Abstract
The levels of organochloride pesticides (OCPs) residues in sediment samples from four stations in the Cienaga Grande (swamp area) of the lower Sinú River in the Caribbean region and receiving run-offs from the Sinú River effluents in Córdoba, Colombia were investigated. Ninety-six composite samples of surface sediments covering a radius of 3 m were randomly collected using the “Van Veen” dredge technique and were subsequently analyzed for pesticide residues using a gas chromatograph equipped with an electron capture detector. A total of five OCPs residues, most of them persistent organic pollutants, were identified and quantified. Results showed that the concentrations of various pesticides in sediments were in the range of 2.85–7.05 ng/g for α-hexachlorocyclohexane (α-HCH), 0.26–2.31 ng/g for β-HCH, 0.59–7.57 ng/g for γ-HCH (lindane), 0.69–1.72 for aldrin, and 1.55–2.25 ng/g for heptachlor epoxide. Among the OCPs, the Σ-HCH (total positional isomers of HCH) was the dominant compounds in the Cienaga Grande sediments. The study concluded that most of the stations monitored in the Cienaga Grande areas were contaminated with OCPs associated with the accumulation of water run-off from surrounding areas arriving at the location during the winter time. This in turn implies that the increased pollution load is related to the discharge of wastewater and solid waste from various municipal centers and towns located about the Cienaga Grande.
Public Interest Statement
Concentrations of five organochloride pesticide (OCP) residues, α-hexachlorocyclohexane (α-HCH), β-HCH, γ-HCH, aldrin and heptachlor epoxide, most of them persistent organic pollutants (POPs), were identified and quantified in sediment samples from four stations on the Cienaga Grande (swamp area) in the lower Sinú River of the Caribbean Colombia region, an approximate area of 791 km2 and receiving runoffs from Sinú River effluents on Cordoba. Results showed that among the OCPs detected, Σ-HCH (total HCH) was the most dominant and widely distributed compound in the sediments. It was also found that most of the stations monitored were contaminated with OCPs, mainly due to intense agricultural activity and slow degradation rates associated with the accumulation of water runoff from surrounding areas arriving. This study will provide a database to government authorities with the perspective to develop monitoring programs that will help minimize the risk of human exposure to organochloride pesticides.
Competing interests
The authors declare no competing interest
1. Introduction
The massive application of any substance or mixture of substances intended for preventing, destroying, repelling, or controlling any pest including unwanted species of plants or animals, is an integral part of modern agriculture and public health programs. In Colombia, as well as worldwide, agriculture without pesticides is not conceived; without them the crops would decrease significantly. The country is ranked as the second largest consumer of pesticides in South America, after Brazil, since it consumes approximately 48,620 tons per year, of which 8,718; 17,588; and 23,314 tons are insecticides, herbicides, and fungicides-bactericides, respectively, according to data compiled by the Food and Agriculture Organization (FAO) in 2013 (Gómez et al., Citation2013).
Research in Colombia on the effect of chemicals on the environment include: assessment of organochloride chemicals on farmers and flower growers based on protection measures used (Muñoz, Citation2009); concentrations of organochloride pesticides in water, sediments, and organisms on Cienaga Grande of Santa Marta (Betancourt & Ramírez, Citation2005, Espinosa & Ramírez, Citation1995); records of concentrations of aldrin, dichlorodiphenyltrichloroethane (DDT), lindane, and heptachlor in the Gulf of Morrosquillo (INVEMAR, Citation2001); determination of pollution from organochloride pesticides in water and two fish species of Cienaga Grande Lower Valley of the Sinú River (Lans, Díaz, & Páez, Citation2011; Lans, Marrugo, & Díaz, Citation2008), among other studies. In the Caribbean region of Colombia, the main sources of pesticides in the marine environment are banana, rice, grasses, cotton, corn, and fruit crops, as well as manufacture of these products in Cartagena and Barranquilla and are transported by rivers and run-off, such is the case of the Magdalena River through the main agricultural regions of the country and in basins that cross the banana region of Urabá, Córdoba, and Magdalena (Garay, Citation2001).
The distribution of various pollutants in sediments depends mainly on the physico-chemical properties of the ecosystem, the partition coefficients of individual contaminants, organic contents, and microflora activities (El Nemr, Moneer, Khaled, & El-Sikaily, Citation2013; Widenfalk, Citation2002). In fact, river sediments are substrates that can be used to infer the agricultural history of a region, since the wastes that are added to the ecosystem are accumulated in sediments, according to the agricultural practices of an area and the properties of agrochemicals used. Usually, the surface layers of sediment represent the entry of anthropogenic substances of the system (Négrel et al., Citation2014).
