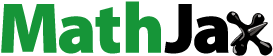
Abstract
Evaluation of concentration of heavy metals found in shooting range soil is important in assessing the pollution risk posed to biota. Soil samples from five shooting ranges found in Botswana were used in this study. All the five shooting ranges accumulated high concentration of Cu ranging from 67.4 ± 0.05 mg/kg to 1569 ± 13 mg/kg followed by Mn (25.9 ± 0.1–953.8 ± 2.8 mg/kg). Pollution risk indices were used to quantify the environmental pollution risk posed by the different heavy metals studied. It was established that even though all the five shooting ranges recorded low concentrations of Cd, this metal still posed the highest pollution risk than any other metal with S/P shooting range recording the highest potential ecological risk index (peri) of 8141 (CCd ~ 3.6 ± 0.03 mg/kg) and TAB at PERI of 3507 (CCd ~ 4.9 ± 0.02 mg/kg). Similarly, contamination factor (CF) value of 271 for Cd was measured at S/P shooting range indicating high contamination from Cd. Pollution risk indices were able to establish that even though concentration of Cd accumulated in the soil was low this heavy metal still posed highest pollution risk to biota. Continuous assessment of the pollution status of these shooting ranges should be carried out in order to establish appropriate best shooting range management practices and remedial strategies.
PUBLIC INTEREST STATEMENT
This study is the first in Botswana in assessing pollution in shooting range soils from ammunition. Shooting ranges are a source of environmental pollution since toxic heavy metals are major constituents of bullets. Concentrations of toxic metals in the five military shooting ranges that have been in operation for over 30 years exceeded the world health organization (WHO) set limits. These toxic metals can be absorbed by plants endangering humans and animals that consume them. Adverse health effects such as respiratory and kidney problems can result from exposure to these hazardous metals. Most of the shooting ranges studied are not fenced making them accessible as grazing fields. Surface and underground water pollution is possible resulting in these toxic metals finding their way into the food chain. Therefore remedial measures such as cleaning of contaminated soils and fencing of shooting ranges should be carried out as soon as possible.
Competing Interests
The authors declares no competing interests.
1. Introduction
Heavy metals such as arsenic (As), antimony (Sb), cadmium (Cd) and zinc (Zn) present multi-element detrimental effects to biota (Seshadri et al., Citation2017). Shooting ranges have been found to be among the main sources of heavy metal pollution of the environment. Shooting ranges are the main source of environmental lead (Pb) pollution after the battery industry (Sehube et al., Citation2017) and most if not all heavy metal pollution of shooting range soil has focused on Pb (Mariussen, Johnsen, & Strømseng, Citation2017). This is due, in part, to the fact that ammunition contains an excess of 80% Pb slug and small traces of As, Sb, Cd, Cu, Ni, Mn and Zn whose environmental impact is rarely studied (Hockmann et al., Citation2015). Ammunition shots and bullets have been found to contain 95–97% metallic Pb by weight, 0.4–2.0% Sb, 0.2–0.8% As and mean concentrations of Sn, Se, Mn, Cd, Cr, Cu and Ni greater than 30 mg/kg (Fayiga & Saha, Citation2016). Pb is mainly found in the core of bullets and shots while Cu, Zn and Sb are constituents of bullet ore heads and cartridges (Mariussen et al., Citation2017; Urrutia-Goyes, Argyraki, & Ornelas-Soto, Citation2017).
Copper, zinc and nickel are essential elements in plants. Copper is a constituent of enzymes and plays a significant role in photosynthesis (Lange et al., Citation2017). Zinc Nickel is a component of the enzyme urease which plays an important function in seed germination (de Queiroz Barcelo et al., Citation2017). However, increased concentrations of these biologically important trace elements can have detrimental effects to biota. Accumulation of Zn in plants inhibits the uptake of iron and copper leading to pale yellow interveinal chlorosis on the leaves (Broadley, White, Hammond, Zelko, & Lux, Citation2007). Exposure to antimony causes respiratory problems such as chronic bronchitis, chronic emphysema, inactive tuberculosis, pleural adhesions and respiratory irritation characterized by chronic coughing, wheezing and upper airway inflammation (Sundar & Chakravarty, Citation2010). The adverse health effects associated with exposure to As include replacing other elements in plant enzymes leading to plant stunted growth and eventual death (Nagajyoti, Lee, & Sreekanth, Citation2010). Adverse health effects associated with exposure to arsenic (As) also include cardiovascular, diabetes and cancer as usually reaches the blood stream through ingestion, inhalation and skin contact(Alexander, Gulledge, & Han, Citation2016). Cardiovascular effects due to exposure to Sb in humans include increased blood pressure and alteration of electrocardiography (ECG). Abdominal pain, diarrhoea, vomiting, and ulcers are some of the gastrointestinal effects associated with exposure to Sb (Okkenhaug et al., Citation2013). High level of Cd causes decrease in seed germination, stunted growth in plants, disrupt enzyme activities and makes plants prone to fungal invasion (Laghlimi, Baghdad, El Hadi, & Bouabdli, Citation2015). Its compounds relatively have high solubility in the soil making Cd more mobile and bioavailable (Sharma & Sachdeva, Citation2015).
