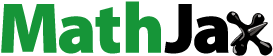
Abstract
The distribution, and ecological risks due to toxic metals in topsoils of an urban commercial centre of Kumasi metropolis were determined. Concentrations of heavy metals in the samples were initially screened using field portable X-ray fluorescence spectrometer prior to confirmation on an inductively coupled plasma-mass spectrometer. The results from the two instruments correlated well with high linearity (R2 > 0.70). Concentrations of metals were in the order: Fe (45246.15 mg/kg) > Mn (347.86 mg/kg) > Zn (176.40 mg/kg) > V (173.10 mg/kg) > Cr (125.12 mg/kg) > Pb (53.47 mg/kg) > Ni (43.16 mg/kg) > Cu (39.85 mg/kg) > As (4.59 mg/kg) > Sn (3.69 mg/kg) > Cd (0.46 mg/kg) > Hg (0.28 mg/kg). Multivariate and geostatistical analyses exploring various hazard indices such as geo-accumulation, contamination, pollution load and ecological risks all suggest contamination of the topsoils with toxic metals and that the contamination due to Pb, Cu, Ni, Cr and Zn is mainly derived from anthropogenic origins whereas, As, Hg, Mn, V, Sn and Cd contaminations are attributable mainly to geological and atmospheric depositions. Contamination factor (CF) suggests that the topsoils were considerably contaminated (CF 3–6) with Zn, moderately contaminated (CF 1–3) with Ni, Hg, V, Sn and Cd and least contaminated (CF < 1) with As, Pb, Cu, Cr and Mn. In general, the soils were found to be moderately contaminated (average contamination factor (1 ≤ CF < 3) with toxic metals and may pose both human and ecological risks.
Public Interest Statement
Some of the metals in the topsoils in urban and industrial settings could be poisonous to humans and animals who come into contact with them. Most of these metals build up in the upper layers of the soil as result of human activities. In this work, standard methods were used to measure how much of those poisonous metals we have in topsoils from the industrial commercial hub of Kumasi, the second largest city in Ghana. Nine out of the 12 metals measured were found to be higher than the world average levels. It was also found that some of the metals have built up high enough in the top soil to be able to cause risks to the people who may come in contact with them.
1. Introduction
Metals, usually present in varying concentrations in urban topsoils, may originate either from natural sources (through lithogenesis, pedogenesis) or from anthropogenic sources (including industrial activities) such as metal processing, combustion of fossil fuel, and mining (Luo, Yu, & Li, Citation2012; Rodríguez Martín, Arias, & Grau Corbí, Citation2006). Unmonitored industrial and human activities generally contribute significantly to the levels of metal contaminants in the top layers of the soil relative to contributions from natural sources. Toxic metals such as arsenic, cadmium, mercury and lead are mainly introduced into the topsoils of urban settings through human activities (Adelekan & Alawode, Citation2001; Bak, Jensen, Larsen, Pritzl, & Scott-Fordsmand, Citation1997) and could potentially pose a threat to the ecological environment as well as human health (Glennon, Harris, Ottesen, Scanlon, & O’Connor, Citation2014; Wong, Li, & Thornton, Citation2006).
As the metals slowly accumulate and distribute in the soil profiles over time, the soil can act as a long-term sink for these toxic metals (Darko et al., Citation2015; Pakade et al., Citation2012) which can affect the health of living organisms and the environment. Topsoil could be eroded into surface water bodies. If the soils are contaminated, this would affect the aquatic ecosystem in various ways. In addition, suspension of contaminated soil particles could also affect air quality in the area. Metals become toxic to organisms when the metal bioaccessible concentration in an organism exceeds the concentration that could be tolerated (Darko, Dodd, Nkansah, Ansah, & Aduse-Poku, Citation2017), resulting in impaired growth, loss of function of an organ or the organism’s life. In general, heavy metal toxicity may affect one or more internal organs or organnelles or an entire system of a living organism (Kapustka et al., Citation2004). The site of toxic action of the metal could be an enzyme, a membrane, or a co-factor that is critically needed in some biochemical pathway. For plants, toxicity may also occur at the root surface without the metal actually entering the internal portions of the plant (Ducic & Polle, Citation2005). For micro-organisms, the toxic effects of the metals may occur at the surface of their external membranes. Metals may also disrupt extracellular enzyme function.
Distribution of metals in the environment is influenced by a number of factors, with the forms in which the metals exist (either in inorganic form as ions and salts or in the organic form as combined compounds) being a major determinant. The stability of the form in which a metal exists also contributes to its distribution in the environment (Caussy, Gochfeld, Gurzau, Neagu, & Ruedel, Citation2003). The topsoils tend to accumulate higher concentrations of metals derived from human activity than other soil horizons due to atmospheric deposition and the organic matter in the topsoil which traps the metals. Urban dwellers can be exposed to the metal contaminants in the topsoils in gardens, playgrounds, market places, streets and sandy sports fields through inhalation of dust, dermal contact or incidental ingestion.