Fertilizers and pesticides are wastes produced from agricultural and livestock activities (Feria, Marrugo, & González, Citation2010), run-off or leaching phenomena entering coastal waters, and occasionally groundwater and sea. Pesticide contamination is of great concern due to their toxicity, long residual action, and high potential to accumulate in sediments, fatty tissues of aquatic organisms and humans (Lalah, Yugi, Jumba, & Wandinga, Citation2003). Such compounds exhibit a high physico-chemical stability, which is due to resistivity to photolytic degradation processes (photolysis), chemical degradation (hydrolysis, oxidation), and biological degradation (microorganisms) and travel long distances, presenting a high degree of dispersion (Aranguré, Zambrano, González, & Robledo, Citation2011; Santamarta, Citation2001). Hexachlorocyclohexane (HCH), lindane, the cyclodienes (aldrin, dieldrin, endrin, chlordane, heptachlor), endosulfan, dichlorodiphenyltrichloroethane (DDT), dicofol, methoxychlor, Toxaphene, and Mirex are compounds included within the classification of organochlorine pesticides, according of National Pesticide Information Center (NPIC).
The lake ecosystem of Cienaga Grande in the lower Sinú River, is located to the north of the Department of Córdoba in the Caribbean region of Colombia. The biophysical characteristics of this important natural freshwater source enable it to be one of the richest in the national territory for its plant biodiversity, associated by the presence of a total of 75 species of aquatic vascular plants from approximately 150 species that exist on the planet. This ecosystem is considered a trap of organic and inorganic substances (organochlorine compounds, heavy metals, etc.) that enter a continental affluent such as Sinú River, which flows mostly through agricultural areas where different types of pesticides are applied. There is an intense agricultural activity about the Cienaga Grande, mainly in the south area, and pesticides are used in an uncontrolled manner.
Pesticides can then migrate from their application sites by several routes, namely: (1) suspended in the air as aerosol particles carried by the wind to other areas during application, (2) by fixation by plants set aside for human food, (3) assimilation by animals and humans via food intake, and (4) fixation on soils and sediments. Current levels of contamination in sediments from Cienaga Grande in the lower Sinú River are unknown. Reported data are from 1993, in which the levels of some organochlorides (OCs) in sediments and soils were 2.33 × 10−3, 2.05 × 10−3, 5.20 × 10−2, 9.30 × 10−4, 4.66 × 10−3, 9.30 × 10−4, and 5.40 × 10−2 μg/kg for lindane, aldrin, dieldrin, endrin, 2,4-DDT, 4,4-dichlorodiphenyldichloroethane (4,4-DDD), and 4,4-DDT, respectively (Corporación Autónoma de los Valles del Sinú y San Jorge y Universidad de Antioquia, Citation1993). However, higher levels of OCs were found in clay sediments compared to sandy sediments (Botello, Rueda-Quintana, Díaz-González, & Toledo, Citation2000), demonstrating that monitoring of the levels of pollution of this area remains important because it is interconnected by caños (sea channels) in which seasonal wet and dry periods receive massive discharges of pesticides during route and may affect the natural habitat of species. On the other hand, owing to their high capacities for bioaccumulation and toxicities in organisms, these compounds pose threats to ecosystems and human health.
It is further proposed that the analysis of sediments should offer a unique help in the reconstruction of historical data analysis of OCPs based on descriptions of pollutants layers from the atmosphere and the basin drainage (Bigus, Tobiszewski, & Namieśnik, Citation2014). The purpose of this study was to determine the current contamination level of OCPs in surface sediments from four selected stations to evaluate the potential of pollution and hazard of sediments on Cienaga Grande in the lower Sinú River in Córdoba, Colombia. Moreover, the results obtained using a gas chromatograph (GC) coupled to an electron capture detector (ECD) as the technique for separation and analysis of samples are presented and discussed, although regional surveys continue to monitor OCPs residues in soils (Marrugo-Negrete, Navarro-Frómeta, & Urango-Cardenas, Citation2014), waters, and fish, few studies have documented the concentrations and spatial patterns of sediments for this area of the Caribbean region of Colombia.
2. Methods and materials
2.1. Study area and sampling
The wetland complex known as the Cienaga Grande in the lower Sinú River of Colombia is located in the northern part of the Department of Córdoba, in the Lower and Middle Sinú River subregions, in the right riverbank of the same name. This area was declared reserved: District of Integrated Management of Natural Resources (DIM) by the Governing Council of the Autonomous Regional Corporation of the valley of the Sinú and San Jorge (CVS), by decision number 76 on October 2007. It covers the territory of the municipalities of Lórica, Purísima, Momil, Chimá and Cotorra; an approximate area of 791 km2. Rainfall on the Cienaga Grande is highly seasonal, with a dry period between January and March, a rainy season between April and December. Since it is connected to the Sinú River it is an important area for the survival of surrounding municipalities mainly due to its function of damping the level of the river during the rainy season, which prevents excessive flooding in winter, thus obtaining an ecological condition through the exchange of waters between lentic type (the lakes) and lotic type (the river) ecosystems. Crops are clearly a major source of sediment and erosion that occurs in them and it is considered as the most polluting factor influencing water quality.
A total of 96 sediment samples were collected at 4 sampling stations selected in the Cienaga Grande area and labeled as: S1: between Purísima and San Sebastian sidewalk; S2: Momil; S3: Chimá west; and S4: Caño de Aguas Prietas. The locations of these selected sampling stations in the Cienaga Grande area are shown in Figure . Six samplings were carried out over a year covering wet (August, September, and October of 2010) and dry (February, March, and May of 2011) climatic transition seasons. Four samples of each station, in different cardinal points directions were collected, covering a radius of 3 m, to finally form a single representative composite sample of each station (Auer, Johnson, Penn, & Effler, Citation1996; Mejias & Jerez, Citation2006).
