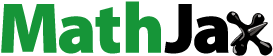
Abstract
Low-cost chitosan beads were prepared by dropping chitosan solution into an alkaline bath and then were used for Cr(VI) and Zn(II) ions adsorption from aqueous solution. Prepared chitosan beads were characterized by Fourier transform infrared spectroscopy (FTIR), thermal gravimetric analysis (TGA), Brunauer–Emmett–Teller (BET) measurements, scanning electron microscopy (SEM), and energy dispersive X-ray spectroscopy (EDS). The effect of solution-pH, contact time, initial ion concentration, and temperature on both metal ions adsorption was investigated. The kinetics of adsorption suggested a pseudo-second-order model fits better than pseudo-first-order model for both metals. The equilibrium adsorption isotherm of both metals matches well with the Langmuir isotherm model. The maximum adsorption capacity of chitosan beads was 79.56 mg Cr(VI)/g and 109.18 mg Zn(II)/g at initial ion concentration 1,000 mg/L, and temperature 10 °C. Thermodynamic parameters showed that the adsorption of Cr(VI) and Zn(II) ions onto chitosan beads was feasible, spontaneous, and exothermic under the studied conditions. While the chitosan beads enabled a good adsorption application, further lab work and field studies are necessary before using in a practical adsorption process.
Public Interest Statement
Cr(VI) and Zn(II) ions in water and wastewater streams are not biodegradable in nature and they have a significant effect on the ecosystem and human health. So, it is important to remove them before supplying water to the public. The objective of this study is to examine the performance of new composite adsorbents that were prepared from sustainable material, chitosan, to remove Cr(VI) and Zn(II) ions from aqueous solution instead of using traditional adsorbents such as activated carbon which is more expensive. In addition, we investigated the optimum adsorption conditions to enhance the adsorption capacity of Zn(II) and Cr(VI) ions from aqueous solution. Overall results from this research suggest that the prepared chitosan beads could be employed as a cost-effective and excellent alternative adsorbent for Cr(VI) and Zn(II) ions removal from water and wastewater.
Competing Interests
The authors declare no competing interest
1. Introduction
The most important and essential compound on the ground is water for all living creatures. However, rapidly growing population, climate change, and environmental deterioration affect the quality of water supplies. Water contamination caused by heavy metals has been identified as serious environmental issues worldwide, since heavy metals are highly toxic, non-biodegradable, non-metabolizable, and can cause many biological abnormalities and tend to accumulate in the food chains. Heavy metals mainly exist in the wastewaters of many industries such as metal plating, mining operations, electric device manufacturing, and battery production (Min et al., Citation2015). There are many heavy metal ions including chromium and zinc that appear in the US Environmental Protection Agency’s priority list of pollutants due to their high toxicity, prevalence, existence, and persistence in the environment (Satya, Citation2015). The accumulation of Cr(VI) in human body causes a stomach erosion, hemorrhaging, and death is likely. The main symptoms of Zn(II) poisoning are an electrolyte imbalance, stomachache, dehydration, nausea, dizziness, and incoordination in muscles (Jain, Singhal, & Sharma, Citation2004). Physical, chemical, and biological processes for heavy metals removal from wastewater have been extensively researched and used such as adsorption, reverse osmosis, ion exchange, evaporation, solvent extraction, chemical precipitation, filtration, flotation, membrane, coagulation and flocculation, and electrochemical methods (Hua et al., Citation2012). Adsorption has been considered as the most cost-effective method for heavy metals removal from aqueous solution because the process is simple in design and chemical consumption or/and waste generation are not a significant issue compared with other methods. Traditional adsorbents such as activated carbon are not efficient in a low concentration of pollutants, and therefore it needs additives with a higher surface area to enhance the adsorption capacity. In recent decades, bioadsorbent materials for biological origin have emerged as an attractive material for removing heavy metals from wastewater streams, largely because these materials are sustainable, abundant in nature, cheap, effective in low and medium metal level, and easy to regenerate for reuse (Babel & Kurniawan, Citation2003). Many biological materials have shown a good adsorption capacities for heavy metals from aqueous solution such as alginate (Pandey, Bera, Shukla, & Ray, Citation2007), seaweed (Ghimire, Inoue, Ohto, & Hayashida, Citation2008), husk (Ricordel, Taha, Cisse, & Dorange, Citation2001), sugar beet pulp (Ozer & Tumen, Citation2005), and chitosan (Zhao, Repo, Yin, & Sillanpää, Citation2013).
Chitosan is a natural organic material, commercially produced by partial deacetylation of chitin, which is the second plentiful organic component in nature next to cellulose polymer. Due to the presence of amino (–NH2) and hydroxyl (–OH) groups in its structure, chitosan is capable to adsorb heavy metals from aqueous solution by creating an electronic bond between these active sites and metal ions (Jagtap et al., Citation2009; Ngah, Ab Ghani, & Kamari, Citation2005). In addition, chitosan has been investigated by many studies as an excellent material for heavy metal adsorption such as cadmium and copper (Shyam & Arun, Citation2014), arsenic (Min et al., Citation2015), and chromium and zinc (Annaduzzaman, Citation2015; Qudsieh, Mirghani, Kabbashi, & Muyibi, Citation2014; Sofiane & Sofia, Citation2015; Toledo et al., Citation2014).
