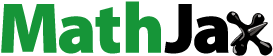
Abstract
Biosorption, despite being initially heralded as a technology offering great potential for detoxification, is still yet to be commercialized. One of the reasons given is the lack of universal understanding of the mechanisms involved. This review focused on the removal of heavy metals from solutions in which Pb(II) co-existed with other metals in binary, ternary and quaternary systems. Trends in sorption capacities were analysed using ratios of maximum adsorption capacities: ,
, and
, where
and
represent the adsorption capacity in the presence and absence of the interfering metal ion, respectively, and
is the metal ion co-existing with Pb(II). The data in literature were analysed for selected metal ions Pb(II), Cu(II), Zn(II), Cd(II), Ni(II) and Cr(III). It was shown that for all systems analysed, the ratio:
< 1, thus confirming that the simultaneous presence of metal ions in solution reduced their adsorption capacities. For the selected Pb/Cu system it was shown that generally
>
,
and
, thus confirming antagonistic effects for the system. It was further shown that Pb(II) contributed to 70–77% of the total adsorption capacity, and the % change in adsorption capacity (ⵠ
) was negative. In conclusion, these findings confirmed the superiority of Pb(II) adsorption across several metal-biosorbent systems, which has implications on the designing of model biotechnologies for possible practical applications.
PUBLIC INTEREST STATEMENT
Biosorption has shown immense potential as a low cost and green approach for the removal of heavy metals from water. However, despite initially making huge strides, the technology is still yet to be commercialized. One of the reasons frequently cited is the lack of universal understanding of the mechanisms involved, especially when biosorption is applied to multi-metal systems. Attention can be drawn to the lack of coherence in findings relating to the interference effects when Pb co-exists with other heavy metals in solution. While most researchers have reported a high preference for Pb biosorption, some have reported otherwise. Since multi-metal sorption is significant in the simulation of real effluent composition, the author found it of much interest to review heavy metal removal from solutions in which Pb co-existed with one, two or three other metals. The author confirmed the superiority of Pb adsorption across several metal-biosorbent systems and conditions, and these findings have implications on the designing of model biotechnologies for real industrial applications.
Competing Interests
The author declares no competing interests.
1. Introduction
Research and development focusing on biosorption phenomena provides a springboard for a whole new environmental technology targeting the removal of various toxic substances or the recovery of precious metals from aquatic systems. It is therefore not surprising that numerous articles (in excess of 13 000) have been produced on biosorption in the past 60 years (Abdulaziz & Musayev, Citation2017). However, despite the significant progress in understanding of this complex phenomenon, commercialization of biosorption technologies has been elusive so far (Fomina & Gadd, Citation2014). In recent times, research has therefore sought to align biosorption technology towards more practical application to detoxification of environmental systems by enhancing physicochemical characteristics of the biosorbents, optimizing the biosorption processes, and applying the technology to more complex systems, e.g., multi-metal systems. More recently, research has focused on harnessing the capabilities offered by nanotechnology to develop biosorbents which are cost-effective, have high adsorption capacity, capable of adsorbing pollutants at lower concentrations (ppb) and can be regenerated in several sorption-desorption cycles (Khajeh, Laurent, & Dastafkan, Citation2013).
Focusing on the removal of metals from aquatic systems, biosorption has mainly been applied for the uptake of toxic metals (e.g., Hg, Pb, Cr, Cu, Zn, Cd, As, Co etc.), radionuclides (e.g., U, Th, Ra, Am etc.) and valuable metals (e.g., Au, Pt, Pd, Ru etc.) (Wang & Chen, Citation2006, Citation2009). It has since been established that factors which significantly influence biosorption include solution pH, ionic strength, initial sorbate concentration, biosorbent nature and the availability of binding sites, temperature, and agitation rate (Fomina & Gadd, Citation2014). Solution pH is a very important variable as it determines the solution chemistry of the sorbate and the functional activity of the biosorbents (Vijayaraghavan & Yun, Citation2008). Whereas the increase in ionic strength reduces biosorptive removal of pollutant species due to competition for binding sites, increase in initial pollutant concentration increases the quantity of biosorbed pollutant per unit weight of biosorbent (Fomina & Gadd, Citation2014). However, the removal efficiency decreases with an increase in initial metal concentration. The advent of nano-biosorbents has shown that the method of synthesis, surface modifications, and dosage play a pivotal role in determining the performance characteristics (Krstic´, Uroševic´, & Pešovski, Citation2018; Shirsath & Shirivastava, Citation2015; Wang et al., Citation2012; Yu et al., Citation2013). Although the temperature has been shown to enhance the biosorption of pollutants, the effect is not significant for metal sorption between 20°C and 35°C. The presence of other pollutants generally lowers individual pollutant removal through competition for adsorption sites in multi-metal systems (Fomina & Gadd, Citation2014).