Figure 1. The study areas and sampling locations in the selected stations in Cienaga Grande of the lower Sinú River.
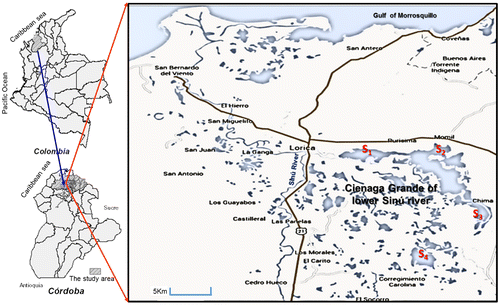
The upper 10 cm of the surface sediments were sampled from the sampling sites using a stainless steel “Van Veen” dredge, hand mare in the laboratory, which guaranteed a sufficient and representative surface sediment intake at each sampling point. Simultaneously, the salt content of the water was measured. Immediately after collection, the sediments were packaged in amber glass bottles with capacity of 1.0 kg, transported to the laboratory and preserved in a deep freezer at −20°C until analysis, to avoid degradation. In general, the sediments of the four stations were characterized by muddy textures (silts and clays) with absence of coarse fractions (sand and gravel). Sludge colors were classified as “medium dark grey” (N4) with colors “brownish-gray” (5YR 4/1) and “dark greenish-grey” (5G 4/1), according to Rock Color Chart, Geological Society of America (Citation2009).
2.2. Extraction, cleanup and analytical procedures
Sediment samples were homogenized and kept at room temperature for several minutes. Samples of sediments of 5.00 g (wet weight) were transferred to Whatman # 1 filter papers. OCPs were extracted for eight hours with Soxhlet apparatus, with 150 mL acetone-hexane (1:1 v/v). The extracts were then concentrated on a rotary evaporator at 45°C, 56.0 rpm, and 0.60 bar. The extracts were further treated with concentrated sulfuric acid to remove lipids and other polar substances. After this procedure, organic residues were concentrated to 5.0 mL and purified through a florisil column (1.5 g, 8.0 mm inner diameter). The OCPs were eluted with a mixture of 6% ethyl ether in hexane to collect the first fraction, which contained α-hexachlorocyclohexane (α-HCH), β-HCH, γ-HCH, and heptachlor epoxide. A second fraction was obtained after elution with a mixture of 15% ethyl ether in hexane to obtain aldrin, dieldrin, and endrin pesticides. The eluates were concentrated to about 1–2 mL on a rotary evaporator and then transferred to 10.0 mL glass tubes using small amounts of hexane.
The solvent in the glass tube was entirely evaporated under a gentle stream of nitrogen and the precipitates were dissolved in 1.0 mL hexane. Aliquots of 0.50 μL each of processed sample were injected into the Perkin-Elmer Autosystem XL GC (splitless mode) equipped with an ECD for the analyses. A fused silica capillary column RTX-5 (30.0 m length × 0.25 mm inner diameter, 0.25 μm film thickness, CAT. Supelco, supplied by Avantik Lab & Process, Springfield, NJ, USA)) was used for the separations. The injector and detector temperature were maintained at 200 and 300°C, respectively. The oven temperature was initially maintained at 100°C, and then programmed to increase at 15°C/min to 160°C and then ramped to 250°C at 3 C/min and this temperature was finally maintained for 20 min. Ultra-pure grade 5 (99.999%) helium and nitrogen gasses purchased and supplied by Linde Colombia, S.A. (Barranquilla, Colombia), were used as the carrier and make-up gasses, respectively. The carrier gas flow rate was 6.2 mL/min at 12.0 psi for optimum performance.
2.3. Quality assurance and validation of method
Quality assurance included instrument calibration using certified standards. A mixture of pesticides standards (M8081SC standard, supplied by AccuStandard, New Haven CT, USA) containing 12 OCs: aldrin, dieldrin, endrin, heptachlor epoxide, α-HCH, β-HCH, γ-HCH, 2,4-DDD, 2,4-DDT, 4,4-DDD, 4,4-DDE, and 4,4-DDT was used to prepare the standard solutions in HPLC-grade methanol and n-hexane (Courtney et al., Citation2003). A typical gas chromatogram of a mixture of the 12 OC pesticide standards is shown in Figure . The 12 pesticides were well resolved and eluted within 45 min. The correlation coefficients standard certificates remained above 0.990 in all runs. A standard solution was analyzed every 12 samples to verify instrument calibration.