In this study, chitosan was prepared in spherical shape instead of using its original form powder, which has been applied in previous studies, to enhance its total surface area, reduce its agglomeration in acidic media, and to increase the metal ions spreading onto active sites, and then it was used to remove Cr(VI) and Zn(II) ions from aqueous solution. In addition, the performance of prepared chitosan beads was characterized by Fourier transform infrared spectroscopy (FTIR), thermal gravimetric analysis (TGA), Brunauer–Emmett–Teller (BET) measurements, scanning electron microscopy (SEM), and energy dispersive X-ray spectroscopy (EDS). Moreover, the adsorption process was examined as a function of solution-pH, contact time, initial metal ion concentration, and temperature. Eventually, adsorption isotherms, thermodynamic parameters, and the mechanism of adsorption were investigated.
2. Materials and methods
2.1. Materials
Medium molecular weight chitosan biopolymer (deacetylation degree is 87%, the molecular weight is ~190,000–310,000 g/mol) was procured from Aldrich Chemical Corporation and used to synthesize chitosan beads. Zinc sulfate heptahydrate (H14O11SZn) and potassium dichromate (K2Cr2O7) were used to prepare a stock solution containing 1,000 mg/L of Cr(VI) or Zn(II) ions. Sodium hydroxide, oxalic acid, and deionized water were used to adjust the solution-pH and to dissolve chitosan. All chemicals that were used in this study were analytical grade and used as received without further purification.
2.2. Preparation of chitosan beads
Chitosan gel solution was prepared by dissolving 20 g of chitosan into 1 L of 0.2 mol/L oxalic acid solution under continuous stirring. The chitosan solution was heated to 65–70 °C to facilitate acylation. Spherical chitosan beads were prepared using the dropwise method—dropwise addition of the chitosan mixture gel solution into a precipitation bath containing 0.8 mol/L NaOH solution. The objective of dropping chitosan gel solution into a basic solution is to rapidly neutralize the drops and get a spherical shape of chitosan beads. The chitosan hydrogel beads remained in the NaOH solution with slowly stirred mixing (60 rpm) for 6 h for hardening. The hardened spherical chitosan beads were washed by deionized water for neutralizing and removing sodium ions that might be attached to the chitosan beads. Eventually, the chitosan beads were dried by vacuum furnace for 24 h at 70 °C. The final dry chitosan beads had an average diameter of 1 mm and they were available for adsorption experimental work as shown in Figure .
2.3. Adsorption experiments
Batch adsorption experiments of Cr(VI) and Zn(II) ions adsorption onto chitosan beads from aqueous solution were studied at initial metal concentrations of 50, 100, 250, 500, and 1,000 mg/L. In a series of 125 mL Erlenmeyer flasks, 50 mL of Cr(VI) or Zn(II) liquid solution at different concentrations and 0.5 g of chitosan beads were agitated in an orbital shaker at 200 rpm for 8 h. The volume of the liquid solution without chitosan beads was 42.5 mL, measured by burette after 8 h of stirring, and that volume was used to determine the chitosan beads adsorption capacity. After a certain time, samples were taken from the flasks and filtrated to determine the final metal ion concentrations in the solution samples. Inductively Coupled Plasma-Mass Spectrometer (ICP-MS) was used to determine the metal ion concentration in the samples. When the steady state of adsorption attained, the adsorption capacity of chitosan beads was determined using Equation (1) that generated from the mass balance of metal ions (Zhou, Li, Jin, Lian, & Han, Citation2017),
(1)
(1)
where qe is the equilibrium adsorption capacity (mmol/g), C0 is the initial metal concentration in the solution (mmol/L), and Ce is the equilibrium metal concentration in the solution (mmol/L), V is the volume of solution (L), and m is the mass of chitosan beads (g). Batch adsorption runs were studied at different temperatures, different initial metal ion concentrations, different solutions-pH, and different contact times.
3. Results and discussion
3.1. Characterization of chitosan beads
Chitosan beads were characterized to estimate some of their physical and chemical properties. They provide a better interpretation of Zn(II) and Cr(VI) adsorption mechanism associated with the adsorption process. Some physical and chemical properties of dried chitosan beads are summarized in Table . We can see that the BET surface area of chitosan beads was found to be 1.9 m2/g, which is not too large compared to other adsorbents like activated carbon and silica because chitosan material in its natural form is soft. The solution-pH of chitosan beads at the point of zero charges (pHZPC) was 5, that means the surface of chitosan beads has a positive charge at pH less than 5, which is presumably created by the protonation of amine groups in the chitosan. Therefore, the negative species are favorite to adsorb at low pH and species that have positive charges (heavy metals) are easily adsorbed and interacted on chitosan beads at pH greater than 5.