Motivated by the need to develop models applicable to real industrial samples, researchers have reported on the performance of biosorption technology when applied to multi-metal systems, mainly focusing on binary, ternary, and quaternary systems. Although these systems may not exhaustively resemble the composition of real industrial effluents, they at least give information useful in determining how adsorption capacity will be affected when metal ions co-exist in solution. Preliminary findings indicated that when Pb was removed from aqueous solution in the presence of other heavy metals, it mostly dominated the adsorption process (Al-Rub, El-Naas, Ashour, & Al-Marzouqi, Citation2006; Chong & Volesky, Citation1995; Hammaini, Ballester, Blázquez, González, & Munõz, Citation2002; Maity & Ray, Citation2018; Wang, Vincent, Faur, & Guibal, Citation2017). In real practice, this means that when Pb is co-existing with other metal ions in solution, removal of the other ions would be suppressed until the Pb ions have been depleted to sufficiently low levels. Such sorption dynamics would, therefore, be important in understanding how the degree of tolerance of a biosorbent to multi-metal sorption and relative selectivity to individual metals vary with a combination of the metals in solution. However, a survey of the literature does not indicate that a systematic review was carried out on the apparent dominance of Pb during competitive biosorption from multi-metal systems.
The current study, therefore, sought to explore the above findings based on published equilibrium sorption data for several biosorbent systems. In particular, the study reviewed the sorption behaviour of multi-metal systems consisting of Pb, Cu, Cd, Zn, Ni, and Cr in binary, ternary, and quaternary systems. The sorption behaviour of the heavy metals was probed using ratios of maximum adsorption capacities: ,
, and
,where
and
represent the adsorption capacity in the presence and absence of the interfering metal ion, respectively, and
is the metal ion co-existing with Pb(II). Furthermore, equilibrium sorption data from multi-metal sorption were compared to that from monocomponent systems. The Pb-Cu system was selected as a typical binary system for an in-depth study whereas ternary and quaternary sorption systems were analysed for varying combination of the metals.
2. Competitive sorption from multi-metal systems
Although most industrial and municipal effluents are typically composed of a complex matrix of pollutants, relatively fewer studies have been conducted on competitive or simultaneous removal of metals from multi-metal systems compared to single-metal sorption. A scan of the literature shows that more than 500 papers have been published on biosorption in multi-component systems over the past few years, mainly in the fields of chemistry, environmental science and chemical engineering. These include studies on binary (Muthusamy & Venkatachalamb, Citation2015; Suzaki et al., Citation2017; Xie, Hao, Mohamad, Liang, & Wei, Citation2013), ternary (Al-Rub et al., Citation2006; Costa, Da Silva, & Vieira, Citation2018; Ray, Jana, Bhanja, & Tripathy, Citation2018) and quaternary systems (Ahmad & Haseeb, Citation2017; Sulaymon, Mohammed, & Al-Musawi, Citation2013; Vijayaraghavan, Rangabhashiyam, Ashokkumar, & Arockiaraj, Citation2016). Table shows the top 10 most cited articles and Figures and present number of papers and number of citations by the field for papers appearing with “lead” or “Pb” and “biosorption” in the topic listed in the Scopus database for period 2000–2017 (database searched on 23.10.18). Figure shows that the number of citations generally increased from the year 2000, reaching a maximum in 2008 before declining in subsequent years. From the top 10 most cited papers, it is clear that most of the biosorbents studied showed greater affinity for Pb adsorption in both single and multi-metal systems. In these studies, whilst Pb effectively suppressed adsorption of competing metal ions, its uptake was not significantly reduced by the presence of the other metal ions. However, among authors with divergent findings, Saeed, Iqbal, and Akhtar (Citation2005) reported that the presence of Pb did not affect the efficiency of removal of other metals when they were removed in binary and ternary solutions. Similar observations were made by Fiol et al. (Citation2006) who reported that sorption of Cd was not significantly affected by the presence of Pb, and their results show that sorption of Cu and Ni was actually enhanced in the presence of Pb. However, one major challenge that arises on the interpretation of the findings from multi-metal studies is the difficulty in drawing meaningful and universal conclusions due to the different methodologies used. The several papers reported in the literature present sorption studies carried out under varying conditions of solution pH, ionic strength, initial pollutant concentration, agitation rate, and temperature.