The determination of the Method Detection Limit (MDL) included performing analysis of blanks: sediments of a reservoir located in Planeta Ric, Córdoba, Colombia free of OCPs. Aliquots of 0.5 μL were injected into the GC to establish the minimum concentration for each pesticide that could differentiate the noise/signal ratios of the instrument analyzing the standards in a decreasing order. The residues of OCPs were determined by matching their retention times to standards and were quantified using peak area integration. The correlation coefficients (R) for the OCPs calibration curves were all >0.998. Table shows recoveries (%), MDL values, and relative standard deviations (RSD) for OCPs found in sediments. The OCPs recoveries were determined relative to the ratio of direct injection of extract and the working standards prepared in HPLC-grade methanol and n-hexane. MDL values were calculated as three standard deviations of the mean from 12 blank solutions and ranged from 0.35 to 1.66 ng/g wet weight and spiked recoveries for the measured OCPs ranged 72.0 and 98.0%. One replica was performed for each site, and the RSD of OCPs in duplicate samples were less than 7% in all samples, as can be observed in Table .
Table 1. Percent recoveries, MDL, and RSD of analyzed results of M8081SC standards of OCPs in sediments
3. Results and discussion
3.1. Concentration profiles of OCPs
The concentrations detected of total OCPs in surface sediments varied in the range of 8.93–19.6 ng/g for the four stations monitored, as can be observed in Figure . The highest levels of α-HCH and lindane (common name for γ-HCH), were found at site S4 (7.05 and 7.57 ng/g, respectively), which is an area where the Caño de Aguas Prietas dumps the waters, and this stream is a body of running water moving to a lower level in a channel on land. The average proportions of HCH isomers measured in sediment samples were: α-HCH 50.3%, β-HCH 15.0%, and γ-HCH 34.7%. The α-HCH was found to be the most abundant HCHs in all samples, at 4.81 ± 0.56 ng/g mean concentration, followed by γ-HCH at 3.32 ± 0.74 ng/g average concentration of the four sites monitored. The detection frequencies of HCH compounds and their isomers in environmental sediment samples showed that contamination of HCH is widely distributed in the Cienaga Grande and the contamination of α-HCH can be attributed to the long-distance transport from other areas. Figure shows distribution of OCPs in sediments from Cienaga Grande in the lower Sinú River. α-HCH was detected in higher concentration in the stations S1 and S4, while γ-HCH was found in higher concentrations in stations S3 and S4 sites. Thus, in general the highest concentration of OCPs was on S3 and S4 sites, followed by S2 that correspond to the western town of Chimá, discharges of Caño de Aguas Prietas and Momil municipality, respectively (Table ).
Table 2. Average concentrations (ng/g) of wet weight and standard deviations of organochloride pesticides in sediments from Cienaga Grande of the lower Sinú River
In all cases, a higher concentration in sediments was observed. This is probably due to the accumulation of years as a result of water run-off from the surrounding areas, arriving at the Cienaga Grande during the winter time, implying in turn the increased pollution load on the site by discharges of wastewater and solid waste from various municipal centers and towns located about the location. The lowest concentrations of organochlorides measured in the sediment samples from the Cienaga Grande area corresponded to β-HCH and aldrin, which had average concentrations of 1.44 ± 0.34 and 1.20 ± 0.51 ng/g, respectively. Appreciably larger values (30.3 ± 1.87 ng/g for β-HCH and 29.1 ± 1.69 ng/g for aldrin) were found in surface sediment samples from the Yamuna River in Delhi, India (Pandey, Khillare, & Kumar, Citation2011). The OCPs in sediments samples of the four stations monitored from the Cienaga Grande area exceeded the permissible limits of 1.00 μg/L (sum of pesticide levels in drinking water). In accordance with Decree 1575 of 2007 and Resolution 2115 of 2007 of the Environment and Social Protection Ministries of Colombia for drinking water, individual pesticide level should not exceed 0.10 μg/L. In this study, the lack of legislation in Colombia on maximum accepted values of OCPs in coastal sediments became necessary in comparisons with other ecosystems and take the permissible value as reference.
Heptachlor levels were not found in sediment samples; however, heptachlor epoxide levels were detected at 1.84 ± 0.23 ng/g average concentrations. Heptachlor epoxide is not commercially available, but is an important metabolite of heptachlor in the environment, which is an oxidation product formed from heptachlor by many plant and animal species. Heptachlor was used extensively in the past for killing insects in homes, buildings, and on food crops, particularly corn. The persistence of OCPs in soil applied to cotton and corn crops has increased more than two decades ago suggesting that they could enter the food chain (Weaver, Ghadiri, Hulugalle, & Harden, Citation2012). Pesticides are applied during the production season until harvest. Fungicide and insecticide applications are usually applied together. Herbicides are typically applied immediately after planting. In this case, the high levels of OCPs residues that still exist in sediments of the Cienaga Grande area could be attributed to past agricultural applications and the fact that their degradation rates are slow. It is likely that these compounds are still used despite that they are banned.
According to official information from the CVS and Antioquia University (UDEA), in 1993 in Colombia, low concentrations of aldrin (2.05 × 10−3 μg/kg) in sediment samples were detected in the Cienaga Grande. In addition, according to the study of contamination by OCPs in the location waters of the low Sinú River valley, high concentration levels of pesticides were found in all stations sampled with a maximum of β-HCH (0.59 μg/L) at the station located west of Chimá (S3).