Copper (Cu) is an essential element to plants, however, high levels of copper can lead to disruption of plant photosynthesis and reproduction processes (Laghlimi et al., Citation2015). High concentrations of Cu have adverse health effects to the gastrointestinal tract and the liver. Pulmonary fibrosis and increased vascularity have also been reported in people exposed to high levels of copper (Laghlimi et al., Citation2015). Low concentration of Cu has been reported to cause brain damage in animals (Department of Water Affairs and Forestry, Citation1996). Nickel is a known epigenetic carcinogen and can cause genetic modifications (Brocato & Costa, Citation2017). Physicochemical processes in the soil such as physical weathering, oxidation, sulfonation, dissolution and precipitation transform these heavy metals into the mobile and bioavailable forms that are detrimental to the biota (Kelebemang et al., Citation2017). Antimony is the second largest shooting range pollutant after Pb comprising 7% of bullet shot and bullet (Rooney, McLaren, & Cresswell, Citation1999). Sb concentration of 13,800 mg/kg has been found in a Swiss shooting range (Johnson, Moench, Wersin, Kugler, & Wenger, Citation2005). Elevated concentrations of 5200 mg/kg, 1100 mg/kg and 830 mg/kg corresponding to Cu, Zn and Sb have been established at a Norwegian shooting range (Mariussen et al., Citation2017). These high concentrations of heavy metals were able to find their way into the water sources with concentrations of Zn, Cu and Sb in surface water measuring 1.6, 0.9 and 0.15 mg/l respectively (Mariussen et al., Citation2017). Other such high pollution from heavy metals such as Cu, Cd, Zn, Sb, As and Ni other than Pb emanating from ammunition have been found in Canada (Lafond, Blais, Martel, & Mercier, Citation2013), Spain (Rodríguez-Seijo, Alfaya, Andrade, & Vega, Citation2016), Switzerland (Evangelou, Hockmann, Pokharel, Jakob, & Schulin, Citation2012), South Korea (Lee et al., Citation2002) and the United States of America (Sehube et al., Citation2017). Most of these shooting range soils accumulated heavy metals concentrations far exceeding the USEPA critical limits. Heavy metal contamination of shooting range soils also poses risk of surface and underground water pollution (Wersin, Johnson, & Furrer, Citation2002). Concentrations of Sb as high as 1.59 mg/L have been reported in water found in close proximity to a shooting range (Okkenhaug et al., Citation2013). The accumulation of heavy metals into the soil which in turn may bind to the soil organic matter and eventual dissolution thereof has the potential to negatively affect the soil carbon sequestration process exacerbating the climate change phenomena (Jastrow, Amonette, & Bailey, Citation2007)
Heavy metals uptake by plants has also been reported leading to heavy metals finding their way into the food chain (Mozafar et al., Citation2002; Robinson et al., Citation2008). Elevated heavy metal concentrations of 25 mg/kg and 50 mg/kg for Cu and Ni respectively, have been found in plant species, which are a source of food for livestock (Robinson et al., Citation2008). Nine plant species in two decommissioned shooting ranges have been evaluated for their Cu, Zn, Sb and Cd concentrations and the potential health risk to animals made to graze in such shooting ranges (Evangelou et al., Citation2012). At a clay pigeon shooting range in Tuscany (central Italy) high concentrations of Sb, Ni, Zn, Mn and Cu were found in arthropod communities inhabiting shooting range soils (Migliorinia, Pigino, Bianchi, Bernini, & Leonzio, Citation2004). A reduction in soil enzymatic activity has also been reported in soils contaminated with heavy metals such as cadmium can have a negative effect in the soil chemistry due to its harmful effect to the microorganisms found in the soil which are important to soil fertility (Vig, Megharaj, Sethunathan, & Naidu, Citation2003). The mobility, bioavailability and bioaccessibility of heavy metals in shooting range soils is dependent on the physicochemical properties of the soil such as soil pH, cation exchange capacity (CEC), soil organic matter and moisture (Kelebemang et al., Citation2017). Under favourable conditions of soil properties the chemical weathering, solubility, mobility and bioavailability of heavy metals in shooting range soils can be enhanced (Ma, Hardison, Harris, Cao, & Zhou, Citation2007).
To the best of our knowledge no research has been carried out on heavy metal pollution of shooting range soils in Botswana except Pb, a study that was carried out by our research group. Botswana has shooting ranges all over the country that are used for shooting practices by the military, police services, prisons services and department of wild life national parks. This study follows our pioneering study that looked into pollution of shooting range soils from Pb which is the main constituent of ammunition (Sehube et al., Citation2017). Our pioneering study found elevated Pb pollution in shooting range soils with highs of up to 38 406.87 mg/kg far exceeding the set USEPA critical limit of 400 mg/kg Pb in soil (Sehube et al., Citation2017). Evaluation of the extent of shooting range soil pollution from heavy metals can help strengthen best management practices of shooting ranges. Soil remedial processes can also be employed for soil cleaning and reclamation. Based on the extent of heavy metal pollution, soil remediation methods and techniques such as chemical immobilization, physical soil excavation, phytoremediation and chemical washing techniques have been utilized in fighting shooting range soil heavy metal pollution (Sorvari, Antikainen, & Pyy, Citation2006). The objectives of this study include (1) quantitative evaluation of heavy metal pollution of shooting range soils found in Botswana (2) environmental pollution risk assessment of such pollution based on such methods as potential ecological risk index (PERI), heavy metal pollution load index (RI) and contamination factor (CF). The findings of this study will provide a baseline data for heavy metal pollution of shooting range soils in Botswana and will also provide a basic measure on the extent of heavy metal pollution emanating from similar ammunition used elsewhere around in world. Appropriate remedial strategies and methods may also be suggested based on the extent of pollution encountered in the studied shooting ranges and shooting ranges using same type of ammunition around the world. In addition best shooting range management practices for future shooting range users will be suggested.
2. Materials and methods
2.1. Location and description of shooting ranges
Five military shooting ranges scattered across Botswana were used in this study. These shooting ranges are mostly used for small arms shooting practices using rifles of calibres 0.50”, 5.56 mm, 7.62 mm, 7.65 mm and 9 mm. It is important to note that small arms ammunition contain predominantly the heavy metals under study. The five shooting ranges were Thebephatshwa (TAB) located in the southern part of the country (GPS coordinates: 24° 14ʹ 42.79” South, 25° 19ʹ 55.84” East), Shoshong (SHO) in central Botswana (GPS coordinates: 23° 2ʹ 8.28” South, 26° 30ʹ 7.12” East), Selibe Phikwe (S/P) in eastern Botswana (GPS Coordinates:27° 50ʹ 14.39” East, 21° 57ʹ 58.55” South), Matsiloje, (MAT) with GPS coordinates: 21° 22ʹ 12.00” South, 27° 52ʹ 12.00” East in north eastern Botswana and lastly Pandamatenga (PANDA) located in northern Botswana (GPS coordinates 18° 31ʹ 57.01” South, 25° 39ʹ 14.22” East). The five shooting ranges and their year of establishment are shown in Figure ) below. It is important to note that all these shooting ranges have been in operation for the past 16–32 years and that there has never been a single study carried out on possible pollution risk from heavy metal accumulation in these shooting ranges. Two of the shooting ranges studied are located in fenced military camps while the other three occur in open areas acting as grazing fields for livestock and small stock.