Table 1. Physical and chemical properties of the chitosan beads
Thermal gravimetric analysis (TGA) (Figure S1, supplementary material) was done to investigate the thermal stability of chitosan beads. Figure S1 shows that there are three distinctive steps of weight loss. First, about 5% weight loss occurred at 144 °C due to the loss of water content (dehydration). Second, 24% weight loss occurred at 370 °C which may correspond to the degradation behavior of the chitosan. Third, about 38% weight loss happened at 686 °C which may correspond to the decomposition (thermal and oxidative) of chitosan. More details can be found in the Supplementary Material
Results of Fourier transform infrared spectroscopy (FTIR) analysis are shown in Figure S2. It can be seen that the chitosan beads exhibit a large band of the amine group (N-H) stretching between 3,200 and 3,510 cm−1, and a peak between 1,400 and 1,655 cm−1 corresponds to C=N stretching bond. These observations indicate that the amine groups are present in chitosan beads and successfully bonded with heavy metals, indicating that the metal ions are adsorbed onto chitosan beads (Qi, Xu, Jiang, Hu, & Zou, Citation2004). It can be seen from scanning electron microscopy (SEM) images (Figure S3) that the surface of fresh chitosan beads is wrinkle and porous, whereas the surface of loaded chitosan beads with heavy metals is less porous due to the adsorption of heavy metals.
In order to determine the elemental composition of the chitosan beads, energy dispersive X-ray spectroscopy (EDS) analysis was attained. EDS analysis clearly shows the presence of C, N, and O, which can be seen in Figure S4. The higher percentage of O along with N enhances the interaction between chitosan beads and heavy metals.
3.2. Effect of initial pH on adsorption
Figure shows the effect of solution-pH on the adsorption of Zn(II) and Cr(VI) ions onto chitosan beads. These results show that the Cr(VI) ion adsorption was raised up from 1.2 mmol/g at pH 2 to 1.53 mmol/g at pH 5 and then was raised down to 1.35 mmol/g at pH 8. The maximum rate of Cr(VI) removal from aqueous solution onto chitosan beads was about 1.53 mmol/g (79.56 mg/g) at pH 5. In acidic solution, the Cr(VI) ions are mostly in form of H2CrO4 which has a neutral charge and does not adsorb onto chitosan beads. It is assumed that the competition happened between the remaining Cr(VI) ions as and the positive hydrogen ions (H+) at low pH, between 2 and 4, which presumably reduced the adsorption capacity of Cr(VI). These results are in a good agreement with Toledo et al. (Citation2014); Arvand and Pakseresht (Citation2013); Hu et al. (Citation2011). In their experiments, in basic solution (pH > 7), the protonation of amine groups was reduced and the concentration of hydroxyl groups (OH−) increased which creates a negative surface charge on chitosan beads. That leads to produce a repulsive interaction between
ions and chitosan beads, which decreases the adsorption capacity of Cr(VI) in basic solution (Malkoc, Nuhoglu, & Dundar, Citation2006; Toledo et al., Citation2014).
Figure 2. Effect of initial solution-pH on Zn(II) and Cr(VI) adsorption onto chitosan beads (initial concentration (C0) for Zn(II) = 15.3 mmol/L; C0 for Cr(VI) = 19.23 mmol/L; temperature = 10 °C; mass of chitosan beads = 0.5 g).
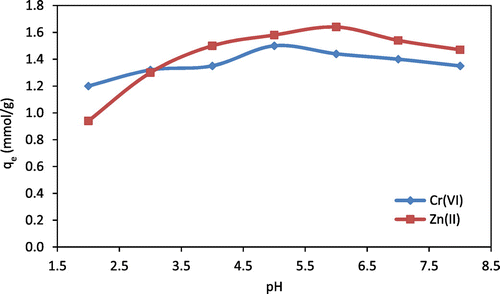
The increase in Zn(II) ions removal with increasing solution-pH from 2 to 6 could be explained by reducing the hydrogen proton (H+) and positive Zn(II) ions competition at the same active sites on chitosan beads. In acidic media, chitosan beads surface is positively charged due to the protonation of amino groups, which decreases the interaction between active sites in chitosan beads and Zn(II) ions (Karthikeyan, Anbalagan, & Andal, Citation2004). After solution-pH exceeded 6, it was observed that the zinc hydroxide was formed and precipitated in the solution. The results showed that the Zn(II) adsorption onto chitosan beads from aqueous solution was affected strongly by solution-pH and the maximum adsorption capacity for Zn(II) ion was 1.67 mmol/g (109.18 mg/g) at pH 6. In addition, it was observed that at low solution-pH (pH = 3), swelling and agglomeration of chitosan beads occurred and tended to form a gel.