The detection frequencies of OCPs in sediments collected from the selected sites were 100% for α-HCH, β-HCH, lindane, and heptachlor epoxide, while that for aldrin was 80%. These compounds can be deposited into the sediments through long-range atmospheric transport. The detection frequency of α-HCH in the selected sites indicates that long-range atmospheric transport maybe the major source of α-HCH. Also, our results show that concentrations levels DDT and its degradation products DDE and DDD were not detected, indicating that most of the DDT residues migrated away from the surface sediments via run-off or were degraded following the prohibition of DDT two decades ago. Also, surface water run-off, soil erosion, and degradation processes maybe the main routes by which the DDT residues are removed from the sediment–water system.
According to previous results obtained by Lans (Lans et al., Citation2011) of the OCPs investigated in the Cienaga Grande of the lower Sinú River, aldrin was the only one found in the three matrices (water, sediment, and two fish species). The concentration of aldrin detected was about four and six times more in sediments than in the bocachico fish (Prochilodus magdelenae) 1.67 ± 1.26 ng/g and the moncholo (Hoplias malabaricus) 0.79 ± 0.28 ng/g wet weight. The two fish species were selected considering their commercial importance and their trophic relationship with the ecosystem. The concentration of aldrin found in sediments was seven times higher than in waters (0.16 ± 0.05 ng/g), according to the results obtained by Lans et al. (Citation2008). Figure shows the comparison of OCPs concentrations identified in waters (Lans et al., Citation2008) and sediments, with results of α-HCH, β-HCH, γ-BCH, and heptachlor epoxide at 48, 3, 55, and 6 times higher in sediments than in water, respectively. In all cases, a higher concentration was observed in the sediment samples. This is probably due to the accumulation of years as a result of water run-off from the surrounding area, arriving at the Cienaga during the winter season. On the other hand, HCHs and heptachlor epoxide were detected in both samples of sediments and waters from the Cienaga Grande. Higher concentrations in sediments with a positive and statistically significant difference were found. Therefore, the determination of OCPs by gas chromatography with electron capture detection gave higher concentrations for sediments compared to the equivalent water samples.
Figure 4. Comparison of concentrations of pesticides in water and sediments from Cienaga Grande in the lower Sinú River of Colombia.
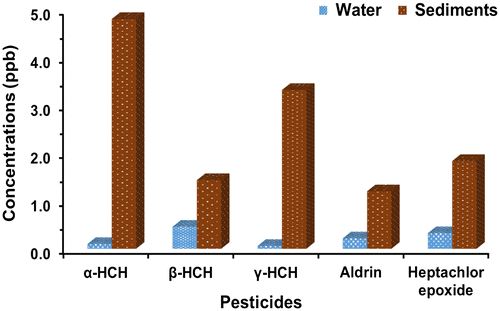
Other studies have detected levels of organochloride pesticides including HCH in soils, sediments, and waters of river ecosystems in various worldwide sites (Abdel-Sata, Goher, & Sayed, Citation2010; Camusso, Galassi, & Vignati, Citation2002; Fu et al., Citation2003; Glynn, Rumbold, & Snedaker, Citation1995; Marrugo-Negrete et al., Citation2014; Minh et al., Citation2007; Wolska, Zygmunt, & Namieśnik, Citation2003; Zhang, Hong, Zhou, & Huang, Citation2003). This is related to intensive farming for decades, pesticide-treated areas (Wasswa, Kiremire, Nkedi-Kizza, Mbabazi, & Ssebugere, Citation2011).
The various HCH isomers are highly toxic compounds and dangerous to humans. This implies the need for very restrictive regulations regarding production and use of HCHs. Over decades, a large quantity of heterogeneous mixtures of various isomers of HCH has been produced and dumped without control and concern to the environment (Calvelo, Monterroso, & Macías, Citation2008). α-, β-, γ-, and δ-HCH (positional HCH isomers) have been detected in sediments at Mount Pleasant, Florida (Site 17), Voorhees Township, New Jersey (Site 19), Wichita, Kansas (Site 28), Newport, Arkansas (Site 36), Cayce, South Carolina (Site 126), Pesticide Lab Yakima, Washington (Site 146), Swannanoa, North Carolina (Site 159), and Kimberton Borough, Pennsylvania (Site 189) of the 1,178 hazardous waste sites as of 7 April 2016, that have been proposed for inclusion on the EPA National Priorities List (U.S.D.H.H.S. Department of Health & Human Services, Citation2005). Studies have shown that residues of organochlorine pesticides can be adsorbed in sediments, mainly when the contents of humic acid and clay are very high (Alongi, Citation2002). In addition, these compounds are water soluble and can be adsorbed on particulate matter to be bioavailable to organisms living there (Ridolfi, Álvarez, & Girault, Citation2014).
High levels of pesticides were found in areas of San Bernardo del Viento, Moñitos and Playa Blanca, Cispatá in Córdoba, according to studies on organochlorine contaminants, for the period 1998–2000, where some values exceeded the concentration of 30.0 ng/L, with high accumulative potential (Negrete, Citation2005). Hence, sediment analysis plays an important role in monitoring the aquatic environment and provides information on the status and progress of pollution due to the ability of the sediment to accumulate contaminants. The results of the analyses of sediments samples from Cienaga Grande in the lower Sinú River of this investigation confirmed the presence of HCH isomers (α-HCH), β-HCH, γ-HCH), aldrin, and heptachlor epoxide of 12 pesticides monitored in the studied area.