2.2. Soil sampling procedure and techniques
Berm soil samples were collected to a depth of 20 cm using a soil recovery probe in all the five the shooting ranges. This soil represents the surface layer (0–5 cm), the subsurface layer (5–10 cm) and the deeper soil layer (10–20 cm). The berm accumulates the highest proportion of bullets and shots since it acts as a barrier for bullets and shots after they have hit the target. The berm section of the shooting range was divided into three segments being; berm-upper, berm-middle and berm-bottom (Figure ). The berm was divided into three sections because some bullets and shots can lend at the bottom, or middle or upper section of the berm. Three circular sampling areas of radius 150 cm were made at the upper, middle and bottom of the berm along a central transect. In addition, four samples were collected from the three sampling points within the sampling area to make three composite samples representative of the upper, middle and bottom berm soils (Figure ). This sampling technique was preferable because it covers a large area making the sample a true representation of the study site. Control soil samples which served as background samples were collected at a distance of 200 m away from the five shooting ranges. The collected samples were then stored in butyrated zip-lock plastic bags for transportation to Botswana University of Agriculture and Natural Resources (BUAN) for further sample treatment and analysis.
2.3. Soil sample preparation and chemical analysis
The three quadruplicate samples from sampling points in three sampling areas of berm-upper, berm-middle and berm-bottom were mixed together to obtain three composite samples corresponding to each berm section. A total of nine berm soil samples were obtained from each range making a grand total of forty-five soil samples. The soil samples were air dried for a day after which a 2 mm stainless steel sieve was used to remove spent bullets, stones and rock pebbles, dry tree leaves and small tree barks. Soil sample digestion was achieved using the dry heating-block digestion procedure (USEPA Method 3050) by taking a 0.500 g of soil samples in 100 mL of 1:3 HNO3/HCl mixture for two hours. After sample digestion total Cd, Cu, Ni, As, Sb and Zn concentrations were analysed using inductively coupled plasma optical emission spectrometry (ICP-OES; Perkin Elmer, Optima 2100 DV). Quality check and control was done using certified reference material (CRM) of soil, NCS DC 73,320, obtained from the Botswana Geoscience Institute (BGI).
2.4. Physicochemical properties of shooting range soils
The physicochemical properties of the soil such as pH, electrical conductivity (EC), organic matter (OM) and cation exchange capacity (CEC) were determined using standard methods. A mixture of 0.01 M CaCl2 solution and 40 mL of deionized water were added to 20 g of soil sample and pH measurements were taken using a calibrated crimson basic 20 pH meter at 25°C. The Walkley-Black procedure was used for the determination of the organic matter (OM) content as reported in the literature (Nelson & Sommers, Citation1982). The soil electrical conductivity (EC) was carried out in the ratio of 1:2 soil to water using the HACH SenseIon EC7 electrical conductivity meter (Tchoumou, Kami-Ouako, Mbilou, & Ossebi, Citation2017).
2.5. Environmental risk assessment of pollution from heavy metals
2.5.1. Potential ecological risk index
Potential ecological risk index (PERI) method was first used by Hakanson in 1980 (Hakanson, Citation1980). This method provides a powerful tool for estimating environmental pollution by combining the ecological and environmental impacts with toxicology (Ke et al., Citation2017). The potential ecological risk index of a single heavy metal (EiR) is given by Equation (1) below while Equation (2) evaluates the ecological risk (RI) emanating from contributions by all the toxic heavy metals present in the soil. The TiR signifies the biological toxic response factor of an individual metal while Ci and C0 are concentrations of individual heavy metal and its background concentration respectively. The TiR values of the heavy metals under study are Cd = 30, Cu = Ni = 5 and Mn = Zn = 1 (Ke et al., Citation2017; Soliman, Nasr, & Okbah, Citation2015). Table below categorises the potential ecological risk indices with corresponding pollution risk level.
Table 1. Categories of PERI and RI indicating the pollution risk level of heavy metals to the soil (Ke et al., Citation2017)
2.5.2. Contamination factor
Assessment of pollution level can also be carried out using other pollution indicators such as the contamination factor (CF), given in Equation (3) below, where Ci and Cb are the mean concentrations of the heavy metal under investigation and its background concentration respectively. This represents the contamination level of an individual target heavy metal. Contamination factor, CF, correlates the background concentration of heavy metals in the soil with contributions from anthropogenic activities. The classification for contamination factor values and the corresponding degree of pollution are shown on Table below.
Table 2. Classification of contamination factor and corresponding level of soil contaminate on from heavy metal accumulation (Ke et al., Citation2017)
The resultant overall integrated contamination given by the different target metals in the soil is evaluated using the pollution load index (PLI), given by Equation (4) below. It is determined by calculating the nth root of the n contamination factors (CFn) multiplied together (Cao et al., Citation2015)
The PLI > 1 denotes polluted soil while PLI < 1 indicates no heavy metal pollution (Cao et al., Citation2015)
2.6. Statistical analysis of data
A statistical analysis technique such as the Pearson correlation coefficient (r) was used to evaluate the concomitant contributions towards heavy metal pollution from the soil physical and chemical properties as shown on Table below. Pearson correlation coefficient can also help ascertain if the heavy metal pollutants under investigation have common anthropogenic source.