Figure 1. Number of papers appearing with “lead” or “Pb” and “biosorption” in the topic listed in the Scopus database for period 2000–2017. Database searched on 23.10.18.
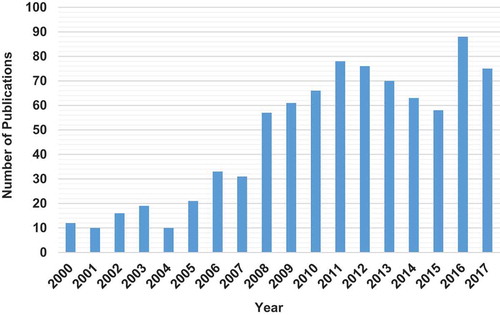
Figure 2. Number of citations of papers appearing with “lead” or “Pb” and “biosorption” in the topic listed in the Scopus database for period 2000–2017. (Total number of articles appearing = 844: database searched on 23.10.18; sum of the times cited = 27, 410; average citations per article = 32.5; h index = 16; articles with zero (0) citations to date = 131).
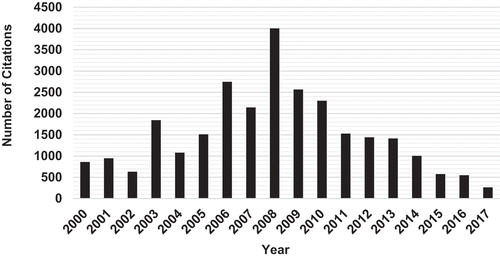
Table 1. The top 10 most cited articles in the Scopus database appearing with “lead” or “Pb” and “biosorption” in the topic (out of a total of 844 articles appearing: database searched 23.10.18)
2.1. A critical analysis of initial metal concentration as an experimental design parameter
Among the experimental conditions discussed earlier, the variation of initial metal concentration is key in characterizing sorption behaviour of a given sorbate-biosorbent system. In both batch and continuous flow systems, useful data are generated when the adsorption capacity of the biosorbent is determined as a function of initial metal concentration. In batch systems, such studies can generate equilibrium sorption data which can be modelled using various adsorption isotherms. The published material shows that it is possible to classify the experimental conditions based on how the initial metal concentration is varied during biosorption studies. It emerges from performing an in-depth analysis that three conditions can be proposed, namely: Condition (I), where the initial concentrations ) of the metals
and
are varied simultaneously, with
; Condition (II), where initial concentrations of both components are varied simultaneously with
; and Condition (III), where the initial concentration of one of the components is varied while keeping the other constant. The initial metal conditions used by several authors are shown in Table .
