and the toxicity observed for the lignite extract, the mechanism-specific toxicity and bioavailability of these compounds were investigated in the present study using the approach of effect-directed analysis. After the separation of the compounds from other PAH, their mutagenic activity (Ames Fluctuation test) and dioxin-like activity (EROD activity) were studied. Both, mutation induction factor (up to 2.9 ± 2.7) and dioxin-like activity (Bio-TEQ of 224 ± 75. pg/g; represents the amount (pg) 2,3,7,8-tetrachlorodibenzo- p-dioxin per g coal that would provoke the same toxic effect) were rather low. Bioavailability estimated by the bioaccumulation test with Lumbriculus variegatus was also very limited. Based on the obtained results, the environmental risk of the highly abundant alkylated chrysenes and picenes in lignites is concluded to be low.
Toxicity evaluation and source apportionment of PAHs in river water and sediments have been monitored in the following places: at three stations in Istanbul, Turkey (Hanedar, Alp et al., Citation2014), Binacional Itaipu Lake, Brazil (Kalwa, Quináia et al. Citation2014), Shandong Peninsula (Li, Lang et al. 2014), Khyber Pakhtunkhwa Province, Pakistan (Waqas, Khan et al. Citation2014), North China Plain (Yang, Qiu et al. 2014), Gulf of Mexico(Alloy, Boube et al. Citation2015), Bohai Bay in northeastern China(An, Zheng et al. Citation2012), Southern Argentina (Ares, Eckl et al. Citation2000), Kaohsiung Harbor, Taiwan(Chen, Chen et al. 2013),UK (Fisher, Law et al. Citation2011), German rivers (Hafner, Gartiser et al., Citation2015), Punjab province (Pakistan) (Kamal, Malik et al. Citation2014), major cities around the Pearl River Delta (Kang, Cheung et al. 2011, Wu, Leung et al. Citation2014), Liaohe estuary (Li, Lang et al. 2014),Yangtze River estuary (Liu, Chen et al., Citation2014), Mediterranean and Black Seas open waters (Mesquita, Dachs et al. Citation2016), urban traffic soil of Dhanbad, India(Suman, Sinha et al. Citation2016) megacity in China (Afreen, Muthoosamy et al. Citation2015), agricultural soils of Delhi, India (Agarwal, Khillare et al. Citation2009), Soil in Japan (Kobayashi and Sumida Citation2015) and Soil in Kumasi Ghana (Bortey-Sam, Ikenaka et al. Citation2014).
9. Biomonitoring
Accumulation of PAHs in biological samples such as plants and animals are commonly used for biomonitoring of PAHs. Biomonitoring is an excellent method for capturing the results of all exposures, regardless of route (Talaska, Thoroman et al., Citation2014). PAHs can accumulate in the food chain, due to their lipophilic properties. Fish can accumulate contaminants including PAHs and frequent consumption of such contaminated fish can pose risk to human health. Bivalve mollusks such as mussels and oysters can accumulate high molecular mass PAHs because they filter large volumes of water and they are not capable to metabolize efficiently all PAHs (Afolabi, Adesulu et al. Citation1983, Harvey Citation1997). The average content of PAHs is lower in fish musculature than in the tissues of mollusks, since fish (in contrast to bivalves) have the ability to oxidize and further metabolize PAHs to water-soluble compounds that are excreted by the living organism. The average concentration of benzo[a]pyrene in bivalve mollusks is mostly much below 10 μg/kg. However, samples taken from polluted waters may contain higher amounts (stolyhwo 2005). Cultured and native mussels were used to evaluate the contamination by PAHs, ranging from uncontaminated aquaculture areas to low and moderately contaminated coastal regions up to a chronically contaminated bay region. Biomonitoring studies, based on pollutant accumulation analyses in tree leaves, allow evaluating the impact caused by air- dispersed pollutants on ecosystems, providing useful data, complementary to those obtained by instrumental monitoring. In particular, leaves of sclerophylls present morphological characteristics, such as the presence of hairs and of a tick cuticle, making them particularly useful in bioaccumulation studies.
Baldantoni (Baldantoni, De Nicola et al. Citation2014) compared PAHs leaf accumulation capabilities of two Mediterranean tree species. They also evaluated the impact of a cement plant and/or of other anthropogenic activities occurring in industrial and urban areas on PAH depositions. For these purposes, holm oak (Quercus ilex L.) and olive (Olea europaea L.) leaves collected along a transect industrial-urban-remote sites in southern Italy were employed. A different accumulation degree was observed for the two species. For HMs, Q. ilex leaves had the highest concentrations. The results showed that the influence of the cement plant emissions on pollutant concentrations was substantial in the area closer to clinker production and storage with the highest indeno(1,2,3-c,d)pyrene, benzo(g,h,i)perylene and benzo(a)anthracene leaf concentrations. However, Q. ilex leaves showed high PAH concentrations also in the urban site, in relation to vehicular traffic emissions and depositions. The comparison of the results of the present study with those from the literature indicates that the overall air quality of the studied sites is not particularly compromised, also in proximity of the cement production. The use of holm oak should be preferred in biomonitoring due to its wider distribution compared to O. europaea.
Zena et al. (Zena, Speciale et al. Citation2015) clarified if acute exposure of sea bream (. Sparus aurata, a fish species of great economic importance in the Atlantic and Mediterranean areas) to a PAH, benzo[a]pyrene (B[a]P), at a dose that can induce CYP1A and pathological changes in fish gills, liver and muscle, can induce accumulation in muscle. They investigated the cytotoxic effects (as changes in cell viability, DNA laddering and glutathione content) of in vitro exposure of human peripheral blood mononuclear cells (PBMCs) to organic extracts obtained from muscle of sea breams previously exposed via water to B[a]P (2. mg/l, for 12, 24 and 72. h). At this level of exposure, B[a]P caused morphological changes, inflammatory response and CYP1A induction not only in sea bream gills and liver but also in muscle, furthermore, in fish muscle they observed a substantial B[a]P accumulation, which may be associated with the increased CYP1A activity in liver and especially in muscle. However, when PBMCs were exposed to organic extracts obtained from sea bream muscle contaminated with B[a]P, a toxic, although modest effect was revealed, consisting in a significant decrease in cell glutathione levels without alterations in cell viability and DNA laddering. This suggests that consumption of sea breams from B[a]P contaminated waters might represent a risk for human health.
Seega et al. (Seegar, Yates et al. Citation2015) measured 16 parent (unsubstituted) PAHs, priority pollutants identified by the United States Environmental Protection Agency (EPA) and components of crude oil, in peripheral blood cells of migrating Peregrine Falcons from 2009 to 2011. The study was designed to assess the spatial and temporal trends of crude oil exposure associated with the 2010 Deepwater Horizon (DWH) oil spill which started 20 April 2010 and was capped on 15 July of that year. Basal PAH blood distributions were determined from pre-DWH oil spill (2009) and unaffected reference area sampling. This sentinel species, a predator of shorebirds and seabirds during migration, was potentially exposed to residual oil from the spill in the northern Gulf of Mexico. Results demonstrated an increased incidence (frequency of PAH detection and blood concentrations) of PAH contamination in 2010 fall migrants sampled along the Texas Gulf Coast, declining to near basal levels in 2011. Kaplan–Meier peak mean ∑PAH blood concentration estimates varied with age (Juveniles-16.28 ± 1.25, Adults-5.41 ± 1.10 ng/g, wet weight) and PAHs detected, likely attributed to the discussed Tundra Peregrine natural history traits. Increased incidence of fluorene, pyrene and anthracene, with the presence of alkylated PAHs in peregrine blood suggests an additional crude oil source after DWH oil spill. The analyses of PAHs in Peregrine Falcon blood provide a convenient repeatable method, in conjunction with ongoing banding efforts, to monitoring crude oil contamination in this avian predator.
Wang et al. (Wang, Sojinu et al. Citation2015) measured the concentrations of selected persistent organic pollutants (POPs) such as parent and halogenated PAHs and HPAHs and polybrominated diphenyl ethers (PBDEs) in indoor dust (ID) and indoor cockroach samples collected from Shenzhen, South China. Biota-dust accumulation factors (BDAFs) were computed and utilized to quantify targeted pollutant bioaccumulation in ID and cockroaches. Generally, halogenated compounds have higher BDAFs when compared to non-halogenated compounds. There are significant differences (p < 0.05) between the BDAFs of non-halogenated POPs (PAHs) and halogenated POPs (HPAHs and PBDEs). Correlation analysis of target pollutants’ levels in ID and cockroaches were also conducted. The correlation coefficients for PAHs are less than 0.2 (p > 0.5) suggesting no significant relationship exists for PAHs between ID and cockroaches. In contrast, significant correlations exist for halogenated POPs (HPAH and PBDE) between ID and cockroaches (correlation coefficients >0.94, p < 0.0001). Based on this, the potential of cockroaches to be used as reliable bioindicators of POPs contamination of indoor environments was preliminarily evaluated. Our results indicate that indoor cockroaches may be useful bioindicator of indoor pollution for HPAHs and PBDEs contaminations.
Van der Wat et al.(Van der Wat and Forbes Citation2015) used Lichens as biomonitors for semi-volatile organic air pollutants, particularly PAHs, as a result of their ability to respond to air pollutants at different levels, their slow growth rate, their longevity and their ability to indicate the presence and the concentrations of PAHs. Consequently, there has been a recent global trend in environmental analytical research to utilize lichens in this way, with Soxhlet and ultrasound-assisted extractions being the most common analyte extraction techniques. A wide range of total PAH concentrations has been determined in lichens from different environments, although phenanthrene, fluoranthene, naphthalene and pyrene tend to dominate the PAH profiles, with higher 2-ring and 3-ring PAH concentrations than 6-ring. In order to facilitate inter-study comparison, there is a need to develop a reproducible, sensitive analytical method for organic pollutants in lichens.
Synder et al. (Snyder, Vestal et al. Citation2014) studied the concentration of PAHs in Coquina on a sandy beach shoreline impacted by a marine oil spill and West et al. (West, O’Neill et al. Citation2014) evaluated background levels and sources of PAHs in naturally spawned embryos of Pacific herring (Clupea pallasii) from Puget Sound, Washington, USA.
Rahmanpour et al. (Rahmanpour, Farzaneh Ghorghani et al. Citation2014) also determine PAHs in four fish species from different trophic levels in the Persian Gulf Snyder, West, O’Neill et al.(West, O’Neill et al., Citation2014) evaluated background levels and sources of PAHs in naturally spawned embryos of Pacific herring (Clupea pallasii) from Puget Sound, Washington, USA
Walker et al. (Walker and MacAskill Citation2014) used mussels as monitoring tools they measured water quality in Sydney Harbour during a large scale, multi-year remediation project of the Sydney Tar Ponds (STPs); one of Canada’s most contaminated sites. Chemical contaminants were measured in blue mussels (Mytilus edulis) in Sydney Harbour, which were used as monitoring tools to assess the spatio-temporal distribution of PAHs; polychlorinated biphenyls (PCBs); metals (As, Cd, Cu, Hg, Pb, Zn) and lipid content during baseline and 3 years of remediation. The overall spatio-temporal distribution of chemicals in mussels was also compared to contaminants in other marine indicators (e.g., sediment, water and crab tissue). Measured metal concentrations in mussels showed some minor temporal variability (4 years), but these did not appear to be directly related to remediation activities, with the highest concentrations of As, Hg and Zn measured at reference stations. Most measured contaminants showed stable or potentially decreasing concentrations during the study, except Pb and Zn. Individual PAH compounds were mostly undetected during baseline and remediation, except for fluoranthene and pyrene.
Concentrations of fluoranthene in mussels and deep water samples were moderately related. Generally, PCBs were undetected (0.05 μg g-1), except during year 2 remediation at some near-field stations. Contaminants measured during this study were at much lower concentrations than previously reported in other studies of mussels in Sydney Harbour and eastern Canada. This is likely due to the ongoing natural recovery of Sydney Harbour and to a lesser extent because of the environmental mitigation protection measures implemented during remediation activities at the STPs. The lack of detection of most individual PAHs and PCBs, plus relatively low bio-accumulation of metals observed during baseline and remediation attest to the effectiveness of using mussels as monitoring tools for environmental quality.
Other biological organism used for biomonitoring include aquatic Mosses (Augusto, Máguas et al. Citation2013, Godzik, Szarek-Łukaszewska et al. Citation2014), Grass goby fish specimens (Zosterisessorophiocephalus (Barhoumi, Clérandeau et al. Citation2014), Teleost fish (Oryzias latipes(Seemann, Peterson et al. Citation2015), Mosses (Ciesielczuk, Olszowski et al. Citation2012, Harmens, Foan et al. Citation2013, Çabuk, Kiliç et al. Citation2014, Foan, Domercq et al. Citation2015, Capozzi, Giordano et al. Citation2016), Mussels (Fernández-Tajes, Flórez et al. Citation2011, Balcioğlu, Aksu et al. Citation2014, Lacroix, Richard et al. Citation2015) Mussels Perna pern, Yoshimine, Carreira et al. 2012, Santiago, Molisani et al. Citation2016), Fish (Saija, Mangano et al. Citation2016, Salvo, Severino et al. Citation2016), Sea snake (Hydrophis cyanocinctus), (Heydari Sereshk and Riyahi Bakhtiari Citation2014) (Lapemis curtus) DNA adducts(Gavina, Yao et al. Citation2014),DNA damage(Teixeira, Pra et al. Citation2012, Martins, Costa et al. Citation2013, Michel, Bourgeault et al. Citation2013), sperm DNA integrity in nonsmoking workers.(Jeng, Pan et al. Citation2013),Urine in Coke oven worker (Ivan, Hudák et al., Citation2011, Pesch, Spickenheuer et al. Citation2011),Urine of Spanish adult (Bartolomé, Ramos et al. Citation2015),Urine of adult in Isreal(Berman, Goldsmith et al. Citation2013), Urine of Children in Saudi Arabia(Alghamdi, Alam et al. Citation2015), Urine of adult in Ghana(Feldt, Fobil et al., Citation2014), Urine of non-smoking Polish pregnant women(Polanska, Hanke et al. Citation2014), Urine of US population (Alshaarawy, Elbaz et al. Citation2016),Urine of children and adults from Afghanistan(Hemat, Wittsiepe et al. Citation2012), Urine samples from 111 healthy in Bejing(Gong, Zhu et al. Citation2015), Blue mussels (Mytilus spp.) (Nahrgang, Brooks et al. Citation2013, Lacroix, Richard et al., Citation2015), Mussel Mytilus galloprovincialis (Moschino, Delaney et al. Citation2011, Viñas, Franco et al. Citation2012, Azis, Yelmiza et al. Citation2016), Blood, serum (Ranzi, Fustinoni et al. Citation2013), Blood and semen (Song, Chen et al. Citation2013).
Others biological organisms used are Pine needles (Ratola, Amigo et al. Citation2011, Ratola, Amigo et al. Citation2012, Tomashuk, Truong et al. Citation2012, Ratola, Amigo et al. Citation2013, Fernández-Varela, Ratola et al. Citation2015, Chropeňová, Gregušková et al. Citation2016, De Nicola, Concha Graña et al. Citation2016), Ruditapes philippinarum (Mollusca: Bivalvia) (Moschino, Delaney et al. Citation2012, Sacchi, Mouneyrac et al. Citation2013), Wild mud snails (Bullacta exarata) (An, Zheng et al., Citation2012), Lumbricus rubella (Jones, Spurgeon et al. Citation2008), Mussels from the Mytilus edulis complex (Olenycz, Sokołowski et al. Citation2012, Martins, Costa et al. Citation2012), Small mammals(Al Sayegh Petkovšek, Kopušar et al. Citation2015), Lichene(Studabaker, Krupa et al. Citation2012, Loppi, Pozo et al. Citation2015, Paoli, Munzi et al. Citation2015, Van der Wat and Forbes, Citation2015, Puy-Alquiza, Reyes et al. Citation2016), Octopus (Octopus vulgaris)(Semedo, Oliveira et al. Citation2014), Lolium multiflorum ‘Lema’ (Rinaldi, Domingos et al. Citation2012), Earthworms (Krauss, Wilcke et al. Citation2000, Pérès, Vandenbulcke et al. Citation2011, Moreno, Cañizares et al. Citation2015, Nam, Jeon et al., Citation2015, Zhang, Yang et al. Citation2015), Serum of Saudi children(Al-Daghri, Alokail et al. Citation2014), scallop Chlamys farreri (Jin, Pan et al. Citation2014), . Caged mussels (Mytilus galloprovincialis)(Mzoughi and Chouba Citation2012), Senegal sole (Solea senegalensis) (Oliva, Perales et al. Citation2012), Quercus ilex leaves (Papa, Bartoli et al. Citation2012), Oysters (Crassostrea rhizophorae) (Ramdine, Fichet et al. Citation2012) Oyster (Saccostrea glomerata) (Edge, Johnston et al. Citation2012, Hong, Jia et al., Citation2016), Lymphocytes(Coronas, Vaz Rocha et al. Citation2016), Sea turtle(Camacho, Boada et al. Citation2012), Atlantic cod (Gadus morhua) (Bratberg, Olsvik et al. Citation2013), Marine sea urchin Paracentrotus lividus (Angioni, Cau et al. Citation2014), Eucalyptus rostrata (Rodriguez, Wannaz et al. Citation2012), Chinook salmon (Blanc, Holland et al. Citation2010),Swineliver and ileum tissue (Peters, Wickstrom et al. Citation2016), Limpet (Patella vulgata) (Bartolomé, Bustamante et al. Citation2011) and Flathead mullet (Mugil cephalus) (Bozcaarmutlu, Sapmaz et al. Citation2015).
10. Bioremediation
Bioremediation is an effective strategy for cleaning up organic contaminants, such as PAHs and volatile organic compounds (VOCs). Bioremediation has been used in several countries for many years and consists in the use of microorganisms (bacteria and fungi) to transform contaminants into inert substances, which is a result of the microbial activity from biochemical processes. Recently a wide range of remediation processes have emerged, such as an the improved use of surfactants, the chemotactic mobilization of bacterial inoculants, the selective biostimulation at pollutant interfaces, rhizoremediation and electrobioremediation, which have increased the bioavailability of PAHs (Wilson and Jones Citation1993, Shukla, Singh et al. Citation2010, Ortega-Calvo, Tejeda-Agredano et al. Citation2013, Sungthong, van West et al. Citation2015). PAH-contaminated sites can be remediated using the microorganisms—algae, bacteria, and fungi individually or in combination (Cerniglia Citation1992).
Environmental awareness has resulted in development of regulatory measures that aim to straighten past mistakes and protect the environment from future contamination and exploitation Table . However, much consideration and research needs to go into the decision-making process for an effective clean-up of a particular contaminated site. Each technology developed has its advantages and limitations for the treatment of specific contaminants. Bioremediation and phytoremediation in association with microbes are innovative technologies having a potential to alleviate numerous environmental pollution problems. Owing to its dominant presence in contaminated sites, the application of the fungi in bioremediation is well documented.
Table 5. Carcinogenic classifications of selected PAHs by specific agencies(Kim, Jahan et al. 2013)
Many studies have been conducted regarding the degradation of PAHs. One of the technologies that have been widely used is bioremediation due to its relatively low cost and greater efficiency for those compounds with structural complexity. Advanced bioremediation implies that biotic agents are more efficient in degrading the contaminants completely.
10.1. PAH fungal bioremediation
Fungi as bioremediation agents have been the subjects of scientific research due to the potential use of their unspecific oxidative enzymes. However, some non-white-rot fungi, mainly belonging to the Ascomycota and Zygomycota phylum, have demonstrated their potential in the enzymatic transformation of environmental pollutants, thus overcoming some of the limitations observed in white-rot fungi with respect to growth in neutral pH, resistance to adverse conditions and the capacity to surpass autochthonous microorganisms. Despite their presence in so many soil and water environments, little information exists on the enzymatic mechanisms and degradation pathways involved in the transformation of hydrocarbons by these fungi.
Marco et al. (Marco-Urrea, García-Romera et al. Citation2015) reviewed the bioremediation potential of non-ligninolytic fungi with respect to chlorinated hydrocarbons and PAHs and also shows known conversion pathways and the prospects for future research. Winquist et al. (Winquist, Björklöf et al. Citation2014) developed a fungal bioremediation method that could be used for soils heavily contaminated with persistent organic compounds, such as PAHs. Sawmill soil, contaminated with PAHs, was mixed with composted green waste (1:1) and incubated with or without fungal inoculum. The treatments were performed at the laboratory and field scales. In the laboratory scale treatment (starting concentration 3500mgkg-1, sum of 16 PAH) the high molecular weight PAHs were degraded significantly more in the fungal-inoculated microcosms than in the uninoculated ones. In the microcosms inoculated with Phanerochaete velutina, 96% of 4-ring PAHs and 39% of 5- and 6-ring PAHs were removed in three months. In the uninoculated microcosms, 55% of 4-ring PAHs and only 7% of 5- and 6-ring PAHs were degraded. However, during the field scale (2t) experiment at lower starting concentration (1400mgkg-1, sum of 16 PAH) the % degradation was similar in both the P.velutina-inoculated and the uninoculated treatments: 94% of the 16 PAHs were degraded in three months. In the field scale experiment the copy number of gram-positive bacteria PAH-ring hydroxylating dioxygenase genes was found to increase 1000 fold, indicating that bacterial PAH degradation also played an important role.
Reyes-César et al. (Reyes-César, Absalón et al. Citation2014) isolated nine native non-ligninolytic fungal strains from Maya crude oil-contaminated soil and selected based on their ability to grow and use crude oil and several PAHs as carbon source, for their application to PAH removal in soil. The fungi were identified by PCR amplification of intergenic transcribed sequences regions and microbiological techniques, and results showed them to be part of the genera Fusarium, Neurospora, Aspergillus, Scedosporium, Penicillium, Neosartorya and Talaromyces. A primary selection of fungi was made in minimal medium plates, considering the tolerance to different concentrations of PAHs for each strain. The radial extension rate exhibited significant differences (plt; 0.05) from 200 to 1,000 mg of PAHs mixture l-1. A secondary selection of Aspergillus terreus, Talaromyces spectabilis, and Fusarium sp. was achieved based on their tolerance to 2,000 mg f a mixture of Phenanathrene and Pyrene kg-1 of soil in a solid-state microcosm system for 2 weeks. The percentage of PAH removal obtained by the three strains was approximately 21% of the mixture.
10.2. Bacterial bioremediation
Bacterial laccase CueO from Escherichia coli and bacterial laccase CotA from Bacillus subtilis can oxidize PAHs; however, their application in the remediation of PAH-contaminated soil mainly suffers from a low oxidation rate and copper dependence (Zeng, Zhu et al. Citation2016).
Huijie et al.(HuiJie, CaiYun et al. Citation2011) collected sediment samples from a mangrove area in Fugong, Longhai, Fujian Province of China. A total of 53 strains of PAH-degrading bacteria were isolated from the mangrove sediments, consisting of 14 strains of phenanthrene (Phe), 13 strains of pyrene (Pyr), 13 strains of benzo[a]pyrene (Bap) and 13 strains of mixed PAH (Phe + Pyr + Bap)-degrading bacteria. All of the individual colonies were identified by 16S rDNA sequencing. Based on the information of bacterial PCR-DGGE profiles obtained during enrichment batch culture, Phe, Pyr, Bap and mixed PAH-degrading consortia consisted of F1, F2, F3, F4 and F15 strains, B1, B3, B6, B7 and B13 strains, P1, P2, P3, P5 and P7 strains, M1, M2, M4, M12 and M13 strains, respectively. In addition, the degradation ability of these consortia was also determined. The results showed that both Phe and mixed PAH-degrading consortia had the highest ability to degrade the Phe in a liquid medium, with more than 91% being degraded in 3 days. But the biodegradation percentages of Pyr by Pyr-degrading consortium and Bap by Bap-degrading consortium were relatively lower than that of the Phe-degrading consortium. These results suggested that a higher degradation of PAHs depended on both the bacterial consortium present and the type of PAH compound. Moreover, using the bacterial community structure analysis method, where the consortia consist of different PAH-degrading bacteria, the information from the PCR-DGGE profiles could be used in the bioremediation of PAHs in the future.
10.3. PAH phytoremediation
In recent years, there is the emergence of green technologies using biological energies involving microorganisms (bioremediation) and plants (phytoremediation). Research has focused on phytoremediation and many have presented this technology as the process ideally combining efficiency, low cost and environmental acceptance(Ouvrard, Leglize et al. Citation2014).
The phytoremediation of PAHs is the use of plants and associated soil microorganisms in terms of reducing the concentrations or toxic effects of these contaminants in the environment. Phytoremediation is an emerging technology for the clean-up of sites contaminated with hazardous chemicals. Phytoremediation is a kind of technology which can thoroughly remove organic pollutants from contaminated sites through phytoextraction, phytodegradation, and rhizosphere biodegradation. phytoremediation has the advantages of relatively lower cost, smaller environmental disturbance, and reuse of natural resources(Wei, Zhou et al. Citation2006). Although there is little evidence to prove that PAHs from soils are accumulated considerably in plants’ parts, there is a lot of evidence that in soils vegetated with grasses and legumes, a significant dissipation of PAHs occurs. Namely, the primary mechanism controlling this process is the rhizospheric microbial degradation, where soil microbial populations use organic compounds as carbon substrates for its growth. This is usually stimulated by roots exudates. The final result of this process is the breakdown and eventual total mineralization of the contaminants. Plants use sunlight and carbon dioxide as the sources of energy and carbon. Plants’ roots absorb a range of natural and anthropogenic toxic compounds for which they have developed some extraordinary detoxification mechanisms. Plants are thus used for cleaning up contaminated soils, leading further to the concept of phytoremediation (He and Chi Citation2016). Plant species was considered to be a significant factor influencing the efficiency of phytoremediation. Phytoremediation has been demonstrated to be a viable cleanup alternative for soils contaminated with petroleum products.
Dealing with soil contaminated with persistent organic pollutants (POP) is an increasing concern amplified by both regulatory constraints and the dramatic impact of human activities on the soil resource. The most used management options are treatments which totally eradicate the toxic compounds targeted. When possible, environmental-friendly processes should be used, and recent years have seen the emergence of green technologies using biological energies involving microorganisms (bioremediation) and plants (phytoremediation). Research has focused on phytoremediation and many have presented this technology as the process ideally combining efficiency, low cost and environmental acceptance. However, the applicability of phytoremediation on soils contaminated by bio-recalcitrant organic compounds, such as PAH, has not yet proved as successful as expected. The mechanism responsible for the phytoremediation of contaminated soil is thought to be an increase in microbial activity. Supporting this hypothesis, the population levels of contaminant-degrading bacteria and the potential of soil to degrade contaminants typically increases during phytoremediation. Phytoremediation is thus an emerging technology for the remediation of PAHs(Sun, Cang et al. Citation2010)
Xiao et al.(Xiao, Liu et al. Citation2015) conducted pot-culture experiments to assess the phytoremediation potential of five ornamental plant species on PAH-contaminated soils obtained from the Shengli Oil Field, one of the largest oil fields in China. The removal rate of eight PAHs (fluoranthene, pyrene, benzo(a)anthracene, chrysene, benzo(b)fluoranthene, benzo(k)fluorant-hene, benzo(a)pyrene and dibenzo(a,h)anthracene) was examined after 60, 120 and 150 days. The results showed that Fire Phoenix and Medicago sativa Linn effectively reduced the PAH content in contaminated soil. After a 150-day pot-culture experiment, the removal rates for eight target PAHs were 86.56% and 90.67% of fluoranthene, 93.27% and 79.53% of pyrene, 100% and 100% of benzo(a)anthracene, 100% and 100% of chrysene, 100% and 100% of benzo(b)fluoranthene, 100% and 100% of benzo(k)fluorant-hene, 99.53% and 99.6% of benzo(a)pyrene, 100% and 100% of dibenzo(a,h)anthracene respectively from Fire Phoenix and Medicago sativa Linn. The removal rates for these two plant types were significantly higher than those of other tested ornamental plants. The results suggested that Fire Phoenix and Medicago sativa Linn should be applied to the phytoremediation of PAH-contaminated soil. Furthermore, soil physicochemical properties (e.g., the alkali-hydrolyzable nitrogen, available phosphorus and cation exchange capacity) was also measured in the soil before and after plant growth and indicated that Fire Phoenix is better able to improve soil physicochemical properties (mainly about available phosphorus and cation exchange capacity).
Moreno et al. (Moreno, Cañizares et al., Citation2015) set up a meso-scale pilot plant to test the efficiency of a bioremediation scheme applied to marine sediments contaminated by heavy metals and hydrocarbons. The experiment was implemented for three years in two stages using two remediation agents: plants (Paspalum vaginatum and Tamarix gallica) and earthworms (Eisenia fetida). DNA and RNA-based methodologies were applied to elucidate the dynamics of the bacterial population and were related to improving biological and chemical conditions of the sediments. Bioremediation strategies were successful in removing pollutants from the contaminated sediments and specialization within the bacterial community related to the type of contamination present was detected in the different stages of the process. The highest response of Gram-positive PAH-degraders to the contamination was detected at the beginning and after the first stage of the experiment, corresponding to the uppermost values of degradation.
Chen et al. (Chen, Tan et al. Citation2016) conducted a 2-year greenhouse study to remediate an actual wastewater-irrigated soil contaminated with PAHs. The remediation methods included microbial remediation, phytoremediation, and microbe-assisted phytoremediation. The maximum PAH removal (96.4%) and PAH mineralization, were obtained by interplanting ryegrass with Seduce alfredii with regular re-inoculation with Microbacterium sp. KL5 and Candida tropicalis C10 in the co-contaminated soil. The plants shoots were harvested at a 4-month interval. After 2 years, the concentrations of 16 individual PAHs were reduced to below the limit of Chinese soil quality standard for agricultural use (grade II, pH 6.5-7.5), and the metal concentrations in ryegrass shoots were below the Chinese national limit for animal feeds (GB13078-2001). The exogenous microbes gradually disappeared with time, and thus a 2-month re-inoculation interval was applied for a purpose to maintain high cell density and activity of the inoculants. KL5 introduction increased soil enzyme activity, plant growth, PAH removal and metal phytoextraction, while C10 promoted soil enzyme activity and removal of high-molecular-weight PAHs. Interplanting with S. alfredii reduced metal concentrations in ryegrass tissues. Ryegrass showed stronger rhizosphere effects than S. alfredii did.
Alagic et al. (Alagić, Maluckov et al. Citation2015) concluded that the main challenge in PAH phytoremediation is to improve the performances of plants, rhizospheric microorganisms and more basic research and knowledge on natural detoxification mechanisms look into.
Luo et al. (Luo, Wang et al.Citation 2014) studied the removeal and transformation of seven high molecular weight PAHs, namely benz[a]anthracene, benzo[b]fluoranthene, benzo[k]fluoranthene, benzo[a]pyrene, dibenzo[a,h]anthracene, indeno[1,2,3-c,d]pyrene and benzo[g,h,i]perylene, by a freshwater microalga Selenastrum capricornutum under gold and white light irradiation. The two light sources did not result in significant differences in the biodegradation of the selected PAHs in live algal cells, but white light was more effective in promoting photodegradation than was gold light in dead cells. The removal efficiency of seven PAHs, as well as the difference between live and dead microalgal cells, was PAH compound-dependent. Benz[a]anthracene and benzo[a]pyrene were highly transformed in live and dead algal cells, and dead cells displayed greater transformation levels than live cells. Further investigation comparing the transformation of single PAH compound, benzo[a]pyrene, by S. capricornutum and another green microalgal species, Chlorella sp., demonstrated that the transformation in dead cells was similar, indicating the process was algal-species independent. Dead algal cells most likely acted as a photosensitizer and accelerated the photodegradation of PAHs. Bisht,et al. (Bisht, Pandey et al. Citation2015) effectected bioremediation of PAHs using rhizosphere technology.
Biswas et al. (Biswas, Sarkar et al. Citation2015) reviewed the role of clay/modified clay minerals in hydrocarbon biormediation through interaction with microbial agents in specific scenarios. The vision is on a faster, more efficient and cost-effective bioremediation technique using clay-based products. This review also proposes future research directions in the field of clay modulated microbial degradation of hydrocarbons. Chirakkara et al. (Chirakkara and Reddy Citation2015) investigated plant species considered suitable for phytoremediation of mixed contaminated soil. Mixed contaminated soil was prepared by spiking silty clay soil with organic contaminants (naphthalene and phenanthrene) and heavy-metal contaminants (lead, cadmium, and chromium). Twelve species of plants were grown in pots filled with contaminated soil. Plants grown in uncontaminated soil were used as control. Results showed that five plant species (sunflower, oat plant, rye grass, tall fescue, and green gram) were able to survive the mixed contaminated conditions. The oat plant had better growth characteristics and minimal stress signs in mixed contaminated soil than the other species. Even though sunflower plants did not show the best growth characteristics, the maximum contamination removal from the soil was achieved by these plants. Exchangeable heavy metals were also reduced in the soil that was planted with sunflower plants compared with contaminated unplanted soil
Eskandary (Eskandary, Hoodaji et al. Citation2014) investigated bioremediation of PAHs from oil-contaminated soils. Bacillus licheniformis and Bacillus mojavensis, were inoculated in rhizospheric soil with Festuca aroundiacea in a greenhouse study. The concentration of PAHs in the rhizosphere of soil inoculated with both bacteria were significantly (p < 0.05) reduced compared to the non-inoculated soil. PAH concentrations in shoots were largely independent of those in soil, roots, or rhizosphere soil. In the soil inoculated with both bacteria, the concentration of PAHs in roots and shoots of the plant was less than for other treatments. Results showed that the plant-promoted biodegradation was the predominant ontribution to remediation of soil PAHs.
García-Delgado (García-Delgado, Yunta et al. Citation2015) investigated the effect of three spent Agaricus bisporus substrate (SAS) application methods on bioremediation of soil polluted with PAH from close to a shooting range with respect natural attenuation (SM). The remediation treatments involve (i) use of sterilized SAS to biostimulate the inherent soil microbiota (SSAS) and two bioaugmentation possibilities (ii) its use without previous treatment to inoculate A. bisporus and inherent microbiota (SAS) or (iii) SAS sterilization and further A. bisporus re-inoculation (Abisp). The efficiency of each bioremediation microcosm was evaluated by: fungal activity, heterotrophic and PAH-degrading bacterial population, PAH removal, Pb mobility and soil eco-toxicity. Biostimulation of the native soil microbiology (SSAS) achieved similar levels of PAH biodegradation as SM and poor soil detoxification. Bioaugmented microcosms produced higher PAH removal and eco-toxicity reduction via different routes. SAS increased the PAH-degrading bacterial population, but lowered fungal activity. Abisp was a good inoculum carrier for A. bisporus exhibiting high levels of ligninolytic activity, the total and PAH-degrading bacteria population increased with incubation time. The three SAS applications produced slight Pb mobilization (<0.3%). SAS sterilization and further A. bisporus re-inoculation (Abisp) proved the best application method to remove PAH, mainly BaP, and detoxify the multi-polluted soil.
He et al. (He and Chi, Citation2016) compared the ability of four submerged aquatic plants to facilitate the remediation of sediments polluted with PAHs. Materials and methods were surface sediments (top 0–15 cm) collected from the Haihe River, Tianjin, China, and spiked with a mixture of phenanthrene and pyrene (about 15 mg kg−1 dry sediment of each PAH). Four submerged aquatic plant species (Hydrilla verticillata, Myriophyllum verticillatum, Vallisneria spiralis, and Potamogeton crispus L.) were used for the remediation experiment. The experiment lasted for 54 days. Plant length and fresh weight, and root morphology were measured. Concentrations of phenanthrene and pyrene in plants and sediments were analysed. Sediment redox potential and PAH-degrading bacterial population were also determined. Results and discussion: During a 54-day experiment, more PAHs were dissipated in planted sediments than in unplanted sediments. At the end, dissipation ratios of phenanthrene and pyrene were 48.0–85.3 and 45.7–82.4% in planted sediments but only 33.9 and 38.3% in unplanted sediments. Dissipation ratios of the PAHs were significantly different among the four plant species and were the highest in sediments with V. spiralis. Mass balance calculation showed that plant accumulation of phenanthrene and pyrene accounted for less than 1.0 and 6.9% of the dissipation increments, respectively. Among the plants tested, there were significantly positive correlations between PAH dissipation ratios and sediment redox potentials. Conclusions: The results suggested that different from terrestrial plant, enhanced dissipation of PAHs by submerged aquatic plants might be mainly attributed to differences in root oxygenation capabilities. Furthermore, root oxygenation may be a useful tool in screening plants for efficient remediation of PAH-polluted sediments. Extension of these results to field-aged PAH-contaminated sediments needs further investigation.
Liu et al. (Liu, Meng et al. 2014) conducted a 54-day-long study to investigate the effect of plant density (260, 780, 1300 and 2080plantsm-2) of Vallisneria spiralis on the remediation of sediments contaminated by PAHs. Dissipation ratios of phenanthrene and pyrene in sediments were initially the highest in treatment of 2080plantsm-2. However, after a 54-day incubation, no statistical difference was observed in the dissipation ratios under different planting density treatments (p;0.05) except lower dissipation ratio of phenanthrene in treatment of 780plantsm-2. Compared with the unplanted sediments, the dissipation ratios of phenanthrene and pyrene in planted sediments increased by 15.2-21.5% and 9.1-12.7%. Considering the sustainability of the ecosystem, lower plant density (e.g., 260plantsm-2) should be a better selection for phytoremediation of PAHs. Mass balance calculation indicated that plant accumulation accounted for less than 0.39% of the dissipation increment. Furthermore, dissipation ratio of PAHs was positively related to PAH-degrading bacterial population, suggesting that microbial degradation played a major role in the V. spiralis-promoted remediation. The redox potential, a signal of oxygen in sediments, was measured. Positive redox potentials were found in sediments with V. spiralis as a result of oxygen released by roots. Moreover, sediment redox potential positively correlated with PAH-degrading bacterial population. Considering high oxygen demand of PAHs catabolism and reduced conditions in unplanted sediments, it can be concluded that the enhanced dissipation of PAHs is mainly related to oxygen released by roots.
Meng et al. (Meng and Chi Citation2015) investigated the effect of plant density of Potamogeton crispus L. on the remediation of sediments contaminated by PAHs, a 54-day experiment with four plant densities(642, 1,604, 2,567 and 3,530 plants/m2)was conducted. The results showed higher plant density with slower plant growth rate. Surface area per plant was the most sensitive root parameter to plant density. At the end of the 54-day experiment, planting P. crispus enhanced the dissipation ratios of phenanthrene and pyrene in sediments by 6.5%—26.2% and 0.95%—13.6%, respectively. The dissipation increment increased with increasing plant density. Plant uptake accounted for only a small portion of the dissipation increments. Furthermore, P. crispus could evidently improve sediment redox potentials, and strong positive correlations between root surface area and the redox potential as well as between the redox potentials and the dissipation ratios of phenanthrene and pyrene were obtained, indicating that the oxygen released by the roots of P. crispus might be the main mechanism by which P. crispus enhanced the dissipation of PAHs in sediments.
Some other plants used for phytoremediation include: alfalfa (Medicago sativa L.) with tall fescue (Festuca arundinacea Schreb.) (Sun, Fu et al. Citation2011, Hamdi, Benzarti et al. Citation2012), Spartina alterniflora(Watts, Ballestero et al. Citation2006), Potamogeton crispus L.(Meng and Chi Citation2015), Vallisneria spiralis(Liu, Meng et al. 2014), Hydrilla verticillata, Myriophyllum verticillatum, Vallisneria spiralis, and Potamogeton crispus L(He and Chi, Citation2016, He and Chi Citation2016), fescue (Festuca arundinacea) and switchgrass (Panicum virgatum) Cofield, Schwab et al. Citation2007), oat plant, rye grass, tall fescue, and green gram(Chirakkara and Reddy Citation2015) tomato plants(Storey, Ashaari et al. Citation2014), Juncus subsecundus(Zhang, Rengel et al. Citation2012), willow (Salix × Aureo-Pendula CL ‘j1011’) (Sun, Xu et al. Citation2016), ornamental plant Tagetes patula(Sun, Zhou et al. Citation2011), maize(Zea mays L.) (Zamani, Hajabbasi et al. Citation2016) and Kandelia obovata seedlings(Li, Liu et al. Citation2016)
10.4. Biosufactant bioremediation
It is well known that many bacteria growing on alkane produce biosurfactants to increase the bioavailability of PAHs. Biosurfactants are small, detergent-like molecules with a hydrophilic head and a lipophilic tail. They help in dissolution of PAHs. For example, biosurfactant produced by a Pseudomonas aeruginosa growing on phenanthrene or naphthalene was shown to increase the apparent solubility of these PAHs (De′ ziel, Paquette et al. Citation1996). Naturally occurring surfactants have been known to enhance PAH-removal from soil by improving PAH solubilization thereby increasing PAH-microbe interactions (Cameotra and Bollag Citation2003). Surfactant-enhanced remediation (SER) has been widely applied in decontaminating PAH-polluted soil (Liang, Guo et al. Citation2016). Surfactant-enhanced remediation is a powerful technology for soil clean-up. However, some drawbacks, such as its high cost and the secondary contamination caused by flushing agents, have hampered this technology and its commercial application has been largely limited to North American and European countries (Long, Li et al. Citation2016)
Xia et al. (Xia, Du et al. Citation2014) isolated a novel biosurfactant-producing Pseudomonas sp. WJ6 to degrade a wide range of n-alkanes and PAHs. Production of lipopeptide biosurfactant was observed in all biodegradable studies. These lipopeptides were purified and identified by C18 RP-HPLC system and electrospray ionization-mass spectrometry. Results of structural analysis showed that these lipopeptides generated from different hydrocarbons were classified to be surfactin, fengycin and lichenysin. Heavy-oil sludge washing experiments demonstrated that lipopeptides produced by Pseudomonas sp. WJ6 have 92.46% of heavy-oil washing efficiency. The obtained results indicate that this novel bacterial strain and its lipopeptides have great potentials in the environmental remediation and petroleum recovery
Blyth et al. (Blyth, Shahsavari et al. Citation2015) determined if a biosurfactant derived from the leaves of the Australian red ash (Alphitonia excelsa) would enhance bioremediation of a heavily PAH-contaminated soil and to determine how the microbial community was affected. Results of GC-MS analysis showed that the extracted biosurfactant was significantly more efficient than the control in regards to the degradation of total 16 US EPA priority PAHs (78.7% degradation compared to 62.0%) and total petroleum hydrocarbons (TPH) (92.9% degradation compared to 44.3%). Furthermore the quantification of bacterial genes by qPCR analysis showed that there was an increase in the number of gene copies associated with Gram positive PAH-degrading bacteria. The results suggest a commercial potential for the use of the Australian red ash tree as a source of biosurfactant for use in the accelerated degradation of hydrocarbons. Cai et al.(Cai, Wang et al. Citation2014) used a static aeration biopile process to bioremediate PAH-contaminated soil using four different approaches for treating about 30 m3 of soil at a former oil-producing site. The four treatments investigated were as follows: (i) fertilizer plus bulking agent (FB); (ii) fertilizer, bulking agent, plus Tween 80 (FBT); (iii) fertilizer, bulking agent, Tween 80, plus fungi agent (FBTF); and (iv) fertilizer, bulking agent, bacterial inoculum, plus fungi agent (FBBF). After bioremediation for 320 days, the total amount of 16 PAHs ranged from 4.14 to 5.31 mg/kg in the final soil, removal rates ranging from 75.5% to 81.5%. The sum concentration of seven carcinogenic PAHs decreased down to 0.15 mg/kg. The values of the total toxicity equivalence concentrations for 16 PAHs ranged from 0.014 to 0.068 mg/kg. The removal rates of the 16 PAHs in these four different treatments decreased in order FBBF; FBT; FBTF; FB.