High concentrations of metals in the environment have been associated with developmental, cardiovascular, reproductive, neurological, ocular, haematological and renal ailments (ASTDR, Citation2012). In addition, exposure to metal contaminants have been associated with serious health effects such as long-term memory activity disorders and damage to DNA in humans (Wu et al., Citation2015). It is therefore necessary to quantify the degree of metal contamination in urban soils so as to assess the potential risks they pose to human and environmental health. In this study, concentrations of toxic metals in the topsoils of the urban areas of the Kumasi metropolis were determined and the environmental pollution risks estimated using various hazard indices based on the concentrations of toxic metals present. The study also assessed the suitability of a field portable X-ray fluorescence (FP-XRF) spectrometer as a quick and relatively inexpensive tool for monitoring heavy metals in the soils by comparing the results from the FP-XRF and the inductively coupled plasma-mass spectrometer (ICP-MS).
2. Materials and methods
2.1. Study area
Kumasi metropolis lies between latitudes 6.35–6.40o and longitude 1.30–1.37o, with an area coverage of about 299 km2. Kumasi features a tropical wet and dry climate, a relatively constant temperature throughout the year and two different rainy seasons: a longer rainy season from March through July and a shorter rainy season from September to November. The annual average rainfall in the area is around 1,400 mm (Akoto, Darko, & Nkansah, Citation2011). Kumasi experiences the harmattan weather during the dry season, from December to February.
Geologically, the metropolis falls within the Kumasi Basin of the Birimian formation and consists of three main lithological units. These are the thin upper oxide haematite layer with a thickness in the range of 0.1–0.3 m, overlying a 5–30 m thick layer of saprolite, which is underlain by a batholitic basin granitoid which are mostly unaltered (Chudasama, Porwal, Kreuzer, & Butera, Citation2016). The outcrops of the K-feldspars rich granitic rocks can be seen in most parts of the metropolis (Losiak, Schulz, Buchwaldt, & Koeberl, Citation2013). The topography of the area is in the range of 250 to 330 m above mean sea level with the highest topography region occurring mainly in the northern section of the area and the lower topographic region occurring at the south (Frempong, Citation1994; Miller, Partington, & Kreuzer, Citation2015). This topographic trend generally controls the stream floor in the metropolis with most rivers and streams flowing in the southern direction.
The Kumasi metropolis is predominantly a commerce/trade centre with trade and commerce employing about 71% of the population followed by industry and agriculture with an employment level of 24 and 5%, respectively (Engelhardt, Citation2012; Nkansah, Korankye, Darko, & Dodd, Citation2016). Kumasi Central Market (also known as Kejetia Market) housing over 45,000 stores is the continent’s largest open air market (Baker, Citation2008) while the Suame Magazine, a light industrial setting with many workshops for metal engineering, fabrication and vehicle repairs, is the largest artisanal auto mechanic hub in the West African sub region (Baker, Citation2008; Iddrisu, Mano, & Sonobe, Citation2012). Due to the presence of numerous commercial and industrial activities in Kumasi, there is the likelihood of anthropogenic contamination of heavy metals in the topsoil.
2.2. Soil sampling
Topsoil samples (0–5 cm depth) were collected across the commercial hub of the metropolis. Sampling points marked on a 0.5 × 0.5 km2 grid net map of the city (Figure ) were identified using a hand-held Garmin Map 6 global positioning system. The points of intersection of the gridlines on the map were selected as the specific sampling points. About 0.5 kg of soils samples were collected from the surface into plastic Ziploc bags using plastic ladles. Duplicate samples were taken at each fifth point. For points where soil sampling were not possible due to interference such as tarred roads, rivers and houses, the locations were shifted to the nearest accessible points within the same sample grid net. In all 107 soil samples were taken during the sampling campaign which span between November and December, 2015.
2.3. Sample preparation and analysis
The soil samples were air-dried and sieved to <250 μm mesh size using USA Standard Testing Sieve ASTM E11 and then stored in coded polyethylene bags. Samples were screened using a Niton XL3t GOLD field portable X-ray fluorescence (FP-XRF) spectrometer following the United States Environmental Protection Agency Method 6200 (US EPA, Citation2007) and and confirmatory analysis by ICP-MS. A system check of the XRF analyzer was completed and a NIST 2709a check sample was ran at the beginning of each working day prior to the analysis of the soil samples. Each sample analysis was performed in triplicate and the average of the readings computed.
For the ICP-MS analysis, the sieved samples were digested using modified aqua regia and analysed based on USEPA Method 200.8 (US EPA, Citation2015). Briefly, 3 mL of 1:1:1 HCl-HNO3-H2O mixture was added to 0.5 g sample and the mixture digested at 95 °C for 1 h in a heating block. The sample was allowed to cool and made to volume with dilute 1 M HCl followed by instrumental analysis.
The soil pH and EC were determined using a multi-parameter probe (Oakton Waterproof Multiparameter PCSTestr35). The pH and EC analysis was performed by mixing 20 g of the dry soil in 40 mL of distilled water (that is 1:2 ratio of dry soil to distilled water). The suspension of water and soil particles was allowed to settle for 1 h following which the pH and EC of the supernatant liquid was then determined.