3.3. Effect of initial ion concentration on adsorption
The effect of initial metal concentration on adsorption capacity of Cr(VI) and Zn(II) ions onto chitosan beads was investigated by considering different ranges of initial Cr(VI) concentrations (0.96, 1.92, 4.8, 9.6, and 19.23 mmol/L) at pH 6 and temperature 10 °C. And for Zn(II) ions, initial concentrations were 0.76, 1.53, 3.8, 7.65, and 15.3 mmol/L at pH 5, temperature 10 °C, and 0.25–8 h contact time. Varying the initial metal concentration can affect the driving forces of adsorption and consequently affect the adsorption behavior of metal ions onto chitosan beads. The results are illustrated in Figure . Cr(VI) ions removal was significantly increased from 0.084 to 1.53 mmol/g when the initial Cr(VI) concentration increased from 0.96 to 19.23 mmol/L. However, increasing the initial Zn(II) concentration from 0.76 to 15.3 mmol/L caused an increase in Zn(II) removal from 0.1 to 1.67 mmol/g onto chitosan beads. That is because, at high initial metal concentration, the concentration gradient between bulk solution and chitosan beads surface overcomes the mass transfer resistance of Zn(II) and Cr(VI) ions. The adsorption of both metals was rapid in the first 1.5 h and then slowed down until it reached the equilibrium in around 3 h in most of the adsorption runs. It is understood that all the adsorption sites are available at the initial stage (fast adsorption), while the adsorption sites are gradually occupied with the adsorption process until all of them are not be free. These results show that the adsorption process of Cr(VI) and Zn(II) ions onto chitosan beads from aqueous solution is fast and affected significantly by initial metal ion concentration.
3.4. Effect of temperature on adsorption
The effect of temperature on the adsorption of Cr(VI) and Zn(II) ions onto chitosan beads was investigated at 10, 20, 30, and 40 °C. As shown in Figure , the results showed that the low temperatures were favorite for Cr(VI) and Zn(II) ions adsorption onto chitosan beads. It can be seen that due to a temperature increase from 10 to 40 °C, the Zn(II) adsorption was reduced to 1.34 mmol/g from 1.67 mmol/g, whereas, the Cr(VI) adsorption was decreased from 1.53 at 10 °C to 1.12 mmol/g at 40 °C. The reason for this phenomenon is when the temperature increases, the solubility of metal ions species increases in the solution. Consequently, the van der Waals interaction forces between the metal ions and solution are stronger than those between metal ions and adsorbent (Saha & Chowdhury, Citation2011). As a result, the metal ions are more difficult to adsorb at high temperature.
Figure 4. Equilibrium adsorption capacity (qe) as a function of temperature (T) for Cr(VI) and Zn(II) (C0 for Zn(II) = 15.3 mmol/L; C0 for Cr(VI) = 19.23 mmol/L; pH = 5–6; mass of chitosan beads = 0.5 g).
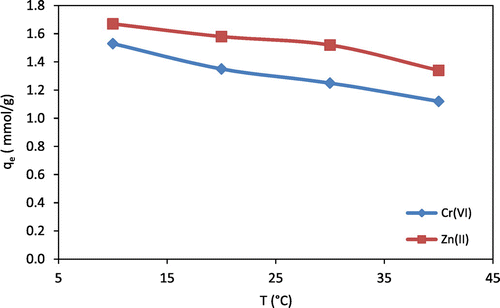
Thermodynamic parameters including Gibb’s free energy (ΔG°), enthalpy (ΔH°), and entropy (ΔS°) were determined for Cr(VI) and Zn(II) adsorption onto chitosan beads using Equation (2) (Tellinghuisen, Citation2006):
(2)
(2)
where ΔGo can be expressed as,
(3)
(3)
Substituting Equation (2) into (3) provides the van’t Hoff equation (Salih & Ghosh, Citation2018; Tellinghuisen, Citation2006),
(4)
(4)
(5)
(5)
where R is the universal gas constant (8.314 J/mol.K), T is the absolute temperature (K), ΔG° is Gibb’s free energy (J/mol), and Kd is the equilibrium partition coefficient between solid and liquid solution (L/g), Ce is the equilibrium concentration of metal ions in the solution (mmol/L), qe is the equilibrium adsorption capacity onto chitosan beads (mmol/g).
By plotting (ln Kd) against 1/T, the values of ΔH° (J/mol) and ΔS° (J/mol) can be determined (Figure ). The determined ΔH° may be considered as the heat of adsorption when the adsorption capacity of chitosan beads reaches its saturated capacity of Cr(VI) or Zn(II) ions. Obtained values of ln Kd, ΔH°, ΔS°, and ΔG° are listed in Table . The results of metal ions adsorption onto chitosan beads show that the adsorption process was exothermic and the negative values of ΔG° at the low temperature specified that the adsorption was spontaneous. The thermodynamic parameter values that were obtained in this study are in a good agreement with Aydin and Aksoy (Citation2009) and Hawari, Rawajfih, and Nsour (Citation2009).
Figure 5. ln Kd as a function of 1/T for Cr(VI) and Zn(II). Lines indicate linear fits (C0 = 1,000 mg/L for both metals).