Bezza et al. (Bezza and Nkhalambayausi Chirwa Citation2015) produced a novel biosurfactant by strain CN5, identified as Paenibacillus dendritiformis isolated from creosote contaminated wood treatment plant soil. The biosurfactant produced by the strain was identified as lipopeptidal after attenuated total reflectance Fourier transform infrared (ATR-FTIR), thin-layer chromatography (TLC), and (LC–MS/MS) analysis. The MS/MS analysis of the biosurfactant showed that it has an amino acid sequence of Cys-Gly-Ala-Gly-Ile-Asn-Leu and a long chain fatty acid of molecular mass 522 Da. The biosurfactant exhibited 74% and 82% emulsification index (E24) with hexane and cyclohexane respectively, and it showed high thermal, pH and saline stability over a wide range of temperature, pH and salinity. The ability of the biosurfactant to desorb PAH from spiked soils and motor oil from spiked sands was tested in batch experiments and it desorbed more than 96% of phenanthrene, 83% of pyrene from the contaminated soil in 5 days and 81% of heavy used motor oil sludge from the spiked sands in 24 h. This suggests the potential application of the biosurfactant for the removal of PAHs and motor oil sludge from contaminated media and enhancing their bioremediation by increasing bioavailability and possible application of the biosurfactant for enhanced oil recovery.
Vecino et al. (Vecino, Rodríguez-López et al. Citation2015) developed a decontamination technique based on the utilization of a lipopeptide biosurfactant extracted from corn steep liquor to eliminate PAHs from sewage sludge. High concentrations of PAHs were used during experiments observing that 408.3 mg/kg of naphthalene was almost completely mobilized and biodegraded, only 1.7% of naphthalene remained in the sewage sludge, whereas anthracene and pyrene were reduced up to 51.7 and 69.4%, respectively. The biodegradation of PAHs was fitted to several kinetic models (zero- and first-order kinetic models), observing good correlation coefficient values when biodegradation was described by the first-order kinetic model. Experimental results suggest that biosurfactant extracted from corn steep liquor may have great potential, as an ecofriendly washing agent, for the treatment of sewage sludge contaminated with PAHs. Therefore, in situ application of natural biosurfactants may be considered to be a good remediation alternative as they are not hazardous for water and soil organisms.
Bezza and Chewan (Bezza and Chirwa Citation2014) investigated a microbial surfactant for its potential to enhance bioavailability and, hence, the biodegradation of PAHs contaminated soil. Phenanthrene, a 3-ring polycyclic aromatic hydrocarbon (PAH), was chosen as a model target compound. The bioavailability and biodegradation tests were performed in aqueous and soil-slurry microscosms .The rhamnolipid biosurfactant used in this study was extracted from culture supernatants after growth of Pseudomonas aeruginosa BP9 strain in nitrogen-limited mineral salts medium. Solubilisation of phenanthrene in aqueous solution was enhanced by 400 mg of the rhamnolipid per litre increasing more than 19 folds. Phenanthrene aqueous phase biodegradation experiments were done with an initial concentration of 200 mg/L and showed 92% mineralization in 6 days with a rhamnolipid concentration of 400 mg/L, in comparison to the 27% mineralization of the other microcosm with no rhamnolipid amendment. Which accelerated the biodegradation rate, by increasing the bioavailabilty and by shortening the lag phase. This shows the potential application of the rhamnolipid in stimulating in-situ and ex-situ enhanced bioremediation of PAHs contaminated media.
10.5. Rhizoremediation
Wide range of innovations in recent years, such as improved use of surfactants, the chemotactic mobilization of bacterial inoculants, the selective biostimulation at pollutant interfaces, rhizoremediation and electrobioremediation have increased the bioavailability of PAHs. Bacterial and fungal community composition in the rhizosphere of plants contributes a lots in the remediation and degradation of PAHs (Storey, Ashaari et al., Citation2014). Rhizoidal microbes in combination other strategies can provide interesting possibilities for bioremediation of PAH-polluted soils (Tervahauta, Fortelius et al. Citation2009) and high amount of root mucilage, selectively enriched the PAHs degrading bacteria population in rhizosphere(Sun, Belcher et al. Citation2015).
The integration of various strategies (such as rhizomisation) into practical remediation protocols would be beneficial to the bioremediation industry, as well as improve the quality of the environment (Ortega-Calvo, Tejeda-Agredano et al., Citation2013).
Liu et al.(Liu, Xiao et al. 2014)investigated the rhizosphere effect of a special phytoremediating species known as Fire Phoenix on the degradation of PAHs, including changes of the enzymatic activity and microbial communities in rhizosphere soil. The study showed that the degradation rate of σ8PAHs by Fire Phoenix was up to 99.40% after a 150-day culture. The activity of dehydrogenase (DHO), peroxidase (POD) and catalase (CAT) increased greatly, especially after a 60-day culture, followed by a gradual reduction with an increase in the planting time. The activity of these enzymes was strongly correlated to the higher degradation performance of Fire Phoenix growing in PAH-contaminated soils, although it was also affected by the basic characteristics of the plant species itself, such as the excessive, fibrous root systems, strong disease resistance, drought resistance, heat resistance, and resistance to barren soil. The activity of polyphenoloxidase (PPO) decreased during the whole growing period in this study, and the degradation rate of σ8PAHs in the rhizosphere soil after having planted Fire Phoenix plants had a significant (R2=0.947) negative correlation with the change in the activity of PPO. Using an analysis of the microbial communities, the results indicated that the structure of microorganisms in the rhizosphere soil could be changed by planting Fire Phoenix plants, namely, there was an increase in microbial diversity compared with the unplanted soil. In addition, the primary advantage of Fire Phoenix was to promote the growth of flora genus Gordonia sp. as the major bacteria that can effectively degrade PAHs.
Other plant’s rhizosphere used are alfalfa rhizosphere(Shrestha, Anderson et al. Citation2015), Sorghum bicolor (L.) Moench(Muratova, Golubev et al. Citation2009), Paspalum vaginatum and Tamarix gallica(Moreno, Cañizares et al., Citation2015), macrophyte Potamogeton crispus L.(Meng, Huang et al. Citation2015), sweet flag rhizosphere (Marecik, Chrzanowski et al. Citation2015), Fire Phoenix(Liu, Xiao et al. 2014), Echinacea purpurea, Festuca arundinacea Schred, Fire Phoenix (a combined F. arundinacea), and Medicago sativa L.(Liu, Dai et al. Citation2015) and Oryza sativa(Su and Zhu Citation2008)
10.6. Microbial/bacterial bioremediation
Bioremediation by microbial degradation is often employed and to make this process efficient, natural and cost-effective materials can serve as supportive matrices. Clay/modified clay minerals are effective adsorbents of PAHs/VOCs, and readily available substrate and habitat for microorganisms in the natural soil and sediment. However, the mechanism underpinning clay-mediated biodegradation of organic compounds is often unclear, and this requires critical investigation. Many biodegradation studies have focused on survival of isolated bacteria to increase the bacteria population and subsequently enhance the efficiency of PAH biodegradation. However, there is limited research on enhancing the performance of isolated bacteria through reinoculation. Mao et al.(Mao and Guan Citation2016) studied
Guo et al.(Guo, Gong et al. Citation2016) demonstrated the variations in bioavailability remaining in industrial and agricultural soils contaminated by PAHs after bioremediation. After inoculation of Mycobacterium sp. and Mucor sp., PAH biodegradation was tested on a manufactured gas plant (MGP) soil and an agricultural soil. PAH bioavailability was assessed before and after biodegradation using solid-phase extraction (Tenax-TA extraction) and solid-phase micro-extraction (SPME) to represent bioaccessibility and chemical activity of PAHs, respectively. Only 3- and 4-ring PAHs were noticeably biodegradable in the MGP soil. PAH biodegradation in the agricultural soil was different from that in the MGP soil. The rapidly desorbing fractions (Frap) extracted by Tenax-TA and the freely dissolved concentrations of 3- and 4-ring PAHs determined by SPME from the MGP soil decreased after 30 days biodegradation; those values of the 5- and 6-ring PAHs changed to a lesser degree. For the agricultural soil, the Frap values of the 3- and 4-ring PAHs also decreased after the biodegradation experiment. The Tenax-TA extraction and the SPME have the potential to assess variations in the bioavailability of PAHs and the degree of biodegradation in contaminated MGP soils. In addition, Tenax-TA extraction is more sensitive than SPME when used in the agricultural soil.
Gong et al. (Gong, Xu et al. Citation2015) designed a remediation technique using both soil washing and microbial degradation to remove PAHs from contaminated soil. PAH biodegradation by inoculation of Mycobacterium sp. was first tested. The effectiveness of washing agents (Tween 80 solution, biodiesel, and a two-phase partition system (TPPS) was then evaluated with column experiments. Third, the combination of TPPS washing and microbial degradation was studied. PAH bioavailability before and after biodegradation and the joint remediation was also assessed using hydroxypropyl-β-cyclodextrin (HPCD) extraction. Only phenanthrene and anthracene were noticeably biodegradable when the soil was inoculated with Mycobacterium sp. TPPS containing 2 % (v/v) biodiesel and 2.5 % (w/v) Tween 80 was used as the washing agent for the joint remediation test because it gave higher PAH extractions than Tween 80 solution with lower doses, and there were fewer residues in the soil. Joint TPPS washing and microbial degradation gave a total PAH removal of 92.6 %, which was much higher than the results from either the biodegradation or washing experiments alone. Removals of all high molecular weight (HMW) PAHs were improved. Bioavailable concentrations of all PAHs decreased significantly after the joint remediation process, indicating that there were reduced risks from all PAHs. The results demonstrate that the combination of TPPS washing and microbial degradation is a useful and innovative process for remediation of PAH-contaminated soils
Rodiric et al. (Rodríguez-Morgado, Gómez et al. Citation2015) studied in the laboratory the bioremediation effects over a 100-day period of three edaphic biostimulants (BS) obtained from sewage sludge (SS) and from two different types of chicken feathers (CF1 and CF2), in a soil polluted with three PAH (phenanthrene, Phe; pyrene, Py; and benzo(a)pyrene, BaP), at a concentration of 100mgkg-1 soil. We determined their effects on enzymatic activities and on soil microbial community. Those BS with larger amounts of proteins and a higher proportion of peptides (<300daltons), exerted a greater stimulation on the soil biochemical properties and microbial community, possibly because low molecular weight proteins can be easily assimilated by soil microorganisms. The soil dehydrogenase, urease, β-glucosidase and phosphatase activities and microbial community decreased in PAH-polluted soil. This decrease was more pronounced in soils contaminated with BaP than with Py and Phe. The application of the BS to PAH-polluted soils decreased the inhibition of the soil biological properties, principally at 7 days into the experiment. This decrease was more pronounced in soils contaminated with BaP than with Py and Phe and was higher in polluted soils amended with CF2, followed by SS and CF1, respectively.
Ouvrard (Ouvrard, Leglize et al., Citation2014) reviewed and discussed the overall question of PAH status in soil and their potential for treatment. The limits and applicability of bioremediation technologies are discussed, and the specific beneficial effect of plants is objectively evaluated with a special interest to processes which lead to rhizoattenuation. Given the PAH high affinity to soil organic matter, availability is the main limitation to phytoremediation. In this context, bioavailability quantification remains an issue as well as the characterization of the recalcitrant fraction.
Waszak et al. (Waszak, Da Cunha et al. Citation2015) developed a bioremediation methodology for the pollutant benzo[a]pyrene (B[a]P), which belongs to the group of PAHs. The potential use of a microbial consortium with Pseudomonas aeruginosa, Candida albicans, Aspergillus flavus, and Fusarium sp. for bioremediation was assessed. To confirm the pollutant reduction, quantifications of the samples were performed via (GC-MS). The contamination was prepared with a soil previously contaminated with B[a]P at the concentration of 3.74 mg kg-1. The microbial consortium was added (16 μL g-1), and samples were incubated for 42 days in an oven at 35 °C. The microbial growth curves showed representative differences between the samples in the presence and absence of the pollutant, demonstrating the possibility of bioremediation process. The final quantification of soil showed a mean concentration of 1.29 mg kg-1, showed that 65.51∈±∈0.95% of the pollutant was degraded, which is an important and representative performance
Moh Kamil et al. (Mohd-Kamil, Hussain et al. Citation2014) evaluated the effects of reinoculation on the performance of Sphingobacterium spiritivorum in degradation of phenanthrene contaminated sand. Experiments were performed in three different reactors. Inoculation was performed once (day 0) in reactor 1. In reactor 2, inoculation was performed twice (day 0 and day 5). The bacteria was isolated from reactor 2 and inoculated into reactor 3. The study results show reactor 3 having the highest degradation rate (13.61 mg/kg/day) and percentage removal (95.36 percent). In contrast, without reinoculation in reactor 1, 68.93 percent of phenanthrene was removed. Thus, the performance of S. spiritivorum in phenanthrene degradation can be enhanced through reinoculation
10.7. Other methods of bioremediation
Tuncal et al. (Tunçal and Uslu Citation2015) treated mechanically dewatered industrial sludge (MDIS) using pure and silver-doped thin films (TFs) grown on quartz substrates. TFs were prepared using a sol-gel dip coating technique. The resulting films were annealed at 450°C for 3h and characterized by X-ray diffraction (XRD), scanning electron microscopy (SEM), transmission electron microscopy (TEM), atomic force microscopy (AFM) and X-ray photoelectron spectroscopy (XPS). Mixtures that were homogeneous in the UV A (380nm) and UVvis (450nm) regions of the electromagnetic spectrum were used as the irradiation source. The results revealed that illumination with different wavelengths helps to generate well-separated e-/h+ pairs, resulting in a decrease in the recombination rate. An electron transfer chain model was also developed using the experimental results. The performance of the applied method was evaluated by observing variations in the sludge bound water content (SBWC), volatile solids removal rate (VSR), and the consumed and generated energy fluxes through endergonic and exergonic reactions. After treatment, SBWC was reduced from 65%±1% to 39% ±1 and the highest VSR was measured to be 27±0.1mg VSS cm-2 h-1. The consumed and recovered energy fluxes were 960±151 and 412±26Jg-1 VSremoved, respectively. Raw sludge and polychlorinated biphenyls (∑15PCB) and PAHs (∑16PAH) concentrations were 4356.82±22μgkg-1 and 446.25±4.8μgkg-1, respectively. The ∑15PCB and ∑16PAH concentrations in the treated sludge samples were 129.86±22μgkg-1 and 34.85±1.3μgkg-1, respectively.
Sherafatmand et al.(Sherafatmand and Ng Citation2015) explored a sediment microbial fuel cell (SMFC) to bioremediate PAHs in water originated from soil. The results showed consistent power generations of 6.02 ± 0.34 and 3.63 ± 0.37 mW/m2 under an external resistance of 1500 Ω by the aerobic and anaerobic SMFC, respectively. Although the power generations were low, they had relatively low internal resistances (i.e., 436.6 ± 69.4 and 522.1 ± 1.8 Ω for the aerobic and anaerobic SMFC, respectively) in comparison with the literature. Nevertheless, the significant benefit of this system was its bioremediation capabilities, achieving 41.7%, 31.4% and 36.2% removal of naphthalene, acenaphthene and phenanthrene, respectively, in the aerobic environment and 76.9%, 52.5% and 36.8%, respectively, in the anaerobic environment. These results demonstrated the ability of SMFCs in stimulating microorganisms for bioremediation of complex and recalcitrant PAHs.
Garcia-Delgado (García-Delgado, Alfaro-Barta et al. Citation2015) used different bioremediation strategies to remediate soils impregnated with creosote contain high concentrations of PAH based on natural attenuation, biochar application, wheat straw biostimulation, Pleurotus ostreatus mycoremediation, and the novel sequential application of biochar for 21 days and P. ostreatus 21 days more. Soil was sampled after 21 and 42 days after the remediation application. The efficiency and effectiveness of each remediation treatment were assessed according to PAH degradation and immobilization, fungal and bacterial development, soil eco-toxicity and legal considerations. Natural attenuation and biochar treatments did not achieve adequate PAH removal and soil eco-toxicity reduction. Biostimulation showed the highest bacterial development but low PAH degradation rate. Mycoremediation achieved the best PAH degradation rate and the lowest bioavailable fraction and soil eco-toxicity. This bioremediation strategy achieved PAH concentrations below Spanish legislation for contaminated soils (RD 9/2005). Sequential application of biochar and P. ostreatus was the second treatment most effective for PAH biodegradation and immobilization. However, the activity of P. ostreatus was increased by previous biochar application and PAH degradation efficiency was increased.
10.8. Electroremediation
This technique, mainly used for heavy metal extraction, applies the electric current to promote the movement of contaminants. Nowadays the application of this technique alone or combined with other techniques as for example Fenton or bioremediation is taking fine results to PAHs removal (Ortega-Calvo, Tejeda-Agredano et al., Citation2013, Lin, Ning et al., Citation2016, Qin, Liu et al. Citation2016). Although the PAHs soil decontamination by means of the electric field is in an initial stage, many researchers have demonstrated the treatment effectiveness. They described the foremost principles to carry out the electroremediation of soils contaminated with PAHs, just like the different alternatives to improve the electroremediation of PAHs and also the new methodologies of PAHs removal by using hybrid technologie (Pazos, Rosales et al. Citation2010)
Hahladakis et al.(Hahladakis, Lekkas et al. Citation2014) focused on the sequential application of a chelating agent (citric acid) followed by a surfactant in the simultaneous electroremediation of real contaminated sediments from toxic metals and PAHs. Furthermore, the efficiency evaluation of two innovative non-ionic surfactants, commercially known as Poloxamer 407 and Nonidet P40, was investigated. The results indicated a removal efficacy of approximately 43% and 48% for the summation of PAHs (SUM PAHs), respectively for the aforementioned surfactants, much better than the one obtained by the use of Tween 80 (nearly 21%). Individual PAHs (e.g. fluorene) were removed in percentages that reached almost 84% and 92% in the respective electrokinetic experiments when these new surfactants were introduced. In addition, the combined-enhanced sequential electrokinetic treatment with citric acid improved dramatically the removal of Zn and As, compared to the unenhanced run, but did not favor the other toxic metals examined. Since no improvement in metal removal percentages occurred when Tween 80 was used, significant contribution to this matter should also be attributed to the solubilization capacity of these innovative, in electrokinetic remediation, non-ionic surfactants.
Examples of areas and places where bioremediation have been used in degrading the contaminants completely (Biswas, Sarkar et al., Citation2015) include : coal-tar contaminated soil (Antizar-Ladislao, Beck et al. Citation2007), creosote contaminated soil(Bezza and Nkhalambayausi Chirwa Citation2016), contaminated sewage sludge (Cai, Mo et al. Citation2007, Zhang, Xu et al. Citation2016), coal tar contaminated soil (Lors, Ryngaert et al. Citation2010), contaminated marine sediment (Lu, Li et al. Citation2012), hydrocarbon-contaminated soil (Mao, Luo et al. Citation2012), contaminated soil through composting (Sayara, Borràs et al. Citation2011), petroleum sludge bioremediation(Bezza, Beukes et al. Citation2015), soils polluted by municipal solid waste(Jobin, Coudert et al. Citation2016), soil spiked with model mixtures of petroleum hydrocarbons (Ivshina, Kostina et al. Citation2016)and pyrene contaminated soil (Li, Wang et al. Citation2016)
11. Biodegradations of PAHs
Abundant microbes including bacteria, fungi, or algae are capable of biodegrading PAHs(Luo, Chen et al. Citation2014). Free cells (FC) and immobilized cells (IC) are also capable of biodegradation of PAHs (Partovinia and Naeimpoor Citation2014). The biodegradation of PAHs by microorganisms is the subject of many excellent reviews (Cerniglia Citation1992, Peng, Xiong et al., Citation2008, Haritash and Kaushik Citation2009, Jiang, Yang et al., Citation2014, Ouvrard, Leglize et al., Citation2014, Biswas, Sarkar et al., Citation2015, Rodríguez-Morgado, Gómez et al., Citation2015) and the biodegradation of PAHs composed of three rings (low molecular weight) is well documented. Low molecular weight PAHs are more degradable than the high molecular weight compounds (Xu, Li et al. Citation2012).
Microbial degradation is one of the promising methods in removing up PAH-contaminated environments and it is a promising soil remediation strategy for PAHs frequently polluting some post-industrial environments (Thomas, Lorgeoux et al. Citation2016). Although PAH may undergo adsorption, volatilisation, photolysis, and chemical degradation, microbial degradation is the major degradation process. PAH degradation depends on the environmental conditions, number and type of the microorganisms, nature and chemical structure of the chemical compound being degraded
There are three types of microbial PAH degradation: complete mineralization, co-metabolic transformation and nonspecific oxidation (Cerniglia Citation1984). Aerobic microorganism-mediated biodegradation of PAHs is another process of biodegradation. To effectively decontaminate polluted sites it is desirable that there is complete mineralisation to prevent accumulation of metabolites. Complete mineralisation of different PAHs has been described for some bacterial strains belonging to different phylogenetic groups (Cerniglia and Heitkamp, Citation1989, Cerniglia Citation1992).
11.1. Bacteria biodegradation
Bacteria species are known to degrade PAHs and they are isolated from soil or sediments and many bacterial strains from different genera are known to degrade PAHs(Deora, Giri et al. Citation2013). It has been observed that PAH degradation in soil is dominated by bacterial strains belonging to taxonomic groups such as Sphingomonas, Burkholderia, Pseudomonas, Mycobacterium, sphingomonads, Sphingobium, Burkholderia fungorum(Al-Thukair and Malik Citation2016), Novosphingobium and Sphingopyxis(Takeuchi Citation2001, Johnsen, Winding et al. Citation2002)
The biochemical pathways of bacterial degradation of low-molecular-mass PAHs such as naphthalene, phenanthrene and anthracene have been elucidated in detail (Nekhavhambe, van Ree et al., Citation2014) Figures and .
Figure 4. Fungal degradation pathway of Phenanthrene using Irpex lacteus(Cajthaml, Mode et al., Citation2002).
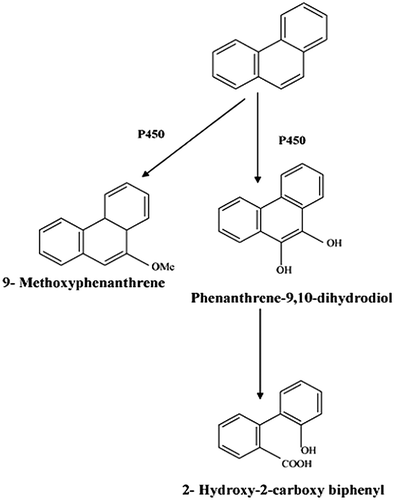
Figure 5. Proposed anaerobic biotransformation pathway of phenanthrene by sulfate-reducing bacteria.
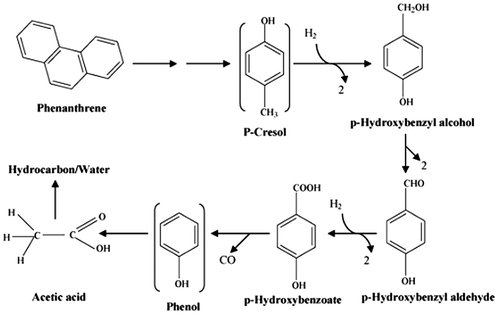
A number of bacterial species are known to degrade PAHs and most of them are isolated from contaminated soil or sediments (Sauret, Tedetti et al. Citation2015). Marinobacter, Enterobacter and Dethiosulfatibacter play important roles in PAH degradation in mangrove sediment (Muangchinda, Pansri et al. Citation2013). Pseudomonas aeruginosa (Liang, Song et al. Citation2014, Mishra and Singh Citation2014, Karimi, Habibi et al. Citation2015, Mangwani, Kumari et al. Citation2015, Ghosh and Mukherji Citation2016), Pseudomonas putida(Rodrigues, Nogueira et al. Citation2013), Pseudomonas gessardii (Huang, Wu et al. Citation2016) Pseudomons fluoresens(Portet-Koltalo, Ammami et al. Citation2013, Zhang, McPhedran et al. Citation2015), Mycobacterium spp(Tejeda-Agredano, Mayer et al. Citation2014, Muangchinda, Chavanich et al. Citation2015, Rodgers-Vieira, Zhang et al., Citation2015, Tauler, Vila et al. Citation2015)., Haemophilus spp.(Haritash and Kaushik Citation2009), Rhodococcus spp.(Isaac, Martínez et al., Citation2015), Paenibacillus spp., rhizobacterial strain Ensifer meliloti P221(Muratova, Pozdnyakova et al. Citation2014), Pseudomonas aeruginosa (Karimi, Habibi et al. Citation2015), consortia-A and N(Kuppusamy, Thavamani et al., Citation2016), Staphylococcus warneri and Bacillus pumilus(Moscoso, Teijiz et al. Citation2012) and Pseudomonas xanthomarina(Sopeña, Laiz et al. Citation2014) are some of the commonly studied PAH-degrading bacteria. Others are Sphingomonas (α-Proteobacteria), Commamonas and Oxalobacteria (β-Proteobacteria), and Xhanthomonas (γ-Proteobacteria) (Tejeda-Agredano, Gallego et al. 2013).
The genus Pseudomonas has been the subject of much research as regards its ability to degrade PAHs (Deschˆenes, Lafrance et al. Citation1996, Tang, Feng et al. Citation2013, Sopeña, Laiz et al., Citation2014, Xia, Du et al., Citation2014, Isaac, Martínez et al. Citation2015, Karimi, Habibi et al. Citation2015, Mangwani, Kumari et al., Citation2015, Mineki, Suzuki et al. Citation2015, Ghosh and Mukherji Citation2016), and genus Sphingobacterium spiritivorum (Mohd-Kamil, Hussain et al., Citation2014) is often used in degradation of phenanthrene contaminated sand. Bacillus stratosphericus, Bacillus subtilis, Bacillus megaterium, and Pseudomonas aeruginosa have been used in degradation of PAHs(Bezza and Nkhalambayausi Chirwa Citation2016).Sphingomonas paucimobilis has been used to degrade anthraquinone, phenanthrene and benzo[a]pyrene (Peng, Xiong et al., Citation2008, Partovinia and Naeimpoor Citation2013, Rodgers-Vieira, Zhang et al. Citation2015, Waigi, Kang et al. Citation2015), Micrococcus sp. PHE3 and Sphingobium sp.(Zhang, Wang et al. 2013) were also use to degrade anthraquinone and phenanthrene. Sphingomonas degrade three to four-ring PAHs extensively (Zhou, Luan et al. Citation2008).
In the last decade, research pertaining specifically to the bacterial biodegradation of PAHs composed of more than three rings has been advanced significantly. The bacterial biodegradation of PAHs with more than three rings, which are often referred to in the biodegradation literature as high-molecular-weight (HMW) PAHs, is the subject of mini review by Kanaly and Harayama. The relationship between PAH environmental persistence and increasing numbers of benzene rings is consistent with the results of various studies correlating environmental biodegradation rates and PAH molecule size. For example, reported half-lives in soil and sediment of the three-ring phenanthrene molecule may range from 16 to 126 days while for the five-ring molecule BaP they may range from 229 to 1,400 days.
PAHs are biodegraded/biotransformed into less complex metabolites, and through mineralization into inorganic minerals, H2O, CO2 (aerobic) or CH4 (anaerobic) and rate of biodegradation depends on pH, temperature, oxygen, microbial population, degree of acclimation, accessibility of nutrients, chemical structure of the compound, cellular transport properties, and chemical partitioning in growth medium. Bacteria most often oxidize PAHs to cis-dihydrodiols by incorporation of both atoms of an oxygen molecule. The cis-dihydrodiols are further oxidized, first to the aromatic dihydroxy compounds (catechols) and then channeled through the ortho- or meta cleavage path-ways (Cerniglia Citation1984, Smith Citation1990).
The initial step in the aerobic degredation of a PAH molecule by bacteria occurs via oxidation of the PAH to a dihydrodiol by a multicomponent enzyme system. These dihydroxylated intermediates are processed through either an ortho cleavage type of pathway or a meta cleavage type of pathway, leading to central intermediates such as protocatechuates and catechols, which are further converted to tricarboxylic acid cycle intermediates fig . Amid early reports which described the microbial oxidation of HMW PAHs (Gibson and Subramanian Citation1984), Gibson et al. (Gibson, Venkatanayarana et al. Citation1975) showed that treatment of a Beijerinckia sp. with N-methyl-N9-nitro-N-nitrosoguanidine created a mutant(strainB8/36) which oxidized BaPandbenz[a]anthracene to dihydrodiols after growth with succinate plus biphenyl. Two products of BaP metabolism were identified as cis-9,10dihydroxy-9,10-dihydrobenzo[a]pyrene and cis-7,8-dihydroxy7,8-dihydrobenzo[a]pyrene. The main metabolites isolated from benz[a]anthracene metabolism were identified as cis-1,2-dihydroxy-1,2-dihydrobenz[a]anthracene and cis-8,9- and cis-10,11dihydrodiols (Jerina Citation1984). Biodegradation of fluoranthene and BaP was also reported in 1974 (Barnsley Citation1974). In the case of fluoranthene, concentrations near aqueous solubility were shown to be degraded by stationary-phase cultures of Pseudomonas strain NCIB 9816 grown on succinate and salicylate (Kanaly and Harayama Citation2000),
Marecik et al. (Marecik, Chrzanowski et al., Citation2015) made an attempt to incorporate a soil-isolated bacterial consortium capable of degrading hydrocarbons into the sweet flag rhizosphere in order to introduce the plant bacteria combination into a wetland environment. The colonisation of the rhizosphere occurred after a diesel oil degradation trial, where the plant was inoculated with the bacterial consortium. Both plant and bacterial growth were monitored. The 16S rRNA gene sequences method was used to verify the presence of the soil-isolates in the plants internal tissues and TTC-dehydrogenase measurements were employed to assess the microbial activity. The incorporation of bacterial cells into the sweet flag rhizosphere resulted in an increased diesel oil removal efficiency (by 50%) and enhanced bacterial and plant growth. Afterwards, the colonised sweet flag rhizomes were planted within a lake shoreline to evaluate the long-term prevalence of microorganisms in the natural environment. The bacterial DNA of the oil-degrading consortium species was detected in the harvested plants even after 8 months.
Alharbi, Zayed et al.(Alharbi, Zayed et al. Citation2014) determined if bacteria can be successfully used to bioremediate PAH pollution as an alternative to physical and chemical methods. The bacteria used in this study were isolated from three PAH polluted sites of Mediterranean Sea, off Alexandria, Egypt. The study was devoted to the isolation of bacteria that can degrade three low molecular weight PAH sand to determine the effect of pH on this process. Nine phenanthrene-degrading, seven-naphthalene-degrading and eight anthracenedegrading bacteria were isolated by enrichment from the marine water sample. All of the isolates grew on the PAHs (phenanthrene, anthracene and naphthalene) at varying rates and utilised them as sole source of carbon and energy. (Margesin, Moertelmaier et al. 2013) evaluated the ability of four cold-adapted bacterial strains to degrade n-alkanes (C12-C22), aromatic hydrocarbons (phenol) and PAHs (anthracene, pyrene) at low temperatures. All four strains belonged to the phylum Actinobacteria and were identified as Rhodococcus erythropolis (strain BZ4), Rhodococcus cercidiphyllus (strain BZ22), Arthrobacter sulfureus (strain BZ73) and Pimelobacter simplex (strain BZ91). The strains could grow over a temperature range of 1-30°C and showed catechol-1, 2-dioxyogenase activity. One of the strains, R. erythropolis BZ4, degraded all of the compounds tested. The strain utilised n-alkanes and high amounts of phenol (7.5mM), anthracene and pyrene (50mgl-1) at 15°C. P.simplex BZ91 degraded n-alkanes as well as up to 7.5mM phenol; phenol degradation was observed at 1-30°C. R. cercidiphyllus BZ22 fully degraded C12 (700mgl-1) at 1-20°C, while degradation of C16 and C20 was delayed and lower compared to C12 degradation. A.sulfureus BZ73 was the best phenol degrader and utilized up to 12.5mM phenol; phenol degradation occurred over a temperature range of 1-25°C. Such strains are promising candidates for low temperature (low-energy) treatment of industrial wastewaters contaminated with hydrocarbons.
Bisht et al. (Bisht, Pandey et al. Citation2014) isolated eight endophytic bacteria from the roots of Populus deltoides growing in non-contaminated sites at natural vegetation of Garhwal Himalayas, Uttarakhand, India. Among these eight, only SBER3 isolate was able to metabolise wide range of PAHs and other hydrocarbon used in the study i.e. anthracene, naphthalene, benzene, toluene and xylene on minimal salt basal medium (MSB) as sole source of carbon and energy. It was identified as Bacillus sp. on the basis of 16S rDNA sequence. Furthermore, quantitatively Bacillus sp. SBER3 was able to produced 22 μg ml−1 of IAA after 4 days and solubilized 0.96 μg ml−1 of available phosphorus respectively after 120 h. In addition, Bacillus sp. SBER3 also produced siderophore and 1 aminocyclopropane-1-carboxylate (ACC) deaminase. Along with these traits, SBER3 under in vitro condition inhibited the phytopathogenic fungi Rhizoctonia solani, Macrophomina phaseolina, Fusarium oxysporum and Fusarium solani with (percent growth inhibition) PGI of 60%, 61.5%, 64.3% and 12%, respectively. Microscopic examination under the influence of Bacillus sp. SBER3 revealed abnormalities of mycelia structure in case of R. solani, F. oxysporum and M. phaseolina. Although, mean growth rate and survival under varying osmotic stress regime were also evaluated under in vitro condition. Interestingly, in liquid culture medium Bacillus sp. SBER3 reduced appreciable amount i.e. 83.4% and 75.1% of anthracene and naphthalene, respectively after 6 days of incubation. Notwithstandingly, isolate SBER3 proved to be a competent rhizobacteria in rhizosphere niche in treatments T1 (Sterile soil + plant cuttings + bacterization) and T2 (sterile soil + anthracene + plant cuttings + bacterization). Rhizoremediation potential of Bacillus sp. SBER3 was demonstrated in PAHs contaminated soil model system. Significant enhancement in shoot, root length, root and shoot biomass including stem girth of P. deltoides with respective to control was also recorded and concurrently endophytic isolate Bacillus sp. SBER3 degraded 45.6% of PAH in soil model system after 120 days as determined by HPLC analysis.
Zang et al.(Zhang, McPhedran et al., Citation2015) used Pseudomonas fluorescens and Pseudomonas putida to determine their ability to biodegrade aromatic compounds including treatments considering the impacts of external carbon and iron addition. Results showed that with added carbon P. fluorescens and P. putida have the capability of biodegrading these aromatics. In the presence of external carbon, gene expression of a functional PAH-ring hydroxylating dioxygenase (PAH-RHDα) was determined through reverse transcription real-time PCR, suggesting active degradation of OSPW aromatic compounds. Although no significant classical NAs removal was observed during this process, toxicity was reduced by 49.3% under optimal conditions. OSPW toxicity was eliminated with the combination of ozonation at a dose of 80 mg/L followed by biodegradation, indicating that it is a promising combined OSPW treatment approach for the safe discharge to the aquatic environment.
Morris et al. (Morris, Gissibl et al. Citation2014) studied the aerobic degradation of PAH via oxygenase reactions and developed enzyme involved in anaerobic PAH degradation, the dearomatizing 2-naphthoyl-CoA reductase, was isolated and characterized. A PCR-based functional assay was developed to detect microorganisms that have the ability to anaerobically degrade naphthalene, as a model for larger PAH. The degenerative oligonucleotide probes introduced here amplified a highly conserved region of the gene encoding 2-naphthoyl-CoA reductase (Ncr) in numerous sulfate-reducing pure cultures and environmental enrichments. The assay provides the first molecular tool for monitoring the anaerobic degradation of a model PAH.
Yu et al. (Yu, Yao et al. Citation2014) isolated indigenous microorganisms capable of degrading PAHs and investigated the efficiency of PAHs removal. Seven PAH-degrading strains were isolated with the ability to grow on naphthalene, phenanthrene, pyrene and fluorene. They belonged to the genus Pseudomonas, Bacillus and Rhodococcus. The strain having the highest degrading capacity for each PAH was selected by the screening test. The removal efficiency of PAHs was found to be in the order of naphthalene > fluorene > phenanthrene > pyrene. The kinetics of PAHs degradation was then followed by liquid chromatography determination and the results showed it conforms to a first-order reaction kinetic model.
Salam et al. (Salam, Obayori et al. Citation2014) studied a gram-negative facultatively methylotrophic bacterium putatively identified as Methylobacterium mesophilicum strain RD1 displayed extensive degradative ability on used engine oil (SAE 40W) in liquid cultures. The rate of degradation of used engine oil (1274.85 mg L1) by the isolate, for the first 12 days and the last 9 days were 65 and 40 mg L1 d1, respectively. GC analyses of residual used engine oil revealed that 61.2% and 89.5% of the initial concentration of the used engine oil were degraded within 12 and 21 days. GC fingerprints of the used engine oil after 12 days of incubation showed total disappearance of C15, C23, C24, C25, and C26 hydrocarbon fractions as well as drastic reductions of C13, C14, C16, and PAHs fractions such as C19-Anthracene and C22-Pyrene. At the end of 21 days incubation, total disappearance of C17-pristane, C22-pyrene, one of the C19-anthracene, and significant reduction of C18-phytane (96.8%) fractions were observed. In addition, less than 10% of Day 0 values of medium fraction ranges C13, C14, and C16 were discernible after 21 days. This study has established the potential of Methylobacterium mesophilicum strain RD1 in degradation of aliphatic, aromatic, and branched alkane components of used engine oils.
Liang et al.(Liang, Song et al., Citation2014) isolated facultative anaerobe Pseudomonas sp. JP1 from Shantou Bay, Shantou, China, which could degrade a variety of HMW PAHs. After 40 days cultivation with strain JP1, anaerobic biodegradation rate of benzo[a]pyrene (BaP), fluoranthene, and phenanthrene was 30, 47, and 5%, respectively. Consumption of nitrate as the electron acceptor was confirmed by N-(1-naphthyl) ethylenediamine spectrophotometry. Supplementation of sodium sulfite, maltose, or glycine, and in a salinity of 0–20 ‰ significantly stimulated anaerobic degradation of BaP. Lastly, the anaerobic degradation metabolites of BaP by strain JP1 were investigated using GC/MS, and the degradation pathway was proposed.
Crampon et al.(Crampon, Bureau et al. Citation2014) studied natural biodegradation of seven PAHs by native microorganisms in five soils from Normandy (France) from diffusely polluted areas, which can also pose a problem in terms of surfaces and amounts of contaminated soils. Bioavailability tests using cyclodextrin-based extractions were performed. The natural degradation of low molecular weight (LMW) PAHs was not strongly correlated to their bioavailability due to their sorption to geosorbents. Conversely, the very low degradation of high molecular weight (HMW) PAHs was partly correlated to their poor availability, due to their sorption on complexes of organic matter and kaolinites or smectites. A principal component analysis allowed us to distinguish between the respective degradation behaviors of LMW and HMW PAHs. LMW PAHs were degraded in less than 2-3 months and were strongly influenced by the relative percentage of phenanthrene-degrading bacteria over total bacteria in soils. HMW PAHs were not significantly degraded, not only because they were less bioavailable but also because of a lack of degrading microorganisms. Benzo[a]pyrene stood apart since it was partly degraded in acidic soils, probably because of a catabolic cooperation between bacteria and fungi.