2.4. Statistical analysis
Descriptive statistical analysis (minimum, maximum and mean) was conducted using SPSS 20.0 software. The inter-relationship between metal concentrations and different soil characteristics was determined through Pearson correlation analysis while principal component analysis (PCA) using varimax rotation was used to explore the possible sources of metals. Eigen values > 1 were only considered for interpreting the results.
2.5. Estimation of level of contamination
The extent of metal contamination can be evaluated by either comparing site specific data to background reference data, or through the use of pollution indices and enrichment factors (Qing, Yutong, & Shenggao, Citation2015; Wong et al., Citation2006). In this work, contamination was measured by the use of enrichment and contamination factors, pollution load and potential ecological risk indices.
2.6. Enrichment Factor
Enrichment factor (EF) was used to assess the degree of heavy metal pollution from anthropogenic sources as well as differentiate between anthropogenic and naturally occurring sources (Swarnalatha, Letha, Ayoob, & Nair, Citation2015). Concentration of Fe was used as the normalisation reference (Abrahim & Parker, Citation2008). The EF was calculated using Equation (1).
(1)
(1)
Where Mx and Fex are metal and Fe concentrations in the soil sample, respectively, Mr and Fer are the concentrations of the metal and Fe in a reference material. An EF>1 means the metal concentration in the soil sample is enriched relative to the average continental crust and surface soil values and the source of the metal in the topsoil is likely to be anthropogenic. EF<1 indicates that the metal concentration is not enriched and may be from natural source. EF = 1 indicates that the metal concentration and its reference value are the same (Swarnalatha et al., Citation2015).
2.7. Contamination factor
Contamination factor (CF) which is the ratio of the concentration of a metal in the soil sample to that in the background (Hakanson, Citation1980) was used to assess the extent of metal contamination. The CF was calculated using Equation (2).(2)
(2)
where Mx and Mr are the mean concentrations of the metal contaminants in the soil samples and background reference material, respectively (Chen, Teng, Lu, Wang, & Wang, Citation2015).
The degree of contamination (Cd) which is based on the CF of the pollutant was used to describe the extent of contamination of a metal contaminant (Swarnalatha et al., Citation2015) employing Equation (3) .(3)
(3)
Where n is number of analysed elements and i is ith element, CF is contamination factor.
2.8. Pollution load index
To estimate the overall pollution status of the samples, the pollution load index (PLI) of the metal contaminants was calculated using Equation (4) (Chen et al., Citation2015; Qing et al., Citation2015).(4)
(4)
Where CF represents the contamination factor of a metal, n represents a specific metal’s contamination factor. The PLI values are used to classify samples as; unpolluted (PLI ≤ 1), moderately polluted (PLI = 1–3), highly polluted (PLI = 3–5) or very highly polluted (PLI>5) (Qing et al., Citation2015).
2.9. Potential Ecological Risk
The potential ecological risk index (PERI) was used to assess the degree of heavy metal pollution in soils based on the contamination factor of heavy metals (CF) and the response of the environment to the contaminant (Trf). The PERI was calculated as the sum of individual risk indices (RI) (Hakanson, Citation1980) as follows:(5)
(5)
Toxic response factor for metals are in the order; Zn = 1, Cr = 2, Co = Cu = Pb = 5, Ni = 6, As = 10, Cd = 30 and Hg = 40. The degree of ecological risk can be classified as; PERI <40 (low risk), 40 ≤ PERI <80 (moderate risk), 80 ≤ PERI <160 (considerable risk), 160 ≤ PERI <320 (high risk), and PERI ≥ 320 (very high risk) (Qing et al., Citation2015; Wu et al., Citation2015).
2.10. Soil pollution index
Soil pollution index (SPI), a simple well-known pollution evaluation tool was used to identify single-element contamination indices. SPI was calculated using Equation (7):(6)
(6)
The tolerable levels of heavy metals for soil were adopted as permissible levels (Kabata-Pendias & Pendias, Citation2001a, Citation2001b) and the level of each heavy metal present was classified as low contamination (SPI ≤ 1), moderate contamination (1 < SPI ≤ 3) or high contamination (SPI > 3) (Chen et al., Citation2005).
3. Results and discussion
3.1. Physicochemical Parameters
The results of soil pH and electrical conductivity are shown in Table . The soil pH ranged from 3.87 to 8.72 with a mean of 7.59 ± 1.02. Quantitatively, 17% of the soil samples were acidic with pH <7. Acidic soils were found in samples collected from locations close to or within heavy industrial activity areas, mainly automobile repair shops and lorry stations. Soil pH has strong effects on the solubility and retention of metals in the soil; greater retention and lower solubility of a metal occur at high soil pH (Smith, Citation1995). The soil pH at most of the sampled points were in the pH range for natural soils which is usually 4.0–9.0. The electrical conductivity of the soil ranged from 127.7 to 6,082.0 μS/cm with an average value of 935.0 μS/cm which indicates that the soils probably have high levels of inorganic ions including metals.