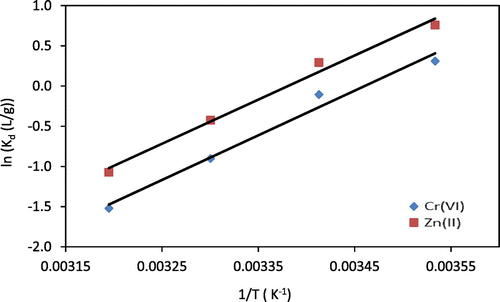
Table 2. Thermodynamic parameters and fitted ln Kd of Cr(VI) and Zn(II) adsorption onto chitosan beads
Isosteric heat of adsorption (ΔHx) is a useful pertinent thermodynamic factor for characterizing the temperature effect on the adsorption process. It is defined as the heat of adsorption occurring at constant adsorption capacities of metal ions onto adsorbent using the well-known Clausius–Clapeyron Equation (Saha & Chowdhury, Citation2011),
(6)
(6)
Integrating Equation (6), assuming that the does not depend on temperature, gives Equation (7) (Saha & Chowdhury, Citation2011),
(7)
(7)
where Kq is the integral constant (mmol/L), Ce is the equilibrium concentration of metal ions in the solution (mmol/L), R is the universal gas constant (8.314 J/mol.K), T is the absolute temperature (K). From the slope of plotting (ln Ce) against 1/T (Figures and ), the value of ΔHx can be determined. Values of ΔHx were obtained at different temperatures (10, 20, 30, and 40 °C) and at different adsorption capacities of Cr(VI) or Zn(II) ions onto chitosan beads. From Table , it could be seen that the variation of ΔHx values with adsorption capacities of Cr(VI) or Zn(II) is indicative that the chitosan beads have energetically homogeneous surfaces. In addition, the variation of ΔHx values with the adsorption capacities can be attributed to the possibility of the competition between the adsorbed heavy metal ions and other ions in solution such as Na, SO4, originating from metal salts onto the same active groups (–NH2, –OH). The results show that the ΔHx was strongly depended on the adsorption capacities, which decreased with increasing the adsorption capacities. This can be seen as a hint on a rather homogeneous surface of chitosan beads. Otherwise, in case the surface of chitosan beads would be heterogeneous, the ΔHx of metal ions adsorption onto chitosan beads would be constant even at varying adsorption capacities of metal ions (Saha & Chowdhury, Citation2011; Doke & Khan, Citation2013). It can be assumed that the high value of ΔHx at low adsorption capacities (qe) refers to high initial interactions between adsorbed metal ions and chitosan beads due to the presence of highly active amine and hydroxyl groups within the chitosan (Saha & Chowdhury, Citation2011).
Figure 6. ln Ce as a function of 1/T for Cr(VI) adsorption onto chitosan beads at different adsorption capacities (qe). Lines indicate linear fits.
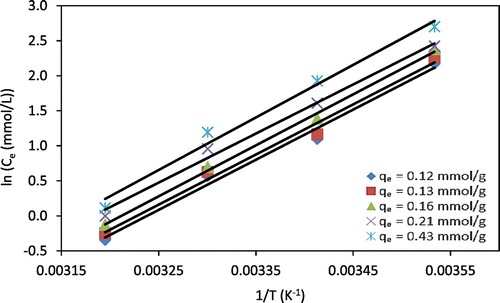
Figure 7. ln Ce as a function of 1/T for Zn(II) adsorption onto chitosan beads at different adsorption capacities (qe). Lines indicate linear fits.
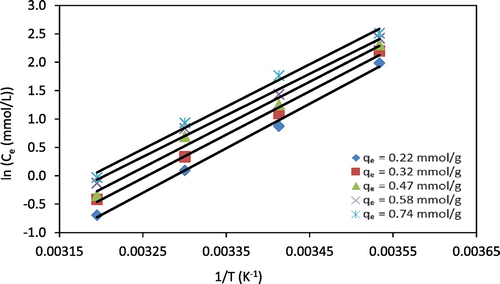
Table 3. Isosteric heat of Cr(VI) and Zn(II) adsorption onto chitosan beads at constant adsorption capacities (qe) with Kq-constant values, and coefficients of determination
3.5. Adsorption kinetics
The adsorption kinetics of Cr(VI) and Zn(II) adsorption onto chitosan beads were determined by carrying out measurements after different contact times (0.25, 0.5, 0.75, 1, 2, 3, 4, and 8 h), a temperature of 10 °C, a solution-pH of 6, and an initial metal concentration of 1,000 mg/L. To explore adsorption behavior, both pseudo-first-order and pseudo-second-order models were used to fit adsorption data, as shown in Figure . Generally, the linear pseudo-first-order and pseudo-second-order models are, respectively, expressed by Equations (8) and (9) (Ho & McKay, Citation1998; HO & McKay, Citation1999),
Figure 8. (a) Pseudo first-order kinetics (solid lines), (b) pseudo second-order kinetics (solid lines) fitted for Cr(VI) and Zn(II) adsorption experiments (pH = 5–6; mass of chitosan beads = 0.5 g; C0 = 1,000 mg/L for both metals; temperature = 10 °C).
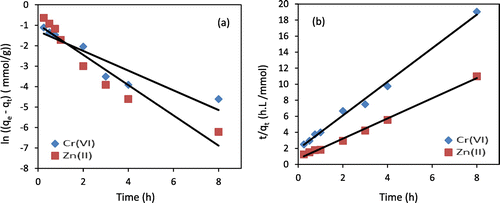
(8)
(8)
(9)
(9)
where qe and qt (mmol/g) are the adsorption capacities of metal ions onto chitosan beads at equilibrium and at time t (h), respectively, k1 is the rate constant of the first-order adsorption process (h−1), k2 is the rate constant of the second-order adsorption process [g/(mmol.h)].