Table 3. Pearson correlation coefficient range indicating the possible correlation between the properties of the soil and degree of pollution (Pejman, Bidhendi, Ardestani, Saeedi, & Baghvand, Citation2017)
3. Results and discussion
3.1. Physicochemical properties of shooting range soils
The soils in the all the five shooting ranges studied experienced neutral to slightly alkaline pH. Shooting ranges such as S/P and SHO experienced neutral pH values of 6.7 and 6.9 respectively (Table ). On the other hand, PANDA, MAT and TAB soils were slightly alkaline recording pH values of 8.3, 8.5 and 8.6 respectively. The soil pH values found in the five shooting ranges are consistent with the soil pH for the respective areas in which the shooting ranges are found (Ekosse & Anyangwe, Citation2012; Joshua, Citation1991). For example, in PANDA, the soil type is mainly dark clay vertisols with a pH range of 6.5 to 9.0 (Almendros et al. Citation2003; Moganane, Van Waveren, & Remmelzwaal, Citation1991). The alkaline soil pH of 8.3 found at the MAT shooting range is consistent with the alkaline soil pH of the soils found in the Matsiloje area which can reach pH values of 9.0 (Mitchell, Citation1976). The organic matter (OM) found in the five shooting ranges was in the range 0.54–1.88% with S/P soils experiencing the highest OM relative to the other four shooting ranges. The soil organic matter found at the five shooting ranges studied is consistent with average organic matter content of (0.5−1.9%) for the soils found in the study sites (Almendros et al. Citation2003; Joshua, Citation1991; Pardo, Ristori, D’acqui, & Almendros, Citation2003). For example, at the PANDA shooting range, organic matter of 0.54% was measured and previous studies found similar organic matter content in the Pandamatenga area in which the PANDA shooting range is located (Moganane et al., Citation1991). The soils electrical conductivity (EC) were in the range 33.9 ± 3.3 to 208.9 ± 8.8 µS/cm and the highest electrical conductivity was recorded at S/P shooting range soil (Table ). The organic matter and pH measurements at the five shooting ranges are within range of our preliminary studies on Pb pollution in the same shooting ranges (Kelebemang et al., Citation2017; Sehube et al., Citation2017).
Table 4. Physical and chemical properties of shooting range soils
3.2. Total heavy metal concentration in shooting range berm soils
The berm soils of all the five shooting ranges accumulated highest concentrations of Cu in the range 67.4 ± 0.05 mg/kg to 1569 ± 13 mg/kg followed by Mn (25.9 ± 0.1–953.8 ± 2.8 mg/kg) as shown on Figure –c) below. On average, the berm soil of TAB shooting range collected the highest concentration of Cu (1190.7 mg/kg) compared to the other shooting ranges. On the other hand, all the shooting ranges investigated experienced lower concentrations of Cd of the range 0.2 ± 0.08–5.0 ± 0.06.4 mg/kg. The lowest concentration of Cd (0.2 ± 0.08 mg/kg) was recorded at MAT shooting range. The degree of pollution at a shooting range correlates to a larger extent with the frequency of shooting range use than the duration period the shooting range has been in use. TAB shooting range, even though started operation in 1995, accumulated the highest concentration of Cu compared to the other shooting ranges studied that were established some years earlier such as MAT (1985) and PANDA (1987) with measured concentrations of Cu of 390.8 ± 0.6 mg/kg and 888.5 ± 2.3 mg/kg respectively. As shown in our earlier studies (Sehube et al., Citation2017), TAB shooting range accumulated the highest concentration of Pb (38 386 ± 10 197 mg/kg) due to its frequent use by the Special Forces commando squadron based at the Thebephatshwa air base. The results from our previous studies correlate well with our current study. The middle of the berm accumulated the highest concentration of heavy metals compared to the upper and bottom sections of the impact berm. For an example, TAB shooting range experienced concentrations of 1569 ± 13, 1490.7 ± 9.1 and 512.3 ± 0.5 mg/kg of Cu at the middle, upper and lower part of the impact berm respectively. One of the reasons for this observation is that when shooting at the targets, most of bullets and shots accumulate in the middle of the impact berm making this section of the berm have the highest density of bullets and shots resulting in higher concentrations of heavy metals around the same area. MAT shooting range acquired the highest average concentration of all the five studied heavy metals. Previous studies have discovered elevated concentrations of heavy metals in berm soils of shooting ranges. A study by Islam, Nguyen, Jung, and Park (Citation2016) found elevated concentrations of Cu at four times higher than the allowed Korean maximum contaminant level (MCL) of 150 mg/kg and Cd was found to be two times higher than the Korean warning level (Islam et al., Citation2016). Similar studies found that concentrations of Cu and Ni in shooting range berm soils far exceeded the Dutch Intervention Values (DIV) of 190 mg/kg and 210 mg/kg respectively (Robinson et al., Citation2008). It is important to note that this study is the first of its kind in Botswana and that there is no local information in the literature for heavy metals pollution in shooting range soils that could be used for comparisons. As a result, the findings obtained in this study were therefore used to investigate the extent of shooting range soils pollution from heavy metals Cd, Cu, Mn, Ni and Zn. A previous study by Mmolawa, Likuku, and Gaboutloeloe (Citation2011) in the Matsiloje area where the MAT shooting range is found was able to measure soil background concentrations of 0.97 mg/kg Mn; 0.50 mg/kg Ni; 0.11 mg/kg Cu and 0.34 mg/kg Zn. In comparison, the concentrations of Cu, Mn, Ni and Zn found at MAT shooting range soil are much higher than these background concentrations, an indication that heavy metals from ammunition contributed to these high amounts of heavy metals deposit into shooting range soils. For example, the average concentration of Cu in the berm soils of MAT (390.8 ± 0.6 mg/kg) shooting range is 3500 times higher than the background concentration while the Ni concentration (44.7 ± 0.2 mg/kg) at the same shooting range was 90 times higher than the background concentrations. Quality control was performed using a soil certified reference material, NCS DC 73,320. A percentage recovery of over 90% was obtained for all the five heavy metals studied with accuracy of measurements within <3% RSD.