11.2. Fungi biodegradation
There are fewer studies on the degradation of PAHs by fungi than those by bacteria. The initial attack on HMW-PAHs in soil by fungal exoenzymes appears to be more likely than attack by bacterial intracellular enzymes. Fungal exoenzymes have the advantage that they may diffuse to the highly immobile HMW-PAHs. Numerous ligninolytic and non-ligninolytic fungi possess the ability to oxidize PAHs(Cerniglia Citation1992). Ligninolytic fungi oxidise lignin extracellularly by the action of lignin peroxidases, Mn-dependent peroxidase and laccases. These enzymes are unspecific and oxidizea wide variety of organic compounds. Variety of white rot funga genera are capable of oxidizing these PAHs(Cerniglia Citation1984). Coriolopsis gallica was shown to oxidize the different PAHs acenaphthene, phenanthrene, biphenylene, anthracene, 2-methylanthracene, 9-methylanthracene and benzo(a)pyrene(Pickard, Roman et al. Citation1999) Fungi such as Aspergillus (Reyes-César, Absalón et al., Citation2014, Jove, Olivella et al. Citation2016), Martelella sp. strain AD-3(Cui, Ma et al. Citation2014) Curvularia(Balaji, Arulazhagan et al. Citation2014), Drechslera(Balaji, Arulazhagan et al., Citation2014), Fusarium(Mineki, Suzuki et al., Citation2015, Waszak, Da Cunha et al., Citation2015), Selenastrum capricornutum, Lasiodiplodia, Mucor, Penicillium (Atagana Citation2015, Jove, Olivella et al., Citation2016), Rhizopus (Ma, Xu et al. Citation2011, Ma, Lv et al. Citation2016), Rhizoctonia zeae SOL3 fungus (Khudhair, Hadibarata et al. Citation2015) Trichoderma(Cobas, Ferreira et al. Citation2013, Tripathi, Singh et al. Citation2013, Szczepaniak, Cyplik et al. Citation2015, Zafra and Cortés-Espinosa Citation2015, Zafra, Moreno-Montaño et al. Citation2015), and two oilseed-associated fungal genera such as Colletotrichum and Lasiodiplodia (Balaji, Arulazhagan et al., Citation2014) were used to test their efficacy in degradation of PAHs in polluted soil. Cordyceps militaris was used to metabolise 3-5 ring PAHs to their corresponding hydroxides (Mori, Watanabe et al. Citation2015).White rot fungi has been used for the degradation of PAHs (Lee, Jang et al. Citation2014), Crassostrea rhizophorae and Cathorops spixii from the Caroni Swamp (Kanhai, Gobin et al. Citation2014). Trametes versicolor and Pleurotus ostreatus grow on agroindustrial wastes, such as orange peels, and they have the ability to degrade PAHs(Rosales, Pazos et al. Citation2013) and fungal laccase has been used for the degradation of PAHs(Chang, Lee et al. Citation2016). Fungal degradation products of PAHs by lignolytic fungus Irpex lacteus was studied by Cajthaml et al.(Cajthaml, Mode et al. Citation2002) and they found that the major degradation products of anthracene and phenanthrene were anthraquinone and phenanthrene-9,10-dihydrodiol, respectively (Figuers and )
Mineki et al.(Mineki, Suzuki et al., Citation2015) isolated three fungi (strains A, B, and C), which could grow on pyrene as the sole source of carbon, from soils at the side of a freeway, a gas station, and a lake in Japan. Strain A was tentatively classified as members of the Trichoderma/Hypocrea genus, and both strains B and C were classified as belonging to the Fusarium genus on the basis of their base sequence of 18S ribosomal DNA and morphological characteristics. After an incubation period of 7 and 14 days at 28°C in an inorganic Czapek-Dox medium with PAHs as the substrate, both strains A and B assimilated anthracene and fluoranthene, whereas strain C assimilated neither of them. The pyrene-degrading activity and the growth of strain A improved by adding 0.02% yeast extract, 0.1% sucrose, or 0.1% lactose. The pyrene-degrading activity was also found to be more effective at pH 4 rather than at pH 6.5.
Lignolytic fungi too have the property of PAH degradation. Phanerochaete chrysosporium, Bjerkandera adusta, and Pleurotus ostreatus are the common PAH-degrading fungi. White-Rot Fungus Pleurotus pulmonarius F043 for Degradation and Transformation of Fluoranthene (Wirasnita and Hadibarata Citation2016)
Balaji et al. (Balaji, Arulazhagan et al., Citation2014) studied fungal strains capable of secreting extracellular enzymes by utilizing hydrocarbons present in the contaminated soil. Fungal strains were enriched from petroleum hydrocarbons contaminated soil samples collected from Chennai city, India. The potential fungi were isolated and screened for their enzyme secretion such as lipase, laccase, peroxidase and protease and also evaluated fungal enzyme mediated PAHs degradation. Total, 21 potential PAHs degrading fungi were isolated from PAHs contaminated soil, which belongs to 9 genera such as Aspergillus, Curvularia, Drechslera, Fusarium, Lasiodiplodia, Mucor, Penicillium, Rhizopus, Trichoderma, and two oilseed-associated fungal genera such as Colletotrichum and Lasiodiplodia were used to test their efficacy in degradation of PAHs in polluted soil. Maximum lipase production was obtained with P. chrysogenum, M. racemosus and L. theobromae VBE1 under optimized cultural condition, which utilized PAHs in contaminated soil as sole carbon source. Fungal strains, P. chrysogenum, M. racemosus and L. theobromae VBE1, as consortia, used in the present study were capable of degrading branched alkane isoprenoids such as pristine (C17) and pyrene (C18) present in PAHs contaminated soil with high lipase production. The fungal consortia acts as potential candidate for bioremediation of PAHs contaminated environments.
11.3. Algae biodegradation
Intense study of microbial degradation by lignolytic fungi has been vigorusly pusured in the recent past few years. (Cajthaml, Pacakova et al. Citation2001). Selenastrum capricornutum (Obuekwe and Semple Citation2013, Luo, Wang et al. Citation2014, Luo, Chen et al. Citation2014) is a fresh water microalge which has been used in degrading PAHs. Prokaryotic and eukaryotic photoautotrophic marine algae (i.e. cyanobacteria, green algae, and diatoms) are known to metabolize naphthalene to a series of metabolites (Cerniglia Citation1984, Cerniglia and Heitkamp, Citation1989, Cerniglia Citation1992). Green algae almost completely metabolized BaP to dihydrodiols, whereas yellow algae and blue green algae failed in metabolizing the PAH and higher doses of PAHs prove phytotoxic to algae (Warshawsky, Radike et al. Citation1988, Warshawsky, Cody et al. Citation1995) S. costatum and Nitzschia sp. are capable of accumulating and degrading phenanthrene and fluoranthene simultaneously(Hong, Yuan et al. Citation2008).
Selenastrum capricornutum degrade Benz[a]anthracene and benzo[a]pyrene (Luo, Wang et al. Citation2014). Luo et al. (Luo, Wang et al. Citation2014) studied degradation of seven high molecular weight PAHs, namely benz[a]anthracene, benzo[b]fluoranthene, benzo[k]fluoranthene, benzo[a]pyrene, dibenzo[a,h]anthracene, indeno[1,2,3-c,d]pyrene and benzo[g,h,i]perylene, by a freshwater microalga Selenastrum capricornutum under gold and white light irradiation. Prokaryotic and eukaryotic photoautomatic marine algae (cyano bacteria, green algae and diatoms) are known to metabolise naphthalene to various metabolites. Green algae metabolise Bap to dihydrodols. Both eukaryotic and prokaryotic, require the presence of molecular oxygen to initiate the enzymatic attack on the PAHs molecules (Cerniglia Citation1992) and Warshawisky et al. (Warshawsky, Radike et al., Citation1988, Warshawsky, Cody et al., Citation1995) found selenastrum capricornutum a fresh withte algae metabolised BaP to cisdihydrodiols. Chlorella vulgaris, Scenedesmus platydiscus, Scenedesmus quadri- cauda, and Selenastrum capricornutum have been used to degrade fluoranthene and pyrene (Lei, Hu et al. Citation2007).
11.4. Enzyme biodegradation
Enzymes involved in the degradation of PAHs are oxygenase, dehydrogenase and lignolytic enzymes. Fungal lignolytic enzymes are lignin peroxidase, laccase, and manganese peroxidase. They are extracellular and catalyze radical formation by oxidation to destabilize bonds in a molecule. The biodegradation of PAHs has been observed under both aerobic and anaerobic conditions and the rate can be enhanced by physical/chemical pretreatment of contaminated soil. Addition of biosurfactant-producing bacteria and light oils can increase the bioavailability of PAHs and metabolic potential of the bacterial community.
Wong et al.(Wong, Huang et al. Citation2012) studied Laccases from basidiomycetes which are efficient enzymes in the degradation of xenobiotics. In the study, they aimed to provide an industrially relevant expression system for Lentinula edodes laccases, to characterise their enzymatic properties, and to evaluate their potential in bioremediation. Two 1573-bp allelic laccase genes from L. edodes L54 were cloned based on gene models in the genome sequence. A novel upstream consensus (GCTCCGA/CCGGAG) was proposed as an alternative signature sequence for laccases. Both alleles were overexpressed in Pichia pastoris, purified, and verified by zymograms. Kinetic analyses suggested an order of catalytic efficiency of 2,2′-azino-bis(3-ethylbenzothiazoline-6-sulfonic acid); 2,6-dimethoxyphenol; guaiacol; l-3,4-dihydroxyphenylalanine; catechol, and a stable range of working temperature below 40 °C. With the appropriate mediators, 1-hydroxybenzotriazole and 2,2,6,6-tetramethylpiperidine-1-oxyl, the recombinant enzymes could catalyze a 70–100% decolorization of selected dyes and a complete degradation of anthracene. These results laid a solid foundation for the use of L. edodes laccases in bioremediations and for improvement with protein engineering.
11.5. Photodegradation
It has been established that when low molecular weight PAHs absorb photons, degradation is initiated (El-Saeid, Al-Turki et al. Citation2015).
Grossman et al.(Grossman, Stern et al. Citation2016) measured photolysis kinetics of the PAHs anthracene and pyrene in several organic solvents and in water, as well as in miscible and phase-separated aqueous-organic mixtures at atmospherically-relevant wavelengths. Photolysis rate constants generally increased with increasing solvent polarity; photolysis of both PAHs was more than ten times faster in water than in octanol. Local polarity had a much greater effect on PAH photolysis kinetics than changes in PAH absorptivity or singlet oxygen concentrations. Photolysis kinetics in homogeneous aqueous-organic mixtures varied monotonically with 2 organic matter (OM) volume fractions. Kinetics in immiscible (phase-separated) solutions were more complex, with different dependences on OM content observed in stagnant and turbulent solutions. Their results suggest that OM could greatly affect the photochemical lifetimes of PAHs in atmospheric condensed phases such as aerosols, even if the OM does not itself absorb photons.
Zhao et al.(Zhao, Cai et al. Citation2016) concluded that catalyst can effectively catalyse photodegradation of phenanthrene (a model PAHs) under simulated solar light. The pseudo first-order rate constant was determined to be 0.39 h-1, which is about 10 times that of the conventional photocatalyst TiO2. TEM, XRD and XPS analyses indicate that Co-TNTs-600 is a composite nanomaterial containing titanate, anatase and CoO crystals.
11.6. Other methods
Bibi et al. (Bibi, Yasin et al. Citation2015) irradiated CNTs in air at 100 kGy under gamma radiations. The Raman spectroscopy of γ-treated CNTs showed distinctive changes in the absorption bands. The CNTs were mixed with blend of chitosan (Cs)/poly (vinyl alcohol) (PVA) and crosslinked with silane. The chemical reactions between the components affected the position and intensities of the infrared bands. SEM of Cs/CNTs nanocomposite membrane showed the homogeneous dispersion of CNTs in the polymer matrix. The addition of CNTs lowered its swelling in water. Naphthalene (NAPH) was selected as a model compound and its removal was studied using HPLC technique. This membrane showed fast uptake of NAPH and 87% was removed from water within 30 min. The NAPH loaded membrane showed strong chemical interactions and cannot be desorbed. The fast uptake of PAHs and the green nature of this membrane made them suitable candidates for clean-up purposes.
Tunçal and Uslu (Tunçal and Uslu, Citation2015) treated mechanically dewatered industrial sludge (MDIS) using pure and silver-doped thin films (TFs) grown on quartz substrates. TFs were prepared using a sol-gel dip coating technique. The resulting films were annealed at 450°C for 3h and characterized by X-ray diffraction (XRD), scanning electron microscopy (SEM), transmission electron microscopy (TEM), atomic force microscopy (AFM) and X-ray photoelectron spectroscopy (XPS). Mixtures that were homogeneous in the UV A (380nm) and UVvis (450nm) regions of the electromagnetic spectrum were used as the irradiation source. The results revealed that illumination with different wavelengths helps to generate well-separated e-/h+ pairs, resulting in a decrease in the recombination rate. An electron transfer chain model was also developed using the experimental results. The performance of the applied method was evaluated by observing variations in the sludge bound water content (SBWC), volatile solids removal rate (VSR), and the consumed and generated energy fluxes through endergonic and exergonic reactions. After treatment, SBWC was reduced from 65%±1% to 39% ±1 and the highest VSR was measured to be 27±0.1mg VSS cm-2 h-1. The consumed and recovered energy fluxes were 960±151 and 412±26Jg−1 VS removed, respectively. Raw sludge and polychlorinated biphenyls (∑15PCB) and PAHs (∑16PAH) concentrations were 4356.82±22μgkg−1 and 446.25±4.8μgkg-1, respectively. The ∑15PCB and ∑16PAH concentrations in the treated sludge samples were 129.86±22μgkg−1 and 34.85±1.3μgkg−1, respectively.
12. Conclusion and recommendation
This review presents recent literature in PAHs as a result of rapid growth in the awareness of PAHs in petroleum products, food, air, sediment, coal, slurge and waste recently witnessed. In recent times various bioremediation and biodegradation processes are now emerging to transform PAHs to less hazardous or non hazardous compound. These processes are approaches to remove or reduce pollutant in an eco-friendly manner. They also reduce the carcinogenicity, mutagenicity and teratogenicity of PAH in contaminated soil, Water, slurge and food.
Due to the widely varying physical and chemical properties of PAHs, their measurement is often difficult and costly. Methodology for sampling, analysis, and emission estimation will need to be harmonised inorder to properly assess current ambient concentrations, the effect of future control measures, and to refine any further action which may be required to adequately assess their human health impacts.
Additional information
Notes on contributors
Abdulazeez T. Lawal
Abdulazeez T Lawal had a B.Sc. Hon Chemistry from University of Ibadan Nigeria. He left for Loughborough University of Technology United Kingdom where he bagged M.Sc. Analytical Chemistry and Instrumentation. He worked for a while as research chemist with Metallurgical development centre Jos Nigeria and later migrated to Australia and gained admission to Monash University where he bagged a PhD in Analytical chemistry. He was a lecturer B and taught for a while in Charles Sturt University, Wagga Australia. He is currently a Professor of Chemistry in Fountain University Oshogbo, Nigeria.
References
- Abdollahi, S., Raoufi, Z., Faghiri, I., Savari, A., Nikpour, Y., & Mansouri, A. (2013). Contamination levels and spatial distributions of heavy metals and PAHs in surface sediment of Imam Khomeini Port, Persian Gulf, Iran. Marine Pollution Bulletin, 71, 336–345.
- Abramsson-Zetterberg, L., Darnerud, P. O., & Wretling, S. (2014). Low intake of polycyclic aromatic hydrocarbons in Sweden: Results based on market basket data and a barbecue study. Food and Chemical Toxicology, 74, 107–111.
- Achten, C. ,& Hofmann, T. (2009). Native polycyclic aromatic hydrocarbons (PAH) in coals – A hardly recognized source of environmental contamination. Science of The Total Environment, 407, 2461–2473.
- Adam, I. K. U., Miltner, A., & Kästner, M. (2015). Degradation of 13C-labeled pyrene in soil-compost mixtures and fertilized soil. Applied Microbiology and Biotechnology, 99, 9813–9824.
- Adedosu, T. A., Sonibare, O. O., Tuo, J., & Ekundayo, O. (2010). Aromatic Hydrocarbons Distribution in Nigerian Coal and Their Geochemical Significance. Energy Sources, Part A: Recovery, Utilization, and Environmental Effects, 33, 145–155.
- Adetona, O., Simpson, C. D., Li, Z., Sjodin, A., Calafat, A. M., & Naeher, L. P. (2015). Hydroxylated polycyclic aromatic hydrocarbons as biomarkers of exposure to wood smoke in wildland firefighters. Journal of Exposure Science and Environmental Epidemiology, 27, 78–83.
- Adeyeye, S. A. O., Oyewole, O. B., Obadina, A. O., Omemu, A. M., & Oyedele, H. A. (2016). Quality characteristics and chemical safety of fried and smoked West Africa Ilisha (Ilisha africana) from Ibadan, Oyo State, Nigeria. Nutrition and Food Science, 46, 246–258.
- Adeyeye, S. A. O., Oyewole, O. B., Obadina, O., Adeniran, O. E., Oyedele, H. A., Olugbile, A., & Omemu, A. M. (2016). Effect of smoking methods on microbial safety, polycyclic aromatic hydrocarbon, and heavy metal concentrations of traditional smoked fish from Lagos State, Nigeria. Journal of Culinary Science and Technology, 14, 91–106.
- Adisa, A., Jimenez, A., Woodham, C., Anthony, K., Nguyen, T., & Saleh, M. A. (2015). Determination of polycyclic aromatic hydrocarbons in dry tea. Journal of Environmental Science and Health - Part B Pesticides, Food Contaminants, and Agricultural Wastes, 50, 552–559.
- Adonis, M., & Gil, L. (2001). Indoor air pollution in a zone of extreme poverty of Metropolitan Santiago, Chile. Indoor and Built Environment, 10, 138–146.
- Aemig, Q., Chéron, C., Delgenès, N., Jimenez, J., Houot, S., Steyer, J.-P., & Patureau, D. (2016). Distribution of Polycyclic Aromatic Hydrocarbons (PAHs) in sludge organic matter pools as a driving force of their fate during anaerobic digestion. Waste Management, 48, 389–396.
- Afolabi, O. A., Adesulu, E. A., & Oke, O. L. (1983). Polynuclear aromatic hydrocarbons in some Nigerian preserved freshwater fish species. Journal of Agricultural and Food Chemistry, 31, 1083–1090.
- Afreen, S., Muthoosamy, K., Manickam, S., & Hashim, U. (2015). Functionalized fullerene (C60) as a potential nanomediator in the fabrication of highly sensitive biosensors. Biosensors and Bioelectronics, 63, 354–364.
- Afshar-Mohajer, N., Wilson, C., Wu, C. Y., & Stormer, J. E. (2016). Source apportionment of atmospheric polycyclic aromatic hydrocarbons (PAHs) in Palm Beach County, Florida. Journal of the Air and Waste Management Association, 66, 377–386.
- Agarwal, A. K., Bothra, P., Gupta, T., & Shukla, P. C. (2014). An evaluation of the emission profile for two-wheelers at a traffic junction. Particuology, 18, 112–119.
- Agarwal, T. (2009). Concentration level, pattern and toxic potential of PAHs in traffic soil of Delhi, India. Journal of Hazardous Materials, 171, 894–900.
- Agarwal, T., Khillare, P. S., Shridhar, V., & Ray, S. (2009). Pattern, sources and toxic potential of PAHs in the agricultural soils of Delhi, India. Journal of Hazardous Materials, 163, 1033–1039.
- Ahad, J. M. E., Jautzy, J. J., Cumming, B. F., Das, B., Laird, K. R., & Sanei, H. (2015). Sources of polycyclic aromatic hydrocarbons (PAHs) to northwestern Saskatchewan lakes east of the Athabasca oil sands. Organic Geochemistry, 80, 35–45.
- Ahmed, T. M., Ahmed, B., Aziz, B. K., Bergvall, C., & Westerholm, R. (2015). Native and oxygenated polycyclic aromatic hydrocarbons in ambient air particulate matter from the city of Sulaimaniyah in Iraq. Atmospheric Environment, 116, 44–50.
- Akdoğan, A., Buttinger, G., & Wenzl, T. (2016). Single-laboratory validation of a saponification method for the determination of four polycyclic aromatic hydrocarbons in edible oils by HPLC-fluorescence detection. Food Additives and Contaminants - Part A Chemistry, Analysis, Control, Exposure and Risk Assessment, 33, 215–224.
- Al-Daghri, N. M., Alokail, M. S., Abd-Alrahman, S. H., & Draz, H. M. (2014). Polycyclic aromatic hydrocarbon distribution in serum of Saudi children using HPLC-FLD: marker elevations in children with asthma. Environmental Science and Pollution Research, 21, 12085–12090.
- Al-Thukair, A. A., & Malik, K. (2016). Pyrene metabolism by the novel bacterial strains Burkholderia fungorum (T3A13001) and Caulobacter sp (T2A12002) isolated from an oil-polluted site in the Arabian Gulf. International Biodeterioration and Biodegradation, 110, 32–37.
- Al-Yakoob, S. N., Saeed, T., & Al-Hashash, H. (1994). Polycyclic aromatic hydrocarbons in fish: Exposure assessment for Kuwaiti consumers after the gulf oil spill of 1991. Environment International, 20, 221–227.
- Al Sayegh Petkovšek, S. A. S., Kopušar, S. N., Tome, D. & Kryštufek, B. (2015). Risk assessment of metals and PAHs for receptor organisms in differently polluted areas in Slovenia. Science of the Total Environment, 532, 404–414.
- Alagić, S. Č., Maluckov, B. S., & Radojičić, V. B. (2015). How can plants manage polycyclic aromatic hydrocarbons? May these effects represent a useful tool for an effective soil remediation? A review. Clean Technologies and Environmental Policy, 17, 597–614.
- Albanese, S., Fontaine, B., Chen, W., Lima, A., Cannatelli, C., Piccolo, A., … De Vivo, B. (2014). Polycyclic aromatic hydrocarbons in the soils of a densely populated region and associated human health risks: the Campania Plain (Southern Italy) case study. Environmental Geochemistry and Health, 37 (1), 1–20.
- Albuquerque, M., Coutinho, M., & Borrego, C. (2016). Long-term monitoring and seasonal analysis of polycyclic aromatic hydrocarbons (PAHs) measured over a decade in the ambient air of Porto, Portugal. Science of The Total Environment, 543 (Part A), 439–448.
- Alghamdi, M. A., Alam, M. S., Stark, C., Mohammed, N., Harrison, R. M., Shamy, M., … Göen, T. (2015). Urinary metabolites of polycyclic aromatic hydrocarbons in Saudi Arabian schoolchildren in relation to sources of exposure. Environmental Research, 140, 495–501.
- Alharbi, S. A., Zayed, M. E., Chinnathambi, A., Alharbi, N. S., & Wainwright, M. (2014). Isolation and characterisation of PAH-biodegrading marine bacteria. Journal of Food, Agriculture and Environment, 12, 793–796.
- Alloy, M. M., Boube, I., Griffitt, R. J., Oris, J. T., & Roberts, A. P. (2015). Photo-induced toxicity of Deepwater Horizon slick oil to blue crab (Callinectes sapidus) larvae. Environmental Toxicology and Chemistry, 34, 2061–2066.
- Alomirah, H., Al-Zenki, S., Al-Hooti, S., Zaghloul, S., Sawaya, W., Ahmed, N., & Kannan, K. (2011). Concentrations and dietary exposure to polycyclic aromatic hydrocarbons (PAHs) from grilled and smoked foods. Food Control, 22, 2028–2035.
- Alonge, D. O. (1988). Carcinogenic polycyclic aromatic hydrocarbons (PAH) determined in Nigerian kundi (smoke-dried meat). Journal of the Science of Food and Agriculture, 43, 167–172.
- Alshaarawy, O., Elbaz, H. A., & Andrew, M. E. (2016). The association of urinary polycyclic aromatic hydrocarbon biomarkers and cardiovascular disease in the US population. Environment International, 89–90, 174–178.
- Aly Salem, D. M. S., Morsy, F. A. E. M., El Nemr, A., El-Sikaily, A., & Khaled, A. (2014). The monitoring and risk assessment of aliphatic and aromatic hydrocarbons in sediments of the Red Sea, Egypt. Egyptian Journal of Aquatic Research, 40, 333–348.
- Amezcua-Allieri, M. A., Ávila-Chávez, M. A., Trejo, A., & Meléndez-Estrada, J. (2012). Removal of polycyclic aromatic hydrocarbons from soil: A comparison between bioremoval and supercritical fluids extraction. Chemosphere, 86, 985–993.
- An, L. H., Zheng, B. H., Wang, L. J., Zhang, Y. Q., Chen, H., Zhao, X. R., … Lei, K. (2012). Biomarker responses and genotoxicity in the mud snail (Bullacta exarata) as indicators of coastal contamination. Marine Pollution Bulletin, 64, 303–309.
- An, Y.-Z., Pei, Y.-Q., Qin, J., Zhao, H., Teng, S.-P., Li, B., & Li, X. (2016). Development of a PAH (polycyclic aromatic hydrocarbon) formation model for gasoline surrogates and its application for GDI (gasoline direct injection) engine CFD (computational fluid dynamics) simulation. Energy, 94, 367–379.
- Ando, M., Katagiri, K., Tamura, K., Yamamoto, S., Matsumoto, M., Li, Y. F., … Liang, C. K. (1996). Indoor and outdoor air pollution in Tokyo and Beijing supercities. Atmospheric Environment, 30, 695–702.
- Andrews, A. W., Thibault, L. H., & Lijinsky, W. (1978). The relationship between carcinogenicity and mutagenicity of some polynuclear hydrocarbons. Mutation Research - Fundamental and Molecular Mechanisms of Mutagenesis, 51, 311–318.
- Angioni, A., Cau, A., Secci, M., & Addis, P. (2014). GC-ITMS analysis of PAH contamination levels in the marine sea urchin Paracentrotus lividus in Sardinia. Marine Pollution Bulletin, 82, 201–207.
- Antizar-Ladislao, B., Beck, A. J., Spanova, K., Lopez-Real, J., & Russell, N. J. (2007). The influence of different temperature programmes on the bioremediation of polycyclic aromatic hydrocarbons (PAHs) in a coal-tar contaminated soil by in-vessel composting. Journal of Hazardous Materials, 144, 340–347.
- Anyakora, C., & Coker, H. (2006). Determination of polynuclear aromatic hydrocarbons (PAHs) in selected water bodies in the Niger Delta. African Journal of Biotechnology, 5, 2024–2031.
- Anyanwu, I. N., & Semple, K. T. (2015). Biodegradation of Phenanthrene-Nitrogen-Containing Analogues in Soil. Water, Air, and Soil Pollution, 226, 252.
- Apostolopoulou, M.-V., Monteyne, E., Krikonis, K., Pavlopoulos, K., Roose, P., & Dehairs, F. (2014). Monitoring polycyclic aromatic hydrocarbons in the Northeast Aegean Sea using Posidonia oceanica seagrass and synthetic passive samplers. Marine Pollution Bulletin, 87, 338–344.
- Appenzeller, B. M. R., Mathon, C., Schummer, C., Alkerwi, A., & Lair, M. L. (2012). Simultaneous determination of nicotine and PAH metabolites in human hair specimen: A potential methodology to assess tobacco smoke contribution in PAH exposure. Toxicology Letters, 210, 211–219.
- Araghi, P. E., Bastami, K. D., & Rahmanpoor, S. (2014). Distribution and sources of polycyclic aromatic hydrocarbons in the surface sediments of Gorgan Bay, Caspian Sea. Marine Pollution Bulletin, 89, 494–498.
- Ares, J., Eckl, P. M., & Raffelsberger, I. (2000). Genotoxicity at low level dose of inspirable urban ambient air particulate in a semiarid regime. Environmental Monitoring and Assessment, 63, 389–408.
- Argyropoulos, C. D., Christolis, M. N., Nivolianitou, Z., & Markatos, N. C. (2012). A hazards assessment methodology for large liquid hydrocarbon fuel tanks. Journal of Loss Prevention in the Process Industries, 25, 329–335.
- Armstrong, B. G., Hutchinson, E., Unwin, J., & Fletcher, T. (2004). Lung cancer risk after exposure to polycyclic aromatic hydrocarbons: a review and meta-analysis. Environ Health Perspect, 112, 970–978.
- Atagana, H. I. (2015). Biological Degradation of Crude Oil Refinery Sludge with Commercial Surfactant and Sewage Sludge by Co-Composting. Soil and Sediment Contamination, 24, 494–513.
- Augusto, S., Máguas, C., & Branquinho, C. (2013). Guidelines for biomonitoring persistent organic pollutants (POPs), usinglichens and aquatic mosses - A review. Environmental Pollution, 180, 330–338.
- Aydin, Y. M., Kara, M., Dumanoglu, Y., Odabasi, M., & Elbir, T. (2014). Source apportionment of polycyclic aromatic hydrocarbons (PAHs) and polychlorinated biphenyls (PCBs) in ambient air of an industrial region in Turkey. Atmospheric Environment, 97, 271–285.
- Azah, E., Kim, H., & Townsend, T. (2015). Source of polycyclic aromatic hydrocarbon in roadway and stormwater system maintenance residues. Environmental Earth Sciences, 74, 3029–3039.
- Azis, M. Y., Yelmiza, L., Asia, A., Piram, B., Bucharil, P., Doumenq, A., & Syakti, D. (2016). Occurrence of aliphatic and polyaromatic hydrocarbons (PAHs) in Mytillus galloprovincialis from the traditional market in Marseille, France, by Gas Chromatography triplequadropole tandem Mass Spectrometry (GC-QQQ/MS). In IOP Conference Series: Materials Science and Engineering.
- Aziz, F., Syed, J. H., Malik, R. N., Katsoyiannis, A., Mahmood, A., Li, J., … Jones, K. C. (2014). Occurrence of polycyclic aromatic hydrocarbons in the Soan River, Pakistan: Insights into distribution, composition, sources and ecological risk assessment. Ecotoxicology and Environmental Safety, 109, 77–84.
- Bach, P. B., Kelley, M. J., Tate, R. C., & McCrory, D. C. (2003). Screening for lung cancer: a review of the current literature. Chest, 123, 72–82.
- Bacosa, H. P., & Inoue, C. (2015). Polycyclic aromatic hydrocarbons (PAHs) biodegradation potential and diversity of microbial consortia enriched from tsunami sediments in Miyagi, Japan. Journal of Hazardous Materials, 283, 689–697.
- Balaji, V., Arulazhagan, P., & Ebenezer, P. (2014). Enzymatic bioremediation of polyaromatic hydrocarbons by fungal consortia enriched from petroleum contaminated soil and oil seeds. Journal of Environmental Biology, 35, 521–529.
- Balcioğlu, E. B., Aksu, A., Balkis, N., & Öztürk, B. (2014). T-PAH contamination in Mediterranean mussels (Mytilus galloprovincialis, Lamarck, 1819) at various stations of the Turkish Straits System. Marine Pollution Bulletin, 88, 344–346.
- Baldantoni, D., De Nicola, F., & Alfani, A. (2014). Air biomonitoring of heavy metals and polycyclic aromatic hydrocarbons near a cement plant. Atmospheric Pollution Research, 5, 262–269.
- Baltrons, O., López-Mesas, M., Palet, C., Le Derf, F., & Portet-Koltalo, F. (2013). Molecularly imprinted polymer-liquid chromatography/fluorescence for the selective clean-up of hydroxylated polycyclic aromatic hydrocarbons in soils. Analytical Methods, 5, 6297–6305.
- Bandowe, B. A. M., Bigalke, M., Boamah, L., Nyarko, E., Saalia, F. K., & Wilcke, W. (2014). Polycyclic aromatic compounds (PAHs and oxygenated PAHs) and trace metals in fish species from Ghana (West Africa): Bioaccumulation and health risk assessment. Environment International, 65, 135–146.
- Bandowe, B. A. M., & Nkansah, M. A. (2016). Occurrence, distribution and health risk from polycyclic aromatic compounds (PAHs, oxygenated-PAHs and azaarenes) in street dust from a major West African Metropolis. Science of the Total Environment, 553, 439–449.
- Banerjee, K., Utture, S., Dasgupta, S., Kandaswamy, C., Pradhan, S., Kulkarni, S., & Adsule, P. (2012). Multiresidue determination of 375 organic contaminants including pesticides, polychlorinated biphenyls and polyaromatic hydrocarbons in fruits and vegetables by gas chromatography–triple quadrupole mass spectrometry with introduction of semi-quantification approach. Journal of Chromatography A, 1270, 283–295.
- Bangia, K. S., Symanski, E., Strom, S. S., & Bondy, M. (2015). A cross-sectional analysis of polycyclic aromatic hydrocarbons and diesel particulate matter exposures and hypertension among individuals of Mexican origin. Environmental Health: A Global Access Science Source, 14, 51.
- Bansal, V., & Kim, K. H. (2015). Review of PAH contamination in food products and their health hazards. Environment International, 84, 26–38.
- Barakat, A. O., Mostafa, A., Wade, T. L., Sweet, S. T., & El Sayed, N. B. (2011). Distribution and characteristics of PAHs in sediments from the Mediterranean coastal environment of Egypt. Marine Pollution Bulletin, 62, 1969–1978.
- Barhoumi, B., Clérandeau, C., Gourves, P. Y., Le Menach, K., Megdiche, Y. E., Peluhet, L., … Cachot, J. (2014). Pollution biomonitoring in the Bizerte lagoon (Tunisia), using combined chemical and biomarker analyses in grass goby, Zosterisessor ophiocephalus (Teleostei, Gobiidae). Marine Environmental Research, 101, 184–195.
- Barnsley, E. A. (1974). The bacterial degradation of fluoranthene and benzo[a]pyrene. Can. J. Microbiol, 21, 1004–1008.
- Barreca, S., Bastone, S., Caponetti, E., Martino, D. F. C., & Orecchio, S. (2014). Determination of selected polyaromatic hydrocarbons by gas chromatography-mass spectrometry for the analysis of wood to establish the cause of sinking of an old vessel (Scauri wreck) by fire. Microchemical Journal, 117, 116–121.
- Bartkow, M. E., Huckins, J. N., & Müller, J. F. (2004). Field-based evaluation of semipermeable membrane devices (SPMDs) as passive air samplers of polyaromatic hydrocarbons (PAHs). Atmospheric Environment, 38, 5983–5990.
- Bartkow, M. E., Kennedy, K. E., Huckins, J. N., Holling, N., Komarova, T., & Müller, J. F. (2006). Photodegradation of polyaromatic hydrocarbons in passive air samplers: Field testing different deployment chambers. Environmental Pollution, 144, 371–376.
- Bartolomé, L., Bustamante, M., Navarro, P., Tajadura, J., Gorostiaga, J. M., Díez, I., … Etxebarria, N. (2011). The use of limpets as monitor of PAHs pollution in the Cantabrian coast. Continental Shelf Research, 31, 1818–1826.
- Bartolomé, M., Ramos, J. J., Cutanda, F., Huetos, O., Esteban, M., Ruiz-Moraga, M., … Román, J. (2015). Urinary polycyclic aromatic hydrocarbon metabolites levels in a representative sample of the Spanish adult population: The BIOAMBIENT.ES project. Chemosphere, 135, 436–446.
- Baumard, P., Budzinski, H., Michon, Q., Garrigues, P., Burgeot, T., & Bellocq, J. (1998). Origin and Bioavailability of PAHs in the Mediterranean Sea from Mussel and Sediment Records. Estuarine, Coastal and Shelf Science, 47, 77–90.
- Baxter, C. S., Hoffman, J. D., Knipp, M. J., Reponen, T., & Haynes, E. N. (2014). Exposure of firefighters to particulates and polycyclic aromatic hydrocarbons. Journal of Occupational and Environmental Hygiene, 11, D85–D91.
- Bayat, J., Hashemi, S. H., Khoshbakht, K., Deihimfard, R., Shahbazi, A., & Momeni-Vesalian, R. (2015). Monitoring of polycyclic aromatic hydrocarbons on agricultural lands surrounding Tehran oil refinery. Environmental Monitoring and Assessment, 187, 451.
- Bayraktar, H., Paloluoğlu, C., Turalioğlu, F. S., & Gaga, E. O. (2016). A multipoint (49 points) study of dry deposition of polycyclic aromatic hydrocarbons (PAHs) in Erzurum, Turkey by using surrogated snow surface samplers. Environmental Science and Pollution Research, 23, 12400–12413.
- Benson, N. U., Essien, J. P., Asuquo, F. E., & Eritobor, A. L. (2014). Occurrence and distribution of polycyclic aromatic hydrocarbons in surface microlayer and subsurface seawater of Lagos Lagoon, Nigeria. Environmental Monitoring and Assessment, 186, 5519–5529.
- Bentzen, T. R., & Larsen, T. (2009). Heavy metal and PAH concentrations in highway runoff deposits fractionated on settling velocities. Journal of Environmental Engineering, 135, 1244–1247.
- Beriro, D. J., Cave, M. R., Wragg, J., Thomas, R., Wills, G., & Evans, F. (2016). A review of the current state of the art of physiologically-based tests for measuring human dermal in vitro bioavailability of polycyclic aromatic hydrocarbons (PAH) in soil. Journal of Hazardous Materials, 305, 240–259.
- Berman, T., Goldsmith, R., Göen, T., Spungen, J., Novack, L., Levine, H., … Grotto, I. (2013). Urinary concentrations of environmental contaminants and phytoestrogens in adults in Israel. Environment International, 59, 478–484.
- Bertrand, O., Mondamert, L., Grosbois, C., Dhivert, E., Bourrain, X., Labanowski, J., & Desmet, M. (2015). Storage and source of polycyclic aromatic hydrocarbons in sediments downstream of a major coal district in France. Environmental Pollution, 207, 329–340.
- Bezza, F. A., Beukes, M., & Chirwa, E. M. N. (2015). Application of biosurfactant produced by Ochrobactrum intermedium CN3 for enhancing petroleum sludge bioremediation. Process Biochemistry, 50, 1911–1922.
- Bezza, F. A., & Chirwa, E. M. N. (2014). Optimization strategy of polycyclic aromatic hydrocarbon contaminated media bioremediation through biosurfactant addition. Chemical Engineering Transactions., 39, 1597–1602.
- Bezza, F. A., & Nkhalambayausi Chirwa, E. M. (2015). Biosurfactant from Paenibacillus dendritiformis and its application in assisting polycyclic aromatic hydrocarbon (PAH) and motor oil sludge removal from contaminated soil and sand media. Process Safety and Environmental Protection, 98, 354–364.
- Bezza, F. A., & Nkhalambayausi Chirwa, E. M. (2016). Biosurfactant-enhanced bioremediation of aged polycyclic aromatic hydrocarbons (PAHs) in creosote contaminated soil. Chemosphere, 144, 635–644.
- Bhargava, A., Dlugogorski, B. Z., & Kennedy, E. M. (2002). Emission of polyaromatic hydrocarbons, polychlorinated biphenyls and polychlorinated dibenzo-p-dioxins and furans from fires of wood chips. Fire Safety Journal, 37, 659–672.
- Bi, X., Luo, W., Gao, J., Xu, L., Guo, J., Zhang, Q., … de Boer, J. (2016). Polycyclic aromatic hydrocarbons in soils from the Central-Himalaya region: Distribution, sources, and risks to humans and wildlife. Science of the Total Environment, 556, 12–22.
- Bibi, S., Yasin, T., Hassan, S., Riaz, M., & Nawaz, M. (2015). Chitosan/CNTs green nanocomposite membrane: Synthesis, swelling and polyaromatic hydrocarbons removal. Materials Science and Engineering C, 46, 359–365.
- Binnemann, P. H. (1979). Benzo(a)pyrene contents of meat products. Zeitschrift für Lebensmittel-Untersuchung und -Forschung, 169, 447–452.
- Bisht, S., Pandey, P., Bhargava, B., Sharma, S., Kumar, V., & Krishan, D. (2015). Bioremediation of polyaromatic hydrocarbons (PAHs) using rhizosphere technology. Brazilian Journal of Microbiology, 46, 7–21.
- Bisht, S., Pandey, P., Kaur, G., Aggarwal, H., Sood, A., Sharma, S., … Bisht, N. S. (2014). Utilization of endophytic strain Bacillus sp. SBER3 for biodegradation of polyaromatic hydrocarbons (PAH) in soil model system. European Journal of Soil Biology, 60, 67–76.
- Biswas, B., Sarkar, B., Rusmin, R., & Naidu, R. (2015). Bioremediation of PAHs and VOCs: Advances in clay mineral-microbial interaction. Environment International, 85, 168–181.
- Blahova, J., Leontovycova, D., Kodes, V., & Svobodova, Z. (2013). Study of polycyclic aromatic hydrocarbon contamination of major rivers in the Czech Republic using biliary metabolite in chub, Leuciscus cephalus L. Bulletin of Environmental Contamination and Toxicology, 90, 521–524.