It can be clearly observed from Figure that the adsorption data of both metals were fitted better by the pseudo-second-order model (Figure (b)) than the pseudo-first-order model (Figure (a)), suggesting that the whole adsorption process is mainly controlled by chemisorption that is involved in valence forces for sharing or exchanging electrons through coordination or chelation between amine groups in chitosan beads and heavy metal ions (Zhang et al., Citation2017).
3.6. Adsorption isotherms
Adsorption equilibrium was studied at different initial metal concentrations, for Cr(VI) ions at 0.96, 1.92, 4.8, 9.6, and 19.23 mmol/L, and for Zn(II) ions at 0.76, 1.53, 3.8, 7.65, and 15.3 mmol/L. The adsorption experiments were carried out at 10 °C for 8 h. The Langmuir and Freundlich models are the most commonly used isotherm models. The Langmuir isotherm model is based on monolayer adsorption onto an adsorbent surface containing a limited number of adsorptive sites with uniform energies. The Freundlich isotherm model, can be used for the adsorption onto heterogeneous surfaces and multilayer adsorption with different energies (Caner, Ahmet, & Mustafa, Citation2015).
Langmuir and Freundlich isotherm models were fitted to the equilibrium adsorption data to determine the relationship between the adsorption isotherm parameters and to shed light on how the heavy metals interact with the chitosan beads surfaces. In linearized form, Langmuir isotherm is expressed as follows (Chou, Wang, Chang, & Chang, Citation2010),
(10)
(10)
where Ce is the equilibrium concentration of metal ions in the solution (mmol/L), qe and qm are the equilibrium and theoretical maximum adsorption capacity (mmol/g), respectively. KL is the Langmuir constant (L/g) related to binding energy.
The Freundlich isotherm is given as follows (Chou et al., Citation2010),
(11)
(11)
where KF is the Freundlich constant (mmol/g) and n is the Freundlich exponent (-). Langmuir and Freundlich isotherms for Cr(VI) and Zn(II) adsorption onto chitosan beads from aqueous solution are presented in Figure , and fitted kinetic parameters are shown in Table . Based on the contrasting results of the correlation coefficients R2 of Langmuir isotherm () and Freundlich model (
), the correlation coefficients of Langmuir isotherm (
) were all above 0.91 for both metal ions, indicating that the data were fitted better by Langmuir isotherm than by Freundlich isotherm, suggesting that Langmuir isotherm model could well interpret the adsorption procedure, which indicates that the adsorption process of both metals on the chitosan beads is driven by the formation of a heavy metal monolayer on the adsorbent surfaces. In this case, such surfaces show a homogeneous morphology of chitosan beads and a finite number of identical active sites (Chou et al., Citation2010; Vaz, Pereira, Fajardo, Azevedo, & Rodrigues, Citation2017).
Figure 9. (a) Langmuir isotherms (solid lines), (b) Freundlich isotherms (solid lines) fitted for Cr(VI) and Zn(II) adsorption experiments (pH = 5–6; mass of chitosan beads = 0.5 g; temperature = 10 °C).
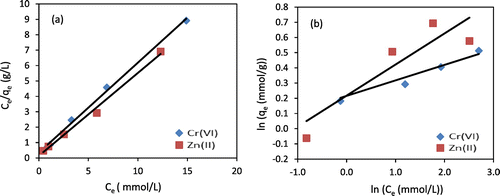
Table 4. Langmuir and Freundlich isotherm parameters for Cr(VI) and Zn(II) adsorption onto chitosan beads
4. Conclusions
This study indicates that the removal rate of Cr(VI) and Zn(II) ions from aqueous solution onto chitosan beads was excellent and up to 1.67 mmol/g (109.18 mg/g) for Zn(II), and 1.53 mmol/g (79.56 mg/g) for Cr(VI). The unique properties of chitosan make it an exciting and promising agent for purification of industrial wastewater purposes. The capacities of Cr(VI) and Zn(II) adsorption onto chitosan were strongly dependent on solution-pH, contact time, and temperature. The highest adsorption capacities were observed at pH 6 for Zn(II) and at pH 5 for Cr(VI), and at temperature 10 °C. Adsorption kinetic data were well-fitting with the pseudo-second-order kinetic model for both metals. Cr(VI) and Zn(II) ions adsorption onto chitosan beads could be described well by the Langmuir model with a R2 equal to 0.997 and 0.915, respectively. Thermodynamic quantities such as Gibb’s free energy (ΔG°), enthalpy (ΔH°), entropy (ΔS°), and isosteric heat of adsorption (ΔHx) indicated that both metal ions adsorption onto chitosan beads was exothermic, spontaneous, and the surface of chitosan beads was energetically homogeneous. It was observed that at low pH, swelling of chitosan beads occurred and tended to form a gel. Therefore, it is necessary to modify chitosan physically or chemically before applicable use in adsorption.
Supplementary material
Supplementary material for this article can be accessed here https://doi.org/10.1080/23311843.2017.1401577.