The main environmental impacts of farming are related to soil compaction by use of heavy machinery, tillage of a field when it is too wet, accelerated erosion by inappropriate practices, soil and water pollution by indiscriminate use agrochemicals (pesticides, fertilizers, salts from irrigation), fragmentation of forests and landscapes, wildlife displacement, and affected human health, among others (PGAR 2002–2012, Citation2002). By destroying and draining wetlands to expand agricultural, livestock, and urban zone, landowners are disrupting ecological cycles and processes, and are depriving non-human organisms of the habitat that wetlands create. Fish, aquatic flora, and waterfowl, among others, depend heavily on wetlands. Therefore, these organisms are also being dispossessed form their natural location. The rural land titling and registry programs, pollution by dumping of domestic sewage, and high rates of sedimentation caused by clear cutting of natural forests in the upper of the basins are other important aspects of environmental impacts. All considerations listed above indicate an inappropriate handling affecting the ecosystem under study. According to Navarrete (Citation2007), there has been a considerable decrease in flood areas (water bodies) on the Cienaga Grande in the lower Sinú River, from 71.5 to 56.0%. Therefore, an estimated wetland loss of 15.5% has occurred because has been drained, dredged, filled, leveled, and flooded for urban, agricultural, and residential development, besides, vegetation becomes increasingly flooded, gradually loses vigor, and dies (see Figure ).
4. Conclusions
The results obtained in this study document the distribution of OCPs in sediment samples collected from the Cienaga Grande in the lower Sinú River, Department of Córdoba, in the Caribbean region of Colombia. Specifically, the OCPs: α-HCH, β-HCH, γ-HCH, aldrin, and heptachlor epoxide were monitored. The total concentration of OCPs in sediments obtained ranged from 7.40 × 10−2 to 19.61 ng/g wet weight, in the four stations monitored. The relatively high concentrations of OCPs found in the present study maybe attributed to the heavy use of these pesticides in weeds and pests control in crops around the Cienaga Grande in the lower Sinú River until recently. The ratios of α-HCH to γ-HCH positional isomers in the sediment samples for sampling stations S1, S2, S3, and S4 were 8.93, 1.73, 1.03, 0.93, respectively, suggesting earlier use of HCH and moreover, unfortunately tends to confirm the continuation use of banned pesticides as well. S3 and S4 showed results close to one, which indicates a recent source of contamination of lindane. The ratio obtained of α-HCH to γ-HCH for S1 and S2 was greater than one indicating significant differences at each station in the internal processing of the contaminants, despite being neighbors. The distribution patterns reflect the fact that contamination of the collected sediments is mainly caused by intense agricultural activity, which in turn led to the extensive use of OCPs.
The Sinú River flow increases during rainy season and the pollution loads are transported mainly to S3 and S4 stations. During this period, the major sources of organic pollution are industrial wastewater, run-off from agricultural fields in the middle-low Sinú River valley, and Sinú River effluents floodplains, garbage dumps and city streets carrying litter. In addition, each site also received run-off from local sources, especially from Lórica, Purísima, Momil, Chimá drains, therefore a high value was observed at S4. Although applications of persistent organic pollutants (POPs) have decreased in recent years, human exposure in countries such as Colombia continues because of the persistence and reuse of banned OCPs. Consequently, monitoring programs of POP concentrations in general population are needed to provide data to government authorities to implement policies for bioremediation. The research work carried out is expected to contribute to the general knowledge on the status of pollution due to OCPs because it will provide database for government authorities with the perspective of developing monitoring programs that will help minimize the risk of human exposure to these chemicals. The need for pesticide regulation and enforcement of such with respect to pest management, safeguarding users and consumers’ health, and the protection of the environment is a task that must be achieved.
Funding
This contribution was supported by the Center Research and Extension of the University of Cordoba, Colombia (CIUC). The authors also acknowledge financial support through contract FCB-06-07.
Additional information
Notes on contributors
Samuel P. Hernández-Rivera
Samuel P. Hernández-Rivera, PhD, is a professor of Physical Chemistry at the Chemistry Department of the University of Puerto Rico-Mayagüez Campus (UPRM). He is the director of the DoD-MURI sponsored Center for Chemical Sensors Development and the UPRM-based Chemical Imaging annd Surface Analysis Center and the UPRM Principal Investigator of the DHS ALERT Center of Excellence for Explosives Research. He also directs the DOD sponsored Chemical Imaging and Surface Analysis Center. He obtained his BS and MS degrees from UPRM and PhD from the Johns Hopkins University. His research interests are in IR/Raman spectroscopies in applied and fundamental studies and in stand-off and point detection of explosives, chemical and biological agents and other hazardous compounds using vibrational spectroscopy. Other interests are: environmental chemistry, SERS and in chemometrics enhancement of spectroscopic data and discriminant and cluster analysis of populations.