- Blahová, J., Schandlová, L., Grabic, R., Fedorová, G., Randák, T., & Svobodová, Z. (2011). Assessment of polycyclic aromatic hydrocarbon contamination of the bílina river (Czech Republic) using passive water samplers and fish biliary metabolites. Acta Veterinaria Brno, 80, 353–357.
- Blanc, A. M., Holland, L. G., Rice, S. D., & Kennedy, C. J. (2010). Anthropogenically sourced low concentration PAHS: In situ bioavailability to juvenile Pacific salmon. Ecotoxicology and Environmental Safety, 73, 849–857.
- Blumer, M., Blumer, W., & Reich, T. (1977). Polycyclic aromatic hydrocarbons in soils of a mountain valley: Correlation with highway traffic and cancer incidence. Environmental Science and Technology, 11, 1082–1084.
- Blyth, W., Shahsavari, E., Morrison, P. D., & Ball, A. S. (2015). Biosurfactant from red ash trees enhances the bioremediation of PAH contaminated soil at a former gasworks site. Journal of Environmental Management, 162, 30–36.
- Boonyatumanond, R., Wattayakorn, G., Togo, A., & Takada, H. (2006). Distribution and origins of polycyclic aromatic hydrocarbons (PAHs) in riverine, estuarine, and marine sediments in Thailand. Marine Pollution Bulletin, 52, 942–956.
- Bortey-Sam, N., Ikenaka, Y., Nakayama, S. M. M., Akoto, O., Yohannes, Y. B., Baidoo, E., … Ishizuka, M. (2014). Occurrence, distribution, sources and toxic potential of polycyclic aromatic hydrocarbons (PAHs) in surface soils from the Kumasi Metropolis, Ghana. Science of The Total Environment, 496, 471–478.
- Botello, A. V., Soto, L. A., Ponce-Vélez, G., & Susana Villanueva, F. (2015). Baseline for PAHs and metals in NW Gulf of Mexico related to the Deepwater Horizon oil spill. Estuarine, Coastal and Shelf Science, 156, 124–133.
- Bourgeault, A., & Gourlay-Francé, C. (2013). Monitoring PAH contamination in water: Comparison of biological and physico-chemical tools. Science of the Total Environment, 454–455, 328–336.
- Bozcaarmutlu, A., Sapmaz, C., Kaleli, G., Turna, S., & Yenisoy-Karakaş, S. (2015). Combined use of PAH levels and EROD activities in the determination of PAH pollution in flathead mullet (Mugil cephalus) caught from the West Black Sea coast of Turkey. Environmental Science and Pollution Research, 22, 2515–2525.
- Bratberg, M., Olsvik, P. A., Edvardsen, R. B., Brekken, H. K., Vadla, R., & Meier, S. (2013). Effects of oil pollution and persistent organic pollutants (POPs) on glycerophospholipids in liver and brain of male Atlantic cod (Gadus morhua). Chemosphere, 90, 2157–2171.
- Broyde, S., Wang, L., Cai, Y., Jia, L., Shapiro, R., Patel, D. J., & Geacintov, N. E. (2011). Covalent polycyclic aromatic hydrocarbon-DNA adducts: Carcinogenicity, structure, and function. Current Cancer Research., 6, 181–207.
- Bruns, E. A., Krapf, M., Orasche, J., Huang, Y., Zimmermann, R., Drinovec, L., … Prévôt, A. S. H. (2015). Characterization of primary and secondary wood combustion products generated under different burner loads. Atmospheric Chemistry and Physics, 15, 2825–2841.
- Çabuk, H., Kiliç, M. S., & Ören, M. (2014). Biomonitoring of polycyclic aromatic hydrocarbons in urban and industrial environments of the Western Black Sea Region, Turkey. Environmental Monitoring and Assessment, 186, 1515–1524.
- Cacho, J. I., Campillo, N., Viñas, P., & Hernández-Córdoba, M. (2015). Evaluation of the contamination of spirits by polycyclic aromatic hydrocarbons using ultrasound-assisted emulsification microextraction coupled to gas chromatography-mass spectrometry. Food Chemistry, 190, 324–330.
- Cai, Q.-Y., Mo, C.-H., Wu, Q.-T., Zeng, Q.-Y., Katsoyiannis, A., & Férard, J.-F. (2007). Bioremediation of polycyclic aromatic hydrocarbons (PAHs)-contaminated sewage sludge by different composting processes. Journal of Hazardous Materials, 142, 535–542.
- Cai, X. D., Wang, D. Y., Li, H., Li, S. Y., & Chen, L. G. (2014). Ex situ bioremediation of polycyclic aromatic hydrocarbon contaminated soil using a static aeration biopile process. Applied Mechanics and Materials., 448–453, 498–504.
- Cajthaml, T., Mode, M., Kacer, P., Sasek, V., & Popp, P. (2002). Study of fungal degradation productsofpolycyclicaromatichydrocarbonsusinggaschromatographywith ion trap mass spectrometry detection. J. Chromatogr. A, 974, 213–222.
- Cajthaml, T., Pacakova, V., & Sasek, V. (2001). Microbialdegradationofpolycyclicaromatic hydrocarbons” Chem. Listy., 95, 404.
- Camacho, M., Boada, L. D., Orós, J., Calabuig, P., Zumbado, M., & Luzardo, O. P. (2012). Comparative study of polycyclic aromatic hydrocarbons (PAHs) in plasma of Eastern Atlantic juvenile and adult nesting loggerhead sea turtles (Caretta caretta). Marine Pollution Bulletin, 64, 1974–1980.
- Cameotra, S. S., & Bollag, J.-M. (2003). Biosurfactant-Enhanced Bioremediation of Polycyclic Aromatic Hydrocarbons. Critical Reviews in Environmental Science and Technology, 33, 111–126.
- Capozzi, F., Giordano, S., Di Palma, A., Spagnuolo, V., De Nicola, F., & Adamo, P. (2016). Biomonitoring of atmospheric pollution by moss bags: Discriminating urban-rural structure in a fragmented landscape. Chemosphere, 149, 211–218.
- Cardellicchio, N., Buccolieri, A., Giandomenico, S., Lopez, L., Pizzulli, F., & Spada, L. (2007). Organic pollutants (PAHs, PCBs) in sediments from the Mar Piccolo in Taranto (Ionian Sea, Southern Italy). Marine Pollution Bulletin, 55, 451–458.
- Caruso, J. A., Zhang, K., Schroeck, N. J., McCoy, B., & McElmurry, S. P. (2015). Petroleum coke in the urban environment: A review of potential health effects. International Journal of Environmental Research and Public Health, 12, 6218–6231.
- Castro-Jiménez, J., Berrojalbiz, N., Wollgast, J., & Dachs, J. (2012). Polycyclic aromatic hydrocarbons (PAHs) in the Mediterranean Sea: Atmospheric occurrence, deposition and decoupling with settling fluxes in the water column. Environmental Pollution, 166, 40–47.
- Castro, D., Slezakova, K., Delerue-Matos, C., Alvim-Ferraz, M. D. C., Morais, S., & Pereira, M. D. C. (2011). Polycyclic aromatic hydrocarbons in gas and particulate phases of indoor environments influenced by tobacco smoke: Levels, phase distributions, and health risks. Atmospheric Environment, 45, 1799–1808.
- Celeiro, M., Lamas, J. P., Garcia-Jares, C., Dagnac, T., Ramos, L., & Llompart, M. (2014). Investigation of PAH and other hazardous contaminant occurrence in recycled tyre rubber surfaces. Case-study: restaurant playground in an indoor shopping centre. International Journal of Environmental Analytical Chemistry, 94, 1264–1271.
- Cerniglia, C. E. (1984). Microbial metabolism of polycyclic aromatic hydrocarbons. Adv. Appl Microbiol, 30, 31–71.
- Cerniglia, C. E. (1992). Biodegradation of polycyclic aromatic hydrocarbons. Bioderedation, 3, 351–368.
- Cerniglia, C. E., & Heitkamp, M. A. (1989). Microbial degradation of polycyclic aromatic hydrocarbons (PAH) in the aquatic environment. Boca Raton: Fla, CRC Press Inc.
- Chaemsai, S., Kunanopparat, T., Srichumpuang, J., Nopharatana, M., Tangduangdee, C., & Siriwattanayotin, S. (2016). Reduction of the polycyclic aromatic hydrocarbon (PAH) content of charcoal smoke during grilling by charcoal preparation using high carbonisation and a preheating step. Food Additives and Contaminants - Part A Chemistry, Analysis, Control, Exposure and Risk Assessment, 33, 385–390.
- Chakraborty, P., Sakthivel, S., Kumar, B., Kumar, S., Mishra, M., Verma, V. K., & Gaur, R. (2014). Spatial distribution of persistent organic pollutants in the surface water of River Brahmaputra and River Ganga in India. Reviews on Environmental Health, 29, 45–48.
- Chang, W.-T., Fang, M.-D., Lee, C.-L., & Brimblecombe, P. (2014). Measuring bioavailable PAHs in estuarine water using semipermeable membrane devices with performance reference compounds. Marine Pollution Bulletin, 89, 376–383.
- Chang, Y. T., Lee, J. F., Liu, K. H., Liao, Y. F., & Yang, V. (2016). Immobilization of fungal laccase onto a nonionic surfactant-modified clay material: application to PAH degradation. Environmental Science and Pollution Research, 23, 4024–4035.
- Chen, B. H., & Lin, Y. S. (1997). Formation of Polycyclic Aromatic Hydrocarbons during Processing of Duck Meat. Journal of Agricultural and Food Chemistry, 45, 1394–1403.
- Chen, C. F., Chen, C. W., Ju, Y. R., & Dong, C. D. (2016). Vertical profile, source apportionment, and toxicity of PAHs in sediment cores of a wharf near the coal-based steel refining industrial zone in Kaohsiung, Taiwan. Environmental Science and Pollution Research, 23, 4786–4796.
- Chen, F., Tan, M., Ma, J., Zhang, S., Li, G., & Qu, J. (2016). Efficient remediation of PAH-metal co-contaminated soil using microbial-plant combination: A greenhouse study. Journal of Hazardous Materials, 302, 250–261.
- Chen, H.-Y., Teng, Y.-G., & Wang, J.-S. (2012). Source apportionment of polycyclic aromatic hydrocarbons (PAHs) in surface sediments of the Rizhao coastal area (China) using diagnostic ratios and factor analysis with nonnegative constraints. Science of The Total Environment, 414, 293–300.
- Chen, H.-Y., Teng, Y.-G., & Wang, J.-S. (2013). Source apportionment for sediment PAHs from the Daliao River (China) using an extended fit measurement mode of chemical mass balance model. Ecotoxicology and Environmental Safety, 88, 148–154.
- Chen, H., Gao, G., Liu, P., Pan, R., Liu, X., & Lu, C. (2016). Determination of 16 Polycyclic Aromatic Hydrocarbons in Tea by Simultaneous Dispersive Solid-Phase Extraction and Liquid-Liquid Extraction Coupled with gas Chromatography-Tandem Mass Spectrometry. Food Analytical Methods, 9, 2374–2384.
- Chen, J., & Chen, S. (2005). Removal of polycyclic aromatic hydrocarbons by low density polyethylene from liquid model and roasted meat. Food Chemistry, 90, 461–469.
- Chen, Y., Shen, G., Su, S., Shen, H., Huang, Y., Li, T., … Tao, S. (2014). Contamination and distribution of parent, nitrated, and oxygenated polycyclic aromatic hydrocarbons in smoked meat. Environmental Science and Pollution Research, 21, 11521–11530.
- Chen, Y., Zhang, J., Ma, Q., Sun, C., Ha, S., & Zhang, F. (2016). Human health risk assessment and source diagnosis of polycyclic aromatic hydrocarbons (PAHs) in the corn and agricultural soils along main roadside in Changchun, China. Human and Ecological Risk Assessment, 22, 706–720.
- Chen, Y., Zhao, R., Xue, J., & Li, J. (2013). Generation and distribution of PAHs in the process of medical waste incineration. Waste Management, 33, 1165–1173.
- Chen, Y., Zhi, G., Feng, Y., Tian, C., Bi, X., Li, J., & Zhang, G. (2015). Increase in polycyclic aromatic hydrocarbon (PAH) emissions due to briquetting: A challenge to the coal briquetting policy. Environmental Pollution, 204, 58–63.
- Cheng, Y. J., Huang, S. H., Chiu, J. Y., Liu, W. L., & Huang, H. Y. (2013). Analyses of polycyclic aromatic hydrocarbons in seafood by capillary electrochromatography-atmospheric pressure chemical ionization/mass spectrometry. Journal of Chromatography A, 1313, 132–138.
- Chiang, H.-L., Lai, Y.-M., & Chang, S.-Y. (2012). Pollutant constituents of exhaust emitted from light-duty diesel vehicles. Atmospheric Environment, 47, 399–406.
- Chiffre, A., Degiorgi, F., Morin-Crini, N., Bolard, A., Chanez, E., & Badot, P. M. (2015). PAH occurrence in chalk river systems from the Jura region (France). Pertinence of suspended particulate matter and sediment as matrices for river quality monitoring. Environmental Science and Pollution Research, 22, 17486–17498.
- Chirakkara, R. A., & Reddy, K. R. (2015). Plant species identification for phytoremediation of mixed contaminated soils. Journal of Hazardous, Toxic, and Radioactive Waste, 19, 04015004.
- Choi, H., Zdeb, M., Perera, F., & Spengler, J. (2015). Estimation of chronic personal exposure to airborne polycyclic aromatic hydrocarbons. Science of the Total Environment, 527–528, 252–261.
- Choi, S.-D. (2014). Time trends in the levels and patterns of polycyclic aromatic hydrocarbons (PAHs) in pine bark, litter, and soil after a forest fire. Science of The Total Environment, 470–471, 1441–1449.
- Choi, S.-D., Baek, S.-Y., & Chang, Y.-S. (2007). Influence of a large steel complex on the spatial distribution of volatile polycyclic aromatic hydrocarbons (PAHs) determined by passive air sampling using membrane-enclosed copolymer (MECOP). Atmospheric Environment, 41, 6255–6264.
- Chou, P. H., Liu, T. C., Ko, F. C., Liao, M. W., Yeh, H. M., Yang, T. H., … Tsai, T. Y. (2014). Occurrence of aryl hydrocarbon receptor agonists and genotoxic compounds in the river systems in Southern Taiwan. Chemosphere, 107, 257–264.
- Christensen, E. R., & Bzdusek, P. A. (2005). PAHs in sediments of the Black River and the Ashtabula River, Ohio: source apportionment by factor analysis. Water Research, 39(4), 511–524.
- Chropeňová, M., Gregušková, E. K., Karásková, P., Přibylová, P., Kukučka, P., Baráková, D., & Čupr, P. (2016). Pine needles and pollen grains of Pinus mugo Turra - A biomonitoring tool in high mountain habitats identifying environmental contamination. Ecological Indicators, 66, 132–142.
- Chrysikou, L., Gemenetzis, P., Kouras, A., Manoli, E., Terzi, E., & Samara, C. (2008). Distribution of persistent organic pollutants, polycyclic aromatic hydrocarbons and trace elements in soil and vegetation following a large scale landfill fire in northern Greece. Environment International, 34, 210–225.
- Chu, I., Villeneuve, D. C., & Rousseaux, C. G. (1994). Toxicology of coal liquefaction products: An overview. Journal of Applied Toxicology, 14, 241–256.
- Ciemniak, A., Witczak, A., & Mocek, K. (2013). Assessment of honey contamination with polycyclic aromatic hydrocarbons. Journal of Environmental Science and Health - Part B Pesticides, Food Contaminants, and Agricultural Wastes, 48, 993–998.
- Ciesielczuk, T., Olszowski, T., Prokop, M., & Kłos, A. (2012). Application of mosses to identification of emission sources of polycyclic aromatic hydrocarbons. Ecological Chemistry and Engineering S, 19, 585–595.
- Clément, N., Muresan, B., Hedde, M., & François, D. (2015). PAH dynamics in roadside environments: Influence on the consistency of diagnostic ratio values and ecosystem contamination assessments. Science of the Total Environment, 538, 997–1009.
- Cobas, M., Ferreira, L., Tavares, T., Sanromán, M. A., & Pazos, M. (2013). Development of permeable reactive biobarrier for the removal of PAHs by Trichoderma longibrachiatum. Chemosphere, 91, 711–716.
- Cofield, N., Schwab, A. P., & Banks, M. K. (2007). Phytoremediation of polycyclic aromatic hydrocarbons in soil: Part I. Dissipation of target contaminants. International Journal of Phytoremediation, 9, 355–370.
- Çolak, H., Hampikyan, H., Bingöl, E. B., Çetin, Ö., & Akhan, M. (2013). Presence of benzo(a)pyrene in baby foods sold in retail. Istanbul Universitesi Veteriner Fakultesi Dergisi, 39, 218–224.
- Commendatore, M. G., Nievas, M. L., Amin, O., & Esteves, J. L. (2012). Sources and distribution of aliphatic and polyaromatic hydrocarbons in coastal sediments from the Ushuaia Bay (Tierra del Fuego, Patagonia, Argentina). Marine Environmental Research, 74, 20–31.
- Conaway, C. H., Draut, A. E., Echols, K. R., Storlazzi, C. D., & Ritchie, A. (2013). Episodic suspended sediment transport and elevated polycyclic aromatic hydrocarbon concentrations in a small, mountainous river in coastal california. River Research and Applications, 29, 919–932.
- Coronas, M. V., Vaz Rocha, J. A., Favero Salvadori, D. M., & Ferrão Vargas, V. M. (2016). Evaluation of area contaminated by wood treatment activities: Genetic markers in the environment and in the child population. Chemosphere, 144, 1207–1215.
- Corrotea, Y., Sánchez, K., Rubio, M. A., & Richter, P. (2014). Extraction of polycyclic aromatic hydrocarbons from water samples into a rotating-disk microextractor and the subsequent determination by gas chromatography-mass spectrometry. Journal of the Chilean Chemical Society, 59, 2477–2480.
- Counihan, T. D., Waite, I. R., Nilsen, E. B., Hardiman, J. M., Elias, E., Gelfenbaum, G., & Zaugg, S. D. (2014). A survey of benthic sediment contaminants in reaches of the Columbia River Estuary based on channel sedimentation characteristics. Science of the Total Environment, 484, 331–343.
- Crampon, M., Bureau, F., Akpa-Vinceslas, M., Bodilis, J., Machour, N., Le Derf, F., & Portet-Koltalo, F. (2014). Correlations between PAH bioavailability, degrading bacteria, and soil characteristics during PAH biodegradation in five diffusely contaminated dissimilar soils. Environmental Science and Pollution Research, 21, 8133–8145.
- Cui, C., Ma, L., Shi, J., Lin, K., Luo, Q., & Liu, Y. (2014). Metabolic pathway for degradation of anthracene by halophilic Martelella sp. AD-3. International Biodeterioration and Biodegradation, 89, 67–73.
- Cui, M., Chen, Y., Tian, C., Zhang, F., Yan, C., & Zheng, M. (2016). Chemical composition of PM2.5 from two tunnels with different vehicular fleet characteristics. Science of the Total Environment, 550, 123–132.
- Dadkhah, A. A., & Akgerman, A. (2006). Hot water extraction with in situ wet oxidation: Kinetics of PAHs removal from soil. Journal of Hazardous Materials, 137, 518–526.
- Das, P., Mukherjee, S., & Sen, R. (2008). Improved bioavailability and biodegradation of a model polyaromatic hydrocarbon by a biosurfactant producing bacterium of marine origin. Chemosphere, 72, 1229–1234.
- de la Rosa, J. M., Paneque, M., Hilber, I., Blum, F., Knicker, H. E., & Bucheli, T. D. (2015). Assessment of polycyclic aromatic hydrocarbons in biochar and biochar-amended agricultural soil from Southern Spain. Journal of Soils and Sediments, 16, 557–565.
- De la Rosa, J. M., Paneque, M., Hilber, I., Blum, F., Knicker, H. E., & Bucheli, T. D. (2016). Assessment of polycyclic aromatic hydrocarbons in biochar and biochar-amended agricultural soil from Southern Spain. Journal of Soils and Sediments, 16, 557–565.
- De Nicola, F., Concha Graña, E., Aboal, J. R., Carballeira, A., Fernández, J. Á., López Mahía, P., … Muniategui Lorenzo, S. (2016). PAH detection in Quercus robur leaves and Pinus pinaster needles: A fast method for biomonitoring purpose. Talanta, 153, 130–137.
- de Oliveira Galvão, M. F., de Melo Cabral, T., de André, P. A., de Fátima Andrade, M., de Miranda, R. M., Saldiva, P. H. N., … De Medeiros, S. R. B. (2014). Cashew nut roasting: Chemical characterization of particulate matter and genotocixity analysis. Environmental Research, 131, 145–152.
- Deziel, E., Paquette, G., Villemur, R., Lepine, F., & Bisaillon, J. (1996). Biosurfactant production by a soil Pseudomonas strain growing on polycyclic aromatic hydrocarbons. Appl. Environ. Microbiol., 62, 1908–1912.
- DellaValle, C. T., Deziel, N. C., Jones, R. R., Colt, J. S., De Roos, A. J., Cerhan, J. R., … Ward, M. H. (2016). Polycyclic aromatic hydrocarbons: determinants of residential carpet dust levels and risk of non-Hodgkin lymphoma. Cancer Causes and Control, 27(1), 1–13.
- Deng, G., Yang, W., Zhou, G., Li, Y., & Liu, S. (2014). Heavy metals and polycyclic aromatic hydrocarbons in sediments from the Shenzhen River, South China. Environmental Science and Pollution Research, 21, 10600–13.
- Deng, Q., Dai, X., Guo, H., Huang, S., Kuang, D., Feng, J., … Wu, T. (2014). Polycyclic aromatic hydrocarbons-associated micrornas and their interactions with the environment: Influences on oxidative dna damage and lipid peroxidation in coke oven workers. Environmental Science and Technology, 48, 4120–4128.
- Deora, A., Giri, R., Suneja, S., Kukreja, K., & Kumar, P. (2013). Evaluation of paenibacillus strains for the degradation of pyrene-A poly cyclic aromatic hydrocarbon. Annals of Biology, 29, 7–14.
- Deschˆenes, L., Lafrance, P., Villeneuve, J. P., & Samon, R. (1996). Adding sodium dodecyl sulfate and Pseudomonas aeruginosa UG2 biosurfactants inhibits polycyclic aromatic hydrocarbon biodegradation in a weathered creosote-contaminated soil. Appl. Microbiol. Biotechnol., 46, 638–646.
- Devi, N. L., Yadav, I. C., Shihua, Q., Dan, Y., Zhang, G., & Raha, P. (2016). Environmental carcinogenic polycyclic aromatic hydrocarbons in soil from Himalayas, India: Implications for spatial distribution, sources apportionment and risk assessment. Chemosphere, 144, 493–502.
- Deziel, N. C., Wei, W. Q., Abnet, C. C., Qiao, Y. L., Sunderland, D., Ren, J. S., … Roth, M. J. (2013). A multi-day environmental study of polycyclic aromatic hydrocarbon exposure in a high-risk region for esophageal cancer in China. Journal of Exposure Science and Environmental Epidemiology, 23, 52–59.
- Di Vaio, P., Cocozziello, B., Corvino, A., Fiorino, F., Frecentese, F., Magli, E., … Perissutti, E. (2016). Level, potential sources of polycyclic aromatic hydrocarbons (PAHs) in particulate matter (PM10) in Naples. Atmospheric Environment, 129, 186–196.
- Diggs, D. L., Huderson, A. C., Harris, K. L., Myers, J. N., Banks, L. D., & Rekhadevi, P. V. (2011). Polycyclic aromatic hydrocarbons and digestive tract cancers: a perspective. J Environ Sci Health C Environ Carcinog Ecotoxicol Rev, 29, 324–357.
- Djinovic, J., Popovic, A., & Jira, W. (2008). Polycyclic aromatic hydrocarbons (PAHs) in different types of smoked meat products from Serbia. Meat Science, 80, 449–456.
- Domingos, M., Bulbovas, P., Camargo, C. Z. S., Aguiar-Silva, C., Brandão, S. E., Dafré-Martinelli, M., … Figueiredo, A. M. G. (2015). Searching for native tree species and respective potential biomarkers for future assessment of pollution effects on the highly diverse Atlantic Forest in SE-Brazil. Environmental Pollution, 202, 85–95.
- Domínguez-Morueco, N., Schuhmacher, M., Sierra, J., Nadal, M., & Domingo, J. L. (2016). Assessment of PAH loss in passive air samplers by the effect of temperature. Atmospheric Pollution Research, 7, 142–146.
- Dong, C., Bai, X., Sheng, H., Jiao, L., Zhou, H., & Shao, Z. (2015). Distribution of PAHs and the PAH-degrading bacteria in the deep-sea sediments of the high-latitude Arctic Ocean. Biogeosciences, 12, 2163–2177.
- Drabova, L., Pulkrabova, J., Kalachova, K., Tomaniova, M., Kocourek, V., & Hajslova, J. (2012). Rapid determination of polycyclic aromatic hydrocarbons (PAHs) in tea using two-dimensional gas chromatography coupled with time of flight mass spectrometry. Talanta, 100, 207–216.
- Duan, X., Shen, G., Yang, H., Tian, J., Wei, F., Gong, J., & Zhang, J. (2016). Dietary intake polycyclic aromatic hydrocarbons (PAHs) and associated cancer risk in a cohort of Chinese urban adults: Inter- and intra-individual variability. Chemosphere, 144, 2469–2475.
- Duan, Y., Shen, G., Tao, S., Hong, J., Chen, Y., Xue, M., … Song, K. (2015). Characteristics of polycyclic aromatic hydrocarbons in agricultural soils at a typical coke production base in Shanxi, China. Chemosphere, 127, 64–69.
- Dubowsky, S. D., Wallace, L. A., & Buckley, T. J. (1999). The contribution of traffic to indoor concentrations of polycyclic aromatic hydrocarbons. Journal of Exposure Analysis and Environmental Epidemiology, 9, 312–321.
- Dudhagara, D. R., Rajpara, R. K., Bhatt, J. K., Gosai, H. B., Sachaniya, B. K., & Dave, B. P. (2016). Distribution, sources and ecological risk assessment of PAHs in historically contaminated surface sediments at Bhavnagar coast, Gujarat, India. Environmental Pollution, 213, 338–346.
- Duedahl-Olesen, L. (2013). Polycyclic aromatic hydrocarbons (PAHs) in foods. In M. Rose & A. Fernandes (Eds.), Persistent Organic Pollutants and Toxic Metals in Foods (pp. 308–333). Woodhead Publishing.
- Duedahl-Olesen, L., Navaratnam, M. A., Jewula, J., & Jensen, A. H. (2015). PAH in Some Brands of Tea and Coffee. Polycyclic Aromatic Compounds, 35, 74–90.
- Duke, O. (2008). Source determination of polynuclear aromatic hydrocarbons in water and sediment of a creek in the Niger Delta region. African Journal of Biotechnology, 7, 282–285.
- Edge, K. J., Johnston, E. L., Roach, A. C., & Ringwood, A. H. (2012). Indicators of environmental stress: Cellular biomarkers and reproductive responses in the Sydney rock oyster (Saccostrea glomerata). Ecotoxicology, 21, 1415–1425.
- El-Saeid, M. H., Al-Turki, A. M., Nadeem, M. E. A., Hassanin, A. S., & Al-Wabel, M. I. (2015). Photolysis degradation of polyaromatic hydrocarbons (PAHs) on surface sandy soil. Environmental Science and Pollution Research, 22, 9603–9616.
- Enault, J., Robert, S., Schlosser, O., de Thé, C., & Loret, J. F. (2015). Drinking water, diet, indoor air: Comparison of the contribution to environmental micropollutants exposure. International Journal of Hygiene and Environmental Health, 218, 723–730.
- Eriksson, A. C., Nordin, E. Z., Nyström, R., Pettersson, E., Swietlicki, E., Bergvall, C., … Pagels, J. H. (2014). Particulate PAH emissions from residential biomass combustion: Time-resolved analysis with aerosol mass spectrometry. Environmental Science and Technology, 48, 7143–7150.
- Eskandary, S., Hoodaji, M., Tahmourespour, A., & Abdollahi, A. (2014). Bioremediation of PAHs from contaminated soils by Festuca aroundiacea in the presence of Bacillus licheniformis and Bacillus mojavensis. Journal of Residuals Science and Technology, 11, 99–105.
- Fadiel, A., Epperson, B., Shaw, M. I., Hamza, A., Petito, J., & Naftolin, F. (2013). Bioinformatic analysis of benzo-α-pyrene-induced damage to the human placental insulin-like growth factor-1 gene. Reproductive Sciences, 20, 917–928.
- Fan, C.-W., Yang, T.-N., & Kao, S.-J. (2010). Characteristics of sedimentary polycyclic aromatic hydrocarbons (PAHs) in the subtropical Feitsui Reservoir, Taiwan. Journal of Hydrology, 391, 217–222.
- Fan, R., Li, J., Chen, L., Xu, Z., He, D., Zhou, Y., … Li, J. (2014). Biomass fuels and coke plants are important sources of human exposure to polycyclic aromatic hydrocarbons, benzene and toluene. Environmental Research, 135, 1–8.
- Fasano, E., Esposito, F., Scognamiglio, G., Cocchieri Amodio, R., & Cirillo, T. (2015). Detection of polycyclic aromatic hydrocarbons in smoked buffalo mozzarella cheese produced in Campania Region, Italy. Journal of the Science of Food and Agriculture.
- Fasano, E., Esposito, F., Scognamiglio, G., Cocchieri Amodio, R., & Cirillo, T. (2016). Detection of polycyclic aromatic hydrocarbons in smoked buffalo mozzarella cheese produced in Campania Region, Italy. Journal of the Science of Food and Agriculture, 96, 1704–1708.
- Feldt, T., Fobil, J. N., Wittsiepe, J., Wilhelm, M., Till, H., Zoufaly, A., … Göen, T. (2014). High levels of PAH-metabolites in urine of e-waste recycling workers from Agbogbloshie, Ghana. Science of the Total Environment, 466–467, 369–376.
- Feng, J., Hu, P., Zhang, F., Wu, Y., Liu, S., & Sun, J. (2016). Ecological risk assessment of polycyclic aromatic hydrocarbons in surface sediments from the middle and lower reaches of the Yellow River, China. Human and Ecological Risk Assessment, 22, 532–542.
- Feng, J., Li, X., Guo, W., Liu, S., Ren, X., & Sun, J. (2014). Potential source apportionment of polycyclic aromatic hydrocarbons in surface sediments from the middle and lower reaches of the Yellow River, China. Environmental Science and Pollution Research, 21, 11447–11456.
- Feng, J., Sun, M., Bu, Y., & Luo, C. (2016). Development of a cheap and accessible carbon fibers-in-poly(ether ether ketone) tube with high stability for online in-tube solid-phase microextraction. Talanta, 148, 313–320.
- Fernández-Luqueño, F., López-Valdez, F., Dendooven, L., Luna-Suárez, S., & Ceballos-Ramírez, J. M. (2016). Why wastewater sludge stimulates and accelerates removal of PAHs in polluted soils? Applied Soil Ecology, 101, 1–4.
- Fernández-Tajes, J., Flórez, F., Pereira, S., Rábade, T., Laffon, B., & Méndez, J. (2011). Use of three bivalve species for biomonitoring a polluted estuarine environment. Environmental Monitoring and Assessment, 177, 289–300.
- Fernández-Varela, R., Ratola, N., Alves, A., & Amigo, J. M. (2015). Relationship between levels of polycyclic aromatic hydrocarbons in pine needles and socio-geographic parameters. Journal of Environmental Management, 156, 52–61.
- Fernando, S., Shaw, L., Shaw, D., Gallea, M., Vandenenden, L., House, R., … McCarry, B. E. (2016). Evaluation of Firefighter Exposure to Wood Smoke during Training Exercises at Burn Houses. Environmental Science and Technology, 50, 1536–1543.
- Fiedler, H., Cheung, C. K., & Wong, M. H. (2002). PCDD/PCDF, chlorinated pesticides and PAH in Chinese teas. Chemosphere, 46, 1429–1433.
- Fisher, T. T., Law, R. J., Rumney, H. S., Kirby, M. F., & Kelly, C. (2011). Towards a scheme of toxic equivalency factors (TEFs) for the acute toxicity of PAHs in sediment. Ecotoxicology and Environmental Safety, 74, 2245–2251.
- Foan, L., Domercq, M., Bermejo, R., Santamaría, J. M., & Simon, V. (2015). Mosses as an integrating tool for monitoring PAH atmospheric deposition: Comparison with total deposition and evaluation of bioconcentration factors. A year-long case-study. Chemosphere, 119, 452–458.
- Fontcuberta, M., Arqués, J. F., Martínez, M., Suárez, A., Villalbí, J. R., Centrich, F., … Casas, C. (2006). Polycyclic aromatic hydrocarbons in food samples collected in Barcelona, Spain. Journal of Food Protection, 69, 2024–2028.
- Frapiccini, E., & Marini, M. (2015). Polycyclic Aromatic Hydrocarbon Degradation and Sorption Parameters in Coastal and Open-Sea Sediment. Water, Air, and Soil Pollution, 226, 246.
- Fretheim, K. (1983). Polycyclic aromatic hydrocarbons in grilled meat products-A review. Food Chemistry, 10, 129–139.
- Fritt-Rasmussen, J., Wegeberg, S., & Gustavson, K. (2015). Review on Burn Residues from in Situ Burning of Oil Spills in Relation to Arctic Waters. Water, Air, and Soil Pollution, 226, 329.
- Froehner, S., MacHado, K. S., Dombroski, L. F., Nunes, A. C., Kishi, R. T., Bleninger, T., & Sanez, J. (2012). Natural biofilms in freshwater ecosystem: Indicators of the presence of polycyclic aromatic hydrocarbons. Water, Air, and Soil Pollution, 223, 3965–3973.
- Galarneau, E., Makar, P. A., Zheng, Q., Narayan, J., Zhang, J., Moran, M. D., … Chlumsky, R. (2014). PAH concentrations simulated with the AURAMS-PAH chemical transport model over Canada and the USA. Atmospheric Chemistry and Physics, 14, 4065–4077.
- Gao, B., Du, X., Wang, X., Tang, J., Ding, X., Zhang, Y., … Zhang, G. (2015). Parent, Alkylated, and Sulfur/Oxygen-Containing Polycyclic Aromatic Hydrocarbons in Mainstream Smoke from 13 Brands of Chinese Cigarettes. Environmental Science and Technology, 49, 9012–9019.
- Gao, B., Wang, X. M., Zhao, X. Y., Ding, X., Fu, X. X., Zhang, Y. L., … Guo, H. (2015). Source apportionment of atmospheric PAHs and their toxicity using PMF: Impact of gas/particle partitioning. Atmospheric Environment, 103, 114–120.
- Gao, C., He, Z., Li, J., Li, X., Bai, Q., Zhang, Z., … Chen, W. (2016). Specific long non-coding RNAs response to occupational PAHs exposure in coke oven workers. Toxicology Reports, 3, 160–166.
- Gao, J., Jian, Y., Cao, C., Chen, L., & Zhang, X. (2015). Indoor emission, dispersion and exposure of total particle-bound polycyclic aromatic hydrocarbons during cooking. Atmospheric Environment, 120, 191–199.
- García-Delgado, C., Alfaro-Barta, I., & Eymar, E. (2015). Combination of biochar amendment and mycoremediation for polycyclic aromatic hydrocarbons immobilization and biodegradation in creosote-contaminated soil. Journal of Hazardous Materials, 285, 259–266.
- García-Delgado, C., Yunta, F., & Eymar, E. (2015). Bioremediation of multi-polluted soil by spent mushroom (Agaricus bisporus) substrate: Polycyclic aromatic hydrocarbons degradation and Pb availability. Journal of Hazardous Materials, 300, 281–288.
- Garcia-Suastegui, W. A., Huerta-Chagoya, A., Carrasco-Colın, K. L., Pratt, M. M., John, K., & Petrosyan, P. (2011). Seasonal variations in the levels of PAH-DNA adducts in young adults living inMexico City. Mutagenesis, 26, 385–391.
- Garcia, L., Tild, V. A., Reynoso, C. M., Garcia, L. & Resnik, S. L. (2015). Polycyclic aromatic hydrocarbons (PAHs) survey on tea (Camellia sinensis) commercialized in Argentina. Food Control, 50, 31–37.
- Garcia London, V. A., Renoso, C. M., & Resnik, S. L. (2015). Polycyclic aromatic hydrocarbons (PAHs) survey on tea (Camellia sinensis) commercialized in Argentina. Food Control, 50, 31–37.
- Gargiulo, V., Apicella, B., Alfè, M., Russo, C., Stanzione, F., Tregrossi, A., … Ciajolo, A. (2015). Structural Characterization of Large Polycyclic Aromatic Hydrocarbons. Part 1: The Case of Coal Tar Pitch and Naphthalene-Derived Pitch. Energy and Fuels, 29, 5714–5722.
- Garrido, A., Jiménez-Guerrero, P., & Ratola, N. (2014). Levels, trends and health concerns of atmospheric PAHs in Europe. Atmospheric Environment, 99, 474–484.
- Gavina, J. M. A., Yao, C., & Feng, Y.-L. (2014). Recent developments in DNA adduct analysis by mass spectrometry: A tool for exposure biomonitoring and identification of hazard for environmental pollutants. Talanta, 130, 475–494.
- Ghosh, I., & Mukherji, S. (2016). Diverse effect of surfactants on pyrene biodegradation by a Pseudomonas strain utilizing pyrene by cell surface hydrophobicity induction. International Biodeterioration and Biodegradation, 108, 67–75.
- Gibson, D. T., & Subramanian, V. (1984). Microbial degradation of aromatic hydrocarbons. New York, NY: Marcel Dekker,.
- Gibson, D. T., Venkatanayarana, M., Jerina, D. M., Yagi, H., & Yeh, H. J. (1975). Oxidationofthecarcinogensbenzo[a]pyreneandbenzo[a]anthracene to dihydrodiols by a bacterium. Science, 189, 295–297.
- Giuliani, S., Piazza, R., El Moumni, B., Polo, F. P., Vecchiato, M., Romano, S., … Bellucci, L. G. (2015). Recognizing different impacts of human and natural sources on the spatial distribution and temporal trends of PAHs and PCBs (including PCB-11) in sediments of the nador lagoon (Morocco). Science of the Total Environment, 526, 346–357.
- Godzik, B., Szarek-Łukaszewska, G., Kapusta, P., & Stȩpień, K. (2014). PAHs concentrations in Poland using moss Pleurozium schreberi as bioindicator. Polish Botanical Journal, 59, 137–144.
- Gomaa, E. A., Gray, J. I., Rabie, S., Lopez-Bote, C., & Booren, A. M. (1993). Polycyclic aromatic hydrocarbons in smoked food products and commercial liquid smoke flavourings. Food Additives and Contaminants, 10, 503–521.
- Gomes, A., Santos, C., Almeida, J., Elias, M., & Roseiro, L. C. (2013). Effect of fat content, casing type and smoking procedures on PAHs contents of Portuguese traditional dry fermented sausages. Food and Chemical Toxicology, 58, 369–374.
- Gong, J., Zhu, T., Kipen, H., Rich, D. Q., Huang, W., Lin, W. T., … Zhang, J. J. (2015). Urinary polycyclic aromatic hydrocarbon metabolites as biomarkers of exposure to traffic-emitted pollutants. Environment International, 85, 104–110.
- Gong, X., Xu, X., Gong, Z., Li, X., Jia, C., Guo, M., & Li, H. (2015). Remediation of PAH-contaminated soil at a gas manufacturing plant by a combined two-phase partition system washing and microbial degradation process. Environmental Science and Pollution Research, 22, 12001–12010.
- Goodarzi, F., & Mukhopadhyay, P. K. (2000). Metals and polyaromatic hydrocarbons in the drinking water of the Sydney Basin, Nova Scotia, Canada: a preliminary assessment of their source. International Journal of Coal Geology, 43, 357–372.
- Gray, R. H. (1984). Chemical and toxicological aspects of coal liquefaction and other complex mixtures. Regulatory Toxicology and Pharmacology, 4, 380–390.
- Grossman, J. N., Stern, A. P., Kirich, M. L., & Kahan, T. F. (2016). Anthracene and pyrene photolysis kinetics in aqueous, organic, and mixed aqueous-organic phases. Atmospheric Environment, 128, 158–164.
- Guillen, M. D., Sopelana, P., & Partearroyo, M. A. (2000). Polycyclic aromatic hydrocarbons in liquid smoke flavorings obtained from different types of wood. Effect of storage in polyethylene flasks on their concentrations. Journal of Agricultural and Food Chemistry, 48, 5083–5087.
- Guillén, M. D., Sopelana, P., & Partearroyo, M. A. (1997). Food as a source of polycyclic aromatic carcinogens. Reviews on Environmental Health, 12, 133–146.
- Gul, O., Dervisoglu, M., Mortas, M., Aydemir, O., Ilhan, E., & Aksehir, K. (2015). Evaluation of polycyclic aromatic hydrocarbons in Circassian cheese by high-performance liquid chromatography with fluorescence detection. Journal of Food Composition and Analysis, 37, 82–86.
- Gunter, M. J., Divi, R. L., Kulldorff, M., Vermeulen, R., Haverkos, K. J., & Kuo, M. M. (2003). Leukocyte polycyclic aromatic hydrocarbon-DNA adduct formation and colorectal adenoma”. Carcinogenesis, 28, 1426–1429.