Table 1. Regression parameters for comparability
3.2. Comparison between ICP-MS and FP-XRF data
A comparison of the Field Portable X-ray Fluorescence (FP-XRF) data to the ICP-MS results showed a strong linear correlation between the two methods for the majority of the metals (Figure ). The Pearson Correlation coefficients (p <0.001) for As, Cu, Pb, and Zn were 0.985, 0.894, 0.879 and 0.985, respectively. The coefficient of determination (R2), the y-intercept and the slope of the line for the linear regression analysis are given in Table . The R2 values for Cr, Cu, Fe, Pb and Zn were between 0.773 and 0.978 indicating excellent comparability of the confirmatory laboratory data to the XRF data (US EPA, Citation2007). The slopes of the regression lines for As, Cr, Cu, Fe, Pb, V and Zn were between 0.7 and 1.11 suggesting that very little data correction would be needed to match the FP-XRF data to confirmatory laboratory data (Al Maliki, Al-lami, Hussain, & Al-Ansari, Citation2017). Correction of the FP-XRF data would be required in order to match the confirmatory laboratory data for Mn due the low R2 value of 0.449 and slope of 0.522 while the R2 value and the slope for Ni indicated very poor comparability between the FP-XRF and the laboratory ICP-MS data. The detection limit of Ni for the FP-XRF (18 mg/kg) was very high compared to that of the ICP-MS (0.1 mg/kg) which may accounted for the poor comparability. Overall the data obtained in this study shows that the FP-XRF is a useful analytical instrument for screening a large number of samples of soil for most metals prior to the use of a more sensitive analytical instrument in the laboratory.
3.3. Heavy metal concentrations
Concentrations of heavy metals (As, Pb, Cu, Ni, Cr, Hg. Zn, Fe, Mn, V, Sn and Cd) in the topsoil of urban areas in Kumasi metropolis at different sampling points, descriptive statistics, the Dutch and Canadian guidelines are summarised in Table . Arsenic content ranged from 2.73 to 36.00 mg/kg. High concentrations of As in Kumasi soils could be due to anthropogenic sources from activities associated with chemical and metallurgical industries. The Cr content ranged from 16.35 to 649.53 mg/kg. High Cr concentrations have also been reported in the Kanpur-Unnao Industrial Region of the Ganga Plain, India (Ansari, Singh, & Tobschall, Citation1999) where the industrial activities have been known to influence Cr content in the environmental samples analysed. The auto mechanic and other shops dealing with steel and metal plating in the area could serve as possible source for the metal. The concentrations of Cu found were between 10.75 and 307.88 mg/kg. The concentration of Cu generally in non-contaminated soils is 50 mg/kg (Ansari et al., Citation1999). The high concentrations of Cu in some pockets of the study area may be due to input from local industrial/garages dotted around. Lead content in the soils ranged from 3.72 to 268.35 mg/kg. Ghana does not use leaded-fuel or lead pipes and Pb in paints is also regulated. It is therefore speculated that these high levels of Pb might be emanating from human activities such as metal scrap recycling which is rampant in the study area or from the bedrock. Nickel content in the soils ranged from 19.10 to 102.41 mg/kg. The high Ni content in the soil samples could be attributed to electroplating and other anthropogenic activities such as burning of fuel which contribute to the increase in Ni content in the soil of the study area (Al Obaidy & Al Mashhadi, Citation2013). Zn content ranged from 23.21 to 783.44 mg/kg. Comparing the metal concentrations obtained from this study to international guidelines for unpolluted soils revealed that the concentrations all the analysed metals were higher than the international average concentrations of unpolluted soils except for As which recorded a lower mean value (4.59 mg/kg) as against the CCME standard of 10 mg/kg.
Table 2. Descriptive statistics of metal concentration (mg/kg) using an ICP-MS and physicochemical parameters (n = 107)
Figure shows the spatial distributions of the metals in the study area. There was a generally high concentration of metals in the northern part of the metropolis. The northern part covers the catchment of Suame artisanal industrial area, the largest artisanal village in the West African sub-region. The area has high activities of vehicle repair shops, metal fabrication, welding, blacksmith, car spraying and painting shops, and other artisanal activities. Most of the activities that go on at the artisanal area are carried out without consideration to its effect on the environment (Baker, Citation2008; Iddrisu et al., Citation2012). Spilling of used oil from automobiles on the ground, filing of metals and spraying of cars in the open are some of the common practices. The high concentration of metals in the area could therefore be attributed to the artisanal activities that happen there. Another hotspot of metal contamination was found at the central business district which includes Kejetia (the largest market and lorry station in the metropolis), Asafo and Abinkyi markets as well as the general stores and numerous other commercial activities in the Adum area.
3.4. Comparison with other cities
Table shows concentrations of heavy metals found in urban topsoils in some cities as well as the world average concentrations of heavy metals in soils. Except for As, the concentrations of all other metals studied (Hg, Mn, Pb, Cu, Ni, Cr, Zn, V, Sn and Cd) were higher than the world average. Compared with a similar work (Odewande & Abimbola, Citation2008) in another West African city, Ibadan City in Nigeria- noted for industrial and commercial activities similar to the Kumasi study area, the concentrations of Pb, Cu, Zn, Mn and Cd found in Kumasi, Ghana, were lower. However, those of As, Ni and Cr were higher in Kumasi than in Ibadan. The concentrations of metals found in the topsoils of the industrial city of Ghaziabad in India with similar commercial activities like the study area (Chabukdhara & Nema, Citation2013) were 1.1 to 3.1 folds higher than those found in Kumasi.