Table 2. Classification of initial concentration variations
It was observed that for most of the studies, the initial concentration, ), is measured in mg/L. Therefore, due to differences in molecular weight, the molar concentration would be different even for the same initial concentration (condition (I)). Since it has already been noted that the removal efficiency decreases with increasing initial concentration, comparison of findings obtained under conditions (I)–(III) would reveal the level of competition between the sorbates. Furthermore, some authors have reported on biosorption studies carried out under a combination of the conditions, e.g., under conditions (I) and (II) (Jiang, Zhou, Liu, Liu, & Wang, Citation2017), conditions (I) and (III) (Ronda, Martín-Lara, Dionisio, Blázquez, & Calero, Citation2013; Yan, Li, Xue, Wei, & Li, Citation2010), and conditions (I), (II) and (III) (Wang et al., Citation2017). Some authors have expanded the multicomponent system to study competitive biosorption in ternary and quaternary systems (Mahamadi & Nharingo, Citation2010; Saeid & Chojnacka, Citation2015; Sulaymon et al., Citation2013).
The findings from these studies confirm the general conclusions made earlier, that most of the biosorbents had strong selective adsorption of Pb compared to other heavy metals. However, as also noted in the earlier discussion, a few authors also reported conflicting observations. Deng et al. (Citation2017) reported that chitosan-pyromellitic dianhydride (PMDA)-modified biochar had strong selective adsorption of Cu(II) compared to Cd(II) and Pb(II). In another study, it was observed that Myriophyllum spicatum had no preference for Cu(II) and Pb(II) in spite of their differences in physicochemical properties (Yan et al., Citation2010). Elsewhere, Pithophora oedogonia biosorbent showed a greater affinity to sorb Cu(II) than Pb(II) from binary solution (Kumar, Singh, & Gaur, Citation2008) and presence of Cu(II) adversely affected Pb(II) adsorption onto Chlorella vulgaris, whereas Zn(II) ions seemed to have negligible effect on the process (El-Naas, Al-Rub, Ashour, & Al Marzouqi, Citation2007). These differences could be a result of variations in physicochemical characteristics of the biosorbents which affect metal sorption preferences. For example, it was observed that the types of effective functional groups involved in the sorption of Cd, Cu, and Pb by chitosan-pyromellitic dianhydride were different, with N-C = O playing a significant role in the process of Pb(II) removal, while C = C and N-containing functional groups were important in the removal of Cd(II) (Deng et al., Citation2017).
2.2. Biosorption isotherms
Applied at a constant temperature, biosorption isotherms describe metal sorption as a function of metal concentration in the solution at equilibrium. Some of the isotherms which are commonly used to describe single-metal sorption on biosorbent include Freundlich, Langmuir, Redlich-Peterson and Sips models. Since these models were primarily developed for monocomponent systems, they fail to adequately describe sorption behaviour for multi-component systems (Kumar et al., Citation2008). Researchers have therefore developed several empirical and mechanistic models to describe sorption for these complex systems. These include three and five-parameter extended Freundlich model (Fritz & Schluender, Citation1974; Kumar et al., Citation2008; Sheintuch & Rebhun, Citation1988), Competitive Hill model (Sellaoui, Franco, Dotto, Lima, & Lamine, Citation2017), and competitive Langmuir model (Ofomaja, Unuabonah, & Oladoja, Citation2010b). Among these models, the Langmuir competitive model is the most tested, probably because of the relative simplicity in application and interpretation of data. Mostly, the model has been applied in the determination of the maximum equilibrium capacity of the biosorbents for the various sorption systems.
2.2.1. Monocomponent Langmuir isotherm
The Langmuir isotherm is described assuming that the receptor sites on the biosorbent are energetically identical regardless of adsorbate under study. Furthermore, it is assumed that a localized adsorbent site accommodates one adsorbate species per active site. The model further assumes that interactions among adsorbates are negligible.
For a monocomponent system, mathematical expression of the Langmuir isotherm can be expressed as below:
and linearized as:
where (mg/g) is the maximum amount of adsorbate per unit weight of the biosorbent required to form a complete monolayer on the surface at equilibrium, and
is a constant reflecting the affinity of the binding sites for the metal ion (1 mg−1).