- Guo, L., Tan, S., Li, X., & Lee, H. K. (2016). Fast automated dual-syringe based dispersive liquid-liquid microextraction coupled with gas chromatography-mass spectrometry for the determination of polycyclic aromatic hydrocarbons in environmental water samples. Journal of Chromatography A, 1438, 1–9.
- Guo, M., Gong, Z., Allinson, G., Tai, P., Miao, R., Li, X., … Zhuang, J. (2016). Variations in the bioavailability of polycyclic aromatic hydrocarbons in industrial and agricultural soils after bioremediation. Chemosphere, 144, 1513–1520.
- Haddaoui, I., Mahjoub, O., Mahjoub, B., Boujelben, A., & Di Bella, G. (2016). Occurrence and distribution of PAHs, PCBs, and chlorinated pesticides in Tunisian soil irrigated with treated wastewater. Chemosphere, 146, 195–205.
- Hafner, C., Gartiser, S., Garcia-Käufer, M., Schiwy, S., Hercher, C., Meyer, W., Achten, C., Larsson, M., Engwall, M., Keiter, S., & Hollert, H. (2015). Investigations on sediment toxicity of German rivers applying a standardized bioassay battery. Environmental Science and Pollution Research,.
- Hahladakis, J. N., Lekkas, N., Smponias, A., & Gidarakos, E. (2014). Sequential application of chelating agents and innovative surfactants for the enhanced electroremediation of real sediments from toxic metals and PAHs. Chemosphere, 105, 44–52.
- Hakura, A., & Sonoda, J. (2013). Benzo[A]pyrene and colonic cancer (pp. 43–78). Chemical Properties, Biochemistry Applications and Toxic Effects: Pyrene.
- Hallare, A. V., Bolinao, J. M. N., Go, J. G. T., Rubio, P. Y. M., Faderl, C., & Macabeo, A. P. G. (2016). Comparative toxicological analysis of polycyclic aromatic hydrocarbon (PAH)-rich soot extracts from gasoline and LPG-fueled taxis using the zebrafish embryo toxicity (ZFET) test. Research Journal of Pharmaceutical, Biological and Chemical Sciences, 7, 159–169.
- Hamdi, H., Benzarti, S., Aoyama, I., & Jedidi, N. (2012). Rehabilitation of degraded soils containing aged PAHs based on phytoremediation with alfalfa (Medicago sativa L.). International Biodeterioration & Biodegradation, 67, 40–47.
- Han, B., Bai, Z., Liu, Y., You, Y., Xu, J., Zhou, J., … Ding, X. (2015). Characterizations, relationship, and potential sources of outdoor and indoor particulate matter bound polycyclic aromatic hydrocarbons (PAHs) in a community of Tianjin, Northern China. Indoor Air, 25, 320–328.
- Hanedar, A., Alp, K., Kaynak, B., & Avşar, E. (2014). Toxicity evaluation and source apportionment of Polycyclic Aromatic Hydrocarbons (PAHs) at three stations in Istanbul, Turkey. Science of the Total Environment, 488–489, 437–446.
- Hao, X., Li, J., & Yao, Z. (2016). Changes in PAHs levels in edible oils during deep-frying process. Food Control, 66, 233–240.
- Haritash, A. K. & Kaushik, C. P. (2009). Biodegradation aspects of Polycyclic Aromatic Hydrocarbons (PAHs): A review. Journal of Hazardous Materials, 169(1–3), 1–15.
- Harmens, H., Foan, L., Simon, V., & Mills, G. (2013). Terrestrial mosses as biomonitors of atmospheric POPs pollution: A review. Environmental Pollution, 173, 245–254.
- Harvey, R. G. (1997). Polycyclic aromatic hydrocarbons. New York, NY: Wiley-VCH.
- Hashemi, H., Ebrahimi, A., Mokhtari, M., & Jasemizad, T. (2016). Removal of PAHs and heavy metals in composting leachate using the anaerobic migrating blanket reactor (AMBR) process. Desalination and Water Treatment, 57, 24960–24969.
- Hassanvand, M. S., Naddafi, K., Faridi, S., Nabizadeh, R., Sowlat, M. H., Momeniha, F., … Yunesian, M. (2015). Characterization of PAHs and metals in indoor/outdoor PM10/PM2.5/PM1 in a retirement home and a school dormitory. Science of The Total Environment, 527–528, 100–110.
- He, X., Pang, Y., Song, X., Chen, B., Feng, Z., & Ma, Y. (2014). Distribution, sources and ecological risk assessment of PAHs in surface sediments from Guan River Estuary, China. Marine Pollution Bulletin, 80, 52–58.
- He, Y. & Chi, J. (2016). Phytoremediation of sediments polluted with phenanthrene and pyrene by four submerged aquatic plants. Journal of Soils and Sediments, 16, 309–317.
- He, Y., Meng, W., Xu, J., Zhang, Y., & Guo, C. (2016). Spatial distribution and potential toxicity of polycyclic aromatic hydrocarbons in sediments from Liaohe River Basin, China. Environmental Monitoring and Assessment, 188(3), 1–10.
- Heintzman, L. J., Anderson, T. A., Carr, D. L., & McIntyre, N. E. (2015). Local and landscape influences on PAH contamination in urban stormwater. Landscape and Urban Planning, 142, 29–37.
- Hemat, H., Wittsiepe, J., Wilhelm, M., Müller, J., & Göen, T. (2012). High levels of 1-hydroxypyrene and hydroxyphenanthrenes in urine of children and adults from Afghanistan. Journal of Exposure Science and Environmental Epidemiology, 22, 46–51.
- Heydari Sereshk, Z., & Riyahi Bakhtiari, A. (2014). Distribution patterns of PAHs in different tissues of annulated sea snake (Hydrophis cyanocinctus) and short sea snake (Lapemis curtus) from the Hara Protected Area on the North Coast of the Persian Gulf, Iran. Ecotoxicology and Environmental Safety, 109, 116–123.
- Hijosa-Valsero, M., Bécares, E., Fernández-Aláez, C., Fernández-Aláez, M., Mayo, R., & Jiménez, J. J. (2016). Chemical pollution in inland shallow lakes in the Mediterranean region (NW Spain): PAHs, insecticides and herbicides in water and sediments. Science of The Total Environment, 544, 797–810.
- Hong, S., Yim, U. H., Ha, S. Y., Shim, W. J., Jeon, S., Lee, S., … Khim, J. S. (2016). Bioaccessibility of AhR-active PAHs in sediments contaminated by the Hebei Spirit oil spill: Application of Tenax extraction in effect-directed analysis. Chemosphere, 144, 706–712.
- Hong, W.-J., Jia, H., Li, Y.-F., Sun, Y., Liu, X., & Wang, L. (2016). Polycyclic aromatic hydrocarbons (PAHs) and alkylated PAHs in the coastal seawater, surface sediment and oyster from Dalian, Northeast China. Ecotoxicology and Environmental Safety, 128, 11–20.
- Hong, Y. W., Yuan, D. X., Lin, Q. M., & Yang, T. L. (2008). Accumulationandbiodegradation of phenanthrene and fluoranthene by the algae enriched from a mangrove aquatic ecosystem. Mar. Poll. Bull., 56, 1400–1405.
- Hoseini, M., Yunesian, M., Nabizadeh, R., Yaghmaeian, K., Ahmadkhaniha, R., Rastkari, N., … Naddafi, K. (2016). Characterization and risk assessment of polycyclic aromatic hydrocarbons (PAHs) in urban atmospheric Particulate of Tehran, Iran. Environmental Science and Pollution Research, 23, 1820–1832.
- Hsu, Y. M., Harner, T., Li, H., & Fellin, P. (2015). PAH measurements in air in the Athabasca oil sands region. Environmental Science and Technology, 49, 5584–5592.
- Hu, C., He, M., Chen, B., Zhong, C., & Hu, B. (2014). Sorptive extraction using polydimethylsiloxane/metal-organic framework coated stir bars coupled with high performance liquid chromatography-fluorescence detection for the determination of polycyclic aromatic hydrocarbons in environmental water samples. Journal of Chromatography A, 1356, 45–53.
- Hu, J., Liu, C., Zhang, G., Zhang, Y., Li, S., Zhao, Z., … Guo, Q. (2016). Distribution characteristics and source apportionment of polycyclic aromatic hydrocarbons (PAHs) in the Liao River drainage basin, northeast China. Environmental Monitoring and Assessment, 188(4), 1–12.
- Hu, Y., Bai, Z., Zhang, L., Wang, X., Zhang, L., Yu, Q., & Zhu, T. (2007). Health risk assessment for traffic policemen exposed to polycyclic aromatic hydrocarbons (PAHs) in Tianjin, China. Science of The Total Environment, 382, 240–250.
- Hu, Y., Li, G., Yan, M., Ping, C., & Ren, J. (2014). Investigation into the distribution of polycyclic aromatic hydrocarbons (PAHs) in wastewater sewage sludge and its resulting pyrolysis bio-oils. Science of The Total Environment, 473–474, 459–464.
- Hua, H., Zhao, X., Wu, S., & Li, G. (2016). Impact of refining on the levels of 4-hydroxy-trans-alkenals, parent and oxygenated polycyclic aromatic hydrocarbons in soybean and rapeseed oils. Food Control, 67, 82–89.
- Hua, L., Wu, W.-X., Liu, Y.-X., Tientchen, C. M., & Chen, Y.-X. (2008). Heavy Metals and PAHs in Sewage Sludge from Twelve Wastewater Treatment Plants in Zhejiang Province. Biomedical and Environmental Sciences, 21, 345–352.
- Huang, H., Wu, K., Khan, A., Jiang, Y., Ling, Z., Liu, P., … Li, X. (2016). A novel Pseudomonas gessardii strain LZ-E simultaneously degrades naphthalene and reduces hexavalent chromium. Bioresource Technology, 207, 370–378.
- Huang, L., & Batterman, S. A. (2014). Multimedia model for polycyclic aromatic hydrocarbons (PAHs) and nitro-PAHs in Lake Michigan. Environmental Science and Technology, 48, 13817–13825.
- Huang, L., Chernyak, S. M., & Batterman, S. A. (2014). PAHs (polycyclic aromatic hydrocarbons), nitro-PAHs, and hopane and sterane biomarkers in sediments of southern Lake Michigan, USA. Science of the Total Environment, 487, 173–186.
- Huang, M., & Penning, T. M. (2014). Processing Contaminants: Polycyclic Aromatic Hydrocarbons (PAHs). In Y. Motarjemi (Ed.), Encyclopedia of Food Safety (pp. 416–423). Waltham: Academic Press.
- Huang, S., He, S., Xu, H., Wu, P., Jiang, R., Zhu, F., … Ouyang, G. (2015). Monitoring of persistent organic pollutants in seawater of the Pearl River Estuary with rapid on-site active SPME sampling technique. Environmental Pollution, 200, 149–158.
- HuiJie, L., CaiYun, Y., Yun, T., GuangHui, L., & TianLing, Z. (2011). Using population dynamics analysis by DGGE to design the bacterial consortium isolated from mangrove sediments for biodegradation of PAHs. International Biodeterioration & Biodegradation, 65, 269–275.
- Hung, C. V., Cam, B. D., Mai, P. T. N., & Dzung, B. Q. (2014). Heavy metals and polycyclic aromatic hydrocarbons in municipal sewage sludge from a river in highly urbanized metropolitan area in Hanoi, Vietnam: levels, accumulation pattern and assessment of land application. Environmental Geochemistry and Health, 37, 133–146.
- Hussain, K., Balachandran, S., & Hoque, R. R. (2015). Sources of polycyclic aromatic hydrocarbons in sediments of the Bharalu River, a tributary of the River Brahmaputra in Guwahati, India. Ecotoxicology and Environmental Safety, 122, 61–67.
- Hussain, K., Rahman, M., Prakash, A., & Hoque, R. R. (2015). Street dust bound PAHs, carbon and heavy metals in Guwahati city – Seasonality, toxicity and sources. Sustainable Cities and Society, 19, 17–25.
- Isaac, P., Martínez, F. L., Bourguignon, N., Sánchez, L. A., & Ferrero, M. A. (2015). Improved PAHs removal performance by a defined bacterial consortium of indigenous Pseudomonas and actinobacteria fromPatagonia, Argentina. International Biodeterioration and Biodegradation, 101, 23–31.
- Ishizaki, A., Sito, K., & Kataoka, H. (2011). Analysis of contaminant polycyclic aromatic hydrocarbons in tea products and crude drugs. Analytical Methods, 3, 299–305.
- Islam, M. N., Jo, Y.-T., Jung, S.-K., & Park, J.-H. (2013). Thermodynamic and kinetic study for subcritical water extraction of PAHs. Journal of Industrial and Engineering Chemistry, 19, 129–136.
- Ivan, J., Hudák, A., Szeghyová, Z., Verbová, E., & Majoroš, J. (2011). Detection of 1-hydroxypyrene in urine of a coke plant workers. Pracovni Lekarstvi, 63, 63–71.
- Ivshina, I., Kostina, L., Krivoruchko, A., Kuyukina, M., Peshkur, T., Anderson, P., & Cunningham, C. (2016). Removal of polycyclic aromatic hydrocarbons in soil spiked with model mixtures of petroleum hydrocarbons and heterocycles using biosurfactants from Rhodococcus ruber IEGM 231. Journal of Hazardous Materials, 312, 8–17.
- Iwegbue, C. M. A., & Bassey, F. I. (2013). Concentrations and health hazards of polycyclic aromatic hydrocarbons in selected commercial brands of milk. Journal of Food Measurement and Characterization, 7, 177–184.
- Iwegbue, C. M. A., Overah, L. C., Tesi, G. O., Bassey, F. I., & Martincigh, B. S. (2014). Polycyclic Aromatic Hydrocarbon Profiles of Some Brands of Canned Fish in the Nigerian Market. Human and Ecological Risk Assessment, 21, 157–168.
- Janoszka, B. (2011). HPLC-fluorescence analysis of polycyclic aromatic hydrocarbons (PAHs) in pork meat and its gravy fried without additives and in the presence of onion and garlic. Food Chemistry, 126, 1344–1353.
- Jariyasopit, N., Liu, Y., Liggio, J., & Harner, T. (2015). Stability of polycyclic aromatic compounds in polyurethane foam-type passive air samplers upon O3 exposure. Atmospheric Environment, 120, 200–204.
- Jarvis, I. W. H., Dreij, K., Mattsson, A., Jernström, B., & Stenius, U. (2014). Interactions between polycyclic aromatic hydrocarbons in complex mixtures and implications for cancer risk assessment. Toxicology, 321, 27–39.
- Jedrychowski, W. A., Perera, F. P., Camann, D., Spengler, J., Butscher, M., Mroz, E., … Sowa, A. (2015). Prenatal exposure to polycyclic aromatic hydrocarbons and cognitive dysfunction in children. Environmental Science and Pollution Research, 22, 3631–3639.
- Jeng, H. A., Pan, C. H., Lin, W. Y., Wu, M. T., Taylor, S., Chang-Chien, G. P., … Diawara, N. (2013). Biomonitoring of polycyclic aromatic hydrocarbons from coke oven emissions and reproductive toxicity in nonsmoking workers. Journal of Hazardous Materials, 244–245, 436–443.
- Jerina, D. M., van Bladeren, P. J., Yagi, H., Gibson, D. T., Mahadevan, V., Neese, A. S., … Bo, D. R. (1984). Synthesis and absolute configuration of the bacterial cis-1,2-, cis-8,9-, and cis-10,11dihydrodiol metabolites of benz[a]anthracene formed by a strain of Beijerinckia. J. Org. Chem., 49, 3621–3628.
- Ji, D., Yang, Q., Wang, Y., & Xi, H. (2015). Estimation of phenanthrene degradation model by Sphingobacterium multivorum isolated from municipal sludge. Fresenius Environmental Bulletin, 24, 48–55.
- Jiang, Y., Yang, Y., & Zhang, X. (2014). Review on the biodegradation and conversion mechanisms of typical polycyclic aromatic hydrocarbons. Shiyou Xuebao, Shiyou Jiagong/Acta Petrolei Sinica [Petroleum Processing Section], 30, 1137–1150.
- Jiang, Y., Yves, U. J., Sun, H., Hu, X., Zhan, H., & Wu, Y. (2016). Distribution, compositional pattern and sources of polycyclic aromatic hydrocarbons in urban soils of an industrial city, Lanzhou, China. Ecotoxicology and Environmental Safety, 126, 154–162.
- Jimenez, A., Adisa, A., Woodham, C., & Saleh, M. (2014). Determination of polycyclic aromatic hydrocarbons in roasted coffee. Journal of Environmental Science and Health - Part B Pesticides, Food Contaminants, and Agricultural Wastes, 49, 828–835.
- Jin, G., Cong, L., Wang, H., He, M., Li, J., Piao, X., … Li, D. (2014). A simple and rapid analysis for gas-phase polycyclic aromatic hydrocarbons using an organic-solvent-based method. Atmospheric Environment, 89, 367–372.
- Jin, Q., Pan, L., Liu, D., Hu, F., & Xiu, M. (2014). Assessing PAHs pollution in Qingdao coastal area (China) by the combination of chemical and biochemical responses in scallops, Chlamys farreri. Marine Pollution Bulletin, 89, 473–480.
- Jobin, P., Coudert, L., Taillard, V., Blais, J. F., & Mercier, G. (2016). Remediation of inorganic contaminants and polycyclic aromatic hydrocarbons from soils polluted by municipal solid waste incineration residues. Environmental Technology (United Kingdom, 37, 1983–1995.
- John, K., Ragavan, N., Pratt, M. M., Singh, P. B., Al‐Buheissi, S., & Matanhelia, S. S. (2009). Quantification of phase I/II metabolizing enzyme gene expression and polycyclic aromatichydrocarbon-DNA adduct levels in human prostate. Prostate, 69, 505–519.
- Johnsen, A. R., Winding, A., Karlson, U., & Roslev, P. (2002). Linking of micro-organisms to phenanthrene metabolism in soil by analysis of C-labelled cell-lipids. Appl. Environ. Microbiol., 68, 6106–6113.
- Johnston, G. P., Lineman, D., Johnston, C. G., & Leff, L. (2015). Characterization, sources and ecological risk assessment of polycyclic aromatic hydrocarbons (PAHs) in long-term contaminated riverbank sediments. Environmental Earth Sciences, 74, 3519–3529.
- Jones, O. A. H., Spurgeon, D. J., Svendsen, C., & Griffin, J. L. (2008). A metabolomics based approach to assessing the toxicity of the polyaromatic hydrocarbon pyrene to the earthworm Lumbricus rubellus. Chemosphere, 71, 601–608.
- Jove, P., Olivella, M. A., Camarero, S., Caixach, J., Planas, C., Cano, L., & De Las Heras, F. X. (2016). Fungal biodegradation of anthracene-polluted cork: A comparative study. Journal of Environmental Science and Health - Part A Toxic/Hazardous Substances and Environmental Engineering, 51, 70–77.
- Ju, J.-H., Lee, I.-S., Sim, W.-J., Eun, H., & Oh, J.-E. (2009). Analysis and evaluation of chlorinated persistent organic compounds and PAHs in sludge in Korea. Chemosphere, 74, 441–447.
- Jung, K. H., Lovinsky-Desir, S., Perzanowski, M., Liu, X., Maher, C., Gil, E., … Miller, R. L. (2015). Repeatedly high polycyclic aromatic hydrocarbon exposure and cockroach sensitization among inner-city children. Environmental Research, 140, 649–656.
- Kem, S. (2007). Monitoring of environmental exposure to polycyclic aromatic hydrocarbons: a re-view. Environ Chem Lett, 5, 169–195.
- Kafilzadeh, F. (2015). Distribution and sources of polycyclic aromatic hydrocarbons in water and sediments of the Soltan Abad River, Iran. Egyptian Journal of Aquatic Research, 37, 1983–1983.
- Kalwa, M., Quináia, S. P., Pletsch, A. L., Torres, Y. R., & Finger, D. (2014). Polycyclic aromatic hydrocarbons in surface sediments of Binacional Itaipu Lake, Brazil: characteristics, sources and toxicity evaluation. Environmental Earth Sciences, 72, 4473–4481.
- Kamal, A., Cincinelli, A., Martellini, T., & Malik, R. N. (2015). A review of PAH exposure from the combustion of biomass fuel and their less surveyed effect on the blood parameters. Environmental Science and Pollution Research, 22, 4076–4098.
- Kamal, A., Malik, R. N., Martellini, T., & Cincinelli, A. (2014). Cancer risk evaluation of brick kiln workers exposed to dust bound PAHs in Punjab province (Pakistan). Science of the Total Environment, 493, 562–570.
- Kanaly, R. A., & Harayama, S. (2000). Biodegradation of High-Molecular-Weight Polycyclic Aromatic Hydrocarbons by Bacteria. J. Bacterology, 182, 2059–2067.
- Kang, B., Lee, B. M., & Shin, H. S. (2014). Determination of polycyclic aromatic hydrocarbon (PAH) content and risk assessment from edible oils in Korea. Journal of Toxicology and Environmental Health - Part A: Current Issues, 77, 1359–1371.
- Kangsadalampai, K., Butryee, C., & Manoonphol, K. (1997). Direct mutagenicity of the polycylic aromatic hydrocarbon-containing fraction of smoked and charcoal-broiled foods treated with nitrite in acid solution. Food and Chemical Toxicology, 35, 213–218.
- Kanhai, L. D. K., Gobin, J. F., Beckles, D. M., Lauckner, B., & Mohammed, A. (2014). Polycyclic aromatic hydrocarbons (PAHs) in Crassostrea rhizophorae and Cathorops spixii from the Caroni Swamp, Trinidad, West Indies. Environmental Science and Pollution Research, 22, 1366–1379.
- Kanzari, F., Asia, L., Syakti, A. D., Piram, A., Malleret, L., Mille, G., & Doumenq, P. (2015). Distribution and risk assessment of hydrocarbons (aliphatic and PAHs), polychlorinated biphenyls (PCBs), and pesticides in surface sediments from an agricultural river (Durance) and an industrialized urban lagoon (Berre lagoon), France. Environmental Monitoring and Assessment, 187, 591.
- Kanzari, F., Syakti, A. D., Asia, L., Malleret, L., Piram, A., Mille, G., & Doumenq, P. (2014). Distributions and sources of persistent organic pollutants (aliphatic hydrocarbons, PAHs, PCBs and pesticides) in surface sediments of an industrialized urban river (Huveaune), France. Science of the Total Environment, 478, 141–151.
- Kara, G., & Aydin, M. E. (2015). Risk Assessment of 26 Polycyclic Aromatic Hydrocarbon (PAH) Compounds in Parkıng Garages. Environmental Forensics, 16, 205–216.
- Karaca, G. (2016). Spatial Distribution of Polycyclic Aromatic Hydrocarbon (PAH) Concentrations in Soils from Bursa, Turkey. Archives of Environmental Contamination and Toxicology, 70, 406–417.
- Karaca, G., & Tasdemir, Y. (2015). Application of Advanced Oxidation Processes for Polycyclic Aromatic Hydrocarbons Removal from Municipal Treatment Sludge. Clean - Soil, Air, Water, 43, 191–196.
- Karimi, B., Habibi, M., & Esvand, M. (2015). Biodegradation of naphthalene using Pseudomonas aeruginosa by up flow anoxic-aerobic continuous flow combined bioreactor. Journal of Environmental Health Science and Engineering, 13, 26.
- Karimi, P., Peters, K. O., Bidad, K., & Strickland, P. T. (2015). Polycyclic aromatic hydrocarbons and childhood asthma. European Journal of Epidemiology, 30, 91–101.
- Karl, H., & Leinemann, M. (1996). Determination of polycyclic aromatic hydrocarbons in smoked fishery products from different smoking kilns. Zeitschrift fur Lebensmittel -Untersuchung und -Forschung, 202, 458–464.
- Katsoyiannis, A., Terzi, E., & Cai, Q.-Y. (2007). On the use of PAH molecular diagnostic ratios in sewage sludge for the understanding of the PAH sources. Is this use appropriate? Chemosphere, 69, 1337–1339.
- Kauneliene, V., Krugly, E., Kliucininkas, L., Stasiulaitiene, I., Prasauskas, T., Auzbikaviciute, A., … Martuzevicius, D. (2015). PAHs in Indoor and Outdoor Air from Decentralized Heating Energy Production: Comparison of Active and Passive Sampling. Polycyclic Aromatic Compounds, 36, 410–428.
- Kayali-Sayadi, M. N. (1998). Rapid determination of polycyclic aromatic hydrocarbons in tea infusion samples by high-performance liquid chromatography and fluorimetric detection based on solid-phase extraction. Analyst, 123, 2145–2148.
- Kendall, M., Duarte, A., Rocha-Santos, T., Hamilton, R., & Williams, I. (2002). Airborne particulate-associated polyaromatic hydrocarbons, n-alkanes, elemental and organic carbon in three European cities. Journal of Environmental Monitoring, 4, 890–896.
- Keshavarzi, B., Mokhtarzadeh, Z., Moore, F., Rastegari Mehr, M., Lahijanzadeh, A., Rostami, S., & Kaabi, H. (2015). Heavy metals and polycyclic aromatic hydrocarbons in surface sediments of Karoon River, Khuzestan Province, Iran. Environmental Science and Pollution Research, 22, 19077–19092.
- Keshavarzifard, M., Zakaria, M. P., Shau Hwai, T., Yusuff, F. F. M., Mustafa, S., Vaezzadeh, V., … Abootalebi-Jahromi, F. (2014). Baseline distributions and sources of Polycyclic Aromatic Hydrocarbons (PAHs) in the surface sediments from the Prai and Malacca Rivers, Peninsular Malaysia. Marine Pollution Bulletin, 88, 366–372.
- Khadhar, S., Higashi, T., Hamdi, H., Matsuyama, S., & Charef, A. (2010). Distribution of 16 EPA-priority polycyclic aromatic hydrocarbons (PAHs) in sludges collected from nine Tunisian wastewater treatment plants. Journal of Hazardous Materials, 183, 98–102.
- Khan, M. F., Latif, M. T., Lim, C. H., Amil, N., Jaafar, S. A., Dominick, D., … Tahir, N. M. (2015). Seasonal effect and source apportionment of polycyclic aromatic hydrocarbons in PM2.5. Atmospheric Environment, 106, 178–190.
- Khanjari, Y., Eikani, M. H., & Rowshanzamir, S. (2016). Remediation of polycyclic aromatic hydrocarbons from soil using superheated water extraction. Journal of Supercritical Fluids, 111, 129–134.
- Khatisashvili, G., Matchavariani, L., & Gakhokidze, R. (2015). Chapter 19 - Improving Phytoremediation of Soil Polluted with Oil Hydrocarbons in Georgia. In K. R. H. S. Ö. R. Mermut (Ed.), Soil Remediation and Plants (pp. 547–569). San Diego, CA: Academic Press.
- Khudhair, A. B., Hadibarata, T., Yusoff, A. R. M., Teh, Z. C., Adnan, L. A., & Kamyab, H. (2015). Pyrene metabolism by new species isolated from soil Rhizoctonia zeae SOL3. Water, Air, and Soil Pollution, 226, 186.
- Kim, B. M., Lee, S.-B., Kim, J. Y., Kim, S., Seo, J., Bae, G.-N., & Lee, J. Y. (2016). A multivariate receptor modeling study of air-borne particulate PAHs: Regional contributions in a roadside environment. Chemosphere, 144, 1270–1279.
- Kim, M. J., Hwang, J. H., & Shin, H. S. (2014). Evaluation of polycyclic aromatic hydrocarbon contents and risk assessment for fish and meat products in Korea. Food Science and Biotechnology, 23, 991–998.
- Kim, Y. H., Tishbee, A., & Gil-Av, E. (1981). Optical resolution of mutagenic and carcinogenic derivatives of polyaromatic hydrocarbons by high pressure liquid chromatography on a chiral support. Journal of the Chemical Society, Chemical Communications, 3, 75–76.
- Kingham, S., Briggs, D., Elliott, P., Fischer, P., & Erik, L. (2000). Spatial variations in the concentrations of traffic-related pollutants in indoor and outdoor air in Huddersfield, England. Atmospheric Environment, 34, 905–916.
- Klein, S. J. (2014). Acenaphthene. In P. Wexler (Ed.), Encyclopedia of Toxicology (Third Edition) (pp. 17–19). Oxford: Academic Press.
- Kobayashi, T., & Sumida, H. (2015). Effects of humic acids on the sorption and bioavailability of pyrene and 1,2-dihydroxynaphthalene. Soil Science and Plant Nutrition, 61, 113–122.
- Kong, S., Li, X., Li, L., Yin, Y., Chen, K., Yuan, L., … Ji, Y. (2015). Variation of polycyclic aromatic hydrocarbons in atmospheric PM2.5 during winter haze period around 2014 Chinese Spring Festival at Nanjing: Insights of source changes, air mass direction and firework particle injection. Science of the Total Environment, 520, 59–72.
- Kozielska, B., & Konieczyński, J. (2015). Polycyclic aromatic hydrocarbons in particulate matter emitted from coke oven battery. Fuel, 144, 327–334.
- Kpoclou, E. Y., Anihouvi, V. B., Azokpota, P., Soumanou, M. M., Douny, C., Brose, F., … Scippo, M. L. (2014). Effect of fuel and kiln type on the polycyclic aromatic hydrocarbon (PAH) levels in smoked shrimp, a Beninese food condiment. Food Additives and Contaminants - Part A Chemistry, Analysis, Control, Exposure and Risk Assessment, 31, 1212–1218.
- Krajian, H., & Odeh, A. (2014). Polycyclic aromatic hydrocarbons in Syrian olive oils and their likely daily intakes. Toxicological and Environmental Chemistry, 96, 342–342.
- Krauss, M., Wilcke, W., & Zech, W. (2000). Availability of Polycyclic Aromatic Hydrocarbons (PAHs) and Polychlorinated Biphenyls (PCBs) to Earthworms in Urban Soils. Environmental Science & Technology, 34, 4335–4340.
- Kronholm, J., Desbands, B., Hartonen, K., & Riekkola, M.-L. (2002). Environmentally friendly laboratory-scale remediation of PAH-contaminated soil by using pressurized hot water extraction coupled with pressurized hot water oxidation. Green Chemistry, 4, 213–219.
- Kumar, V., Kothiyal, N. C., & Saruchi (2015). Analysis of Polycyclic Aromatic Hydrocarbon, Toxic Equivalency Factor and Related Carcinogenic Potencies in Roadside Soil within a Developing City of Northern India. Polycyclic Aromatic Compounds, 36, 506–506.
- Kuo, C. Y., Hsu, Y. W., & Lee, H. S. (2003). Study of human exposure to particulate PAHs using personal air samplers. Arch Environ Contam Toxicol, 44, 454–459.
- Kuppusamy, S., Thavamani, P., Megharaj, M., & Naidu, R. (2016). Biodegradation of polycyclic aromatic hydrocarbons (PAHs) by novel bacterial consortia tolerant to diverse physical settings - Assessments in liquid- and slurry-phase systems. International Biodeterioration and Biodegradation, 108, 149–157.
- Kwon, H.-O., & Choi, S.-D. (2014). Polycyclic aromatic hydrocarbons (PAHs) in soils from a multi-industrial city, South Korea. Science of The Total Environment, 470–471, 1494–1501.
- Lacroix, C., Richard, G., Seguineau, C., Guyomarch, J., Moraga, D., & Auffret, M. (2015). Active and passive biomonitoring suggest metabolic adaptation in blue mussels (Mytilus spp.) chronically exposed to a moderate contamination in Brest harbor (France). Aquatic Toxicology, 162, 126–137.
- Laitinen, A., Michaux, A., & Aaltonen, O. (1994). Soil cleaning by carbon-dioxide extraction - A review. Environmental Technology, 15, 715–727.
- Lan, J. C., Sun, Y. C., Shi, Y., & Liang, Z. B. (2015). Contamination and ecological risk assessment of polycyclic aromatic hydrocarbons in surface sediment in karst underground river. Huanjing Kexue/Environmental Science, 36, 855–861.
- Lan, J. C., Sun, Y. C., Tian, P., Lu, B. Q., Shi, Y., Xu, X., … Yang, P. H. (2014). Contamination and ecological risk assessment of polycyclic aromatic hydrocarbons in water and in karst underground river catchment. Huanjing Kexue/Environmental Science, 35, 3722–3730.
- Larsson, B. K. (1982). Polycyclic aromatic hydrocarbons in smoked fish. Zeitschrift für Lebensmittel-Untersuchung und -Forschung, 174, 101–107.
- Larsson, B. K., Sahlberg, G. P., Eriksson, A. T., & Busk, L. Å. (1983). Polycyclic aromatic hydrocarbons in grilled food. Journal of Agricultural and Food Chemistry, 31, 867–873.
- Lau, E. V., Gan, S., Ng, H. K., & Poh, P. E. (2014). Extraction agents for the removal of polycyclic aromatic hydrocarbons (PAHs) from soil in soil washing technologies. Environmental Pollution, 184, 640–649.
- Lawrence, J. F., & Weber, D. F. (1984). Determination of polycyclic aromatic hydrocarbons in some canadian commercial fish, shellfish, and meat products by liquid chromatography with confirmation by capillary gas chromatography-mass spectrometry. Journal of Agricultural and Food Chemistry, 32, 789–794.
- Ledesma, E., Rendueles, M., & Díaz, M. (2014). Benzo(a)pyrene penetration on a smoked meat product during smoking time. Food Additives and Contaminants - Part A Chemistry, Analysis, Control, Exposure and Risk Assessment, 31, 1688–1698.
- Lee, H., Jang, Y., Choi, Y. S., Kim, M. J., Lee, J., Lee, H., … Kim, J. J. (2014). Biotechnological procedures to select white rot fungi for the degradation of PAHs. Journal of Microbiological Methods, 97, 56–62.
- Lee, J. G., Kim, S. Y., Moon, J. S., Kim, S. H., Kang, D. H., & Yoon, H. J. (2016). Effects of grilling procedures on levels of polycyclic aromatic hydrocarbons in grilled meats. Food Chemistry, 199, 632–638.
- Lei, A. P., Hu, Z., Wong, Y. S., & Tam, N. F. Y. (2007). Removal of fluoranthene and pyrene by different microalgal species. Bioresour. Technol., 98, 273–280.
- Leung, A. O. W., Cheung, K. C., & Wong, M. H. (2015). Spatial distribution of polycyclic aromatic hydrocarbons in soil, sediment, and combusted residue at an e-waste processing site in southeast China. Environmental Science and Pollution Research, 22, 8786–8801.
- Levengood, J. M., & Schaeffer, D. J. (2011). Polycyclic aromatic hydrocarbons in fish and crayfish from the Calumet region of southwestern Lake Michigan. Ecotoxicology, 20, 1411–1421.
- Li, B., Feng, C., Li, X., Chen, Y., Niu, J., & Shen, Z. (2012). Spatial distribution and source apportionment of PAHs in surficial sediments of the Yangtze Estuary, China. Marine Pollution Bulletin, 64, 636–643.
- Li, D., Huijbregts, M. A. J., & Jolliet, O. (2015). Life cycle health impacts of polycyclic aromatic hydrocarbon for source-specific mixtures. International Journal of Life Cycle Assessment, 20, 87–99.
- Li, F. S., Han, C., Zhou, B. H., Xu, Z. B., Wang, Y., Lin, D. S., … Jin, T. S. (2016). Distribution and source analysis of polycyclic aromatic hydrocarbons in indoor dust from Anhui Province, China. Zhongguo Huanjing Kexue/China Environmental Science, 36, 363–369.
- Li, G.-L., Lang, Y.-H., Gao, M.-S., Yang, W., Peng, P., & Wang, X.-M. (2014). Carcinogenic and mutagenic potencies for different PAHs sources in coastal sediments of Shandong Peninsula. Marine Pollution Bulletin, 84, 418–423.
- Li, G., Lang, Y., Yang, W., Peng, P., & Wang, X. (2014). Source contributions of PAHs and toxicity in reed wetland soils of Liaohe estuary using a CMB–TEQ method. Science of The Total Environment, 490, 199–204.
- Li, G., Wu, S., Wang, L., & Akoh, C. C. (2016). Concentration, dietary exposure and health risk estimation of polycyclic aromatic hydrocarbons (PAHs) in youtiao, a Chinese traditional fried food. Food Control, 59, 328–336.
- Li, G. L., Lang, Y. H., Gao, M. S., Yang, W., Peng, P., & Wang, X. M. (2014). Carcinogenic and mutagenic potencies for different PAHs sources in coastal sediments of Shandong Peninsula. Marine Pollution Bulletin, 84, 418–423.
- Li, H., Duan, D., Huang, W., & Ran, Y. (2014). Seasonal distribution, sources, and in-situ partitioning of polycyclic aromatic hydrocarbons in water and suspended particulate matters from the Pearl River Delta. Huanjing Kexue Xuebao/Acta Scientiae Circumstantiae, 34, 2963–2972.
- Li, J., Dong, H., Li, X., Han, B., Zhu, C., & Zhang, D. (2016). Quantitatively assessing the health risk of exposure to PAHs from intake of smoked meats. Ecotoxicology and Environmental Safety, 124, 91–95.
- Li, J. L., Wang, Y. X., Zhang, C. X., Dong, Y. H., Du, B., & Liao, X. P. (2014). The source apportionment of polycyclic aromatic hydrocarbons (PAHs) in the topsoil in Xiaodian sewage irrigation area, North of China. Ecotoxicology, 23, 1943–1950.
- Li, P., Xue, R., Wang, Y., Zhang, R., & Zhang, G. (2015). Influence of anthropogenic activities on PAHs in sediments in a significant gulf of low-latitude developing regions, the Beibu Gulf, South China Sea: Distribution, sources, inventory and probability risk. Marine Pollution Bulletin, 90, 218–226.
- Li, P. H., Wang, Y., Li, Y. H., Li, H. L., & Yi, X. (2015). Origin and Distribution of PAHs in Ambient Particulate Samples at High Mountain Region in Southern China. Advances in Meteorology, 2015.
- Li, P. H., Wang, Y., Li, Y. H., Wai, K. M., Li, H. L., & Tong, L. (2016). Gas-particle partitioning and precipitation scavenging of polycyclic aromatic hydrocarbons (PAHs) in the free troposphere in southern China. Atmospheric Environment, 128, 165–174.
- Li, R. L., Liu, B. B., Zhu, Y. X., & Zhang, Y. (2016). Effects of flooding and aging on phytoremediation of typical polycyclic aromatic hydrocarbons in mangrove sediments by Kandelia obovata seedlings. Ecotoxicology and Environmental Safety, 128, 118–125.
- Li, T., Wang, Y., Guo, S., Li, X., Xu, Y., Wang, Y., & Li, X. (2016). Effect of polarity-reversal on electrokinetic enhanced bioremediation of Pyrene contaminated soil. Electrochimica Acta, 187, 567–575.
- Li, W., Wang, C., Shen, H., Su, S., Shen, G., Huang, Y., … Tao, S. (2015). Concentrations and origins of nitro-polycyclic aromatic hydrocarbons and oxy-polycyclic aromatic hydrocarbons in ambient air in urban and rural areas in northern China. Environmental Pollution, 197, 156–164.
- Li, X.-H., Ma, L.-L., Liu, X.-F., Fu, S., Cheng, H.-X., & Xu, X.-B. (2006). Polycyclic aromatic hydrocarbon in urban soil from Beijing, China. Journal of Environmental Sciences, 18, 944–950.
- Li, X. Y., Li, N., Luo, H. D., Lin, L. R., Zou, Z. X., Jia, Y. Z., & Li, Y. Q. (2011). A novel synchronous fluorescence spectroscopic approach for the rapid determination of three polycyclic aromatic hydrocarbons in tea with simple microwave-assisted pretreatment of sample. Journal of Agricultural and Food Chemistry, 59, 5899–5905.
- Li, Y. & Duan, X. (2015). Polycyclic aromatic hydrocarbons in sediments of China Sea. Environmental Science and Pollution Research, 22, 15432–15432.
- Liang, L., Song, X., Kong, J., Shen, C., Huang, T., & Hu, Z. (2014). Anaerobic biodegradation of high-molecular-weight polycyclic aromatic hydrocarbons by a facultative anaerobe Pseudomonas sp. JP1. Biodegradation, 25, 825–833.
- Liang, X., Guo, C., Wei, Y., Lin, W., Yi, X., Lu, G., & Dang, Z. (2016). Cosolubilization synergism occurrence in codesorption of PAH mixtures during surfactant-enhanced remediation of contaminated soil. Chemosphere, 144, 583–590.
- Liang, Y., Tse, M. F., Young, L., & Wong, M. H. (2007). Distribution patterns of polycyclic aromatic hydrocarbons (PAHs) in the sediments and fish at Mai Po Marshes Nature Reserve, Hong Kong. Water Research, 41, 1303–1311.
- Liberti, L., Notarnicola, M., Primerano, R., & Zannetti, P. (2006). Air Pollution from a Large Steel Factory: Polycyclic Aromatic Hydrocarbon Emissions from Coke-Oven Batteries. Journal of the Air & Waste Management Association, 56, 255–260.
- Lijinsky, W., & Ross, A. E. (1967). Production of carcinogenic polynuclear hydrocarbons in the cooking of food. Food and Cosmetics Toxicology, 5, 343–347.
- Lijinsky, W., & Shubik, P. (1965a). The detection of polycyclic aromatic hydrocarbons in liquid smoke and some foods. Toxicology and Applied Pharmacology, 7, 337–343.