Supplementary_Material.docx
Download MS Word (560.2 KB)Acknowledgments
The authors thank the Chemical Engineering, and Nuclear Science and Engineering Institute at the University of Missouri-Columbia for financial support of the experimental studies and in general help with other analyses.
Additional information
Funding
Notes on contributors
Suhaib S. Salih
Suhaib S. Salih is currently a research assistant at the University of Missouri-Columbia majoring in chemical engineering. He received his BE degree in 2006 and MSc in 2012, in chemical engineering from the University of Tikrit, Tikrit, Iraq. In 2015, he joined the department of chemical engineering, University of Missouri-Columbia, as a PhD student. His current research interests are in adsorption processes. He has expertise in lab management, operation of atomic absorption spectrophotometer, intra red spectrophotometer, ultra violet spectrophotometer, and HPLC spectrophotometer.
References
- Annaduzzaman, M. (2015). Chitosan biopolymer as an adsorbent for drinking water treatment: Investigation on arsenic and uranium ((Doctoral dissertation). KTH Royal Institute of Technology, Stockholm.
- Arvand, M., & Pakseresht, M. A. (2013). Cadmium adsorption on modified chitosan-coated bentonite: Batch experimental studies. Journal of Chemical Technology and Biotechnology, 88(4), 572–578.10.1002/jctb.2013.88.issue-4
- Aydın, Y. A., & Aksoy, N. D. (2009). Adsorption of chromium on chitosan: Optimization, kinetics, and thermodynamics. Chemical Engineering Journal, 151(1–3), 188–194.10.1016/j.cej.2009.02.010
- Babel, S., & Kurniawan, T. A. (2003). Low-cost adsorbents for heavy metals uptake from contaminated water: A review. Journal of Hazardous Materials, 97(1–3), 219–243.10.1016/S0304-3894(02)00263-7
- Caner, N., Ahmet, S., & Mustafa, T. (2015). Adsorption characteristics of mercury (II) ions from aqueous solution onto chitosan-coated diatomite. Industrial & Engineering Chemistry Research, 54(30), 7524–7533.10.1021/acs.iecr.5b01293
- Chou, W. L., Wang, C. T., Chang, W. C., & Chang, S. Y. (2010). Adsorption treatment of oxide chemical mechanical polishing wastewater from a semiconductor manufacturing plant by electrocoagulation. Journal of Hazardous Materials, 180(1–3), 217–224.10.1016/j.jhazmat.2010.04.017
- Doke, K. M., & Khan, E. M. (2013). Adsorption thermodynamics to clean up wastewater; critical review. Reviews in Environmental Science and Bio/Technology, 12(1), 25–44.10.1007/s11157-012-9273-z
- Ghimire, K. N., Inoue, K., Ohto, K., & Hayashida, T. (2008). Adsorption study of metal ions onto crosslinked seaweed Laminaria japonica. Bioresource Technology, 99(1), 32–37.10.1016/j.biortech.2006.11.057
- Hawari, A., Rawajfih, Z., & Nsour, N. (2009). Equilibrium and thermodynamic analysis of zinc ions adsorption by olive oil mill solid residues. Journal of Hazardous Materials, 168(2–3), 1284–1289.10.1016/j.jhazmat.2009.03.014
- Ho, Y. S., & McKay, G. (1998). Sorption of dye from aqueous solution by peat. Chemical Engineering Journal, 70(2), 115–124.10.1016/S0923-0467(98)00076-1
- Ho, Y. S., & McKay, G. (1999). Pseudo-second order model for sorption processes. Process Biochemistry, 34(5), 451–465.10.1042/bj3440451
- Hu, X. J., Wang, J. S., Liu, Y. G., Li, X., Zeng, G. M., Bao, Z. L., & Long, F. (2011). Adsorption of chromium (VI) by ethylenediamine-modified cross-linked magnetic chitosan resin: Isotherms, kinetics and thermodynamics. Journal of Hazardous Materials, 185(1), 306–314.10.1016/j.jhazmat.2010.09.034
- Hua, M., Zhang, S., Pan, B., Zhang, W., Lv, L., & Zhang, Q. (2012). Heavy metal removal from water/wastewater by nanosized metal oxides: A review. Journal of Hazardous Materials, 211, 317–331.10.1016/j.jhazmat.2011.10.016
- Jagtap, S., Thakre, D., Wanjari, S., Kamble, S., Labhsetwar, N., & Rayalu, S. (2009). New modified chitosan-based adsorbent for defluoridation of water. Journal of Colloid and Interface Science, 332(2), 280–290.10.1016/j.jcis.2008.11.080
- Jain, C. K., Singhal, D. C., & Sharma, M. K. (2004). Adsorption of zinc on bed sediment of River Hindon: Adsorption models and kinetics. Journal of Hazardous Materials, 114(1), 231–239.10.1016/j.jhazmat.2004.09.001
- Karthikeyan, G., Anbalagan, K., & Andal, N. M. (2004). Adsorption dynamics and equilibrium studies of Zn(II) onto chitosan. Journal of Chemical Sciences, 116(2), 119–127.10.1007/BF02708205
- Malkoc, E., Nuhoglu, Y., & Dundar, M. (2006). Adsorption of chromium (VI) on panacea olive oil industry waste: Batch and column studies. Journal of Hazardous Materials, 138(1), 142–151.10.1016/j.jhazmat.2006.05.051
- Min, L. L., Yuan, Z. H., Zhong, L. B., Liu, Q., Wu, R. X., & Zheng, Y. M. (2015). Preparation of chitosan-based electrospun nanofiber membrane and its adsorptive removal of arsenate from aqueous solution. Chemical Engineering Journal, 267, 132–141.10.1016/j.cej.2014.12.024
- Ngah, W. W., Ab Ghani, S., & Kamari, A. (2005). Adsorption behavior of Fe(II) and Fe(III) ions in aqueous solution on chitosan and cross-linked chitosan beads. Bioresource Technology, 96(4), 443–450.10.1016/j.biortech.2004.05.022
- Ozer, A., & Tumen, F. (2005). Cu(II) adsorption from aqueous solutions on sugar beet pulp carbon. The European Journal of Mineral Processing and Environmental Protection, 5(1), 26–34.