3.3. Effect of pH, organic matter and electrical conductivity on the distribution of heavy metals in shooting range soils
The soil properties such as pH, organic matter (OM) and electrical conductivity (EC) may have had an effect on such elevated levels of heavy metals at MAT shooting range. The alkaline soils (pH 8.6) at TAB shooting range provided for a suitable environment for the corrosion of the elemental heavy metals under study (Table above). Concentrations of heavy metals Cu and Mn of up to 1569 ± 23 mg/kg and 184 ± 0.9 mg/kg respectively were recorded at TAB shooting range. At elevated pH, oxides, hydroxides, carbonates, sulphates and phosphates of the heavy metals under study become insoluble, thereby increasing their retention time in the soil. Their mobility become greatly reduced at elevated pH levels (Sporting Arms and Ammunition Manufacturers’ Institute [SAAMI] Citation1996). Previous studies have found that soils with pH values of ≥ 7.0 experience restricted mobility of heavy metals whereas soils with pH values of ≤ 6.5 undergo enhanced metal mobility (SAAMI Citation1996). Our findings corroborate our previous studies where it was found that alkaline soils increased the stability of heavy metal oxides such as Pb oxides and carbonates (Sehube et al., Citation2017). A study by Ma et al. (Citation2007) found out that as soil pH increased the weathering processes of Pb shot was greatly reduced leading to a decrease in mobility of Pb. The amount of organic matter (OM) present in the soil has been found to provide a suitable environment for the weathering of bullets and shots. Organic matter helps in the transformation of heavy metals present in shots and bullets into soluble complexes and thereby increasing the bioavailability and mobility of such heavy metals in the soil (Kelebemang et al., Citation2017; Sehube et al., Citation2017). S/P shooting range recorded the highest amount of organic matter (1.88 ± 0.42% OM) while the lowest amount of organic matter (0.54 ± 0.01% OM) was measured at PANDA shooting range.
The electrical conductivity evaluates the concentration of soluble salts present in the soil (Carmo, Lima, & Silva, Citation2016). Higher electrical conductivity (EC) in the soil implies higher concentrations of heavy metals in the soil are present in soluble form. S/P and TAB shooting range soils recorded the highest electrical conductivity values of 208.9 ± 8.8 µS/cm and 170.8 ± 8.4 µS/cm respectively. Consequently, TAB and S/P shooting ranges accumulated the highest concentrations of heavy metals in the berm soils. Concentrations reaching highs of 1569 ± 23 mg/kg and 282 ± 2 mg/kg Cu were recorded at TAB and S/P shooting range soils respectively. There is a direct relationship between the amount of organic matter in the soil and its electrical conductivity. Organic matter leads to production of organic and inorganic acids that are capable of dissolving heavy metal complexes in the soil registering high values of electrical conductivity giving rise to increased bioavailability and mobility of heavy metals in the soil (Verma, Sharma, & Paramanick, Citation2015). To the best of our knowledge, no study has been conducted in Botswana shooting ranges that assessed the environmental impact of heavy metals such as Cd, Cu, Mn, Ni and Zn making it difficult to compare our findings with the similar data found elsewhere in Botswana.
3.4. Environmental risk assessment of pollution from heavy metals
3.4.1. Potential ecological risk index and RI
All the five shooting ranges experienced between moderate to very-high ecological pollution risk from Cd. The ecological risk index level due to Cd was in the range 52–8141 as shown on Table below. The ecological risk index (PERI) for Cd was highest for S/P (8140.9) followed by TAB (3507.4) and lowest for PANDA (51.9) shooting ranges. It is worth noting that even though all the five shooting ranges accumulated the lowest amounts of Cd compared to the other four heavy metals (Mn, Zn, Cu and Ni), these small amounts of Cd pose the highest ecological risk compared to the other four heavy metals due to its high toxicity effect. Cd has lower USEPA critical limit (7.1 × 101 mg/kg) implying accumulation of smaller amounts of Cd into the soil pose a pollution risk. On the other hand the other heavy metals studied have much higher critical limits in the soil in which Cu = 3.1 × 103 mg/kg, Ni = 8.2 × 102 mg/kg, Zn = 2.3 × 104 mg/kg and Mn = 1.8 × 103 mg/kg. Most of the shooting ranges such as MAT and PANDA showed very low ecological risk from Mn with risk indices of 0.07 and 0.04 respectively even though the two shooting ranges accumulated higher concentrations of Mn (Table ). This means that even though the shooting ranges may have experienced elevated levels of Mn, such high concentrations do not pose an ecological risk to the environment due in part to the high critical limit of Mn in the soil. The sum of the ecological risk indices (RI) of all the heavy metals studied indicates very high ecological risk with RI values greater than 600 for the three ranges TAB, SHO and S/P as shown in Table below. The high PERI and RI indices quantify the potential risk posed by heavy metals to the living organisms inhabiting the shooting range soils and immediate environment. High potential ecological risk index of 1158 for Cd has been reported before in literature implying ecological hazard to biota (Shen et al., Citation2017). The high PERI and RI indices indicate that drastic remedial measures need to be taken to arrest the ecological contamination risk posed by these heavy metals to shooting range soils.
Table 5. PERI and RI indicating the pollution risk level of heavy metals to five shooting range soils under study
3.4.2. Contamination factor
The contamination factors (CF) corroborate the PERI and RI results. Cadmium (Cd) displayed the highest contamination factor than any other heavy metal studied in the five shooting range soils. CF for Cd was determined in the range 2–271 in all the five shooting ranges, indicating moderate to very high contamination of the soil from Cd (Table ). As seen from the PERI results, the CF for S/P indicate that this shooting range experienced the highest contamination from Cd than any other shooting range under study. Contamination factor has been used as a good estimate of pollution risk of heavy metals in the soil (Matong, Nyaba, & Nomngongo, Citation2016; Mmolawa et al., Citation2011).