- Lijinsky, W., & Shubik, P. (1965). Polynuclear hydrocarbon carcinogens in cooked meat and smoked food. Industrial medicine & surgery, 34, 152–154.
- Lim, H., Mattsson, A., Jarvis, I. W. H., Bergvall, C., Bottai, M., Morales, D. A., … Dreij, K. (2015). Detection of benz[j]aceanthrylene in urban air and evaluation of its genotoxic potential. Environmental Science and Technology, 49, 3101–3109.
- Lin, D., Tu, Y., & Zhu, L. (2005). Concentrations and health risk of polycyclic aromatic hydrocarbons in tea. Food and Chemical Toxicology, 43, 41–48.
- Lin, D. & Zhu, L. (2004). Polycyclic aromatic hydrocarbons: Pollution and source analysis of a black tea. Journal of Agricultural and Food Chemistry, 52, 8268–8271.
- Lin, D., Zhu, L., & Luo, L. (2006). Factors affecting transfer of polycyclic aromatic hydrocarbons from made tea to tea infusion. Journal of Agricultural and Food Chemistry, 54, 4350–4354.
- Lin, M., Ning, X. A., An, T., Zhang, J., Chen, C., Ke, Y., … Liu, J. (2016). Degradation of polycyclic aromatic hydrocarbons (PAHs) in textile dyeing sludge with ultrasound and Fenton processes: Effect of system parameters and synergistic effect study. Journal of Hazardous Materials, 307, 7–16.
- Lindén, O., & Pålsson, J. (2013). Oil contamination in ogoniland, Niger delta. Ambio, 42, 685–701.
- Liu, C., Chen, J., Li, Y., Chen, R., Yuan, G., & Yang, Z. (2014). Level and composition characteristic of PAHs in three typical rivers of China. WIT Transactions on Ecology and the Environment, 189, 885–890.
- Liu, F., Liao, C., Fu, J., Lv, J., Xue, Q., & Jiang, G. (2014). Polycyclic aromatic hydrocarbons and organochlorine pesticides in rice hull from a typical e-waste recycling area in southeast China: Temporal trend, source, and exposure assessment. Environmental Geochemistry and Health, 36, 65–77.
- Liu, F., Liu, J., Chen, Q., Wang, B., & Cao, Z. (2012). Pollution characteristics, ecological risk and sources of polycyclic aromatic hydrocarbons (PAHs) in surface sediment from Tuhai-Majia River system, China. Procedia Environmental Sciences, 13, 1301–1314.
- Liu, G., Niu, Z., Van Niekerk, D., Xue, J., & Zheng, L. (2008). Polycyclic aromatic hydrocarbons (PAHs) from coal combustion: Emissions, analysis, and toxicology. Reviews of Environmental Contamination and Toxicology., 192, 1–28.
- Liu, H., Meng, F., Tong, Y., & Chi, J. (2014). Effect of plant density on phytoremediation of polycyclic aromatic hydrocarbons contaminated sediments with Vallisneria spiralis. Ecological Engineering, 73, 380–385.
- Liu, J. L., Zhang, J., Liu, F., & Zhang, L. L. (2014). Polycyclic aromatic hydrocarbons in surface sediment of typical estuaries and the spatial distribution in Haihe river basin. Ecotoxicology, 23, 486–494.
- Liu, K., Heltsley, R., Zou, D., Pan, W. P., & Riley, J. T. (2002). Polyaromatic hydrocarbon emissions in fly ashes from an atmospheric fluidised bed combustor using thermal extraction coupled with GC/TOF/MS. Energy Fuels, 16, 330–337.
- Liu, L., Chen, L., Shao, Y., Zhang, L., Floehr, T., Xiao, H., … Wu, L. (2014). Evaluation of the ecotoxicity of sediments from Yangtze River estuary and contribution of priority PAHs to Ah receptor-mediated activities. PLoS ONE, 9(8).
- Liu, Q., Baumgartner, J., Zhang, Y., & Schauer, J. J. (2016). Source apportionment of Beijing air pollution during a severe winter haze event and associated pro-inflammatory responses in lung epithelial cells. Atmospheric Environment, 126, 28–35.
- Liu, R., Dai, Y., & Sun, L. (2015). Effect of rhizosphere enzymes on phytoremediation in PAH-contaminated soil using five plant species. PLoS ONE, 10, e0120369.
- Liu, R., Xiao, N., Wei, S., Zhao, L., & An, J. (2014). Rhizosphere effects of PAH-contaminated soil phytoremediation using a special plant named Fire Phoenix. Science of the Total Environment, 473–474, 350–358.
- Liu, S., Wang, C., Zhang, S., Liang, J., Chen, F., & Zhao, K. (2012). Formation and distribution of polycyclic aromatic hydrocarbons (PAHs) derived from coal seam combustion: A case study of the Ulanqab lignite from Inner Mongolia, northern China. International Journal of Coal Geology, 90–91, 126–134.
- Liu, T., Pan, L., Jin, Q., & Cai, Y. (2015). Differential gene expression analysis of benzo(a)pyrene toxicity in the clam, Ruditapes philippinarum. Ecotoxicology and Environmental Safety, 115, 126–136.
- Liu, Y., Gao, Y., Yu, N., Zhang, C., Wang, S., Ma, L., … Lohmann, R. (2015). Particulate matter, gaseous and particulate polycyclic aromatic hydrocarbons (PAHs) in an urban traffic tunnel of China: Emission from on-road vehicles and gas-particle partitioning. Chemosphere, 134, 52–59.
- Liu, Y., Zhu, L., & Shen, X. (2001). Polycyclic aromatic hydrocarbons (PAHs) in indoor and outdoor air of Hangzhou, China. Environmental Science and Technology, 35, 840–844.
- Liu, Z., He, L., Lu, Y., Su, J., Song, H., Zeng, X., & Yu, Z. (2015). Distribution, source, and ecological risk assessment of polycyclic aromatic hydrocarbons (PAHs) in surface sediments from the Hun River, northeast China. Environmental Monitoring and Assessment, 187, 1–10.
- Löf, M., Sundelin, B., Bandh, C., & Gorokhova, E. (2016). Embryo aberrations in the amphipod Monoporeia affinis as indicators of toxic pollutants in sediments: A field evaluation. Ecological Indicators, 60, 18–30.
- Long, J., Li, L., Jin, Y., Sun, H., Zheng, Y., & Tian, S. (2016). Synergistic solubilization of polycyclic aromatic hydrocarbons by mixed micelles composed of a photoresponsive surfactant and a conventional non-ionic surfactant. Separation and Purification Technology, 160, 11–17.
- Loppi, S., Pozo, K., Estellano, V. H., Corsolini, S., Sardella, G., & Paoli, L. (2015). Accumulation of polycyclic aromatic hydrocarbons by lichen transplants: Comparison with gas-phase passive air samplers. Chemosphere, 134, 39–43.
- Lors, C., Ryngaert, A., Périé, F., Diels, L., & Damidot, D. (2010). Evolution of bacterial community during bioremediation of PAHs in a coal tar contaminated soil. Chemosphere, 81, 1263–1271.
- Louvado, A., Gomes, N. C. M., Simões, M. M. Q., Almeida, A., Cleary, D. F. R., & Cunha, A. (2015). Polycyclic aromatic hydrocarbons in deep sea sediments: Microbe-pollutant interactions in a remote environment. Science of the Total Environment, 526, 312–328.
- Lowe, J. P., & Silverman, B. D. (1984). Predicting carcinogenicity of polycyclic aromatic hydrocarbons. Accounts of Chemical Research, 17, 332–338.
- Lu, X.-Y., Li, B., Zhang, T., & Fang, H. H. P. (2012). Enhanced anoxic bioremediation of PAHs-contaminated sediment. Bioresource Technology, 104, 51–58.
- Luo, L., Wang, P., Lin, L., Luan, T., Ke, L., & Tam, N. F. Y. (2014). Removal and transformation of high molecular weight polycyclic aromatic hydrocarbons in water by live and dead microalgae. Process Biochemistry, 49, 1723–1732.
- Luo, P., Bao, L.-J., Li, S.-M., & Zeng, E. Y. (2015). Size-dependent distribution and inhalation cancer risk of particle-bound polycyclic aromatic hydrocarbons at a typical e-waste recycling and an urban site. Environmental Pollution, 200, 10–15.
- Luo, S., Chen, B., Lin, L., Wang, X., Tam, N. F. Y., & Luan, T. (2014). Pyrene degradation accelerated by constructed consortium of bacterium and microalga: Effects of degradation products on the microalgal growth. Environmental Science and Technology, 48, 13917–13924.
- Luo, W., Gao, J., Bi, X., Xu, L., Guo, J., Zhang, Q., … Kang, S. (2016). Identification of sources of polycyclic aromatic hydrocarbons based on concentrations in soils from two sides of the Himalayas between China and Nepal. Environmental Pollution, 212, 424–432.
- Luo, X., Zheng, Y., Wu, B., Lin, Z., Han, F., Zhang, W., & Wang, X. (2013). Impact of carbonaceous materials in soil on the transport of soil-bound PAHs during rainfall-runoff events. Environmental Pollution, 182, 233–241.
- Lv, J., Xu, J., Guo, C., Zhang, Y., Bai, Y., & Meng, W. (2014). Spatial and temporal distribution of polycyclic aromatic hydrocarbons (PAHs) in surface water from Liaohe River Basin, northeast China. Environmental Science and Pollution Research, 21, 7088–7096.
- Ma, B., Lv, X., He, Y., & Xu, J. (2016). Assessing adsorption of polycyclic aromatic hydrocarbons on Rhizopus oryzae cell wall components with water-methanol cosolvent model. Ecotoxicology and Environmental Safety, 125, 55–60.
- Ma, B., Xu, M., Wang, J., Chen, H., He, Y., Wu, L.,… Xu, J. (2011). Adsorption of polycyclic aromatic hydrocarbons (PAHs) on Rhizopus oryzae cell walls: Application of cosolvent models for validating the cell wall-water partition coefficient. Bioresource Technology, 102, 10542–10547.
- Ma, C., Ye, S., Lin, T., Ding, X., Yuan, H., & Guo, Z. (2014). Source apportionment of polycyclic aromatic hydrocarbons in soils of wetlands in the Liao River Delta, Northeast China. Marine Pollution Bulletin, 80, 160–167.
- Ma, W. L., Liu, L. Y., Qi, H., Zhang, Z. F., Song, W. W., Shen, J. M., … Li, Y. F. (2013). Polycyclic aromatic hydrocarbons in water, sediment and soil of the Songhua River Basin, China. Environmental Monitoring and Assessment, 185, 8399–8409.
- Machado, K. S., Figueira, R. C. L., Figueira, R. C. L., Côcco, L. C., Fernandes, C. V. S., & Ferreira, P. A. L. (2014). Sedimentary record of PAHs in the Barigui River and its relation to the socioeconomic development of Curitiba, Brazil. Science of the Total Environment, 482–483, 42–52.
- Mahler, B. J., Van Metre, P. C., & Foreman, W. T. (2014). Concentrations of polycyclic aromatic hydrocarbons (PAHs) and azaarenes in runoff from coal-tar- and asphalt-sealcoated pavement. Environmental Pollution, 188, 81–87.
- Mangwani, N., Kumari, S., & Das, S. (2015). Involvement of quorum sensing genes in biofilm development and degradation of polycyclic aromatic hydrocarbons by a marine bacterium Pseudomonas aeruginosa N6P6. Applied Microbiology and Biotechnology, 99, 10283–10297.
- Manoli, E., Kouras, A., Karagkiozidou, O., Argyropoulos, G., Voutsa, D., & Samara, C. (2016). Polycyclic aromatic hydrocarbons (PAHs) at traffic and urban background sites of northern Greece: source apportionment of ambient PAH levels and PAH-induced lung cancer risk. Environmental Science and Pollution Research, 23, 3556–3568.
- Mao, J., & Guan, W. (2016). Fungal degradation of polycyclic aromatic hydrocarbons (PAHs) by Scopulariopsis brevicaulis and its application in bioremediation of PAH-contaminated soil. Acta Agriculturae Scandinavica Section B–Soil and Plant Science, 66, 399–405).
- Mao, J., Luo, Y., Teng, Y., & Li, Z. (2012). Bioremediation of polycyclic aromatic hydrocarbon-contaminated soil by a bacterial consortium and associated microbial community changes. International Biodeterioration & Biodegradation, 70, 141–147.
- Marco-Urrea, E., García-Romera, I., & Aranda, E. (2015). Potential of non-ligninolytic fungi in bioremediation of chlorinated and polycyclic aromatic hydrocarbons. New Biotechnology, 32, 620–628.
- Marecik, R., Chrzanowski, Ł., Piotrowska-Cyplik, A., Juzwa, W., & Biegańska-Marecik, R. (2015). Rhizosphere as a tool to introduce a soil-isolated hydrocarbon-degrading bacterial consortium into a wetland environment. International Biodeterioration & Biodegradation, 97, 135–142.
- Marquès, M., Mari, M., Audí-Miró, C., Sierra, J., Soler, A., Nadal, M., & Domingo, J. L. (2016). Climate change impact on the PAH photodegradation in soils: Characterization and metabolites identification. Environment International, 89–90, 155–165.
- Marquez-Bravo, L. G., Briggs, D., Shayler, H., McBride, M., Lopp, D., Stone, E., … Spliethoff, H. M. (2016). Concentrations of polycyclic aromatic hydrocarbons in New York City community garden soils: Potential sources and influential factors. Environmental Toxicology and Chemistry, 35, 357–367.
- Marr, C., Dzepina, K., Jimenez, J. L., Reisen, F., Bethel, H. L., Arey, J., … Molina, M. J. (2006). Sources and transformations of particle-bound polycyclic aromatic hydrocarbons in Mexico City. Atmospheric Chemistry and Physics, 6, 1733–1745.
- Marr, L. C., Kirchstetter, T. W., Harley, R. A., Miguel, A. H., Hering, S. V., & Hammond, S. K. (1999). Characterization of polycyclic aromatic hydrocarbons in motor vehicle fuels and exhaust emissions. Environmental Science and Technology, 33, 3091–3099.
- Marrucci, A., Marras, B., Campisi, S. S., & Schintu, M. (2013). Using SPMDs to monitor the seawater concentrations of PAHs and PCBs in marine protected areas (Western Mediterranean). Marine Pollution Bulletin, 75, 69–75.
- Martins, C. C., Bícego, M. C., Mahiques, M. M., Figueira, R. C. L., Tessler, M. G., & Montone, R. C. (2011). Polycyclic aromatic hydrocarbons (PAHs) in a large South American industrial coastal area (Santos Estuary, Southeastern Brazil): Sources and depositional history. Marine Pollution Bulletin, 63, 452–458.
- Martins, C. C., Bícego, M. C., Rose, N. L., Taniguchi, S., Lourenço, R. A., Figueira, R. C. L., … Montone, R. C. (2010). Historical record of polycyclic aromatic hydrocarbons (PAHs) and spheroidal carbonaceous particles (SCPs) in marine sediment cores from Admiralty Bay, King George Island, Antarctica. Environmental Pollution, 158, 192–200.
- Martins, M., Costa, P. M., Ferreira, A. M., & Costa, M. H. (2013). Comparative DNA damage and oxidative effects of carcinogenic and non-carcinogenic sediment-bound PAHs in the gills of a bivalve. Aquatic Toxicology, 142–143, 85–95.
- Martins, M., Costa, P. M., Raimundo, J., Vale, C., Ferreira, A. M., & Costa, M. H. (2012). Impact of remobilized contaminants in Mytilus edulis during dredging operations in a harbour area: Bioaccumulation and biomarker responses. Ecotoxicology and Environmental Safety, 85, 96–103.
- Masih, A., & Lal, J. K. (2014). Concentrations and carcinogenic profiles of polycyclic aromatic hydrocarbons (PAHs) in groundwater of an urban site at a terai belt of North India. International Journal of Applied Engineering Research, 9(1), 1–7.
- Mastral, A., Callén, M., Murillo, R., Garcia, T., & Viñas, M. (1999). Influence on PAH emissions of the air flow in AFB coal combustion. Fuel, 78, 1553–1557.
- Mastral, A. M., Callén, M., Mayoral, C., & Galbán, J. (1995). Polycyclic aromatic hydrocarbon emissions from fluidized bed combustion of coal. Fuel, 74, 1762–1766.
- Mastral, A. M., Callén, M., & Murillo, R. (1996). Assessment of PAH emissions as a function of coal combustion variables. Fuel, 75, 1533–1536.
- Mastral, A. M., Callén, M., Murillo, R., & García, T. (1998). Assessment of PAH emissions as a function of coal combustion variables in fluidised bed. 2. Air excess percentage. Fuel, 77, 1513–1516.
- Mastral, A. M., Callén, M. S., Murillo, R., García, T., & Lopez, I. M. (2002). Polyaromatic Hydrocarbons in Flue Gases from Waste Tire Combustion. Polycyclic Aromatic Compounds, 22, 561–570.
- Mauro, D. M. (2004). Benzofluorene/methylpyrene ratios as a source identification tool. In Proceedings - Natural Gas Technologies II: Ingenuity and Innovation.
- McVeety, B. D., & Hites, R. A. (1988). Atmospheric deposition of polycyclic aromatic hydrocarbons to water surfaces: a mass balance approach. Atmos. Environ., 22, 511–536.
- Mekonnen, K. N., Chandravanshi, B. S., Redi-Abshiro, M., Ambushe, A. A., McCrindle, R. I., & Moyo, S. (2015). Distribution of polycyclic aromatic hydrocarbons in sediments of Akaki River, Lake Awassa, and Lake Ziway, Ethiopia. Environmental Monitoring and Assessment, 187(7), 1–13.
- Melnyk, A., Dettlaff, A., Kuklińska, K., Namieśnik, J., & Wolska, L. (2015). Concentration and sources of polycyclic aromatic hydrocarbons (PAHs) and polychlorinated biphenyls (PCBs) in surface soil near a municipal solid waste (MSW) landfill. Science of the Total Environment, 530–531, 18–27.
- Menchaca, I., Rodríguez, J. G., Borja, A., Belzunce-Segarra, M. J., Franco, J., Garmendia, J. M., & Larreta, J. (2014). Determination of polychlorinated biphenyl and polycyclic aromatic hydrocarbon marine regional Sediment Quality Guidelines within the European Water Framework Directive. Chemistry and Ecology, 30, 693–700.
- Meng, F., & Chi, J. (2015). Phytoremediation of PAH-contaminated sediments by Potamogeton crispus L. with four plant densities. Transactions of Tianjin University, 21, 440–445.
- Meng, F., Huang, J., Liu, H., & Chi, J. (2015). Remedial effects of Potamogeton crispus L. on PAH-contaminated sediments. Environmental Science and Pollution Research, 22, 7547–7556.
- Mesquita, S. R., Dachs, J., van Drooge, B. L., Castro-Jiménez, J., Navarro-Martín, L., Barata, C., … Piña, B. (2016). Toxicity assessment of atmospheric particulate matter in the Mediterranean and Black Seas open waters. Science of the Total Environment, 545–546, 163–170.
- Messer, D. C., & Taylor, L. T. (1995). Method development for the quantitation of trace polyaromatic hydrocarbons from water via solid-phase extraction with supercritical fluid elution. J. Chromatogr. Sci., 33, 290–296.
- Meyer, W., Seiler, T. B., Christ, A., Redelstein, R., Puettmann, W., Hollert, H., & Achten, C. (2014). Mutagenicity, dioxin-like activity and bioaccumulation of alkylated picene and chrysene derivatives in a German lignite. Science of the Total Environment, 497, 634–641.
- Miao, Q., Bouchard, M., Chen, D., Rosenberg, M. W., & Aronson, K. J. (2015). Commuting behaviors and exposure to air pollution in Montreal, Canada. Science of the Total Environment, 508, 193–198.
- Michel, C., Bourgeault, A., Gourlay-Francé, C., Palais, F., Geffard, A., & Vincent-Hubert, F. (2013). Seasonal and PAH impact on DNA strand-break levels in gills of transplanted zebra mussels. Ecotoxicology and Environmental Safety, 92, 18–26.
- Mineki, S., Suzuki, K., Iwata, K., Nakajima, D., & Goto, S. (2015). Degradation of Polyaromatic Hydrocarbons by Fungi Isolated from Soil in Japan. Polycyclic Aromatic Compounds, 35, 120–128.
- Mirvakili, H. S., & Hadjizadeh Zaker, N. (2014). Evaluation of PAHs in hydrocarbons pollution, biodegradation and weathering extent in surface sediments of the Kharg Island in the Persian Gulf. International Journal of Environmental Research, 8, 387–394.
- Mishra, S., & Singh, S. N. (2014). Biodegradation of benzo(a)pyrene mediated by catabolic enzymes of bacteria. International Journal of Environmental Science and Technology, 11, 1571–1580.
- Mocek, K., & Ciemniak, A. (2016). Influence of physical factors on polycyclic aromatic hydrocarbons (PAHs) content in vegetable oils. Journal of Environmental Science and Health - Part B Pesticides, Food Contaminants, and Agricultural Wastes, 51, 96–102.
- Mohd-Kamil, N. A. F., Hussain, N. H., Mizad, M. B., & Abdul-Talib, S. (2014). Enhancing Performance of Sphingobacterium spiritivorum in Bioremediation Phenanthrene Contaminated Sand. Remediation, 24, 119–128.
- Mollahosseini, A., Rokue, M., Mojtahedi, M. M., Toghroli, M., Kamankesh, M., & Motaharian, A. (2016). Mechanical stir bar sorptive extraction followed by gas chromatography as a new method for determining polycyclic aromatic hydrocarbons in water samples. Microchemical Journal, 126, 431–437.
- Monza, L. B., Loewy, R. M., Savini, M. C., & Pechen De Dangelo, A. M. (2013). Sources and distribution of aliphatic and polyaromatic hydrocarbons in sediments from the Neuquen River, Argentine Patagonia. Journal of Environmental Science and Health - Part A Toxic/Hazardous Substances and Environmental Engineering, 48, 370–379.
- Moorthy, B., Chu, C., & Carlin, D. J. (2015). Polycyclic aromatic hydrocarbons: From metabolism to lung cancer. Toxicological Sciences, 145, 5–15.
- Morais, S., Gomes, F., Ramalhosa, M. J., Delerue-Matos, C., & Oliveira, M. B. P. P. (2013). Occurrence of polycyclic aromatic hydrocarbons in cephalopods. Handbook of Polycyclic Aromatic Hydrocarbons: Chemistry, Occurrence and Health Issues, 197–216.
- Mordukhovich, I., Beyea, J., Herring, A. H., Hatch, M., Stellman, S. D., Teitelbaum, S. L., … Gammon, M. D. (2016). Vehicular traffic–related polycyclic aromatic hydrocarbon exposure and breast cancer incidence: The long island breast cancer study project (LIBCSP). Environmental Health Perspectives, 124, 30–38.
- Moreno, B., Cañizares, R., Macci, C., Doni, S., Masciandaro, G., & Benitez, E. (2015). Molecular tools to understand the bioremediation effect of plants and earthworms on contaminated marine sediments. Journal of Hazardous Materials, 300, 398–405.
- Moret, S., Conte, L., & Dean, D. (1999). Assessment of polycyclic aromatic hydrocarbon content of smoked fish by means of a fast HPLC/HPLC method. Journal of Agricultural and Food Chemistry, 47, 1367–1371.
- Mori, T., Watanabe, M., Taura, H., Kuno, T., Kamei, I., & Kondo, R. (2015). Degradation of chlorinated dioxins and polycyclic aromatic hydrocarbons (PAHs) and remediation of PAH-contaminated soil by the entomopathogenic fungus, Cordyceps militaris. Journal of Environmental Chemical Engineering, 3, 2317–2322.
- Morris, B. E. L., Gissibl, A., Kümmel, S., Richnow, H. H., & Boll, M. (2014). A PCR-based assay for the detection of anaerobic naphthalene degradation. FEMS Microbiology Letters, 354, 55–59.
- Moschino, V., Delaney, E., & Da Ros, L. (2012). Assessing the significance of Ruditapes philippinarum as a sentinel for sediment pollution: Bioaccumulation and biomarker responses. Environmental Pollution, 171, 52–60.
- Moschino, V., Delaney, E., Meneghetti, F., & Ros, L. D. (2011). Biomonitoring approach with mussel Mytilus galloprovincialis (Lmk) and clam Ruditapes philippinarum (Adams and Reeve, 1850) in the Lagoon of Venice. Environmental Monitoring and Assessment, 177, 649–663.
- Moscoso, F., Teijiz, I., Deive, F. J., & Sanromán, M. A. (2012). Efficient PAHs biodegradation by a bacterial consortium at flask and bioreactor scale. Bioresource Technology, 119, 270–276.
- Motorykin, O., Santiago-Delgado, L., Rohlman, D., Schrlau, J. E., Harper, B., Harris, S., … Massey Simonich, S. L. (2015). Metabolism and excretion rates of parent and hydroxy-PAHs in urine collected after consumption of traditionally smoked salmon for Native American volunteers. Science of the Total Environment, 514, 170–177.
- Mottier, P., Parisod, V., & Turesky, R. J. (2000). Quantitative determination of polycyclic aromatic hydrocarbons in barbecued meat sausages by gas chromatography coupled to mass spectrometry. Journal of Agricultural and Food Chemistry, 48, 1160–1166.
- Muangchinda, C., Chavanich, S., Viyakarn, V., Watanabe, K., Imura, S., Vangnai, A. S., & Pinyakong, O. (2015). Abundance and diversity of functional genes involved in the degradation of aromatic hydrocarbons in Antarctic soils and sediments around Syowa Station. Environmental Science and Pollution Research, 22, 4725–4735.
- Muangchinda, C., Pansri, R., Wongwongsee, W., & Pinyakong, O. (2013). Assessment of polycyclic aromatic hydrocarbon biodegradation potential in mangrove sediment from Don Hoi Lot, Samut Songkram Province, Thailand. Journal of Applied Microbiology, 114, 1311–1324.
- Mugica-Alvarez, V., Santiago-de la Rosa, N., Figueroa-Lara, J., Flores-Rodríguez, J., Torres-Rodríguez, M., & Magaña-Reyes, M. (2015). Emissions of PAHs derived from sugarcane burning and processing in Chiapas and Morelos México. Science of the Total Environment, 527–528, 474–482.
- Mulder, M. D., Heil, A., Kukučka, P., Kuta, J., Přibylová, P., Prokeš, R., & Lammel, G. (2015). Long-range atmospheric transport of PAHs, PCBs and PBDEs to the central and eastern Mediterranean and changes of PCB and PBDE congener patterns in summer 2010. Atmospheric Environment, 111, 51–59.
- Muratova, A., Golubev, S., Wittenmayer, L., Dmitrieva, T., Bondarenkova, A., Hirche, F., … Turkovskaya, O. (2009). Effect of the polycyclic aromatic hydrocarbon phenanthrene on root exudation of Sorghum bicolor (L.) Moench. Environmental and Experimental Botany, 66, 514–521.
- Muratova, A., Pozdnyakova, N., Makarov, O., Baboshin, M., Baskunov, B., Myasoedova, N., … Turkovskaya, O. (2014). Degradation of phenanthrene by the rhizobacterium Ensifer meliloti. Biodegradation, 25, 787–795.
- Mzoughi, N., & Chouba, L. (2012). Heavy metals and PAH assessment based on mussel caging in the North coast of Tunisia (Mediterranean sea). International Journal of Environmental Research, 6(1), 109–118.
- Na, G., Liu, C., Wang, Z., Ge, L., Ma, X., & Yao, Z. (2011). Distribution and characteristic of PAHs in snow of Fildes Peninsula. Journal of Environmental Sciences, 23, 1445–1451.
- Nadal, M., Schuhmacher, M., & Domingo, J. L. (2004). Levels of PAHs in soil and vegetation samples from Tarragona County, Spain. Environmental Pollution, 132(1), 1–11.
- Nagpure, A. S., Gurjar, B. R., Kumar, V., & Kumar, P. (2016). Estimation of exhaust and non-exhaust gaseous, particulate matter and air toxics emissions from on-road vehicles in Delhi. Atmospheric Environment, 127, 118–124.
- Nahrgang, J., Brooks, S. J., Evenset, A., Camus, L., Jonsson, M., Smith, T. J., … Renaud, P. E. (2013). Seasonal variation in biomarkers in blue mussel (Mytilus edulis), Icelandic scallop (Chlamys islandica) and Atlantic cod (Gadus morhua)-Implications for environmental monitoring in the Barents Sea. Aquatic Toxicology, 127, 21–35.
- Nam, T. H., Jeon, H. J., Mo, H. H., Cho, K., Ok, Y. S., & Lee, S. E. (2015). Determination of biomarkers for polycyclic aromatic hydrocarbons (PAHs) toxicity to earthworm (Eisenia fetida). Environmental Geochemistry and Health, 37, 943–951.
- Nascimbene, J., Tretiach, M., Corana, F., Lo Schiavo, F., Kodnik, D., Dainese, M., & Mannucci, B. (2014). Patterns of traffic polycyclic aromatic hydrocarbon pollution in mountain areas can be revealed by lichen biomonitoring: A case study in the Dolomites (Eastern Italian Alps). Science of the Total Environment, 475, 90–96.
- Nekhavhambe, T. J., van Ree, T., & Fatoki, O. S. (2014). Determination and distribution of polycyclic aromatic hydrocarbons in rivers, surface runoff, and sediments in and around Thohoyandou, Limpopo Province, South Africa. Water SA, 40, 415–424.
- Net, S., Dumoulin, D., El-Osmani, R., Rabodonirina, S., & Ouddane, B. (2014). Case study of PAHs, Me-PAHs, PCBs, phthalates and pesticides contamination in the Somme River water, France. International Journal of Environmental Research, 8, 1159–1170.
- Nguyen, T., Hlangothi, D., Martinez Iii, R. A., Jacob, D., Anthony, K., Nance, H., & Saleh, M. A. (2013). Charcoal burning as a source of polyaromatic hydrocarbons in waterpipe smoking. Journal of Environmental Science and Health - Part B Pesticides, Food Contaminants, and Agricultural Wastes, 48, 1097–1102.
- Nguyen, T. C., Loganathan, P., Nguyen, T. V., Vigneswaran, S., Kandasamy, J., Slee, D., Stevenson, G., & Naidu, R. (2014). Polycyclic aromatic hydrocarbons in road-deposited sediments, water sediments, and soils in Sydney, Australia: Comparisons of concentration distribution, sources and potential toxicity. Ecotoxicology and Environmental Safety, 104, 339–348.
- Nieva-Cano, M. J., Rubio-Barroso, S., & Santos-Delgado, M. J. (2001). Determination of PAH in food samples by HPLC with fluorimetric detection following sonication extraction without sample clean-up. Analyst, 126, 1326–1331.
- Nilsen, E. B., Rosenbauer, R. J., Fuller, C. C., & Jaffe, B. J. (2015). Sedimentary organic biomarkers suggest detrimental effects of PAHs on estuarine microbial biomass during the 20th century in San Francisco Bay, CA, USA. Chemosphere, 119, 961–970.
- Ning, X. A., Lin, M. Q., Shen, L. Z., Zhang, J. H., Wang, J. Y., Wang, Y. J., … Liu, J. Y. (2014). Levels, composition profiles and risk assessment of polycyclic aromatic hydrocarbons (PAHs) in sludge from ten textile dyeing plants. Environmental Research, 132, 112–118.
- Nisha, A. R., Dinesh Kumar, V., Arivudainambi, S., Umer, M., & Khan, M. S. (2015). Polycyclic aromatic hydrocarbons in processed meats: A toxicological perspective. Research Journal of Chemistry and Environment, 19, 72–76.
- Nordin, E. Z., Uski, O., Nyström, R., Jalava, P., Eriksson, A. C., Genberg, J., … Hirvonen, M. R. (2015). Influence of ozone initiated processing on the toxicity of aerosol particles from small scale wood combustion. Atmospheric Environment, 102, 282–289.
- Nøst, T. H., Halse, A. K., Randall, S., Borgen, A. R., Schlabach, M., Paul, A., … Breivik, K. (2015). High Concentrations of Organic Contaminants in Air from Ship Breaking Activities in Chittagong, Bangladesh. Environmental Science and Technology, 49, 11372–11380.
- Nwaichi, E. O., & Ntorgbo, S. A. (2016). Assessment of PAHs levels in some fish and seafood from different coastal waters in the Niger Delta. Toxicology Reports, 3, 167–172.
- Nwaichi, E. O., Wegwu, M. O., & Nwosu, U. L. (2014). Distribution of selected carcinogenic hydrocarbon and heavy metals in an oil-polluted agriculture zone. Environmental Monitoring and Assessment, 186, 8697–8706.
- Obinaju, B. E., Graf, C., Halsall, C., & Martin, F. L. (2015). Linking biochemical perturbations in tissues of the African catfish to the presence of polycyclic aromatic hydrocarbons in Ovia River, Niger Delta region. Environmental Pollution, 201, 42–49.
- Obrist, D., Zielinska, B., & Perlinger, J. A. (2015). Accumulation of polycyclic aromatic hydrocarbons (PAHs) and oxygenated PAHs (OPAHs) in organic and mineral soil horizons from four U.S. remote forests. Chemosphere, 134, 98–105.
- Obuekwe, I. S., & Semple, K. T. (2013). Impact of Al and Fe on the development of phenanthrene catabolism in soil. Journal of Soils and Sediments, 13, 1589–1599.
- Odabasi, M., Vardar, N., Sofuoglu, A., Tasdemir, Y., & Holsen, T. M. (1999). Polycyclic aromatic hydrocarbons (PAHs) in Chicago air. Science of The Total Environment, 227, 57–67.
- Ohura, T., Sakakibara, H., Watanabe, I., Shim, W. J., Manage, P. M., & Guruge, K. S. (2015). Spatial and vertical distributions of sedimentary halogenated polycyclic aromatic hydrocarbons in moderately polluted areas of Asia. Environmental Pollution, 196, 331–340.
- Olenycz, M., Sokołowski, A., Niewińska, A., Wołowicz, M., Namieśnik, J., Hummel, H., & Jansen, J. (2012). Comparison of PCBs and PAHs levels in European coastal waters using mussels from the Mytilus edulis complex as biomonitors. Oceanologia, 57, 196–211.
- Oleszczuk, P. (2009). Application of three methods used for the evaluation of polycyclic aromatic hydrocarbons (PAHs) bioaccessibility for sewage sludge composting. Bioresource Technology, 100, 413–420.
- Oleszczuk, P., Zielińska, A., & Cornelissen, G. (2014). Stabilization of sewage sludge by different biochars towards reducing freely dissolved polycyclic aromatic hydrocarbons (PAHs) content. Bioresource Technology, 156, 139–145.
- Oliva, M., Perales, J. A., Gravato, C., Guilhermino, L., & Galindo-Riaño, M. D. (2012). Biomarkers responses in muscle of Senegal sole (Solea senegalensis) from a heavy metals and PAHs polluted estuary. Marine Pollution Bulletin, 64, 2097–2108.
- Olsson, A. C., Fevotte, J., Fletcher, T., Cassidy, A., Mannetje, A., & Brennan, P. (2010). Occupational exposure to polycyclic aromatic hydrocarbons and lung cancer risk: amulticenter studyin Europe. Occup Environ Med, 67, 98–103.
- Orecchio, S. (2011). Polycyclic aromatic hydrocarbons (PAHs) in indoor emission from decorative candles. Atmospheric Environment, 45, 1888–1895.
- Orecchio, S., Amorello, D., Barreca, S., & Valenti, A. (2016). Wood pellets for home heating can be considered environmentally friendly fuels? Polycyclic aromatic hydrocarbons (PAHs) in their ashes. Microchemical Journal, 124, 267–271.
- Orisakwe, O. E., Igweze, Z. N., Okolo, K. O., & Udowelle, N. A. (2015). Human health hazards of poly aromatic hydrocarbons in Nigerian smokeless tobacco. Toxicology Reports, 2, 1019–1023.
- Ortega-Calvo, J. J., Tejeda-Agredano, M. C., Jimenez-Sanchez, C., Congiu, E., Sungthong, R., Niqui-Arroyo, J. L., & Cantos, M. (2013). Is it possible to increase bioavailability but not environmental risk of PAHs in bioremediation? Journal of Hazardous Materials, 261, 733–745.
- Ortuño, N., Conesa, J. A., Moltó, J., & Font, R. (2014). Pollutant emissions during pyrolysis and combustion of waste printed circuit boards, before and after metal removal. Science of the Total Environment, 499, 27–35.
- Otte, J. C., Keiter, S., Faßbender, C., Higley, E. B., Rocha, P. S., Brinkmann, M., … Hollert, H. (2013). Contribution of Priority PAHs and POPs to Ah Receptor-Mediated Activities in Sediment Samples from the River Elbe Estuary, Germany. PLoS ONE, 8, e75596.
- Ouvrard, S., Leglize, P., & Morel, J. L. (2014). PAH Phytoremediation: Rhizodegradation or Rhizoattenuation? International Journal of Phytoremediation, 16, 46–61.
- Oyo-Ita, I. O., Oyo-Ita, O. E., Dosunmu, M. I., Domínguez, C., Bayona, J. M., & Albaigés, J. (2016). Distribution and Sources of Petroleum Hydrocarbons in Recent Sediments of the Imo River, SE Nigeria. Archives of Environmental Contamination and Toxicology, 70, 372–382.
- Oyo-Ita, O. E., Offem, J. O., Ekpo, B. O., & Adie, P. A. (2013). Anthropogenic PAHs in mangrove sediments of the Calabar River, SE Niger Delta, Nigeria. Applied Geochemistry, 28, 212–219.
- Ozaki, N., Takamura, Y., Kojima, K., & Kindaichi, T. (2015). Loading and removal of PAHs in a wastewater treatment plant in a separated sewer system. Water Research, 80, 337–345.
- Paalme, L., Irha, N., Urbas, E., Tsyban, A., & Kirso, U. (1990). Model studies of photochemical oxidation of carcinogenic polyaromatic hydrocarbons. Marine Chemistry, 30, 105–111.
- Paoli, L., Munzi, S., Guttová, A., Senko, D., Sardella, G., & Loppi, S. (2015). Lichens as suitable indicators of the biological effects of atmospheric pollutants around a municipal solid waste incinerator (S Italy). Ecological Indicators, 52, 362–370.
- Papa, S., Bartoli, G., Nacca, F., D’Abrosca, B., Cembrola, E., Pellegrino, A., … Fioretto, A. (2012). Trace metals, peroxidase activity, PAHs contents and ecophysiological changes in Quercus ilex leaves in the urban area of Caserta (Italy). Journal of Environmental Management, 113, 501–509.
- Park, J. M., Lee, S. B., Kim, J. P., Kim, M. J., Kwon, O. S., & Jung, D. I. (2009). Behavior of PAHs from sewage sludge incinerators in Korea. Waste Management, 29, 690–695.
- Parnis, J. M., Eng, A., Mackay, D., & Harner, T. (2016). Characterizing PUF disk passive air samplers for alkyl-substituted PAHs: Measured and modelled PUF-AIR partition coefficients with COSMO-RS. Chemosphere, 145, 360–364.
- Partovinia, A., & Naeimpoor, F. (2013). Phenanthrene biodegradation by immobilized microbial consortium in polyvinyl alcohol cryogel beads. International Biodeterioration and Biodegradation, 85, 337–344.
- Partovinia, A., & Naeimpoor, F. (2014). Comparison of phenanthrene biodegradation by free and immobilized cell systems: Formation of hydroxylated compounds. Environmental Science and Pollution Research, 21, 5889–5898.
- Patelarou, E., & Kelly, F. J. (2014). Indoor exposure and adverse birth outcomes related to fetal growth, miscarriage and prematurity - A systematic review. International Journal of Environmental Research and Public Health, 11, 5904–5933.
- Patil, R. R., Chetlapally, S. K., & Bagavandas, M. (2014). Global review of studies on traffic police with special focus on environmental health effects. International Journal of Occupational Medicine and Environmental Health, 27, 523–535.
- Patrolecco, L., Ademollo, N., Capri, S., Pagnotta, R., & Polesello, S. (2010). Occurrence of priority hazardous PAHs in water, suspended particulate matter, sediment and common eels (Anguilla anguilla) in the urban stretch of the River Tiber (Italy). Chemosphere, 81, 1386–1392.
- Paulik, L. B., Donald, C. E., Smith, B. W., Tidwell, L. G., Hobbie, K. A., Kincl, L., … Anderson, K. A. (2015). Impact of natural gas extraction on PAH levels in ambient air. Environmental Science and Technology, 49, 5203–5210.
- Pazos, M., Rosales, E., Alcántara, T., Gómez, J., & Sanromán, M. A. (2010). Decontamination of soils containing PAHs by electroremediation: A review. Journal of Hazardous Materials, 177, 1–11.
- Pena-Pereira, F., Costas-Mora, I., Lavilla, I., & Bendicho, C. (2012). Rapid screening of polycyclic aromatic hydrocarbons (PAHs) in waters by directly suspended droplet microextraction-microvolume fluorospectrometry. Talanta, 89, 217–222.
- Pena, E. A., Ridley, L. M., Murphy, W. R., Sowa, J. R., & Bentivegna, C. S. (2015). Detection of polycyclic aromatic hydrocarbons (PAHs) in raw menhaden fish oil using fluorescence spectroscopy: Method development. Environmental Toxicology and Chemistry, 34, 1946–1958.