2.2.2. Competitive Langmuir isotherm
For competitive adsorption, the model is similar to the single sorption model in that the rate of adsorption still depends on only the adsorbing component (Mahamadi & Nharingo, Citation2010). However, the rate of adsorption must account for available sites only (i.e., ). After setting the adsorption and desorption rates equal, it can be shown that the extent of adsorption is given by:
were and
are physical parameters, and
represent the equilibrium concentrations in the mixture of the sorbates. Assuming that the concentrations of the sorbates are sufficiently large that surface coverage is substantially complete, the unit term in Equation (4) may be neglected, and after some rearrangements, the expression can be expressed in a linear form as shown in Equations (1) and (2) for binary and ternary systems, respectively:
By plotting as a function of
and
as a function of
would give intercepts of
and
, slope of
for binary and ternary systems, respectively.
2.3. Analysis of competitive Langmuir equilibrium sorption data
2.3.1. Binary sorption systems
For binary systems, the equilibrium data can be analysed using the ratio of biosorption capacity of one metal ion in the presence of the other metal ion () to biosorption capacity for the same metal when it is present alone (
) (Ofomaja et al., Citation2010b). The following scenarios thus can be encountered:
Synergism: the biosorption is promoted in the presence of the other metal ion.
Non-interaction: there is no observable net effect
Antagonism: biosorption is suppressed, i.e., the effect of the mixture is less than that of the individual adsorbates in the mixture.
2.3.1.1. Pb-cu system
Various binary systems in which Pb co-exist with other metals in solution have been reported, and these include: Pb-Cd, Pb-Zn, Pb-Cu, Pb-Ni, and Pb-Cr. The Pb-Cu system was selected as a typical binary system for an in-depth probing of equilibrium sorption data using the adsorption capacity ratios. Data showing maximum equilibrium adsorption capacities (), obtained from selected publications is shown in Table . It can be seen that in most cases, Condition (I) was used, i.e., equal initial concentrations of Pb(II) and Cu(II) (mg/L) (
). An analysis of the data shows that except for the sorption of Pb(II) and Cu(II) ions using alginate and alginate-immobilized Trichoderma asperellum which gave a values of 2.967 and 1.258 for the ratio
, respectively, the values computed for the rest of the biosorbents were less than unity, indicating that biosorption of both metals was largely suppressed in the binary system. This suggests that the simultaneous presence of both metals reduced their biosorption through competition for biosorbent binding sites. The results further show that except for Mansonia wood sawdust and Almond shell,
>
for the biosorbents, thus indicating that although competitive effect reduced the adsorption capacities for both metals, there was preferential adsorption for Pb(II). Valonia tannin resin biosorbent presented an interesting case in that
, thus suggesting that there was no preferential sorption by the biosorbent for both metals, although the metals mutually experienced antagonistic effects reducing their individual adsorption capacities relative to single-component sorption.
Table 3. Binary sorption: Pb/Cu system
From the adsorption capacities obtained for single-metal sorption systems, it can be seen that > 1 for all of the biosorbents investigated, thus indicating that the biosorbents had a greater affinity for Pb(II) than Cu(II). Further analysis shows that in single-metal systems, cabbage waste, pine cone shell, and alginate ranked highly as adsorbents for Pb(II). Further analysis of the equilibrium sorption data for binary removal of Pb(II) and Cu(II) can be done by comparing the ratio
and
. From Table , it can be observed that for some of the biosorbents including alginate-immobilized Trichoderma asperellum, cabbage waste, Potassium hydroxide treated pine cone powder, and Aspergillus flavus,
>
. This suggests that Pb(II) removal was enhanced relative to the removal of Cu(II) ions in the binary system compared to the single-metal system. Interestingly, however, the order was reversed for almond shell, pine cone shell, and TEPA modified chitosan/CoFe2O4 although the individual
values were higher for Pb(II) biosorption than Cu(II). Furthermore, the values obtained for
and
for Mansonia wood sawdust (1.222 and 1.250, respectively) and for Valonia tannin resin (2.513 and 2.514, respectively) suggest that the relative preference by the biosorbent for either metal was not affected by competitive effects. This suggests that the sorption sites available on Valonia tannin resin for Pb(II) and Cu(II) adsorption could be different (Wang & Sun, Citation2013).