Table 3. Concentrations of heavy metals in urban topsoils elsewhere
3.5. Correlation analysis
Correlation analyses have been widely applied in environmental studies. They provide an effective way to reveal the relationships between multiple variables and thus have been helpful for understanding the influencing factors (pH, weathering of rocks, filing of metals, etc.) as well as the sources of chemical components. Heavy metals in soil usually have complicated relationships among them. The high correlations between heavy metals in soil may reflect that the accumulated concentrations of these heavy metals come from similar pollution sources e.g. industrial and commercial activities in the urban area. A high and significant positive correlation (p <0.01) was observed among some of the metals. The highest correlations were found between Mn and Fe (r = 0.809); Cr and Fe (r = 0.744); Cr and Mn (r = 0.705); Ni and Cr (r = 0.688); Ni and Fe (r = 0.686); Pb and Zn (r = 0.735). The strong positive correlations among Cr, Fe, Mn, Ni, Pb and Zn suggest that they are probably released from a similar origin.
3.6. Principal component analysis
Principal component analysis was used to explore the extent of metal pollution and their source as predicted by the correlation analysis following standard procedures (Dragović, Mihailović, & Gajić, Citation2008; Franco-Uría, López-Mateo, Roca, & Fernández-Marcos, Citation2009). Varimax rotation was used to maximise the sum of the variance of the factor coefficients which better explained the possible groups or sources that influence the soil system (Gotelli & Ellinson, Citation2013). Components loadings were classified as “strong”, “moderate”, and “weak” corresponding to absolute loading values of > 0.75, 0.75–0.50, and 0.50–0.30, respectively (Liu, Lin, & Kuo, Citation2003).
Factor loadings for heavy metals are shown in Table . Based on eigenvalue >1, 3 principal components (PC1, PC2, and PC3) cumulatively explained 58.79% of the total variance. The PC1 explained 29.34% of total variance and was found to be strongly and positively correlated to Fe, V, Cr and Ni (0.85–0.92) indicating an anthropogenic source. This relates to the artisanal activities and metal processing works in the area and shows the pollution by this source; PC2 explained 17.98% of total variance and showed high positive factor loadings for Pb, Zn, Cu, As and Mn (0.61–0.87) which indicate industrial source and activities of scrap metal dealers in the study area. This shows the pollution from industrial activities which deal with steel, metal smelting and recycling of metals as well as lead-acid accumulators and vehicle emissions in urban areas; PC3 explained 11.47% of total variance and showed high positive factor loadings for Cd and Hg (0.79–0.86) which could be attributed to atmospheric deposition and waste incineration as there are no gold mining sites within the area which makes use of Hg also cadmium could originate from the activities in the artisanal hub.
Table 4. Matrix of principal component analysis using varimax rotation for heavy metals concentrations in topsoils
3.7. Assessment of the heavy metal contamination
The assessment of soil contamination was also done using the contamination factor, pollution load index and risk indices (Hakanson, Citation1980). Table shows the summary of the various soil pollution assessment tools employed. Average pollution load index recorded for the study area was 0.34 and the average modified degree was 0.46. On the basis of average CF, the soil was classified as least contaminated with As, Pb, Cu, Cr and Mn, moderately contaminated with Ni, Hg, V, Sn and Cd, and considerably contaminated with Zn. Single element pollution index (SPI) revealed that the urban soils were ranging from low contamination to moderate contamination with the analysed metals in the order: Cr > V > Ni > Zn > Cu > As > Pb > Cd > Hg.
Table 5. Mean enrichment factor (EF), mean contamination factor (CF) of metals in Kumasi and mean risk index (RI)
In order to get a more accurate assessment of heavy metal pollution in terms of ecological risks, potential ecological risk indices (PERI) were determined. Potential ecological risk indices represent the overall contamination (Hakanson, Citation1980). The metals were found to exhibit significant increase in their risk indices at the various sampling points ranging from low risk to high risk in the order; Hg > Cd > Ni > Zn > Cu > As > Pb > Cr. The PERI values ranged from 0.24 to 4388 in the samples with an average of 128 ± 56 which indicates considerable ecological risk. About 39.3% of the sampled points recorded a low ecological risk and the remaining 60.7% (HR, Figure ) recorded a very high ecological risk with respect to the metals. The high PERI values indicate risk to the environment.