- Pandey, A., Bera, D., Shukla, A., & Ray, L. (2007). Studies on Cr(VI), Pb(II) and Cu(II) adsorption-desorption using calcium alginate as biopolymer. Chemical Speciation & Bioavailability, 19(1), 17–24.10.3184/095422907X198031
- Qi, L., Xu, Z., Jiang, X., Hu, C., & Zou, X. (2004). Preparation and antibacterial activity of chitosan nanoparticles. Carbohydrate Research, 339(16), 2693–2700.10.1016/j.carres.2004.09.007
- Qudsieh, I. Y., Mirghani, E. S., Kabbashi, N. A., & Muyibi, S. A. (2014). Central composite design of zinc removal from model water using Chitosan biopolymer. Advances in Environmental Biology, 658–667.
- Ricordel, S., Taha, S., Cisse, I., & Dorange, G. (2001). Heavy metals removal by adsorption onto peanut husks carbon: Characterization, kinetic study, and modeling. Separation and Purification Technology, 24(3), 389–401.10.1016/S1383-5866(01)00139-3
- Saha, P., & Chowdhury, S. (2011). Insight into adsorption thermodynamics. INTECH.10.5772/558
- Salih, S. S., & Ghosh, T. K. (2018). Adsorption of Zn (II) ions by chitosan coated diatomaceous earth. International Journal of Biological Macromolecules, 106C, 602–610.
- Satya, C. W. S. (2015). Development of magnetic separation using modified magnetic chitosan for removal of pollutants in solution (PhD theses). Sapporo: Hokkaido University.
- Shyam, R. K., & Arun, R. R. (2014). Adsorption studies for the removal heavy metal by chitosan-g-poly (acrylic acid-co-acrylamide) composite. Science Journal of Analytical Chemistry, 2(6), 67–70.
- Sofiane, B., & Sofia, K. S. (2015). Biosorption of heavy metals by chitin and the chitosan. Dev Pharma Chemica, 7, 54–63.
- Tellinghuisen, J. (2006). Van’t Hoff analysis of K°(T): How good … or bad? Biophysical Chemistry, 120(2), 114–120.10.1016/j.bpc.2005.10.012
- Toledo, T. V., Bellato, C. R., Souza, C. H., Domingues, J. T., Silva, D. D. C., Reis, C., & Fontes, M. P. F. (2014). Preparation and evaluation of magnetic chitosan particles modified with ethylenediamine and Fe(III) for the removal of Cr(VI) from aqueous solutions. Química Nova, 37(10), 1610–1617.
- Vaz, M. G., Pereira, A. G., Fajardo, A. R., Azevedo, A. C., & Rodrigues, F. H. (2017). Methylene blue adsorption on chitosan-g-poly (acrylic acid)/rice husk ash superabsorbent composite: Kinetics, equilibrium, and thermodynamics. Water, Air, & Soil Pollution, 228(1), 14.10.1007/s11270-016-3185-4
- Zhang, H., Dang, Q., Liu, C., Cha, D., Yu, Z., Zhu, W., & Fan, B. (2017). Uptake of Pb(II) and Cd(II) on chitosan microsphere surface successively grafted by methyl acrylate and diethylenetriamine. ACS Applied Materials & Interfaces, 9(12), 11144–11155.10.1021/acsami.7b00480
- Zhao, F., Repo, E., Yin, D., & Sillanpää, M. E. (2013). Adsorption of Cd(II) and Pb(II) by a novel EGTA-modified chitosan material: Kinetics and isotherms. Journal of Colloid and Interface Science, 409, 174–182.10.1016/j.jcis.2013.07.062
- Zhou, T., Li, C., Jin, H., Lian, Y., & Han, W. (2017). Effective Adsorption/Reduction of Cr(VI) Oxyanion by Halloysite@ Polyaniline Hybrid Nanotubes. ACS Applied Materials & Interfaces, 9(7), 6030–6043.10.1021/acsami.6b14079