References
- Abdel-Sata, A. M., Goher, M. E., & Sayed, M. F. (2010). Recent environmental changes in water and sediment quality of lake Qarun, Egypt. Journal of Fisheries and Aquatic Science, 5(2), 56–69.
- Alongi, D. M. (2002). Present state and future of the world’s mangrove forests. Environmental Conservation, 29(03), 331–349.
- Aranguré, F. J., Zambrano, R. M., González, L., & Robledo, M. (2011). Evaluation of contamination levels by organochlorine pesticides residues on sediment and fishes from Aguamilpa reservoir, Nayarit, México. Waxapa, 3(1), 4.
- Auer, M. T., Johnson, N. A., Penn, M. R., & Effler, S. W. (1996). Pollutant sources, depositional environment, and the surficial sediments of Onondaga lake, New York. Journal of Environment Quality, 25, 46–55.10.2134/jeq1996.00472425002500010006x
- Betancourt, J. M., & Ramírez, G. (2005). Study of the processes related to the presence of organochlorides pesticides in the Cienaga Grande de Santa Marta. Boletín de Investigaciones Marinas y Costeras-INVEMAR, 34(1), 121–139.
- Bigus, P., Tobiszewski, M., & Namieśnik, J. (2014). Historical records of organic pollutants in sediment cores. Marine Pollution Bulletin, 78(1–2), 26–42.10.1016/j.marpolbul.2013.11.008
- Botello, A. V., Rueda-Quintana, L., Díaz-González, G., & Toledo, A. (2000). Persistent organochlorine pesticides (POPs) in coastal Lagoons of the subtropical Mexican pacific. Bulletin of Environmental Contamination and Toxicology, 64, 390–397.10.1007/s001280000013
- Calvelo, R., Monterroso, M. C., & Macías, F. (2008). El hexaclorociclohexano (HCH). Edafología, 15(1–3), 3–24.
- Camusso, M., Galassi, S., & Vignati, D. (2002). Assessment of river Po sediment quality by micropollutant analysis. Water Research, 36(10), 2491–2504.10.1016/S0043-1354(01)00485-7
- Corporación Autónoma de los Valles del Sinú y San Jorge y Universidad de Antioquia. (1993). Estudio Ecológico y Ambiental Ciénaga Grande de Lorica (p. 250p). Montería: Author.
- Corporación Autónoma Regional de los Valles del Sinú y del San Jorge. (2002). Plan de Gestión Ambiental Regional. PGAR 2002–2012. Montería: Author.
- Courtney, D., Andreas, S., Mark, D., John, R., Vincent, L., Alyson, L., … Donald, G. (2003). Comprehensive solid-phase extraction method for persistent organic pollutants. Validation and application to the analysis of persistent chlorinated pesticides. Analytical Chemistry, 75(1), 71–77.
- El Nemr, A., Moneer, A. A., Khaled, A., & El-Sikaily, A. (2013). Levels, distribution, and risk assessment of organochlorines in surficial sediments of the Red Sea coast, Egypt. Environmental Monitoring and Assessment, 185(6), 4835–4853.10.1007/s10661-012-2907-3
- Espinosa, L. F., & Ramírez, G. (1995). Análisis de residuos organoclorados en los sedimentos de zonas de manglar en la Ciénaga Grande de Santa Marta y la Bahía de Chengue, Caribe colombiano. Anales del Instituto de Investigaciones Marinas de Punta de Betin, 24, 79–94.
- Feria, J., Marrugo, J., & González, H. (2010). Heavy metals in Sinú River, Department of Córdoba, Colombia, South America. Revista de la facultad de Ingeniería de la Universidad de Antioquia, 55, 35–44.
- Fu, J., Mai, B., Sheng, G., Zhang, G., Wang, X., Peng, P., … Wa, Tang U. (2003). Persistent organic pollutants in environment of the Pearl River Delta, China: An overview. Chemosphere, 52, 1411–1422.10.1016/S0045-6535(03)00477-6
- Garay, J. A. (2001). Fuentes de contaminación de origen terrestre y marítimo que afectan las zonas marino costeras del Caribe y Pacífico colombiano. Vías de entrada a los sistemas marinos y su impacto. Diagnóstico y evaluación de la calidad ambiental marina en el Caribe y pacífico colombiano. Red de vigilancia para la protección y conservación de la calidad de las aguas marinas y costeras. Informe final. Tomo I (31 p).
- Geological Society of America. (2009). Rock color chart: Grand Rapids, MI. The Geological Society of America, Rev., 2009, 10p.
- Glynn, P. W., Rumbold, D. G., & Snedaker, S. C. (1995). Organochlorine pesticide residues in marine sediment and biota from the northern Florida reef tract. Marine Pollution Bulletin, 30, 397–402.10.1016/0025-326X(94)00206-O
- Gómez, S., Martínez, C., Carbajal, Y., Martínez, A., Calderón, M., Villalobos, R., & Waliszewski, S. (2013). Riesgo genotóxico por la exposición ocupacional a plaguicidas en América Latina. Revista Internacional de Contaminación Ambiental, 29, 159–180.
- INVEMAR. (2001). Informe del Estado de los ambientes marinos y costeros en Colombia: 2000. Serie Documentos Generales. No. 3 (138 p). Santa Marta.