4. Conclusion
Topsoils in the commercial/industrial hub of the Kumasi metropolis has been assessed for heavy metals in this study and have been found to contain significant concentrations of heavy metals (lead, chromium, zinc, copper, arsenic, nickel, cobalt, cadmium and mercury), with most of the metal concentrations exceeding the world soil average concentrations, the Dutch target of heavy metals in soils and the Canadian Council of Ministers of the Environment guidelines. The metals detected were found to be evenly distributed in the study area with a slightly higher concentration found at the northern part where Suame magazine (the largest artisanal engineering cluster in sub-region) is located. Accumulation profiles and correlation analysis of the metals revealed strong correlations between the metals and also showed that the metals were generally of anthropogenic origin. Due to the relatively high concentrations of the metals, risk indices revealed that the toxic metals have moderate human health implications on urban dwellers and could also potentially impact the ecosystem. A very strong correlation was found between the results obtained from FPXRF and ICP-MS. Therefore, the FP-XRF could be a good instrument for fast measurements of heavy metals in soils from the metropolis. An oral bioaccessibility study of these metals is suggested to help quantify the risk level of these metals to humans to know how much of the total concentration of metal is ingested and their effect on the human system.
Additional information
Funding
Notes on contributors
Godfred Darko
Godfred Darko is a senior lecturer in Chemistry with research interests covering environmental chemistry, analytical chemistry and nano-chemistry. He currently runs the SHEATHE project (www.sheathe.org) which seeks to characterise xenobiotic substances and heavy metals on the local and national scales in Ghana.
Matt Dodd
Matt Dodd is an environmental analytical chemist with research interests in metal speciation and bioaccessibility, soil toxicity testing and persistent organic pollutants.
Marian Asantewah Nkansah
Marian Asantewah Nkansah is an environmental analytical chemist with interest in environmental problems associated with levels and fate of toxic substances in the environment and foodstuffs.
Yaw Aduse-Poku
Yaw Aduse-Poku and Eugene Ansah are MPhil and PhD candidates respectively.
David Dotse Wemegah
David Dotse Wemega is a lecturer in the in Physics with interest in hydrogeophysics.
Lawrence Sheringham Borquaye
Lawrence Sheringham Borquaye is a bioorganic chemist with interest on science at the interface of chemistry and biology.
All the authors collaborate in the SHEATHE Project.
References
- Abrahim, G. M. S., & Parker, R. J. (2008). Assessment of heavy metal enrichment factors and the degree of contamination in marine sediments from Tamaki Estuary, Auckland, New Zealand. Environmental Monitoring and Assessment, 136, 227–238. doi:10.1007/s10661-007-9678-2
- Adelekan, B. A., & Alawode, A. O. (2001). Contribution of municipal refuse dumps to heavy metal concentration in soil profile and groundwater in Ibadan, Nigeria. Journal of Applied Bioscience, 40, 2727–2737.
- Akoto, O., Darko, G., & Nkansah, M. A. (2011). Chemical composition of rainwater over a mining area in Ghana. Internationall Journal of Environmental Research, 5, 847–854.
- Al Maliki, A., Al-lami, A. K., Hussain, H. M., & Al-Ansari, N. (2017). Comparison between inductively coupled plasma and X-ray fluorescence performance for Pb analysis in environmental soil samples. Environmental Earth Sciences, 76, 433. doi:10.1007/s12665-017-6753-z
- Al Obaidy, A. H. M. J., & Al Mashhadi, A. A. M. (2013). Heavy metal contaminations in urban soil within Baghdad City, Iraq. Journal of Environmental Protection, 4, 72–82. doi:10.4236/jep.2013.41008
- Ansari, A. A., Singh, I. B., & Tobschall, H. J. (1999). Status of anthropogenically induced metal pollution in the Kanpur-Unnao industrial region of the Ganga Plain, India. Environmental Geology, 38, 25–33. doi:10.1007/s002540050397
- ASTDR. (2012). Agency for toxic substances and disease registry. Toxicological Profile for Chromium, (September), 592. Retrieved from http://www.atsdr.cdc.gov/toxprofiles/tp7.pdf
- Bak, J., Jensen, J., Larsen, M. M., Pritzl, G., & Scott-Fordsmand, J. (1997). A heavy metal monitoring-programme in Denmark. Science of The Total Environment, 207, 179–186. doi:10.1016/S0048-9697(97)00262-3
- Baker, G. (2008). Assessing infrastructure constraints on business activity in Kumasi, Ghana (03/2008) (Vol. 3). New York, NY. Retrieved from http://academiccommons.columbia.edu/catalog/ac:125864
- Cannon, W. F., & Horton, J. D. (2009). Soil geochemical signature of urbanization and industrialization – Chicago, Illinois, USA. Applied Geochemistry, 24, 1590–1601. doi:10.1016/j.apgeochem.2009.04.023
- Caussy, D., Gochfeld, M., Gurzau, E., Neagu, C., & Ruedel, H. (2003). Lessons from case studies of metals: Investigating exposure, bioavailability, and risk. Ecotoxicology and Environmental Safety, 56, 45–51. doi:10.1016/S0147-6513(03)00049-6
- CCME. (2007). Canadian soil quality guidelines for the Protection of Environmental and Human Health (Technical Report, pp. 1–10). CCME soil quality index 1.0.