A comparison of the sums: and
gives further insights into how the total number of available binding sites were affected by the competitive effects. From Table , it can be seen that
>
) for most of the biosorbents studies, including TEPA modified chitosan/CoFe2O4, Pine cone shell, Alginate-immobilized Trichoderma asperellum, Almond shell, Valonia tannin resin, and Aspergillus flavus. These findings suggest that metal ion access to binding sites was reduced, probably as a result of mutual competitive effects. In related studies, Chew and Ting (Citation2016) reported inferior biosorption in multi-metal systems, with cumulative means of total metals of 151.05 and 152.23 mg/g adsorbed compared to 233.96 and 209.25 mg/g in single-metal solutions using alginate-immobilized T. asperellum and plain-alginate beads, respectively. The authors attributed the lower metal sorption to competitive solute-solute (metal ion-metal ion) interaction as well as solute-surface (metal ion-surface of biosorbent) interaction for available binding sites.
Table 4. Comparison of total equilibrium sorption capacity for selected biosorbents-Pb/Cu system
It can also be observed that for all biosorbents analysed, ratios and
were greater than unity. This suggests that all biosorbent studies showed higher adsorption for Pb(II) compared to Cu(II) in both single metal and binary component systems. This observation is very significant in that, despite the differences in experimental conditions (initial metal concentration, pH, biosorbent dosages, etc.), the findings confirm one major general observation: Pb(II) biosorption clearly dominated the process in the presence of Cu(II). From the data shown in Table , an average value of 70% can be computed for the contribution of Pb(II) to the total metal adsorption capacity for the binary Pb-Cu system. In related studies, Hammaini et al. (Citation2002), showed that Cu(II) uptake decreased by 56% when Pb(II) was present at high concentration, whereas the interference of Cu(II) with Pb(II) uptake was much less pronounced and observed at much higher levels of Cu(II). Furthermore, the same authors reported that when the residuals of Pb(II) and Cu(II) were the same, about 97% of the total metal uptake was due to Pb(II) uptake. Generally, it can be concluded that regardless of conditions used (Table ), Pb(II) uptake was superior to that of Cu(II) for the various biosorption systems analysed.
It has been suggested that the extent to which metals bind to a biosorbent with different functional groups depends on their ionic properties. For metals, these include electronegativity, ionic radius, and redox potential (Naja, Vanessa, & Volesky, Citation2010; Sulaymon et al., Citation2013). For the current system, the differences in sorption affinities for these metals have been attributed to differences in the ionic radii and electronegativity of the atoms, which follow the order Pb(II)>Cu(II) (Al-Rub et al., Citation2006; Chong & Volesky, Citation1995).
2.3.2. Ternary system
As expected basing on the findings made for binary systems, the ratios were all less than unity for the selected ternary systems shown in Table . This confirms that in all cases, antagonistic effects due to competitive adsorption were significant. Further insights into the competitive biosorption can be made by analysing the trends in the adsorption capacities for monocomponent and ternary systems. From Table , it can be seen that except for Date seed biochar and Casuarina equisetifolia where there were changes in the order of
for the Pb/Cu/Ni system, the rest of the metal combinations showed the same order for the monocomponent and ternary systems. For example, C. equisetifolia shows a preference for Pb(II) adsorption when compared to Cu(II) in the ternary system whereas
in the monocomponent system. This shows the complexity of the biosorption phenomenon as it depends not only on the physicochemical characteristics of the metal ions but also on the accessibility and energies of the binding sites on the biosorbent. However, it is very clear from Tables and , that for all of the biosorbents, Pb(II) ions dominated the adsorption process in ternary systems. Furthermore, it can be shown that Pb(II) adsorption accounts for more than 77% of the total adsorption capacity for the ternary system. Again, these findings are very significant in that the trend demonstrates superior adsorption for Pb(II) despite the differences in experimental conditions used during the adsorption process.