- Lalah, J. O., Yugi, P. O., Jumba, I. O., & Wandinga, S. O. (2003). Organochlorine Residues in Tana and Sabaki Rivers in Kenia. Bulletin of Environmental Contamination and Toxicology, 71, 298–307.
- Lans, E., Díaz, B., & Páez, M. (2011). Compuestos organoclorados residuales en dos especies ícticas de la Ciénaga Grande del Bajo Sinú, Córdoba, Colombia. Revista MVZ Córdoba, 16(1), 2402–2409.
- Lans, E., Marrugo, J. L., & Díaz, B. (2008). Estudio de la contaminación por pesticidas organoclorados en aguas de la Ciénaga Grande del Valle Bajo del río Sinú. Temas Agrarios, 13(1), 49–56.
- Marrugo-Negrete, J. L., Navarro-Frómeta, A., & Urango-Cardenas, I. (2014). Organochlorine pesticides in soils from the middle and lower Sinú River Basin (Córdoba, Colombia). Water, Air, & Soil Pollution, 225, 65.10.1007/s11270-014-2053-3
- Mejias, J., & Jerez, J. (2006). Guía para la toma de muestras de residuos de plaguicidas agua, sedimento y suelo (34 p.). Santiago de Chile: Instituto de Investigaciones Agropecuarias. Centro Regional de Investigación Carillanca.
- Minh, N. H., Minh, T., Iwata, H., Kajiwara, N., Kunisue, T., Takahashi, S., … Tanabe, S. (2007). Persistent organic pollutants in sediments from Sai Gon–Dong Nai River Basin, Vietnam: Levels and temporal trends. Archives of Environmental Contamination and Toxicology, 52, 458–465.10.1007/s00244-006-0157-5
- Muñoz, A. F. (2009). Evaluación del daño en el ADN en dos poblaciones colombianas de agricultores y floricultores. Actualidad y Divulgación Científica, 12, 7–16.
- Navarrete, F. (2007). Diseño de Políticas Ambientales Corporativas de la CVS. Política Ambiental de los Humedales del Departamento de Córdoba. Informe final. Convenio CVS-CI No. 083 de 2005. Montería.
- Négrel, P., Merly, C., Gourcy, L., Cerdan, O., Petelet-Giraud, E., Kralik, M., & Klaver, Gerard (2014). Soil-sediment-river connections: Catchment processes delivering pressures to river catchments. Risk-Informed Management of European River Basins (pp. 21–52). Berlin Heidelberg: Springer.10.1007/978-3-642-38598-8
- Negrete, V. (2005). Las fuentes de agua en el departamento de Córdoba: Un inventario desalentador, 1952–2000. Montería: Universidad del Sinú.
- Pandey, P., Khillare, P., & Kumar, K. (2011). Assessment of organochlorine pesticide residues in the surface sediments of River Yamuna in Delhi, India. Journal of Environmental Protection, 2(5), 511–524.10.4236/jep.2011.25059
- Ridolfi, A. S., Álvarez, G. B., & Girault, M. E. (2014). Organochlorinated contaminants in general population of Argentina and other Latin American Countries. In Bioremediation in Latin America (pp. 17–40). Springer International Publishing.
- Santamarta, J. (2001). World Watch. Contaminantes Orgánicos Persistentes (COPs). Boletín Informativo, 38.
- U.S.D.H.H.S. Department of Health and Human Services. (2005). Toxicological profile for Alpha-, Beta-, Gamma-, and Delta-Hexachlorocyclohexane (354 p.). Atlanta, GA: Agency for Toxic Substances and Disease Registry. Last updated on March 3, 2011. Retrieved February 15, 2015
- Wasswa, J., Kiremire, B., Nkedi-Kizza, P., Mbabazi, J., & Ssebugere, P. (2011). Organochlorine pesticide residues in sediments from the Uganda side of Lake Victoria. Chemosphere, 82, 130–136.10.1016/j.chemosphere.2010.09.010
- Weaver, T. B., Ghadiri, H., Hulugalle, N. R., & Harden, S. (2012). Organochlorine pesticides in soil under irrigated cotton farming systems in Vertisols of the Namoi Valley, north-western New South Wales, Australia. Chemosphere, 88(3), 336–343.10.1016/j.chemosphere.2012.03.008
- Widenfalk, A. (2002). Pesticide bioavailability in aquatic sediments: A literature review. Uppsala: Inst. för miljöanalys, SLU, IMA Rapport, Department of Environmental Assessment Swedish University of Agricultural Sciences. Sveriges lantbruksuniv.
- Wolska, L., Zygmunt, B., & Namieśnik, J. (2003). Organic pollutants in the Odra river ecosystem. Chemosphere, 53, 561–569.10.1016/S0045-6535(03)00368-0
- Zhang, Z. L., Hong, H. S., Zhou, J. L., & Huang, J. (2003). Fate and assessment of persistent organic pollutants in water and sediment from Minjiang River Estuary, Southeast China. Chemosphere, 52, 1423–1430.10.1016/S0045-6535(03)00478-8