Table 6. Contamination factor of the five shooting ranges showing level of contamination of shooting range soils from the five heavy metals investigated
3.5. Statistical assessment of pollution using the Pearson correlation coefficient
The effects of physicochemical properties of the soils such as organic matter (OM) and electrical conductivity (EC) on the pollution risk of heavy metals in shooting range soils were determined using the person correlation coefficient (r). There is direct correlation between the ecological pollution risk from heavy metals and the soil organic matter and electrical conductivity as indicated by the high Pearson correlation coefficients. Pearson correlation coefficient (r) of 0.95 depicted high ecological risk from heavy metals at high electrical conductivities of the soil (Figure ). Similarly, correlation coefficient (r) of 0.98 suggested positive relationship between the ecological pollution risk of heavy metals and the soil organic matter (Figure ). Our previous have shown that high organic matter stabilizes heavy metal complexes and salts in the soil enhancing the accumulation of such heavy metals in the soil (Kelebemang et al., Citation2017; Sehube et al., Citation2017)
4. Conclusions
This study is the first in Botswana to assess the environmental pollution risk associated with heavy metal deposition in shooting range soils arising from ammunition. The findings from this study will provide a baseline data in Botswana for subsequent studies on heavy metal contamination of shooting range soils. High concentrations of Cu (1569 ± 13 mg/kg) and Mn (953.8 ± 2.8 mg/kg) were found in shooting range soils. However, the high concentration of heavy metal in the soil does not give a true picture of the environmental impact and risk from such heavy metal. Potential ecological risk (PERI) and contamination factor (CF) were able to establish that Cd, even though had lower concentrations measured in shooting range soils, posed greater environmental pollution risk than the other four heavy metals studied. The ecological risk (RI) emanating from contributions by all the five heavy metals present in the soil indicated very high pollution in three shooting ranges TAB, SHO and S/P. The environmental pollution risk posed by these heavy metals call for remedial actions and best shooting range management practices to be taken to arrest the situation before it spirals out of control. Remedial control measures such as chemical and phytoremediation processes can be employed to control soil pollution of shooting range soils. Chemical remediation processes involving use of chemicals such phosphate salts and lime have been found to immobilize heavy metals in the soil, reducing their mobility and bioavailability. Future studies into the best soil cleaning and soil reclamation strategies and methods should be conducted in order to come up with remedies and measures to control and manage pollution in shooting range soils.
Acknowledgements
The authors are grateful to the Botswana Defence Force (BDF) for providing their shooting ranges to be used in the study. We would like to acknowledge the Botswana University of Agriculture and Natural Resources (BUAN) for their assistance with ICP-OES instrument for heavy metal analysis.
Disclosure Statement
The authors report no potential conflict of interest.
Additional information
Funding
Notes on contributors
Pogisego Dinake
Pogisego Dinake works as a lecturer in the department of Chemical and Forensic Sciences, Botswana International University of Science & Technology (BIUST), Botswana. He obtained his PhD in Chemistry in 2012 at Howard University in the United States of America (USA). His research interests are in Environmental Analytical Chemistry. He investigates environmental pollution of air, soil, water and living organisms from trace metals and organic pollutants. He also studies; chemical speciation of heavy metals in water, soil and biological systems and chemical remediation of toxic trace metal contaminated water and soil. He also applies supramolecular chemistry in the design, synthesis and application of macro ligands for environmental pollution monitoring such as molecular sieves for waste water purification. He has collaboration with researchers in Botswana, South Africa and USA. He has published in international journals. Recently he has established his own research laboratory and working on various environmental pollution projects.
References
- Alexander, T. C., Gulledge, E., & Han, F. (2016). Arsenic occurrence, ecotoxicity and its potential remediation. Journal of Bioremediation and Biodegradation, 7, e174. doi:10.4172/2155-6199.1000e174
- Almendros, G., Kgathi, D., Sekhwela, M., Zancada, M. C., Tinoco, P., & Pardo, M. T. (2003). Biogeochemical assessment of resilient humus formations from virgin and cultivated Northern Botswana soils. Journal of Agricultural and Food Chemistry, 51, 4321–4330. doi:10.1021/jf034006u
- Broadley, M. R., White, P. J., Hammond, J. P., Zelko, I., & Lux, A. (2007). Zinc in plants. New Phytologist, 173, 677–702. doi:10.1111/nph.2007.173.issue-4
- Brocato, J., & Costa, M. (2017). The biological chemistry of nickel: nickel toxicity and carcinogenesis. In D. Zamble, M. Rowinska-Zyrek, & H. Kozlowski (Eds.), Royal society of chemistry (pp. 27–42). Croydon, CR0 4YY, UK: CPI Group (UK) Ltd.
- Cao, L., Tian, H., Yang, J., Shi, P., Lou, Q., Waxi, L., … Peng, X. (2015). Multivariate analyses and evaluation of heavy metals by chemometric BCR sequential extraction method in surface sediments from Lingdingyang Bay, South China. Sustainability, 7, 4938–4951. doi:10.3390/su7054938
- Carmo, D. L., Lima, L. B., & Silva, C. A. (2016). Soil fertility and electrical conductivity affected by organic waste rates and nutrient inputs. Revista Brasileira de Ciência do Solo, 40, 150–152. doi:10.1590/18069657rbcs20150152
- de Queiroz Barcelo, J. P., de Souza Osóri, C. R. V., Leal, A. J. F., Alves, C. Z., Santos, E. F., Reis, H. P. G., & Dos Reis, A. R. (2017). Effects of foliar nickel (Ni) application on mineral nutrition status, urease activity and physiological quality of soybean seeds. Australian Journal of Crop Science, 11, 184–192. doi:10.21475/ajcs.17.11.02.p240
- Department of Water Affairs and Forestry. (1996). South African water quality guidelines. Aquatic Ecosystems, 7, 45–49.