- Penezić, A., Gašparović, B., Stipaničev, D., & Nelson, A. (2014). In-situ electrochemical method for detecting freely dissolved polycyclic aromatic hydrocarbons in water. Environmental Chemistry, 11, 173–180.
- Peng, R. H., Xiong, A. S., Xue, Y., Fu, X. Y., Gao, F., Zhao, W., … Yao, Q. H. (2008). Microbial biodegradation of polyaromatic hydrocarbons. FEMS Microbiology Reviews, 32, 927–955.
- Pérès, G., Vandenbulcke, F., Guernion, M., Hedde, M., Beguiristain, T., Douay, S., … Cluzeau, D. (2011). Earthworm indicators as tools for soil monitoring, characterization and risk assessment. An example from the national Bioindicator programme (France). Pedobiologia, 54 (Suppl.), S77–S87.
- Pergal, M. M., Relić, D., Tešić, Ž. L., & Popović, A. R. (2014). Leaching of polycyclic aromatic hydrocarbons from power plant lignite ash-influence of parameters important for environmental pollution. Environmental Science and Pollution Research, 21, 3435–3442.
- Perra, G., Pozo, K., Guerranti, C., Lazzeri, D., Volpi, V., Corsolini, S., & Focardi, S. (2011). Levels and spatial distribution of polycyclic aromatic hydrocarbons (PAHs) in superficial sediment from 15 Italian marine protected areas (MPA). Marine Pollution Bulletin, 62, 874–877.
- Pesch, B., Spickenheuer, A., Kendzia, B., Schindler, B. K., Welge, P., Marczynski, B., … Brüning, T. (2011). Urinary metabolites of polycyclic aromatic hydrocarbons in workers exposed to vapours and aerosols of bitumen. Archives of Toxicology, 85(SUPPL. 1), S29–S39.
- Peters, R. E., Wickstrom, M., & Siciliano, S. D. (2016). Do biomarkers of exposure and effect correlate with internal exposure to PAHs in swine? Biomarkers, 21, 283–291.
- Pettersson, M., Adolfsson-Erici, M., Parkkonen, J., Förlin, L., & Asplund, L. (2006). Fish bile used to detect estrogenic substances in treated sewage water. Science of The Total Environment, 366, 174–186.
- Pham, C. T., Kameda, T., Toriba, A., & Hayakawa, K. (2013). Polycyclic aromatic hydrocarbons and nitropolycyclic aromatic hydrocarbons in particulates emitted by motorcycles. Environmental Pollution, 183, 175–183.
- Pham, C. T., Tang, N., Toriba, A., & Hayakawa, K. (2015). Polycyclic Aromatic Hydrocarbons and Nitropolycyclic Aromatic Hydrocarbons in Atmospheric Particles and Soil at a Traffic Site in Hanoi, Vietnam. Polycyclic Aromatic Compounds, 35, 355–371.
- Pickard, M. A., Roman, R., Tinoco, R., & Vasquez-Duhalt, R. (1999). Polycyclic aromatic hydrocarbon metabolism by white rot fungi and oxidation by Coriolopsis gallica UAMH 8260 laccase. Appl. Environ. Microbiol., 65, 3805–3809.
- Pincemaille, J., Schummer, C., Heinen, E., & Moris, G. (2014). Determination of polycyclic aromatic hydrocarbons in smoked and non-smoked black teas and tea infusions. Food chemistry, 145, 807–813.
- Pissinatti, R., Nunes, C. M., de Souza, A. G., Junqueira, R. G., & de Souza, S. V. C. (2015). Simultaneous analysis of 10 polycyclic aromatic hydrocarbons in roasted coffee by isotope dilution gas chromatography-mass spectrometry: Optimization, in-house method validation and application to an exploratory study. Food Control, 51, 140–148.
- Plaza-Bolaños, P., Frenich, A. G., & Vidal, J. L. M. (2010). Polycyclic aromatic hydrocarbons in food and beverages. Analytical methods and trends. Journal of Chromatography A, 1217, 6303–6326.
- Polanska, K., Hanke, W., Dettbarn, G., Sobala, W., Gromadzinska, J., Magnus, P., & Seidel, A. (2014). The determination of polycyclic aromatic hydrocarbons in the urine of non-smoking Polish pregnant women. Science of the Total Environment, 487, 102–109.
- Pongpiachan, S. (2015). Impacts of agricultural waste burning on the enhancement of PM 2.5-bound polycyclic aromatic hydrocarbons in northern Thailand. WIT Transactions on Ecology and the Environment, 198, 3–14.
- Pongpiachan, S., Tipmanee, D., Deelaman, W., Muprasit, J., Feldens, P., & Schwarzer, K. (2013). Risk assessment of the presence of polycyclic aromatic hydrocarbons (PAHs) in coastal areas of Thailand affected by the 2004 tsunami. Marine Pollution Bulletin, 76, 370–378.
- Portet-Koltalo, F., Ammami, M. T., Benamar, A., Wang, H., Le Derf, F., & Duclairoir-Poc, C. (2013). Investigation of the release of PAHs from artificially contaminated sediments using cyclolipopeptidic biosurfactants. Journal of Hazardous Materials, 261, 593–601.
- Potthast, K., & Eigner, G. (1975). A new method for the rapid isolation of polycyclic aromatic hydrocarbons from smoked meat products. Journal of Chromatography A, 103, 173–176.
- Pozo, K., Estellano, V. H., Harner, T., Diaz-Robles, L., Cereceda-Balic, F., Etcharren, P., … Vergara-Fernández, A. (2015). Assessing Polycyclic Aromatic Hydrocarbons (PAHs) using passive air sampling in the atmosphere of one of the most wood-smoke-polluted cities in Chile: The case study of Temuco. Chemosphere, 134, 475–481.
- Puy-Alquiza, M. J., Reyes, V., Wrobel, K., Wrobel, K., Torres Elguera, J. C., & Miranda-Aviles, R. (2016). Polycyclic aromatic hydrocarbons in urban tunnels of Guanajuato city (Mexico) measured in deposited dust particles and in transplanted lichen Xanthoparmelia mexicana (Gyeln.) Hale. Environmental Science and Pollution Research, 23, 11947–11956.
- Qi, H., Li, W. L., Zhu, N. Z., Ma, W. L., Liu, L. Y., Zhang, F., & Li, Y. F. (2014). Concentrations and sources of polycyclic aromatic hydrocarbons in indoor dust in China. Science of the Total Environment, 491–492, 100–107.
- Qi, W., Liu, H., Pernet-Coudrier, B., & Qu, J. (2013). Polycyclic aromatic hydrocarbons in wastewater, WWTPs effluents and in the recipient waters of Beijing, China. Environmental Science and Pollution Research, 20, 4254–4260.
- Qin, N., He, W., Kong, X.-Z., Liu, W.-X., He, Q.-S., Yang, B., … Xu, F.-L. (2013). Ecological risk assessment of polycyclic aromatic hydrocarbons (PAHs) in the water from a large Chinese lake based on multiple indicators. Ecological Indicators, 24, 599–608.
- Qin, N., He, W., Kong, X.-Z., Liu, W.-X., He, Q.-S., Yang, B., … Zhao, X.-L. (2014). Distribution, partitioning and sources of polycyclic aromatic hydrocarbons in the water–SPM–sediment system of Lake Chaohu, China. Science of the Total Environment, 496, 414–423.
- Qin, Z., Liu, S., Liang, S. X., Kang, Q., Wang, J., & Zhao, C. (2016). Advanced treatment of pharmaceutical wastewater with combined micro-electrolysis, Fenton oxidation, and coagulation sedimentation method. Desalination and Water Treatment, 57, 1–10.
- Qiu, Y.-W., Zhang, G., Liu, G.-Q., Guo, L.-L., Li, X.-D., & Wai, O. (2009). Polycyclic aromatic hydrocarbons (PAHs) in the water column and sediment core of Deep Bay, South China. Estuarine, Coastal and Shelf Science, 83, 60–66.
- Rahmanpour, S., Farzaneh Ghorghani, N., & Lotfi Ashtiyani, S. M. (2014). Polycyclic aromatic hydrocarbon (PAH) in four fish species from different trophic levels in the Persian Gulf. Environmental Monitoring and Assessment, 186, 7047–7053.
- Ramdine, G., Fichet, D., Louis, M., & Lemoine, S. (2012). Polycyclic aromatic hydrocarbons (PAHs) in surface sediment and oysters (Crassostrea rhizophorae) from mangrove of Guadeloupe: Levels, bioavailability, and effects. Ecotoxicology and Environmental Safety, 79, 80–89.
- Ranzi, A., Fustinoni, S., Erspamer, L., Campo, L., Gatti, M. G., Bechtold, P., … Lauriola, P. (2013). Biomonitoring of the general population living near a modern solid waste incinerator: A pilot study in Modena, Italy. Environment International, 61, 88–97.
- Ratola, N., Amigo, J. M., Lacorte, S., Barceló, D., Psillakis, E., & Alves, A. (2012). Comparison of PAH Levels and Sources in Pine Needles from Portugal, Spain, and Greece. Analytical Letters, 45, 508–525.
- Ratola, N., Amigo, J. M., Lacorte, S., Barceló, D., Psillakis, E., & Alves, A. (2013). Biomonitoring of polycyclic aromatic hydrocarbons by pine needles-levels and trends in Southern Europe. Handbook of Polycyclic Aromatic Hydrocarbons: Chemistry, Occurrence and Health Issues, 83–113.
- Ratola, N., Amigo, J. M., Oliveira, M. S. N., Araújo, R., Silva, J. A., & Alves, A. (2011). Differences between Pinus pinea and Pinus pinaster as bioindicators of polycyclic aromatic hydrocarbons. Environmental and Experimental Botany, 72, 339–347.
- Ravindra, K., Sokhi, R., & Van Grieken, R. (2008). Atmospheric polycyclic aromatic hydrocarbons: sourceattribution, emission factors and regulation. Atmos Environ, 42, 2895–921.
- Ré, N., Kataoka, V. M. F., Cardoso, C. A. L., Alcantara, G. B., & De Souza, J. B. G. (2015). Polycyclic Aromatic Hydrocarbon Concentrations in Gas and Particle Phases and Source Determination in Atmospheric Samples from a Semiurban Area of Dourados, Brazil. Archives of Environmental Contamination and Toxicology, 69, 69–80.
- Reátegui-Zirena, E. G., Stewart, P. M., Whatley, A., Chu-Koo, F., Sotero-Solis, V. E., Merino-Zegarra, C., & Vela-Paima, E. (2014). Polycyclic aromatic hydrocarbon concentrations, mutagenicity, and Microtox® acute toxicity testing of Peruvian crude oil and oil-contaminated water and sediment. Environmental Monitoring and Assessment, 186, 2171–2184.
- Ren, C., Wu, Y., Zhang, S., Wu, L. L., Liang, X. G., Chen, T. H., … Wang, J. Z. (2014). PAHs in sediment cores at main river estuaries of Chaohu Lake: implication for the change of local anthropogenic activities. Environmental Science and Pollution Research, 22, 1687–1696.
- Ren, C., Wu, Y., Zhang, S., Wu, L. L., Liang, X. G., Chen, T. H., … Wang, J. Z. (2015). PAHs in sediment cores at main river estuaries of Chaohu Lake: implication for the change of local anthropogenic activities. Environmental science and pollution research international, 22, 1687–1696.
- Rengarajan, T., Rajendran, P., Nandakumar, N., Lokeshkumar, B., Rajendran, P., & Nishigaki, I. (2015). Exposure to polycyclic aromatic hydrocarbons with special focus on cancer. Asian Pacific Journal of Tropical Biomedicine, 5, 182–189.
- Reyes-César, A., Absalón, Á. E., Fernández, F. J., González, J. M., & Cortés-Espinosa, D. V. (2014). Biodegradation of a mixture of PAHs by non-ligninolytic fungal strains isolated from crude oil-contaminated soil. World Journal of Microbiology and Biotechnology, 30, 999–1009.
- Rinaldi, M. C. S., Domingos, M., Dias, A. P. L., Esposito, J. B. N., & Pagliuso, J. D. (2012). Leaves of Lolium multiflorum ‘Lema’ and tropical tree species as biomonitors of polycyclic aromatic hydrocarbons. Ecotoxicology and Environmental Safety, 79, 139–147.
- Roda, A., Simoni, P., Ferri, E. N., Girotti, S., Ius, A., Rauch, P., … Fukal, L. (1999). Determination of PAHs in various smoked meat products and different samples by enzyme immunoassay. Journal of the Science of Food and Agriculture, 79, 58–62.
- Rodgers-Vieira, E. A., Zhang, Z., Adrion, A. C., Gold, A., & Aitken, M. D. (2015). Identification of anthraquinone-degrading bacteria in soil contaminated with polycyclic aromatic hydrocarbons. Applied and Environmental Microbiology, 81, 3775–3781.
- Rodrigues, A., Nogueira, R., Melo, L. F., & Brito, A. G. (2013). Effect of low concentrations of synthetic surfactants on polycyclic aromatic hydrocarbons (PAH) biodegradation. International Biodeterioration and Biodegradation, 83, 48–55.
- Rodríguez-Morgado, B., Gómez, I., Parrado, J., García, C., Hernández, T., & Tejada, M. (2015). Accelerated degradation of PAHs using edaphic biostimulants obtained from sewage sludge and chicken feathers. Journal of Hazardous Materials, 300, 235–242.
- Rodriguez, J. H., Wannaz, E. D., Salazar, M. J., Pignata, M. L., Fangmeier, A., & Franzaring, J. (2012). Accumulation of polycyclic aromatic hydrocarbons and heavy metals in the tree foliage of Eucalyptus rostrata, Pinus radiata and Populus hybridus in the vicinity of a large aluminium smelter in Argentina. Atmospheric Environment, 55, 35–42.
- Rojo-Nieto, E., Oliva, M., Sales, D., & Perales, J. A. (2014). Feral finfish, and their relationships with sediments and seawater, as a tool for risk assessment of PAHs in chronically polluted environments. Science of The Total Environment, 470–471, 1030–1039.
- Romagnoli, P., Balducci, C., Perilli, M., Gherardi, M., Gordiani, A., Gariazzo, C., … Cecinato, A. (2014). Indoor PAHs at schools, homes and offices in Rome, Italy. Atmospheric Environment, 92, 51–59.
- Rosales, E., Pazos, M., & Ángeles Sanromán, M. (2013). Feasibility of Solid-State Fermentation Using Spent Fungi-Substrate in the Biodegradation of PAHs. Clean - Soil, Air, Water, 41, 610–615.
- Rose, M., Holland, J., Dowding, A., Petch, S., White, S., Fernandes, A., & Mortimer, D. (2015). Investigation into the formation of PAHs in foods prepared in the home to determine the effects of frying, grilling, barbecuing, toasting and roasting. Food and Chemical Toxicology, 78, 1–9.
- Roseiro, L. C., Gomes, A., Patarata, L., & Santos, C. (2012). Comparative survey of PAHs incidence in Portuguese traditional meat and blood sausages. Food and Chemical Toxicology, 50, 1891–1896.
- Ruiz-Fernández, A. C., Betancourt Portela, J. M., Sericano, J. L., Sanchez-Cabeza, J.-A., Espinosa, L. F., Cardoso-Mohedano, J. G., … Garay Tinoco, J. A. (2016). Coexisting sea-based and land-based sources of contamination by PAHs in the continental shelf sediments of Coatzacoalcos River discharge area (Gulf of Mexico). Chemosphere, 144, 591–598.
- Ruokojärvi, P., Aatamila, M., & Ruuskanen, J. (2000). Toxic chlorinated and polyaromatic hydrocarbons in simulated house fires. Chemosphere, 41, 825–828.
- Ruokojärvi, P., Ruuskanen, J., Ettala, M., Rahkonen, P., & Tarhanen, J. (1995). Formation of polyaromatic hydrocarbons and polychlorinated organic compounds in municipal waste landfill fires. Chemosphere, 31, 3899–3908.
- Sacchi, A., Mouneyrac, C., Bolognesi, C., Sciutto, A., Roggieri, P., Fusi, M., … Capri, E. (2013). Biomonitoring study of an estuarine coastal ecosystem, the Sacca di Goro lagoon, using Ruditapes philippinarum (Mollusca: Bivalvia). Environmental Pollution, 177, 82–89.
- Sadeghi, R., Kobarfard, F., Yazdanpanah, H., Eslamizad, S., & Bayat, M. (2016). Validation of an analytical method for determination of 13 priority polycyclic aromatic hydrocarbons in mineral water using dispersive liquid-liquid microextraction and GC-MS. Iranian Journal of Pharmaceutical Research, 15, 157–168.
- Saija, E., Mangano, V., Casale, K. E., La Torre, G. L., Dugo, G., & Salvo, A. (2016). Determination and quantification of PCBs, POCs and PAHs in Thunnus thynnus from the Straits of Messina (Italy). Data in Brief, 7, 129–134.
- Sakulthaew, C., Comfort, S., Chokejaroenrat, C., Harris, C., & Li, X. (2014). A combined chemical and biological approach to transforming and mineralizing PAHs in runoff water. Chemosphere, 117, 1–9.
- Sakulthaew, C., Comfort, S. D., Chokejaroenrat, C., Li, X., & Harris, C. E. (2015). Removing PAHs from urban runoff water by combining ozonation and carbon nano-onions. Chemosphere, 141, 265–273.
- Salam, L. B., Obayori, O. S., & Raji, S. A. (2014). Biodegradation of used engine oil by a methylotrophic bacterium, methylobacterium mesophilicum isolated from tropical hydrocarbon-contaminated soil. Petroleum Science and Technology, 33, 186–195.
- Salvo, L. M., Severino, D., Silva de Assis, H. C., & da Silva, J. R. M. C. (2016). Photochemical degradation increases polycyclic aromatic hydrocarbon (PAH) toxicity to the grouper Epinephelus marginatus as assessed by multiple biomarkers. Chemosphere, 144, 540–547.
- Santana, J. L., Massone, C. G., Valdés, M., Vazquez, R., Lima, L. A., & Olivares-Rieumont, S. (2015). Occurrence and source appraisal of polycyclic aromatic hydrocarbons (PAHs) in surface waters of the Almendares River, Cuba. Archives of Environmental Contamination and Toxicology, 69, 143–152.
- Santiago, I. U., Molisani, M. M., Nudi, A. H., Scofield, A. L., Wagener, A. D. L. R., & Limaverde Filho, A. M. (2016). Hydrocarbons and trace metals in mussels in the Macaé coast: Preliminary assessment for a coastal zone under influence of offshore oil field exploration in southeastern Brazil. Marine Pollution Bulletin, 103, 349–353.
- Sarigiannis, D. Α., Karakitsios, S. P., Zikopoulos, D., Nikolaki, S., & Kermenidou, M. (2015). Lung cancer risk from PAHs emitted from biomass combustion. Environmental Research, 137, 147–156.
- Sarria-Villa, R., Ocampo-Duque, W., Páez, M., & Schuhmacher, M. (2016). Presence of PAHs in water and sediments of the Colombian Cauca River during heavy rain episodes, and implications for risk assessment. Science of The Total Environment, 540, 455–465.
- Sather, P. J., Ikonomou, M. G., & Haya, K. (2006). Occurrence of persistent organic pollutants in sediments collected near fish farm sites. Aquaculture, 254, 234–247.
- Sauret, C., Tedetti, M., Guigue, C., Dumas, C., Lami, R., Pujo-Pay, M., … Ghiglione, J. F. (2015). Influence of PAHs among other coastal environmental variables on total and PAH-degrading bacterial communities. Environmental Science and Pollution Research, 23, 4242–4256.
- Saxena, M., Sharma, S. K., Tomar, N., Ghayas, H., Sen, A., Garhwal, R. S., … Mandal, T. K. (2016). Residential biomass burning emissions over Northwestern Himalayan region of India: Chemical characterization and budget estimation. Aerosol and Air Quality Research, 16, 504–518.
- Sayara, T., Borràs, E., Caminal, G., Sarrà, M., & Sánchez, A. (2011). Bioremediation of PAHs-contaminated soil through composting: Influence of bioaugmentation and biostimulation on contaminant biodegradation. International Biodeterioration & Biodegradation, 65, 859–865.
- Sazakli, E., Siavalas, G., Fidaki, A., Christanis, K., Karapanagioti, H. K., & Leotsinidis, M. (2015). Concentrations of persistent organic pollutants and organic matter characteristics as river sediment quality indices. Toxicological and Environmental Chemistry, 98, 1–13.
- Scheurer, M. E., Danysh, H. E., Follen, M., & Lupo, P. J. (2014). Association of traffic-related hazardous air pollutants and cervical dysplasia in an urban multiethnic population: A cross-sectional study. Environmental Health: A Global Access Science Source, 13, 52.
- Schlemitz, S., & Pfannhauser, W. (1997). Supercritical fluid extraction of mononitrated polycyclic aromatic hydrocarbons from tea - Correlation with the PAH concentration. Zeitschrift für Lebensmitteluntersuchung und-Forschung A, 205, 305–310.
- Schlemitz, S., & Pfannhauser, W. (1997). Supercritical fluid extraction of mononitrated polycyclic aromatic hydrocarbons from tea - Correlation with the PAH concentration. European Food Research and Technology, 205, 305–310.
- Seegar, W. S., Yates, M. A., Doney, G. E., Peter Jenny, J., Seegar, T. C. M., Perkins, C., & Giovanni, M. (2015). Migrating Tundra Peregrine Falcons accumulate polycyclic aromatic hydrocarbons along Gulf of Mexico following Deepwater Horizon oil spill. Ecotoxicology, 24, 1102–1111.
- Seemann, F., Peterson, D. R., Witten, P. E., Guo, B. S., Shanthanagouda, A. H., Ye, R. R., … Au, D. W. T. (2015). Insight into the transgenerational effect of benzo[a]pyrene on bone formation in a teleost fish (Oryzias latipes). Comparative Biochemistry and Physiology Part - C: Toxicology and Pharmacology, 178, 60–67.
- Semedo, M., Oliveira, M., Gomes, F., Reis-Henriques, M. A., Delerue-Matos, C., Morais, S., & Ferreira, M. (2014). Seasonal patterns of polycyclic aromatic hydrocarbons in digestive gland and arm of octopus (Octopus vulgaris) from the Northwest Atlantic. Science of the Total Environment, 481, 488–497.
- Shamsipur, M., Gholivand, M. B., Shamizadeh, M., & Hashemi, P. (2015). Preparation and Evaluation of a Novel Solid-Phase Microextraction Fiber Based on Functionalized Nanoporous Silica Coating for Extraction of Polycyclic Aromatic Hydrocarbons From Water Samples Followed by GC–MS Detection. Chromatographia, 78, 795–803.
- Sharma, T. (2014). In silico investigation of polycyclic aromatic hydrocarbons against bacterial 1-2 dioxygenase. Journal of Chemical and Pharmaceutical Research, 6, 873–877.
- Shen, G., Zhang, Y., Wei, S., Chen, Y., Yang, C., Lin, P., … Tao, S. (2014). Indoor/outdoor pollution level and personal inhalation exposure of polycyclic aromatic hydrocarbons through biomass fuelled cooking. Air Quality, Atmosphere & Health, 7, 449–458.
- Sherafatmand, M. & Ng, H. Y. (2015). Using sediment microbial fuel cells (SMFCs) for bioremediation of polycyclic aromatic hydrocarbons (PAHs). Bioresource Technology, 195, 122–130.
- Shi, J., Zheng, G. J. S., Wong, M. H., Liang, H., Li, Y., Wu, Y., Li, P., & Liu, W. (2016). Health risks of polycyclic aromatic hydrocarbons via fish consumption in Haimen bay (China), downstream of an e-waste recycling site (Guiyu). Environmental Research, 147, 233–240.
- Shi, L. K., Zhang, D. D., & Liu, Y. L. (2016). Incidence and survey of polycyclic aromatic hydrocarbons in edible vegetable oils in China. Food Control, 62, 165–170.
- Shi, L. K., Zhang, D. D., & Liu, Y. L. (2016). Survey of polycyclic aromatic hydrocarbons of vegetable oils and oilseeds by GC-MS in China. Analysis, Control, Exposure and Risk Assessment: Food Additives and Contaminants - Part A Chemistry, 33, 603–611.
- Shi, Y., Wu, H., Wang, C., Guo, X., Du, J., & Du, L. (2016). Determination of polycyclic aromatic hydrocarbons in coffee and tea samples by magnetic solid-phase extraction coupled with HPLC-FLD. Food Chemistry, 199, 75–80.
- Shih, Y. J., Binh, N. T., Chen, C. W., Chen, C. F., & Dong, C. D. (2016). Treatability assessment of polycyclic aromatic hydrocarbons contaminated marine sediments using permanganate, persulfate and Fenton oxidation processes. Chemosphere, 150, 294–303.
- Shrestha, B., Anderson, T. A., Acosta-Martinez, V., Payton, P., & Cañas-Carrell, J. E. (2015). The influence of multiwalled carbon nanotubes on polycyclic aromatic hydrocarbon (PAH) bioavailability and toxicity to soil microbial communities in alfalfa rhizosphere. Ecotoxicology and Environmental Safety, 116, 143–149.
- Shukla, K. P., Singh, N. K., & Sharma, S. (2010). Bioremediation: Developments, current practices and perspectives. Genetic Engineering and Biotechnology Journal, 3 (8), 1–20.
- Siebielska, I. (2014). Comparison of changes in selected polycyclic aromatic hydrocarbons concentrations during the composting and anaerobic digestion processes of municipal waste and sewage sludge mixtures. Water Science and Technology, 70, 1617–1624.
- Sikorski, Z. E., & Sinkiewicz, I. (2014). Principles of Smoking. Handbook of Fermented Meat and Poultry (2nd ed.). (pp. 39–45). Hoboken, NJ: John Wiley & Sons.
- Simko, P. (1991). Changes of benzo(a)pyrene contents in smoked fish during storage. Food Chemistry, 40, 293–300.
- Šimko, P. (2002). Determination of polycyclic aromatic hydrocarbons in smoked meat products and smoke flavouring food additives. Journal of Chromatography B, 770, 3–18.
- Šimko, P. (2005). Factors affecting elimination of polycyclic aromatic hydrocarbons from smoked meat foods and liquid smoke flavorings. Molecular Nutrition and Food Research, 49, 637–647.
- Simonich, S. L., & Hites, R. A. (1994). Importance of vegetation in removing polycyclic aromatic hydrocarbons from the atmosphere. Nature, 370, 49–51.
- Simonich, S. L., & Hites, R. A. (1994). Vegetation-atmosphere partitioning of polycyclic aromatic hydrocarbons. Environmental Science & Technology, 28, 939–943.
- Simonich, S. L., & Hites, R. A. (1995). Organic pollutant accumulation in vegetation. Environmental Science & Technology, 29, 2905–2914.
- Singh, A., Chandrasekharan Nair, K., Kamal, R., Bihari, V., Gupta, M. K., Mudiam, M. K. R., … Srivastava, A. K. (2016). Assessing hazardous risks of indoor airborne polycyclic aromatic hydrocarbons in the kitchen and its association with lung functions and urinary PAH metabolites in kitchen workers. Clinica Chimica Acta, 452, 204–213.
- Singh, D. K., & Gupta, T. (2016). Effect through inhalation on human health of PM1 bound polycyclic aromatic hydrocarbons collected from foggy days in northern part of India. Journal of Hazardous Materials, 306, 257–268.
- Singh, L., Varshney, J. G., & Agarwal, T. (2016). Polycyclic aromatic hydrocarbons’ formation and occurrence in processed food. Food Chemistry, 199, 768–781.
- Singh, S., Vashishth, A., & Vishal, A. (2011). PAHs in some brands of tea. Environmental Monitoring and Assessment, 177, 35–38.
- Slezakova, K., Castro, D., Delerue-Matos, C., Alvim-Ferraz, M. d. C., Morais, S., & Pereira, M. d. C. (2013). Impact of vehicular traffic emissions on particulate-bound PAHs: Levels and associated health risks. Atmospheric Research, 127, 141–147.
- Smith, M. R. (1990). The biodegradation of aromatic hydrocarbons by bacteria. Biodegradation, 1, 191–206.
- Snyder, R. A., Vestal, A., Welch, C., Barnes, G., Pelot, R., Ederington-Hagy, M., & Hileman, F. (2014). PAH concentrations in Coquina (Donax spp.) on a sandy beach shoreline impacted by a marine oil spill. Marine Pollution Bulletin, 83, 87–91.
- Soceanu, A., Dobrinas, S., Stanciu, G., & Popescu, V. (2014). Polycyclic aromatic hydrocarbons in vegetables grown in urban and rural areas. Environmental Engineering and Management Journal, 13, 2311–2315.
- Soliman, Y. S., Al Ansari, E. M. S., & Wade, T. L. (2014). Concentration, composition and sources of PAHs in the coastal sediments of the exclusive economic zone (EEZ) of Qatar, Arabian Gulf. Marine Pollution Bulletin, 85, 542–548.
- Soltani, N., Keshavarzi, B., Moore, F., Tavakol, T., Lahijanzadeh, A. R., Jaafarzadeh, N., & Kermani, M. (2015). Ecological and human health hazards of heavy metals and polycyclic aromatic hydrocarbons (PAHs) in road dust of Isfahan metropolis, Iran. Science of The Total Environment, 505, 712–723.
- Song, X., Hu, X., He, M., Liang, R., Li, Y., & Li, F. (2013). Distribution and sources of polycyclic aromatic hydrocarbons in the surface water of Taizi River, Northeast of China. Environmental Monitoring and Assessment, 185, 8375–8382.
- Song, X. F., Chen, Z. Y., Zang, Z. J., Zhang, Y. N., Zeng, F., Peng, Y. P., & Yang, C. (2013). Investigation of polycyclic aromatic hydrocarbon level in blood and semen quality for residents in Pearl River Delta Region in China. Environment International, 60, 97–105.
- Sopeña, F., Laiz, L., Morillo, E., Sanchez-Trujillo, M. A., Villaverde, J., Jurado, V., & Saiz-Jimenez, C. (2014). Phenanthrene Biodegradation by Pseudomonas xanthomarina Isolated from an Aged Contaminated Soil. Clean– Soil, Air, Water, 42, 785–790.
- Sørensen, L., Silva, M. S., Booth, A. M., & Meier, S. (2016). Optimization and comparison of miniaturized extraction techniques for PAHs from crude oil exposed Atlantic cod and haddock eggs. Analytical and Bioanalytical Chemistry, 408, 1023–1032.
- Squadrone, S., Favaro, L., Abete, M. C., Vivaldi, B., & Prearo, M. (2014). Polycyclic aromatic hydrocarbon levels in European catfish from the upper Po River basin. Environmental Monitoring and Assessment, 186, 2313–2320.
- Srivastava, V. K., Srivastava, P. K., & Misra, U. K. (1985). Polycyclic aromatic hydrocarbons of coal fly ash: Analysis by gas-liquid chromatography using nematic liquid crystals. Journal of Toxicology and Environmental Health, 15, 333–337.
- Steinig, J. (1976). Benzo(a)pyrene contents in smoked fish in dependence of smoking procedure. Zeitschrift für Lebensmittel-Untersuchung und -Forschung, 162, 235–242.
- Storey, S., Ashaari, M. M., McCabe, G., Harty, M., Dempsey, R., Doyle, O., … Doyle, E. M. (2014). Microbial community structure during fluoranthene degradation in the presence of plants. Journal of Applied Microbiology, 117, 74–84.
- Stout, S. A., & Emsbo-Mattingly, S. D. (2008). Concentration and character of PAHs and other hydrocarbons in coals of varying rank—Implications for environmental studies of soils and sediments containing particulate coal. Organic Geochemistry, 39, 801–819.
- Studabaker, W. B., Krupa, S., Jayanty, R. K. M., & Raymer, J. H. (2012). Measurement of polynuclear aromatic hydrocarbons (PAHs) in epiphytic lichens for receptor modeling in the athabasca oil sands region (AOSR): A Pilot study. Developments in Environmental Science, 11, 391–425.
- Stumpe-Viksna, I., Bartkevičs, V., Kukare, A., & Morozovs, A. (2008). Polycyclic aromatic hydrocarbons in meat smoked with different types of wood. Food Chemistry, 110, 794–797.
- Su, Y.-H., & Zhu, Y.-G. (2008). Uptake of selected PAHs from contaminated soils by rice seedlings (Oryza sativa) and influence of rhizosphere on PAH distribution. Environmental Pollution, 155, 359–365.
- Subramanian, A., Kunisue, T., & Tanabe, S. (2015). Recent status of organohalogens, heavy metals and PAHs pollution in specific locations in India. Chemosphere, 137, 122–134.
- Suciu, N. A., Lamastra, L., & Trevisan, M. (2015). PAHs content of sewage sludge in Europe and its use as soil fertilizer. Waste Management, 41, 119–127.
- Suman, S., Sinha, A., & Tarafdar, A. (2016). Polycyclic aromatic hydrocarbons (PAHs) concentration levels, pattern, source identification and soil toxicity assessment in urban traffic soil of Dhanbad, India. Science of The Total Environment, 545–546, 353–360.
- Sun, C., Zhang, J., Ma, Q., Chen, Y., & Ju, H. (2016). Polycyclic aromatic hydrocarbons (PAHs) in water and sediment from a river basin: sediment–water partitioning, source identification and environmental health risk assessment. Environmental Geochemistry and Health, 39, 1–12.
- Sun, C., Zhang, J., Ma, Q., Zhang, F., & Chen, Y. (2016). Risk assessment of polycyclic aromatic hydrocarbons (PAHs) in sediments from a mixed-use reservoir. Human and Ecological Risk Assessment, 22, 447–459.
- Sun, H., Wang, W., Guo, S., & Zhang, Y. (2016). In situ investigation into surfactant effects on the clearance of polycyclic aromatic hydrocarbons adsorbed onto soybean leaf surfaces. Environmental Pollution, 210, 330–337.
- Sun, L., & Zang, S. (2013). Relationship between polycyclic aromatic hydrocarbons (PAHs) and particle size in dated core sediments in Lake Lianhuan, Northeast China. Science of The Total Environment, 461–462, 180–187.
- Sun, M., Fu, D., Teng, Y., Shen, Y., Luo, Y., Li, Z., & Christie, P. (2011). In situ phytoremediation of PAH-contaminated soil by intercropping alfalfa (Medicago sativa L.) with tall fescue (Festuca arundinacea Schreb.) and associated soil microbial activity. Journal of Soils and Sediments, 11, 980–989.
- Sun, R., Belcher, R. W., Liang, J., Wang, L., Thater, B., Crowley, D. E., & Wei, G. (2015). Effects of cowpea (Vigna unguiculata) root mucilage on microbial community response and capacity for phenanthrene remediation. Journal of Environmental Sciences (China), 33, 45–59.
- Sun, T. R., Cang, L., Wang, Q. Y., Zhou, D. M., Cheng, J. M., & Xu, H. (2010). Roles of abiotic losses, microbes, plant roots, and root exudates on phytoremediation of PAHs in a barren soil. Journal of Hazardous Materials, 176, 919–925.
- Sun, Y., Zhou, Q., Xu, Y., Wang, L., & Liang, X. (2011). Phytoremediation for co-contaminated soils of benzo[a]pyrene (B[a]P) and heavy metals using ornamental plant Tagetes patula. Journal of Hazardous Materials, 186, 2075–2082.
- Sun, Y. Y., Xu, H. X., Li, J. H., Shi, X. Q., Wu, J. C., Ji, R., & Guo, H. Y. (2016). Phytoremediation of soils contaminated with phenanthrene and cadmium by growing willow (Salix × Aureo-Pendula CL ‘j1011’). International Journal of Phytoremediation, 18, 150–156.
- Sundt, R. C., Pampanin, D. M., Grung, M., Baršiene, J., & Ruus, A. (2011). PAH body burden and biomarker responses in mussels (Mytilus edulis) exposed to produced water from a North Sea oil field: Laboratory and field assessments. Marine Pollution Bulletin, 62, 1498–1505.
- Sundt, R. C., Ruus, A., Jonsson, H., Skarphédinsdóttir, H., Meier, S., Grung, M., … Pampanin, D. M. (2012). Biomarker responses in Atlantic cod (Gadus morhua) exposed to produced water from a North Sea oil field: Laboratory and field assessments. Marine Pollution Bulletin, 64, 144–152.
- Sungthong, R., van West, P., Cantos, M., & Ortega-Calvo, J. J. (2015). Development of eukaryotic zoospores within polycyclic aromatic hydrocarbon (PAH)-polluted environments: A set of behaviors that are relevant for bioremediation. Science of the Total Environment, 511, 767–776.
- Sunner, J., Gahm, K.-H., Ikonomou, M., & Kebarle, P. (1988). Real-time analysis of polyaromatic hydrocarbons in flames using Atmospheric Pressure Ionization and tandem mass spectrometry. Combustion and Flame, 73, 147–161.
- Szczepaniak, Z., Cyplik, P., Juzwa, W., Czarny, J., Staninska, J., & Piotrowska-Cyplik, A. (2015). Antibacterial effect of the Trichoderma viride fungi on soil microbiome during PAH’s biodegradation. International Biodeterioration and Biodegradation, 104, 170–177.
- Szulejko, J. E., Kim, K.-H., Brown, R. J. C., & Bae, M.-S. (2014). Review of progress in solvent-extraction techniques for the determination of polyaromatic hydrocarbons as airborne pollutants. TrAC Trends in Analytical Chemistry, 61, 40–48.
- Taghvaee, Z., Piravivanak, Z., Rezaei, K., & Faraji, M. (2015). Determination of Polycyclic Aromatic Hydrocarbons (PAHs) in Olive and Refined Pomace Olive Oils with Modified Low Temperature and Ultrasound-Assisted Liquid-Liquid Extraction Method Followed by the HPLC/FLD. Food Analytical Methods, 9, 1220–1227.
- Tai, C., Zhang, K., Zhou, T., Zhao, T., Xiao, C., & Wu, L. (2012). Distribution characteristics of polycyclic aromatic hydrocarbons in the soil from the middle line source area of the south-to-north water diversion project. Huanjing Kexue Xuebao/Acta Scientiae Circumstantiae, 32, 402–409.
- Tai, C., Zhou, T., Zhao, T., Wu, L., & Wang, Q. (2009). Level and source of polycyclic aromatic hydrocarbons in river water and runoff from Danjiangkou reservoir area, China (Vol. 2, pp. 620–622). In 2009 International Conference on Energy and Environment Technology, ICEET 2009.
- Takeuchi, M., Hamana, K., & Hiraishi, A. (2001). Proposal of the thegenus Sphingomonas sensu stricto and the three new genera, Sphingobium, Novosphingobium and Sphingopyxis, on the basis ofphylogenetic and chemotaxonomic analyses. Int. J. Sys. Evol. Microbiol, 51, 1405–1417.
- Talaska, G., Thoroman, J., Schuman, B., & Käfferlein, H. U. (2014). Biomarkers of polycyclic aromatic hydrocarbon exposure in European coke oven workers. Toxicology Letters, 231, 213–216.
- Tanabe, A., Suzuki, S., & Hanada, Y. (2000). Evaluation of an ultrasonic extraction method compared with the Soxhlet extraction method for the determination of polyaromatic hydrocarbons and n-alkanes in airborne particulate matter. Bunseki Kagaku, 48, 939–944.
- Tang, J., Feng, T., Cui, C., & Feng, Y. (2013). Simultaneous biodegradation of phenanthrene and oxidation of arsenite by a dual-functional bacterial consortium. International Biodeterioration and Biodegradation, 82, 173–179.
- Tang, N., Hakamata, M., Sato, K., Okada, Y., Yang, X., Tatematsu, M., … Hayakawa, K. (2015). Atmospheric behaviors of polycyclic aromatic hydrocarbons at a Japanese remote background site, Noto peninsula, from 2004 to 2014. Atmospheric Environment, 120, 144–151.
- Tang, X., Shen, C., Cheema, S. A., Chen, L., Xiao, X., Zhang, C., … Chen, Y. (2010). Levels and distributions of polycyclic aromatic hydrocarbons in agricultural soils in an emerging e-waste recycling town in Taizhou area, China. Journal of Environmental Science and Health - Part A Toxic/Hazardous Substances and Environmental Engineering, 45, 1076–1084.
- Tansel, B., Fuentes, C., Sanchez, M., Predoi, K.& Acevedo, M. (2011). Persistence profile of polyaromatic hydrocarbons in shallow and deep Gulf waters and sediments: Effect of water temperature and sediment–water partitioning characteristics. Marine Pollution Bulletin, 62, 2659–2665.
- Tansel, B., Lee, M., & Tansel, D. Z. (2013). Comparison of fate profiles of PAHs in soil, sediments and mangrove leaves after oil spills by QSAR and QSPR. Marine Pollution Bulletin, 73, 258–262.
- Tauler, M., Vila, J., Nieto, J. M., & Grifoll, M. (2015). Key high molecular weight PAH-degrading bacteria in a soil consortium enriched using a sand-in-liquid microcosm system. Applied Microbiology and Biotechnology, 1–16.
- Tavakoly Sany, S. B., Hashim, R., Salleh, A., Rezayi, M., Mehdinia, A., & Safari, O. (2014). Polycyclic aromatic hydrocarbons in coastal sediment of Klang Strait, Malaysia: Distribution pattern, risk assessment and sources. PLoS ONE, 9, e94907.
- Teixeira, E. C., Pra, D., Idalgo, D., Henriques, J. A. P., & Wiegand, F. (2012). DNA-damage effect of polycyclic aromatic hydrocarbons from urban area, evaluated in lung fibroblast cultures. Environmental Pollution, 162, 430–438.