- Chabukdhara, M., & Nema, A. K. (2013). Heavy metals assessment in urban soil around industrial clusters in Ghaziabad, India: Probabilistic health risk approach. Ecotoxicology and Environmental Safety, 87, 57–64. doi:10.1016/j.ecoenv.2012.08.032
- Chen, T.-B., Zheng, Y.-M., Lei, M., Huang, Z.-C., Wu, H.-T., Chen, H., … Fan, Ke-Ke. (2005). Assessment of heavy metal pollution in surface soils of urban parks in Beijing, China. Chemosphere, 60, 542–551. doi:10.1016/j.chemosphere.2004.12.072
- Chen, Z., He, M., Sakurai, K., Kang, Y., & Iwasaki, K. (2007). Concentrations and chemical forms of heavy metals in urban soils of Shanghai, China. Soil Science and Plant Nutrition, 53, 517–529. doi:10.1111/j.1747-0765.2007.00173.x
- Chen, H., Teng, Y., Lu, S., Wang, Y., & Wang, J. (2015). Contamination features and health risk of soil heavy metals in China. Science of The Total Environment, 512–513, 143–153. doi:10.1016/j.scitotenv.2015.01.025
- Chudasama, B., Porwal, A., Kreuzer, O. P., & Butera, K. (2016). Geology, geodynamics and orogenic gold prospectivity modelling of the Paleoproterozoic Kumasi Basin, Ghana, West Africa. Ore Geology Reviews, 78, 692–711. doi:10.1016/j.oregeorev.2015.08.012
- Darko, G., Faanu, A., Akoto, O., Acheampong, A., Goode, E. J., & Gyamfi, O. (2015). Distribution of natural and artificial radioactivity in soils, water and tuber crops. Environmental Monitoring and Assessment, 187(6), 1–11. doi:10.1007/s10661-015-4580-9
- Darko, G., Dodd, M., Nkansah, M. A., Ansah, E., & Aduse-Poku, Y. (2017). Distribution and bioaccessibility of metals in urban soils of Kumasi, Ghana. Environmental Monitoring and Assessment, 189(6), 260. doi:10.1007/s10661-017-5972-9
- Doležalová Weissmannová, H., Pavlovský, J., & Chovanec, P. (2015). Heavy metal contaminations of urban soils in Ostrava, Czech Republic: Assessment of metal pollution and using principal component analysis. International Journal Environmental Research, 9, 683–696.
- Dragović, S., Mihailović, N., & Gajić, B. (2008). Heavy metals in soils: Distribution, relationship with soil characteristics and radionuclides and multivariate assessment of contamination sources. Chemosphere, 72, 491–495. doi:10.1016/j.chemosphere.2008.02.063
- Ducic, T., & Polle, A. (2005). Transport and detoxification of manganese and copper in plants. Brazilian Journal of Plant Physiology, 17, 103–112. doi:10.1590/S1677-04202005000100009
- Engelhardt, F. (2012). Creating an Environmental Geographic Information System for the City of Kumasi, Ghana. Diva-Portal, 1–5.
- Franco-Uría, A., López-Mateo, C., Roca, E., & Fernández-Marcos, M. L. (2009). Source identification of heavy metals in pastureland by multivariate analysis in NW Spain. Journal of Hazardous Materials, 165, 1008–1015. doi:10.1016/j.jhazmat.2008.10.118
- Frempong, E. M. (1994). Geotechnical properties of some residual micaceous soils in the Kumasi Metropolitan area (Ghana). Bulletin of the International Association of Engineering Geology, 49, 47–54. doi:10.1007/BF02595000
- Glennon, M. M., Harris, P., Ottesen, R. T., Scanlon, R. P., & O’Connor, P. J. (2014). The Dublin SURGE Project: Geochemical baseline for heavy metals in topsoils and spatial correlation with historical industry in Dublin, Ireland. Environmental Geochemistry and Health, 36, 235–254. doi:10.1007/s10653-013-9561-8
- Gotelli, N. J., & Ellinson, A. M. (2013). Summary for policymakers. In Intergovernmental Panel on Climate Change (Ed.), Climate Change 2013—The physical science basis (2nd ed., Vol. 53, pp. 1–30). Cambridge, MA: Cambridge University Press. doi:10.1017/CBO9781107415324.004
- Hakanson, L. (1980). An ecological risk index for aquatic pollution control.a sedimentological approach. Water Research, 14, 975–1001. doi:10.1016/0043-1354(80)90143-8
- Iddrisu, A., Mano, Y., & Sonobe, T. (2012). Entrepreneurial skills and industrial development: The case of a car repair and metalworking cluster in Ghana. Journal of the Knowledge Economy, 3, 302–326. doi:10.1007/s13132-011-0047-6
- Kabata-Pendias, A., & Pendias, H. (2001a). Trace element in soils and plants (4th ed.). London: CRC Press.
- Kabata-Pendias, A., & Pendias, H. (2001b). Trace elements in soils and plants (3rd ed., pp. 1–533). Boca Raton: CRC Press.