- Ekosse, G. I., & Anyangwe, S. (2012). Mineralogical and particulate morphological characterization of Geophagic clayey soils from Botswana. Bulletin of the Chemical Society of Ethiopia, 26, 373–382. doi:10.4314/bcse.v26i3.6
- Evangelou, M. W. H., Hockmann, K., Pokharel, R., Jakob, A., & Schulin, R. (2012). Accumulation of Sb, Pb, Cu, Zn and Cd by various plants species on two different relocated military shooting range soils. Journal of Environmental Management, 108, 102–107. doi:10.1016/j.jenvman.2012.04.044
- Fayiga, A. O., & Saha, U. K. (2016). Review: Soil pollution at outdoor shooting ranges: Health effects, bioavailability and best management practices. Environmental Pollution, 216, 135–145. doi:10.1016/j.envpol.2016.05.062
- Hakanson, L. (1980). An ecological risk index for aquatic pollution control. A sedimentological approach. Water Research, 14, 975–1001. doi:10.1016/0043-1354(80)90143-8
- Hockmann, K., Tandy, S., Lenz, M., Reiser, R., Conesa, H. M., Keller, M., … Schulin, R. (2015). Antimony retention and release from drained and waterlogged shooting range soil under field conditions. Chemosphere, 134, 536–543. doi:10.1016/j.chemosphere.2014.12.020
- Islam, M. N., Nguyen, X. P., Jung, H. Y., & Park, J. H. (2016). Chemical speciation and quantitative evaluation of heavy metal pollution hazards in two army shooting range backstop soils. Bulletin of Environmental Contamination and Toxicology, 96, 179–185. doi:10.1007/s00128-015-1689-z
- Jastrow, J. D., Amonette, J. E., & Bailey, V. L. (2007). Mechanisms controlling soil carbon turnover and their potential application for enhancing carbon sequestration. Climatic Change, 80, 5–23. doi:10.1007/s10584-006-9178-3
- Johnson, C. A., Moench, H., Wersin, P., Kugler, P., & Wenger, C. (2005). Solubility of antimony and other elements in samples taken from shooting ranges. Journal of Environmental Quality, 34, 248–254. doi:10.2134/jeq2004.0398
- Joshua, W. D. (1991). Physical properties of the soils of Botswana. Gaborone: Soil Mapping and Advisory Services Botswana, Food and Agricultural Organization (FAO) of the United Nations, United Nations Development Programme (UNDP), Government of Botswana.
- Ke, X., Gui, S., Huang, H., Zhang, H., Wang, C., & Guo, W. (2017). Ecological risk assessment and source identification for heavy metals in surface sediment from the Liaohe river protected area, China. Chemosphere, 175, 473–481. doi:10.1016/j.chemosphere.2017.02.029
- Kelebemang, R., Dinake, P., Sehube, N., Daniel, B., Totolo, O., & Laetsang, M. (2017). Speciation and mobility of lead in shooting range soils. Chemical Speciation and Bioavailability, 29, 143–152. doi:10.1080/09542299.2017.1349552
- Lafond, S., Blais, J. F., Martel, R., & Mercier, G. (2013). Chemical leaching of antimony and other metals from small arms shooting range soil. Water Air Soil and Pollution, 224, 1371–1385. doi:10.1007/s11270-012-1371-6
- Laghlimi, M., Baghdad, B., El Hadi, H., & Bouabdli, A. (2015). Phytoremediation mechanisms of heavy metal contaminated soils: A review. Open Journal of Ecology, 5, 375–388. doi:10.4236/oje.2015.58031
- Lange, B., Van der Ent, A., Baker, A. J. M., Echevarria, G., Mahy, G., Malaisse, F., … Faucon, M. P. (2017). Research review: Copper and Cobalt accumulation in plants: A critical assessment of the current state of knowledge. New Phytologist, 213, 537–551. doi:10.1111/nph.14223
- Lee, I. S., Kim, O. K., Chang, Y. Y., Bae, B., Kim, H. H., & Baek, K. H. (2002). Heavy metal concentrations and enzyme activities in soil from a contaminated korean shooting range. Journal of Bioscience and Bioengineering, 94, 406–411. doi:10.1016/S1389-1723(02)80217-1
- Ma, L. Q., Hardison, D., Jr, Harris, W. G., Cao, X., & Zhou, Q. (2007). Effects of soil property and soil amendment on weathering of abraded metallic Pb in shooting ranges. Water Air and Soil Pollution, 178, 297–307. doi:10.1007/s11270-006-9198-7
- Mariussen, E., Johnsen, I. V., & Strømseng, A. E. (2017). Distribution and mobility of Lead (Pb), Copper (Cu), Zinc (Zn), and Antimony (Sb) from ammunition residues on shooting ranges for small arms located on mires. Environmental Science and Pollution Research International, 24, 10182–10196. doi:10.1007/s11356-017-8647-8
- Matong, J. M., Nyaba, L., & Nomngongo, P. N. (2016). Fractionation of trace elements in agricultural soils using ultrasound assisted sequential extraction prior to inductively coupled plasma mass spectrometric determination. Chemosphere, 154, 249–257. doi:10.1016/j.chemosphere.2016.03.123
- Migliorinia, M., Pigino, G., Bianchi, N., Bernini, F., & Leonzio, C. (2004). The effects of heavy metal contamination on the soil arthropod community of a shooting range. Environmental Pollution, 129, 331–340. doi:10.1016/j.envpol.2003.09.025
- Mitchell, A. J. B. (1976). The irrigation potential of soils along the main rivers of Eastern Botswana - A reconnaissance assessment. Surbiton, Surrey, England: Land Resource Study 7, Land Resources, Division, Ministry of Overseas Development, Tolworth Tower.
- Mmolawa, K. B., Likuku, A. S., & Gaboutloeloe, G. K. (2011). Assessment of heavy metal pollution in soils along major roadside areas in Botswana. African Journal of Environmental Science and Technology, 5, 186–196.
- Moganane, B. G., Van Waveren, E., & Remmelzwaal, A. (1991). The soils of the pandamatenga plains. Botswana: Soil Mapping and Advisory Services. Field Document: 12, Gaborone. AG:BOT/85/011.