- Tejeda-Agredano, M. C., Mayer, P., & Ortega-Calvo, J. J. (2014). The effect of humic acids on biodegradation of polycyclic aromatic hydrocarbons depends on the exposure regime. Environmental Pollution, 184, 435–442.
- Tervahauta, A. I., Fortelius, C., Tuomainen, M., Åkerman, M.-L., Rantalainen, K., Sipilä, T., … Yrjälä, K. (2009). Effect of birch (Betula spp.) and associated rhizoidal bacteria on the degradation of soil polyaromatic hydrocarbons, PAH-induced changes in birch proteome and bacterial community. Environmental Pollution, 157, 341–346.
- Terzaghi, E., Scacchi, M., Cerabolini, B., Jones, K. C., & Di Guardo, A. (2015). Estimation of polycyclic aromatic hydrocarbon variability in air using high volume, film, and vegetation as samplers. Environmental Science and Technology, 49, 5520–5528.
- Thea, A. E., Ferreira, D., Brumovsky, L. A., & Schmalko, M. E. (2016). Polycyclic aromatic hydrocarbons (PAHs) in yerba maté (Ilex paraguariensis St. Hil) traditional infusions (mate and tereré). Food Control, 60, 215–220.
- Thomas, F., Lorgeoux, C., Faure, P., Billet, D., & Cébron, A. (2016). Isolation and substrate screening of polycyclic aromatic hydrocarbon degrading bacteria from soil with long history of contamination. International Biodeterioration and Biodegradation, 107, 1–9.
- Thorsteinsson, T. (1969). Polycyclic hydrocarbons in commercially- and home-smoked food in Iceland. Cancer, 23, 455–457.
- Tiruta-Barna, L., Mahjoub, B., Faure, L., Hanna, K., Bayard, R., & Gourdon, R. (2006). Assessment of the multi-compound non-equilibrium dissolution behaviour of a coal tar containing PAHs and phenols into water. Journal of Hazardous Materials, 132, 277–286.
- Tobiszewski, M., & Namieśnik, J. (2012). PAH diagnostic ratios for the identification of pollution emission sources. Environmental Pollution, 162, 110–119.
- Tomashuk, T. A., Truong, T. M., Mantha, M., & McGowin, A. E. (2012). Atmospheric polycyclic aromatic hydrocarbon profiles and sources in pine needles and particulate matter in Dayton, Ohio, USA. Atmospheric Environment, 51, 196–202.
- Tóth, L., & Potthast, K. (1984). Chemical aspects of the smoking of meat and meat products. Advances in Food Research., 29, 87–158.
- Tripathi, P., Singh, P. C., Mishra, A., Chauhan, P. S., Dwivedi, S., Bais, R. T., & Tripathi, R. D. (2013). Trichoderma: A potential bioremediator for environmental clean up. Clean Technologies and Environmental Policy, 15, 541–550.
- Tripathy, V., Basak, B. B., Varghese, T. S., & Saha, A. (2015). Residues and contaminants in medicinal herbs - A review. Phytochemistry Letters, 14, 67–78.
- Tsapakis, M., & Stephanou, E. G. (2005). Occurrence of gaseous and particulate polycyclic aromatic hydrocarbons in the urban atmosphere: study of sources and ambient temperature effect on the gas/particle concentration and distribution. Environmental Pollution, 133, 147–156.
- Tunçal, T., & Uslu, O. (2015). Industrial sludge remediation with photonic treatment using Ti-Ag nano-composite thin films: Persistent organic pollutant removal from sludge matrix. Journal of Environmental Management, 149, 37–45.
- Unwin, J., Cocker, J., Scobbie, E., & Chambers, H. (2006). An assessment of occupational exposure to polycyclic aromatic hydrocarbons in the UK. Ann Occup Hyg, 50, 395–403.
- Usman, M., Chaudhary, A., Biache, C., Faure, P., & Hanna, K. (2015). Effect of thermal pre-treatment on the availability of PAHs for successive chemical oxidation in contaminated soils. Environmental Science and Pollution Research, 23, 1371–1380.
- Van der Wat, L., & Forbes, P. B. C. (2015). Lichens as biomonitors for organic air pollutants. TrAC Trends in Analytical Chemistry, 64, 165–172.
- Van Metre, P. C., & Mahler, B. J. (2014). PAH concentrations in lake sediment decline following ban on coal-tar-based pavement sealants in Austin, Texas. Environmental Science and Technology, 48, 7222–7228.
- Varnosfaderany, M. N., Bakhtiari, A. R., Gu, Z., & Chu, G. (2014). Vertical distribution and source identification of polycyclic aromatic hydrocarbons (PAHs) in southwest of the Caspian Sea: Most petrogenic events during the late Little Ice Age. Marine Pollution Bulletin, 87, 152–163.
- Vecino, X., Rodríguez-López, L., Cruz, J. M., & Moldes, A. B. (2015). Sewage Sludge Polycyclic Aromatic Hydrocarbon (PAH) Decontamination Technique Based on the Utilization of a Lipopeptide Biosurfactant Extracted from Corn Steep Liquor. Journal of Agricultural and Food Chemistry, 63, 7143–7150.
- Verma, R., Patel, K. S., & Verma, S. K. (2015). Indoor polycyclic aromatic hydrocarbon concentration in Central India. Polycyclic Aromatic Compounds,.
- Verma, R., Patel, K. S., & Verma, S. K. (2016). Indoor polycyclic aromatic hydrocarbon concentration in Central India. Polycyclic Aromatic Compounds, 36, 152–168.
- Verma, S. K., Masto, R. E., Gautam, S., Choudhury, D. P., Ram, L. C., Maiti, S. K., & Maity, S. (2015). Investigations on PAHs and trace elements in coal and its combustion residues from a power plant. Fuel, 162, 138–147.
- Veses, O., Mosteo, R., Ormad, M. P., & Ovelleiro, J. L. (2014). Freshwater sediment quality in Spain. Environmental Earth Sciences, 72, 2917–2929.
- Vichi, S., Pizzale, L., Conte, L. S., Buxaderas, S., & López-Tamames, E. (2005). Simultaneous determination of volatile and semi-volatile aromatic hydrocarbons in virgin olive oil by headspace solid-phase microextraction coupled to gas chromatography/mass spectrometry. Journal of Chromatography A, 1090, 146–154.
- Vidal, M., Domínguez, J., & Luís, A. (2011). Spatial and temporal patterns of polycyclic aromatic hydrocarbons (PAHs) in eggs of a coastal bird from northwestern Iberia after a major oil spill. Science of The Total Environment, 409, 2668–2673.
- Vieira de Souza, C., & Corrêa, S. M. (2015). Polycyclic aromatic hydrocarbon emissions in diesel exhaust using gas chromatography-mass spectrometry with programmed temperature vaporization and large volume injection. Atmospheric Environment, 103, 222–230.
- Vignet, C., Joassard, L., Lyphout, L., Guionnet, T., Goubeau, M., Le Menach, K., … Cousin, X. (2015). Exposures of zebrafish through diet to three environmentally relevant mixtures of PAHs produce behavioral disruptions in unexposed F1 and F2 descendant. Environmental Science and Pollution Research, 22, 16371–16383.
- Villanueva, F., Tapia, A., Cabañas, B., Martínez, E., & Albaladejo, J. (2015). Characterization of particulate polycyclic aromatic hydrocarbons in an urban atmosphere of central-southern Spain. Environmental Science and Pollution Research, 22, 18814–18823.
- Villar, P., Callejón, M., Alonso, E., Jiménez, J. C., & Guiraúm, A. (2006). Temporal evolution of polycyclic aromatic hydrocarbons (PAHs) in sludge from wastewater treatment plants: Comparison between PAHs and heavy metals. Chemosphere, 64, 535–541.
- Viñas, L., Franco, A., Blanco, X., Bargiela, J., Soriano, J. A., Perez-Fernandez, B., & Gonzalez, J. J. (2012). Temporal and spatial changes of PAH concentrations in Mytilus galloprovincialis from Ria de Vigo (NW Spain). Environmental Science and Pollution Research, 19, 529–539.
- Viñas, P., Campillo, N., Aguinaga, N., Pérez-Cánovas, E., & Hernández-Córdoba, M. (2007). Use of headspace solid-phase microextraction coupled to liquid chromatography for the analysis of polycyclic aromatic hydrocarbons in tea infusions. Journal of Chromatography A, 1164, 10–17.
- Vojtisek-Lom, M., Pechout, M., Dittrich, L., Beránek, V., Kotek, M., Schwarz, J., … Topinka, J. (2015). Polycyclic aromatic hydrocarbons (PAH) and their genotoxicity in exhaust emissions from a diesel engine during extended low-load operation on diesel and biodiesel fuels. Atmospheric Environment, 109, 9–18.
- Vuković, G., Aničić Uroševic, M., Razumenić, I., Kuzmanoski, M., Pergal, M., Škrivanj, S., & Popović, A. (2014). Air quality in urban parking garages (PM10, major and trace elements, PAHs): Instrumental measurements vs. active moss biomonitoring. Atmospheric Environment, 85, 31–40.
- Wagrowski, D. M., & Hites, R.A (1997). Polycyclic aromatic hydrocarbon accumulation in urban, suburban, and rural vegetation. Environmental Science & Technology, 31, 279–282.
- Waigi, M. G., Kang, F., Goikavi, C., Ling, W., & Gao, Y. (2015). Phenanthrene biodegradation by sphingomonads and its application in the contaminated soils and sediments: A review. International Biodeterioration and Biodegradation, 104, 333–349.
- Walker, T. R., & MacAskill, D. (2014). Monitoring water quality in Sydney Harbour using blue mussels during remediation of the Sydney Tar Ponds, Nova Scotia, Canada. Environmental Monitoring and Assessment, 186, 1623–1638.
- Walker, T. R., Willis, R., Gray, T., MacLean, B., McMillan, S., Leroy, M., … Smith, M. (2015). Ecological Risk Assessment of Sediments in Sydney Harbour, Nova Scotia, Canada. Soil and Sediment Contamination, 24, 471–493.
- Wang, C., Dao, X., Zhang, L. L., Lv, Y. B., & Teng, E. J. (2015). Characteristics and toxicity assessment of airborne particulate polycyclic aromatic hydrocarbons of four background sites in China. Zhongguo Huanjing Kexue/China Environmental Science, 35, 3543–3549.
- Wang, C., Yu, L., Zhang, Z., Wang, B., & Sun, H. (2014). Tourmaline combined with Phanerochaete chrysosporium to remediate agricultural soil contaminated with PAHs and OCPs. Journal of Hazardous Materials, 264, 439–448.
- Wang, D., Feng, C., Huang, L., Niu, J., & Shen, Z. (2013). Historical deposition behaviors of PAHs in the Yangtze River Estuary: Role of the sources and water currents. Chemosphere, 90(6), 2020–2026.
- Wang, J.-Z., Chen, T.-H., Zhu, C.-Z., & Peng, S.-C. (2014). Trace organic pollutants in sediments from Huaihe River, China: Evaluation of sources and ecological risk. Journal of Hydrology, 512, 463–469.
- Wang, J., Geng, N. B., Xu, Y. F., Zhang, W. D., Tang, X. Y., & Zhang, R. Q. (2014). PAHs in PM2.5 in Zhengzhou: concentration, carcinogenic risk analysis, and source apportionment. Environmental Monitoring and Assessment, 186, 7461–7473.
- Wang, L., Xu, X., & Lu, X. (2016). Composition, source and potential risk of polycyclic aromatic hydrocarbons (PAHs) in vegetable soil from the suburbs of Xianyang City, Northwest China: a case study. Environmental Earth Sciences, 75(1), 1–13.
- Wang, L., Zhao, Y., Liu, X., Huang, T., Wang, Y., Gao, H., & Ma, J. (2015). Cancer risk of petrochemical workers exposed to airborne PAHs in industrial Lanzhou City, China. Environmental Science and Pollution Research, 22, 19793–19803.
- Wang, M., Wang, C., Hu, X., Zhang, H., He, S., & Lv, S. (2015). Distributions and sources of petroleum, aliphatic hydrocarbons and polycyclic aromatic hydrocarbons (PAHs) in surface sediments from Bohai Bay and its adjacent river, China. Marine Pollution Bulletin, 90, 88–94.
- Wang, W., Huang, M.-J., Chan, C.-Y., Cheung, K. C., & Wong, M. H. (2013). Risk assessment of non-dietary exposure to polycyclic aromatic hydrocarbons (PAHs) via house PM2.5, TSP and dust and the implications from human hair. Atmospheric Environment, 73, 204–213.
- Wang, W., Simonich, S., Giri, B., Chang, Y., Zhang, Y., Jia, Y., … Lu, X. (2011). Atmospheric concentrations and air–soil gas exchange of polycyclic aromatic hydrocarbons (PAHs) in remote, rural village and urban areas of Beijing-Tianjin region, North China. Science of The Total Environment, 409, 2942–2950.
- Wang, W., Wu, F., Zheng, J., & Wong, M. H. (2013). Risk assessments of PAHs and Hg exposure via settled house dust and street dust, linking with their correlations in human hair. Journal of Hazardous Materials, 263, 627–637.
- Wang, X.-T., Miao, Y., Zhang, Y., Li, Y.-C., Wu, M.-H., & Yu, G. (2013). Polycyclic aromatic hydrocarbons (PAHs) in urban soils of the megacity Shanghai: Occurrence, source apportionment and potential human health risk. Science of The Total Environment, 447, 80–89.
- Wang, X., Thai, P. K., Li, Y., Li, Q., Wainwright, D., Hawker, D. W., & Mueller, J. F. (2016). Changes in atmospheric concentrations of polycyclic aromatic hydrocarbons and polychlorinated biphenyls between the 1990s and 2010s in an Australian city and the role of bushfires as a source. Environmental Pollution, 213, 223–231.
- Wang, X., Xi, B., Huo, S., Sun, W., Pan, H., Zhang, J., … Liu, H. (2013). Characterization, treatment and releases of PBDEs and PAHs in a typical municipal sewage treatment plant situated beside an urban river, East China. Journal of Environmental Sciences, 25, 1281–1290.
- Wang, Y., Rong, Z., Qin, Y., Peng, J., Li, M., Lei, J., … Shijin, S. (2016). The impact of fuel compositions on the particulate emissions of direct injection gasoline engine. Fuel, 166, 543–552.
- Wang, Y., Tian, Z., Zhu, H., Cheng, Z., Kang, M., Luo, C., … Zhang, G. (2012). Polycyclic aromatic hydrocarbons (PAHs) in soils and vegetation near an e-waste recycling site in South China: Concentration, distribution, source, and risk assessment. Science of The Total Environment, 439, 187–193.
- Wang, Y. B., Liu, C. W., Kao, Y. H., & Jang, C. S. (2015). Characterization and risk assessment of PAH-contaminated river sediment by using advanced multivariate methods. Science of the Total Environment, 524–525, 63–73.
- Wang, Y. B., Sojinu, S. O., Sun, J. L., Ni, H. G., Zeng, H., & Zou, M. (2015). Are cockroaches reliable bioindicators of persistent organic pollutant contamination of indoor environments? Ecological Indicators, 50, 44–49.
- Wang, Z., Liu, M., & Yang, Y. (2014). Characterization and sources analysis of polycyclic aromatic hydrocarbons in surface sediments in the Yangtze River Estuary. Environmental Earth Sciences, 73, 2453–2462.
- Wang, Z., Wang, S., Nie, J., Wang, Y., & Liu, Y. (2016). Assessment of polycyclic aromatic hydrocarbons in indoor dust from varying categories of rooms in Changchun city, northeast China. Environmental Geochemistry and Health, 1–13.
- Waqas, M., Khan, S., Chao, C., Shamshad, I., Qamar, Z., & Khan, K. (2014). Quantification of PAHs and health risk via ingestion of vegetable in Khyber Pakhtunkhwa Province, Pakistan. Science of the Total Environment, 497, 448–458.
- Warshawsky, D., Radike, M., Jayasimhulu, K., & Cody, T. (1988). Metabolism of Benzo[a]pyrenebyadioxygenasesystemoffreshwatergreenalga Selenastrum capricornutum. Biochemical and Biophysical Research Communications, 152, 540–544.
- Warshawsky, J. D., Cody, T., Radike, M., Reilman, R., Schumann, B., LaDow, K., & Schneider, J. (1995). Biotransformation of Benzo[a]pyrene and other polycyclic aro- matichydrocarbonsandheterocyclicanalogsbyseveralgreenalgaeandother algal species under gold and white light. Chemico-Biol. Interact., 97, 131–148.
- Waszak, D. Q., Da Cunha, A. C. B., Agarrallua, M. R. A., Goebel, C. S., & Sampaio, C. H. (2015). Bioremediation of a Benzo[a]Pyrene-Contaminated Soil Using a Microbial Consortium with Pseudomonas aeruginosa, Candida albicans, Aspergillus flavus, and Fusarium sp. Water, Air, and Soil Pollution, 226, 319.
- Watts, A. W., Ballestero, T. P., & Gardner, K. H. (2006). Uptake of polycyclic aromatic hydrocarbons (PAHs) in salt marsh plants Spartina alterniflora grown in contaminated sediments. Chemosphere, 62, 1253–1260.
- Wei, C., Bandowe, B. A. M., Han, Y., Cao, J., Zhan, C., & Wilcke, W. (2015). Polycyclic aromatic hydrocarbons (PAHs) and their derivatives (alkyl-PAHs, oxygenated-PAHs, nitrated-PAHs and azaarenes) in urban road dusts from Xi’an, Central China. Chemosphere, 134, 512–520.
- Wei, C., Han, Y., Bandowe, B. A. M., Cao, J., Huang, R.-J., Ni, H., … Wilcke, W. (2015). Occurrence, gas/particle partitioning and carcinogenic risk of polycyclic aromatic hydrocarbons and their oxygen and nitrogen containing derivatives in Xi’an, central China. Science of The Total Environment, 505, 814–822.
- Wei, H., Guangbin, L., Yong, T., & Qin, Z. (2015). Emission of polycyclic aromatic hydrocarbons from different types of motor vehicles’ exhaust. Environmental Earth Sciences, 74, 5557–5564.
- Wei, S., Zhou, Q., Koval, P. V., & Belogolova, G. A. (2006). Phytoremediation of organic pollutants-contaminated environment: A research review. Chinese Journal of Ecology, 25, 716–721.
- Wenzl, T., Simon, R., Anklam, E., & Kleiner, J. (2006). Analytical methods for polycyclic aromatic hydrocarbons (PAHs) in food and the environment needed for new food legislation in the European Union. TrAC Trends in Analytical Chemistry, 25, 716–725.
- West, J. E., O’Neill, S. M., Ylitalo, G. M., Incardona, J. P., Doty, D. C., & Dutch, M. E. (2014). An evaluation of background levels and sources of polycyclic aromatic hydrocarbons in naturally spawned embryos of Pacific herring (Clupea pallasii) from Puget Sound, Washington, USA. Science of the Total Environment, 499, 114–124.
- White, A. J., Bradshaw, P. T., Herring, A. H., Teitelbaum, S. L., Beyea, J., Stellman, S. D., … Gammon, M. D. (2016). Exposure to multiple sources of polycyclic aromatic hydrocarbons and breast cancer incidence. Environment International, 89–90, 185–192.
- White, A. J., Chen, J., Teitelbaum, S. L., McCullough, L. E., Xu, X., Hee Cho, Y., … Gammon, M. D. (2016). Sources of polycyclic aromatic hydrocarbons are associated with gene-specific promoter methylation in women with breast cancer. Environmental Research, 145, 93–100.
- White, A. J., Teitelbaum, S. L., Stellman, S. D., Beyea, J., Steck, S. E., Mordukhovich, I., … Gammon, M. D. (2015). Indoor air pollution exposure from use of indoor stoves and fireplaces in association with breast cancer: A case-control study (pp. 1–12). A Global Access Science Source: Environmental Health.
- Wickliffe, J., Overton, E., Frickel, S., Howard, J., Wilson, M., Simon, B., … Kane, A. (2014). Evaluation of polycyclic aromatic hydrocarbons using analytical methods, toxicology, and risk assessment research: Seafood safety after a petroleum spill as an example. Environmental Health Perspectives, 122, 6–9.
- Wickramasinghe, A. P., Karunaratne, D. G. G. P., & Sivakanesan, R. (2011). PM10-bound polycyclic aromatic hydrocarbons: Concentrations, source characterization and estimating their risk in urban, suburban and rural areas in Kandy, Sri Lanka. Atmospheric Environment, 45, 2642–2650.
- Wild, S. R., Berrow, M. L., & Jones, K. C. (1991). The persistence of polynuclear aromatic hydrocarbons (PAHs) in sewage sludge amended agricultural soils. Environmental Pollution, 72, 141–157.
- Wilk, A., Waligórski, P., Lassak, A., Vashistha, H., Lirette, D., Tate, D., … Reiss, K. (2013). Polycyclic aromatic hydrocarbons-induced ROS accumulation enhances mutagenic potential of T-antigen from human polyomavirus JC. Journal of Cellular Physiology, 228, 2127–2138.
- Wilson, S. C., & Jones, K. C. (1993). Bioremediation of soil contaminated with polynuclear aromatic hydrocarbons (PAHs): A review. Environmental Pollution, 81, 229–249.
- Wilson, W. B., Hewitt, U., Miller, M., & Campiglia, A. D. (2014). Water analysis of the sixteen environmental protection agency-polycyclic aromatic hydrocarbons via solid-phase nanoextraction-gas chromatography/mass spectrometry. Journal of Chromatography A, 1345, 1–8.
- Winquist, E., Björklöf, K., Schultz, E., Räsänen, M., Salonen, K., Anasonye, F., … Tuomela, M. (2014). Bioremediation of PAH-contaminated soil with fungi - From laboratory to field scale. International Biodeterioration and Biodegradation, 86, 238–247.
- Wirasnita, R., & Hadibarata, T. (2016). Potential of the White-Rot Fungus Pleurotus pulmonarius F043 for Degradation and Transformation of Fluoranthene. Pedosphere, 26, 49–54.
- Włodarczyk-Makuła, M. (2014). Persistence of two-, three- and four-ring of PAHs in sewage sludge deposited in different light conditions. Desalination and Water Treatment, 57, 1184–1199.
- Wolf, A., Bergmann, A., Wilken, R. D., Gao, X., Bi, Y., Chen, H., & Schüth, C. (2013). Occurrence and distribution of organic trace substances in waters from the Three Gorges Reservoir, China. Environmental Science and Pollution Research, 20, 7124–7139.
- Wong, K.-S., Huang, Q., Au, C.-H., Wang, J., & Kwan, H.-S. (2012). Biodegradation of dyes and polyaromatic hydrocarbons by two allelic forms of Lentinula edodes laccase expressed from Pichia pastoris. Bioresource Technology, 104, 157–164.
- Wu, D., Wang, Y. L., Liu, W. J., Chen, Y. C., Fu, X. F., Tao, S., & Liu, W. X. (2016). Concentrations and component profiles PAHs in surface soils and wheat grains from the cornfields close to the steel smelting industry in Handan, Hebei Province. Huanjing Kexue/Environmental Science, 37, 740–749.
- Wu, J., Teng, Y., & Chen, H. (2014). Source apportionment for sediment PAHs using hybrid genetic pattern search treatment of a chemical mass balance receptor model: Application to the Pearl River Delta region, China. Environmental Monitoring and Assessment, 186, 6651–6662.
- Wu, Q., Leung, J. Y. S., Tam, N. F. Y., Chen, S., Mai, B., Zhou, X., … Geng, X. (2014). Biological risk and pollution history of polycyclic aromatic hydrocarbons (PAHs) in Nansha mangrove, South China. Marine Pollution Bulletin, 85, 92–98.
- Wu, Y., Wang, X., Li, Y., Ya, M., Luo, H., & Hong, H. (2015). Polybrominated diphenyl ethers, organochlorine pesticides, and polycyclic aromatic hydrocarbons in water from the Jiulong River Estuary, China: levels, distributions, influencing factors, and risk assessment. Environmental Science and Pollution Research, 24, 8933–8945.
- Wynder, E. L., & Hoffmann, D. (1959). The carcinogenicity of benzofluoranthenes. Cancer, 12, 1194–1199.
- Xia, W., Du, Z., Cui, Q., Dong, H., Wang, F., He, P., & Tang, Y. (2014). Biosurfactant produced by novel Pseudomonas sp. WJ6 with biodegradation of n-alkanes and polycyclic aromatic hydrocarbons. Journal of Hazardous Materials, 276, 489–498.
- Xia, Z., Duan, X., Qiu, W., Liu, D., Wang, B., Tao, S., … Hu, X. (2010). Health risk assessment on dietary exposure to polycyclic aromatic hydrocarbons (PAHs) in Taiyuan, China. Science of The Total Environment, 408, 5331–5337.
- Xiao, N., Liu, R., Jin, C., & Dai, Y. (2015). Efficiency of five ornamental plant species in the phytoremediation of polycyclic aromatic hydrocarbon (PAH)-contaminated soil. Ecological Engineering, 75, 384–391.
- Xu, J., Guo, J.-Y., Liu, G.-R., Shi, G.-L., Guo, C.-S., Zhang, Y., & Feng, Y.-C. (2014). Historical trends of concentrations, source contributions and toxicities for PAHs in dated sediment cores from five lakes in western China. Science of The Total Environment, 470–471, 519–526.
- Xu, J., Peng, X., Guo, C. S., Xu, J., Lin, H. X., Shi, G. L., … Tysklind, M. (2016). Sediment PAH source apportionment in the Liaohe River using the ME2 approach: A comparison to the PMF model. Science of the Total Environment, 553, 164–171.
- Xu, S. N., Zhao, Q., He, H. B., Yuan, B. F., Feng, Y. Q., & Yu, Q. W. (2014). Rapid determination of polycyclic aromatic hydrocarbons in environmental water based on magnetite nanoparticles/polypyrrole magnetic solid-phase extraction. Analytical Methods, 6, 7046–7053.
- Xu, T., Tang, H., Chen, D., Dong, H., & Li, L. (2015). Simultaneous determination of 24 polycyclic aromatic hydrocarbons in edible oil by tandem solid-phase extraction and gas chromatography coupled/tandem mass spectrometry. Journal of AOAC International, 98, 529–537.
- Xu, X., Li, X.-G., & Sun, S.-W. (2012). A QSAR study on the biodegradation activity of PAHs in aged contaminated sediments. Chemometrics and Intelligent Laboratory Systems, 114, 50–55.
- Yagishita, M., Kageyama, S., Ohshima, S., Matsumoto, M., Aoki, Y., Goto, S., & Nakajima, D. (2015). Atmospheric concentration and carcinogenic risk of polycyclic aromatic hydrocarbons including benzo[c]fluorene, cyclopenta[c, d]pyrene, and benzo[j]fluoranthene in Japan. Atmospheric Environment, 115, 263–268.
- Yan, C., Zheng, M., Yang, Q., Zhang, Q., Qiu, X., Zhang, Y., … Zhu, Y. (2015). Commuter exposure to particulate matter and particle-bound PAHs in three transportation modes in Beijing, China. Environmental Pollution, 204, 199–206.
- Yancheshmeh, R. A., Bakhtiari, A. R., Mortazavi, S., & Savabieasfahani, M. (2014). Sediment PAH: Contrasting levels in the Caspian Sea and Anzali Wetland. Marine Pollution Bulletin, 84, 391–400.
- Yang, X., Yu, L., Chen, Z., & Xu, M. (2016). Bioavailability of Polycyclic Aromatic Hydrocarbons and their Potential Application in Eco-risk Assessment and Source Apportionment in Urban River Sediment. Scientific Reports, 6.
- Yang, Z. Z., Li, Y. F., & Fan, J. (2015). Polycyclic aromatic hydrocarbons in deposited bedroom dust collected from Xinxiang, a fast developing city in North China. Environmental Monitoring and Assessment, 187, 4150.
- Yates, K., Pollard, P., Davies, I. M., Webster, L., & Moffat, C. F. (2011). Application of silicone rubber passive samplers to investigate the bioaccumulation of PAHs by Nereis virens from marine sediments. Environmental Pollution, 159, 3351–3356.
- Yebra-Pimentel, I., Fernández-González, R., Martínez-Carballo, E., & Simal-Gándara, J. (2014). Optimization of purification processes to remove polycyclic aromatic hydrocarbons (PAHs) in polluted raw fish oils. Science of the Total Environment, 470–471, 917–924.
- Yin, X., Liang, X., Xu, G., & Zhan, X. (2014). Effect of phenanthrene uptake on membrane potential in roots of soybean, wheat and carrot. Environmental and Experimental Botany, 99, 53–58.
- Yoshimine, R. V., Carreira, R. S., Scofield, A. L., & Wagener, A. L. R. (2012). Regional assessment of PAHs contamination in SE Brazil using brown mussels (Perna perna Linnaeus 1758). Marine Pollution Bulletin, 64, 2581–2587.
- Yu, C., Yao, J., Cai, M., Yuan, H., Chen, H., & Ceccanti, B. (2014). Polycyclic aromatic hydrocarbons degrading microflora in a tropical oil-production well. Bulletin of Environmental Contamination and Toxicology, 93, 632–636.
- Yu, K., Huang, L., Lou, L. L., Chang, Y., Dong, Y., Wang, H., & Liu, S. (2015). Degradation of polycyclic aromatic hydrocarbons in crumb tyre rubber catalysed by rutile TiO2 under UV irradiation. Environmental Technology (United Kingdom), 36, 1008–1015.
- Yu, K. P., Yang, K. R., Chen, Y. C., Gong, J. Y., Chen, Y. P., Shih, H. C., & Candice Lung, S. C. (2015). Indoor air pollution from gas cooking in five Taiwanese families. Building and Environment, 93, 258–266.
- Yu, W., Liu, R., Wang, J., Xu, F., & Shen, Z. (2015). Source apportionment of PAHs in surface sediments using positive matrix factorization combined with GIS for the estuarine area of the Yangtze River, China. Chemosphere, 134, 263–271.
- Yu, W., Liu, R., Xu, F., & Shen, Z. (2015). Environmental risk assessments and spatial variations of polycyclic aromatic hydrocarbons in surface sediments in Yangtze River Estuary, China. Marine Pollution Bulletin, 100, 507–515.
- Yuan, H., Li, T., Ding, X., Zhao, G., & Ye, S. (2014). Distribution, sources and potential toxicological significance of polycyclic aromatic hydrocarbons (PAHs) in surface soils of the Yellow River Delta, China. Marine Pollution Bulletin, 83, 258–264.
- Yunker, M. B., Macdonald, R. W., Ross, P. S., Johannessen, S. C., & Dangerfield, N. (2015). Alkane and PAH provenance and potential bioavailability in coastal marine sediments subject to a gradient of anthropogenic sources in British Columbia, Canada. Organic Geochemistry, 89–90, 80–116.
- Zafra, G., & Cortés-Espinosa, D. V. (2015). Biodegradation of polycyclic aromatic hydrocarbons by Trichoderma species: a mini review. Environmental Science and Pollution Research, 22, 19426–19433.
- Zafra, G., Moreno-Montaño, A., Absalón, Á., & Cortés-Espinosa, D. V. (2015). Degradation of polycyclic aromatic hydrocarbons in soil by a tolerant strain of Trichoderma asperellum. Environmental science and pollution research international, 22, 1034–1042.
- Zamani, J., Hajabbasi, M. A., Alaie, E., Sepehri, M., Leuchtmann, A., & Schulin, R. (2016). The effect of Piriformospora indica on the root development of maize (Zea mays L.) and remediation of petroleum contaminated soil. International Journal of Phytoremediation, 18, 278–287.
- Zander, M. (1983). Physical and chemical properties of polycyclic aromatic hydrocarbons. New York, NY: Marcel Dekker Inc.
- Zena, R., Speciale, A., Calabrò, C., Calò, M., Palombieri, D., Saija, A., … Lo Cascio, P. (2015). Exposure of sea bream (Sparus aurata) to toxic concentrations of benzo[a]pyrene: Possible human health effect. Ecotoxicology and Environmental Safety, 122, 116–125.
- Zeng, J., Zhu, Q., Wu, Y., & Lin, X. (2016). Oxidation of polycyclic aromatic hydrocarbons using Bacillus subtilis CotA with high laccase activity and copper independence. Chemosphere, 148, 1–7.
- Zhai, Y., Li, P., Zhu, Y., Xu, B., Peng, C., Wang, T., … Zeng, G. (2016). Source apportionment coupled with gas/particle partitioning theory and risk assessment of polycyclic aromatic hydrocarbons associated with size-segregated airborne particulate matter. Water, Air, and Soil Pollution, 227.
- Zhang, D., Liu, J., Jiang, X., Cao, K., Yin, P., & Zhang, X. (2016). Distribution, sources and ecological risk assessment of PAHs in surface sediments from the Luan River Estuary, China. Marine Pollution Bulletin, 102, 223–229.
- Zhang, F., Zhang, R., Guan, M., Shu, Y., Shen, L., Chen, X., & Li, T. (2016). Polycyclic aromatic hydrocarbons (PAHs) and Pb isotopic ratios in a sediment core from Shilianghe Reservoir, eastern China: Implying pollution sources. Applied Geochemistry, 66, 140–148.
- Zhang, G., Pan, Z., Wang, X., Mo, X., & Li, X. (2015). Distribution and accumulation of polycyclic aromatic hydrocarbons (PAHs) in the food web of Nansi Lake, China. Environmental monitoring and assessment, 187, 173.
- Zhang, G., Zang, X., Li, Z., Wang, C., & Wang, Z. (2014). Polydimethylsiloxane/metal-organic frameworks coated fiber for solid-phase microextraction of polycyclic aromatic hydrocarbons in river and lake water samples. Talanta, 129, 600–605.
- Zhang, H., Xu, L., Zhang, Y., & Jiang, M. (2016). The transformation of PAHs in the sewage sludge incineration treatment. Frontiers of Environmental Science and Engineering, 10, 336–340.
- Zhang, J., Liu, G., Wang, R., & Liu, J. (2014). Concentrations and sources of polycyclic aromatic hydrocarbons in water and sediments from the Huaihe River, China. Analytical Letters, 47, 2294–2305.
- Zhang, J., Liu, G., Wang, R., & Liu, J. (2015). Distribution and source apportionment of polycyclic aromatic hydrocarbons in bank soils and river sediments from the Middle Reaches of the Huaihe River, China. Clean–Soil, Air, Water, 43, 1207–1214.
- Zhang, Y., Cui, B., Zhang, Q., & Liu, X. (2015). Polycyclic aromatic hydrocarbons in the food web of Coastal Wetlands: Distribution, sources and potential toxicity. Clean - Soil, Air, Water, 43, 881–891.
- Zhang, Y., McPhedran, K. N., & Gamal El-Din, M. (2015). Pseudomonads biodegradation of aromatic compounds in oil sands process-affected water. Science of the Total Environment, 521–522, 59–67.
- Zhang, Y., Yu, H., Xi, B., Hou, H., Gao, R., Liang, Q., … Xu, F. (2015). Levels, sources, and potential ecological risks of polycyclic aromatic hydrocarbons (PAHs) in a typical effluent-receiving river (Wangyang River), North China. Arabian Journal of Geosciences, 8, 6535–6543.
- Zhang, Y. N., Yang, X. L., Bian, Y. R., Gu, C. G., Liu, Z. T., Li, J., … Jiang, X. (2015). Aging law of PAHs in contaminated soil and their enrichment in earthworms characterized by chemical extraction techniques. Huanjing Kexue/Environmental Science, 36, 4582–4590.
- Zhang, Z., Rengel, Z., Chang, H., Meney, K., Pantelic, L., & Tomanovic, R. (2012). Phytoremediation potential of Juncus subsecundus in soils contaminated with cadmium and polynuclear aromatic hydrocarbons (PAHs). Geoderma, 175–176, 1–8.
- Zhang, Z. H., & Balasubramanian, R. (2016). Investigation of particulate emission characteristics of a diesel engine fueled with higher alcohols/biodiesel blends. Applied Energy, 163, 71–80.
- Zhao, X., Cai, Z., Wang, T., O’Reilly, S. E., Liu, W., & Zhao, D. (2016). A new type of cobalt-deposited titanate nanotubes for enhanced photocatalytic degradation of phenanthrene. Applied Catalysis B: Environmental, 187, 134–143.
- Zhao, X., Kim, S. K., Zhu, W., Kannan, N., & Li, D. (2015). Long-range atmospheric transport and the distribution of polycyclic aromatic hydrocarbons in Changbai Mountain. Chemosphere, 119, 289–294.
- Zhao, X., Zhu, W., Huang, J., Li, M., & Gong, M. (2015). Emission characteristics of PCDD/Fs, PAHs and PCBs during the combustion of sludge-coal water slurry. Journal of the Energy Institute, 88, 105–111.
- Zhao, Y., Cao, L., Zhou, Q., Que, Q., & Hong, B. (2015). Effects of oil pipeline explosion on ambient particulate matter and their associated polycyclic aromatic hydrocarbons. Environmental Pollution, 196, 440–449.
- Zhao, Z., Zhang, L., Cai, Y., & Chen, Y. (2014). Distribution of polycyclic aromatic hydrocarbon (PAH) residues in several tissues of edible fishes from the largest freshwater lake in China, Poyang Lake, and associated human health risk assessment. Ecotoxicology and Environmental Safety, 104, 323–331.
- Zheng, H., Xing, X. L., Gu, Y. S., Gui, F. K., Qi, S. H., & Huang, H. F. (2016). Distribution characteristics of polycyclic aromatic hydrocarbons in different environmental media from Qingbang Island, Zhoushan, China. Huanjing Kexue/Environmental Science, 37, 513–519.
- Zheng, H. B., Ding, J., Zheng, S. J., Zhu, G. T., Yuan, B. F., & Feng, Y. Q. (2016). Facile synthesis of magnetic carbon nitride nanosheets and its application in magnetic solid phase extraction for polycyclic aromatic hydrocarbons in edible oil samples. Talanta, 148, 46–53.
- Zheng, T., Ran, Y., & Chen, L. (2014). Polycyclic aromatic hydrocarbons (PAHs) in rural soils of Dongjiang River Basin: Occurrence, source apportionment, and potential human health risk. Journal of Soils and Sediments, 14, 110–120.
- Zheng, X., Han, B., Thavamani, P., Duan, L., & Naidu, R. (2015). Composition, source identification and ecological risk assessment of polycyclic aromatic hydrocarbons in surface sediments of the Subei Grand Canal, China. Environmental Earth Sciences, 74, 2669–2677.
- Zheng, Y., Luo, X., Zhang, W., Wu, B., Han, F., Lin, Z., & Wang, X. (2012). Enrichment behavior and transport mechanism of soil-bound PAHs during rainfall-runoff events. Environmental Pollution, 171, 85–92.
- Zhi, H., Zhao, Z., & Zhang, L. (2015). The fate of polycyclic aromatic hydrocarbons (PAHs) and organochlorine pesticides (OCPs) in water from Poyang Lake, the largest freshwater lake in China. Chemosphere, 119, 1134–1140.
- Zhong, Y., Carmella, S. G., Upadhyaya, P., Hochalter, J. B., Rauch, D., Oliver, A., … Hecht, S. S. (2011). Immediate consequences of cigarette smoking: Rapid formation of polycyclic aromatic hydrocarbon diol epoxides. Chemical Research in Toxicology, 24, 246–252.
- Zhou, H., Wang, H., Huang, Y., & Fang, T. (2016). Characterization of pyrene degradation by halophilic Thalassospira sp. strain TSL5-1 isolated from the coastal soil of Yellow Sea, China. International Biodeterioration and Biodegradation, 107, 62–69.
- Zhou, H. W., Luan, T. G., Zou, F., & Tam, N. F. Y. (2008). Different bacterial groups for biodegradation of three- and four-ring PAHs isolated from a Hong Kong mangrove sediment. Journal of Hazardous Materials, 152, 1179–1185.
- Zhou, J. L., Siddiqui, E., Ngo, H. H., & Guo, W. (2014). Estimation of uncertainty in the sampling and analysis of polychlorinated biphenyls and polycyclic aromatic hydrocarbons from contaminated soil in Brighton, UK. Science of the Total Environment, 497, 163–171.
- Zhou, Q., & Gao, Y. (2014). Determination of polycyclic aromatic hydrocarbons in water samples by temperature-controlled ionic liquid dispersive liquid-liquid microextraction combined with high performance liquid chromatography. Analytical Methods, 6, 2553–2559.
- Zhu, L., & Wang, J. (2003). Sources and patterns of polycyclic aromatic hydrocarbons pollution in kitchen air, China. Chemosphere, 50, 611–618.
- Ziegenhals, K., Jira, W., & Speer, K. (2008). Polycyclic aromatic hydrocarbons (PAH) in various types of tea. European Food Research and Technology, 2u28, 83–91.
- Zielińska, A., & Oleszczuk, P. (2015). The conversion of sewage sludge into biochar reduces polycyclic aromatic hydrocarbon content and ecotoxicity but increases trace metal content. Biomassand Bioenergy, 75, 235–244.
- Zongmao, C., & Haibin, W. (1997). Degradation of pesticides on plant surfaces and its prediction - A case study on tea plant. Environmental Monitoring and Assessment, 44, 303–313.
- Zuin, V. G., Montero, L., Bauer, C., & Popp, P. (2005). Stir bar sorptive extraction and high-performance liquid chromatography-fluorescence detection for the determination of polycyclic aromatic hydrocarbons in Mate teas. Journal of Chromatography A, 1091, 2–10.