- Kaminski, M. D., & Landsberger, S. (2000). Heavy metals in urban soils of East St. Louis, IL, Part I: Total concentration of heavy metals in soils. Journal of the Air & Waste Management Association, 50, 1667–1679. doi:10.1080/10473289.2000.10464195
- Kapustka, L. A., Clements, W. H., Ziccardi, L., Paquin, P. R., Sprenger, M., & Wall, D. (2004). Issue paper on the ecological effects of metals. Washington, DC: U.S. Environmental Protection Agency.
- Li, X., Liu, L., Wang, Y., Luo, G., Chen, X., Yang, X., … He, X. (2013). Heavy metal contamination of urban soil in an old industrial city (Shenyang) in Northeast China. Geoderma, 192, 50–58. doi:10.1016/j.geoderma.2012.08.011
- Liu, C. W., Lin, K. H., & Kuo, Y. M. (2003). Application of factor analysis in the assessment of groundwater quality in a blackfoot disease area in Taiwan. Science of The Total Environment, 313, 77–89.10.1016/S0048-9697(02)00683-6
- Losiak, A., Schulz, T., Buchwaldt, R., & Koeberl, C. (2013). Petrology, major and trace element geochemistry, geochronology, and isotopic composition of granitic intrusions from the vicinity of the Bosumtwi impact crater, Ghana. Lithos, 177, 297–313. doi:10.1016/j.lithos.2013.06.002
- Luo, X., Yu, S., & Li, X. (2012). The mobility, bioavailability, and human bioaccessibility of trace metals in urban soils of Hong Kong. Applied Geochemistry, 27, 995–1004. doi:10.1016/j.apgeochem.2011.07.001
- Miller, A., Partington, G., & Kreuzer, O. (2015). Regional prospectivity modelling in data-poor areas: The Kumasi Basin, Ghana. In Proceedings of AusIMM. Perth.
- Nkansah, M. A., Korankye, M., Darko, G., & Dodd, M. (2016). Heavy metal content and potential health risk of geophagic white clay from the Kumasi Metropolis in Ghana. Toxicology Reports, 3, 644–651. doi:10.1016/j.toxrep.2016.08.005
- Odewande, A. A., & Abimbola, A. F. (2008). Contamination indices and heavy metal concentrations in urban soil of Ibadan metropolis, southwestern Nigeria. Environmental Geochemistry and Health, 30, 243–254. doi:10.1007/s10653-007-9112-2
- Pakade, V. E., Cukrowska, E. M., Darkwa, J., Darko, G., Torto, N., & Chimuka, L. (2012). Simple and efficient ion imprinted polymer for recovery of uranium from environmental samples. Water Science and Technology, 65, 728–736. doi:10.2166/wst.2012.911
- Qing, X., Yutong, Z., & Shenggao, L. (2015). Assessment of heavy metal pollution and human health risk in urban soils of steel industrial city (Anshan), Liaoning, Northeast China. Ecotoxicology and Environmental Safety, 120, 377–385. doi:10.1016/j.ecoenv.2015.06.019
- Rodríguez Martín, J. A., Arias, M. L., & Grau Corbí, J. M. (2006). Heavy metals contents in agricultural topsoils in the Ebro basin (Spain). Application of the multivariate geoestatistical methods to study spatial variations. Environmental Pollution, 144, 1001–1012. doi:10.1016/j.envpol.2006.01.045
- Smith, J. U. (1995). Urban soil in landscape design. Journal of Environment Quality, 24, 793. doi:10.2134/jeq1995.00472425002400040037x
- Swarnalatha, K., Letha, J., Ayoob, S., & Nair, A. G. (2015). Risk assessment of heavy metal contamination in sediments of a tropical lake. Environmental Monitoring and Assessment, 187, 322. doi:10.1007/s10661-015-4558-7
- Tume, P., Bech, J., Sepulveda, B., Tume, L., & Bech, J. (2008). Concentrations of heavy metals in urban soils of Talcahuano (Chile): A preliminary study. Environmental Monitoring and Assessment, 140, 91–98. doi:10.1007/s10661-007-9850-8
- US EPA. (2007). Method 6200 - Field portable X-ray fluorescence spectrometry for the determination of elemental concentrations in soil and sediment.
- US EPA. (2015). Guidance for sample collection for in vitro bioaccessibility assay for lead (Pb) in soil, (March). Retrieved from https://epa.gov/superfund/bioavailability/pdfs/EPA_Pb_IVBA_SOP_040412_FINAL_SRC.pdf
- VROM. (2000). Circular on target values and intervention values for soil remediation. Netherlands Government Gazette, 2000, 1–12.
- Wong, C. S. C., Li, X., & Thornton, I. (2006). Urban environmental geochemistry of trace metals. Environmental Pollution, 142(1), 1–16. doi:10.1016/j.envpol.2005.09.004
- Wu, Q., Leung, J. Y. S., Geng, X., Chen, S., Huang, X., Li, H., … Lu, Y. (2015). Heavy metal contamination of soil and water in the vicinity of an abandoned e-waste recycling site: Implications for dissemination of heavy metals. Science of The Total Environment, 506–507, 217–225. doi:10.1016/j.scitotenv.2014.10.121