- Mozafar, A., Ruh, R., Klingel, P., Gamper, H., Egli, S., & Frossard, E. (2002). Effect of heavy metal contaminated shooting range soils on mycorrhizal colonization of roots and metal uptake by leek. Environmental Monitoring and Assessment, 79, 177–191. doi:10.1023/A:1020202801163
- Nagajyoti, P. C., Lee, K. D., & Sreekanth, T. V. M. (2010). Heavy metals, occurrence and toxicity for plants: A review. Environmental Chemistry Letters, 8, 199–216. doi:10.1007/s10311-010-0297-8
- Nelson, D. W., & Sommers, L. E. (1982). Total carbon, organic carbon, and organic matter. In A. L. Page, R. H. Miller, & D. R. Keeney (editors), Methods of soil analysis, part 2 chemical and microbiological properties (Vol. 9, pp. 539–577). Madison (WI: ASA.
- Okkenhaug, G., Amstatter, K., Bue, H. L., Cornelissen, G., Breedveld, G. D., Henriksen, T., & Mulder, J. (2013). Antimony (Sb) contaminated shooting range soil: Sb mobility and immobilization by soil amendments. Environmental Science and Technology, 47, 6431−6439. doi:10.1021/es302448k
- Pardo, M. T., Ristori, G., D’acqui, P., & Almendros, G. (2003). An assessment of soil fertility and agronomic constraints in Southern African Savannas: A case study of the Pandamatenga area, Botswana. South African Geographical Journal, 85, 35–41. doi:10.1080/03736245.2003.9713782
- Pejman, A., Bidhendi, G. N., Ardestani, M., Saeedi, M., & Baghvand, A. (2017). Fractionation of heavy metals in sediments and assessment of their availability risk: A case study in the Northwestern of Persian Gulf. Marine Pollution Bulletin, 114, 881–887. doi:10.1016/j.marpolbul.2016.11.021
- Robinson, B. H., Bischofberger, S., Stoll, A., Schroer, D., Furrer, G., Roulier, S., … Schulin, R. (2008). Plant uptake of trace elements on a Swiss military shooting range: Uptake pathways and land management implications. Environmental Pollution, 153, 668–676. doi:10.1016/j.envpol.2007.08.034
- Rodríguez-Seijo, A., Alfaya, M. C., Andrade, M. L., & Vega, F. A. (2016). Copper, chromium, nickel, lead and zinc levels and pollution degree in firing range soils. Land Degradation and Development, 27, 172–1730. doi:10.1002/ldr.2497
- Rooney, C. P., McLaren, R. G., & Cresswell, R. J. (1999). Distribution and phytoavailability of lead in a soil contaminated with lead shot. Water Air and Soil Pollution, 116, 535–548. doi:10.1023/A:1005181303843
- Sehube, N., Kelebemang, R., Totolo, O., Laetsang, M., Kamwi, O., & Dinake, P. (2017). Lead pollution of shooting range soils. South African Journal of Chemistry, 70, 21–28. doi:10.17159/0379-4350/2017/v70a4
- Seshadri, B., Bolan, N. S., Choppala, G., Kunhikrishnan, A., Sanderson, P., Wang, H., … Kim, K. (2017). Potential value of phosphate compounds in enhancing immobilization and reducing bioavailability of mixed heavy metal contaminants in shooting range soil. Chemosphere, 184, 197–206. doi:10.1016/j.chemosphere.2017.05.172
- Sharma, A., & Sachdeva, S. (2015). Cadmium toxicity and its phytoremediation: A review. International Journal of Scientific and Engineering Research, 9, 395–405.
- Shen, F., Liao, R., Ali, A., Mahar, A., Guo, D., Li, R., … Zhang, Z. (2017). Spatial distribution and risk assessment of heavy metals in soil near a Pb/Zn smelter in Feng County, China. Ecotoxicology and Environmental Safety, 139, 254–262. doi:10.1016/j.ecoenv.2017.01.044
- Soliman, N. F., Nasr, S. M., & Okbah, M. A. (2015). Potential ecological risk of heavy metals in sediments from the Mediterranean Coast, Egypt. Journal of Environmental Health Science and Engineering, 13, 70–81. doi:10.1186/s40201-015-0223-x
- Sorvari, J., Antikainen, R., & Pyy, O. (2006). Environmental contamination at finnish shooting ranges—The scope of the problem and management options. Science of the Total Environment, 366, 21–31. doi:10.1016/j.scitotenv.2005.12.019
- Sporting Arms and Ammunition Manufacturers’ Institute, SAAMI. (1996). Lead mobility at shooting ranges. (Project No. 13081.01), Flintlock Ridge Office Center, 11 Mile Hill Road, Newtown, CT 06470, 22–24.
- Sundar, S., & Chakravarty, J. (2010). Review: Antimony toxicity. International Journal of Environmental Research and Public Health, 7, 4267–4277. doi:10.3390/ijerph7124267
- Tchoumou, M., Kami-Ouako, M., Mbilou, G. U., & Ossebi, J. (2017). Comparative study of physicochemical parameters of water of four waterways in the Town of Brazzaville, Republic of Congo. LARHYSS Journal, 29, 61–74.
- Urrutia-Goyes, R., Argyraki, A., & Ornelas-Soto, N. (2017). Assessing lead, nickel, and zinc pollution in topsoil from a historic shooting range rehabilitated into a Public Urban Park. International Journal of Environmental Research and Public Health, 14, 698–712. doi:10.3390/ijerph14070698
- Verma, J. K., Sharma, A., & Paramanick, K. K. (2015). To evaluate the values of electrical conductivity and growth parameters of apple saplings in nursery fields. International Journal of Applied Sciences and Engineering Research, 4, 321–332.
- Vig, K., Megharaj, M., Sethunathan, N., & Naidu, R. (2003). Bioavailability and toxicity of cadmium to microorganisms and their activities in soil: A review. Advances in Environmental Research, 8, 121–135. doi:10.1016/S1093-0191(02)00135-1
- Wersin, P., Johnson, A. A., & Furrer, G. (2002). Antimony contamination in soil and ground water by shooting range activities. Geochimica et Cosmochimica Acta, 66, A829.