Table 5. Ternary sorption
Table 6. Trends in adsorption capacities for monocomponent and ternary systems
The ability of Pb(II) to outcompete co-existing ions is further illustrated by the change in the order of adsorption capacities for single and multi-metal systems. Park and Chon (Citation2016) reported the order: Cd≥Pb>Zn for single-metal sorption by Exiguobacterium sp. which changed to Pb(II)>Cd(II)>Zn(II) in a mixed metal solution. In similar studies, Engl and Kunz (Citation1995) reported that the order of adsorption changed from Cu(II)>Cd = Pb>Zn to Pb>Cu>Zn = Cd for single and mixed metal systems, respectively. The differences in the sorption order were explained in terms of the higher electronegativity of Pb(II) resulting in more stable binding to the ligands on the biosorbent surface.
2.3.3. Quaternary system
Published work shows that limited research has been carried out on biosorption in quaternary systems, probably due to the greater experimental demands and complexity for such systems. However, it is important to study such systems as they represent the complex nature of most industrial effluents, e.g., from mining companies. Data obtained from typical quaternary systems is shown in Table . It is clear that except for the removal of Zn(II) from a Pb/Cu/Zn/Cd system using cabbage waste, ratios were less than unity for all sorption systems. The data were further analysed by calculating the % change in adsorption capacity (ⵠ
). If % ⵠ
, then it would suggest that adsorption capacity was reduced due to competitive effects, % ⵠ
would suggest no effect, and % ⵠ
would suggest enhancement of adsorption capacity in the presence of two or more metal ions. Except for cabbage waste biosorbent, % ⵠ
negative for all of the systems confirming the mutual antagonistic effects experienced by the sorbate species. This means that the simultaneous presence of heavy metals in solution reduced their respective adsorption capacities.
Table 7. Selected quaternary sorption systems
2.4. Interpretation of multi-metal batch sorption data
The reviewed publications showed that most authors reported on the following: physical characterization of the biosorbent, optimum conditions, removal efficiency expressed as maximum adsorption capacities (mostly in mg/g but occasionally in mmol/g) or percentages, comparison of adsorption capacities, sorption thermodynamics, and suitability of kinetic and equilibrium models in describing the sorption data, among many others. Table shows some of the major conclusions made for selected biosorbents when Pb was one of the metals in solution. As an example, Hossain et al. (Citation2014) reported that when Cu, Pb, Zn and Cd were removed from multi-metal solution using cabbage waste, the Competitive Langmuir equation indicated that the biosorbent was more selective towards Pb than to other three metals, and Pb strongly inhibited the adsorption of the other metal ions. In a related study, Martín-Lara et al. (Citation2016) applied the Extended Sips isotherm and showed that the biosorption capacity of Pb was consistently greater than that of Cu for both single and binary solution using pine cone shell bisorbent. Preferential adsorption of Pb was also observed by Şengil and Özacar (Citation2009) for the Pb/Cu/Zn system suing Volania tannin resin. According to Wang et al. (Citation2017), three biosorbents: calcium alginate, algal biomass, algal/glutaraldehyde-crosslinked polyethyleneimine (PEI) showed a greater affinity for Pb regardless of the molar ratio of competitor ions. More recently, Maity and Ray (Citation2018) applied the Competitive Langmuir equation and reported that for the same operating condition, a chitosan-based nano-composite showed higher adsorption of Pb(II) than Cd(II).
Table 8. Major conclusions from selected multi-metal sorption studies
However, in some cases authors failed to adequately interpret their data, leading to inaccurate conclusions. For example, Ofomaja et al. (Citation2010b) on studying the biosorptive removal of Cu(II) and Pb(II) from aqueous solution by Mansonia wood sawdust reported values of and
of 0.8676 and 0.8356, respectively, but went on to write that
>
, which suggested that copper biosorption onto Mansonia sawdust was more affected by the simultaneous presence of a competing metal ion than for lead ions. However, the results actually show that it is the Pb(II) ions that would be more affected by the presence of copper, since it has a lower
value. Furthermore, the authors noted that the amount of copper ions biosorbed reduced from 26.8 to 19.2 mg/g when lead ion concentration was increased from 0 to 60 mg/dm3. Again, this is not correct according to results presented in the paper which show that in fact the adsorption capacity of 26.8 mg/g was obtained using an initial concentration of 140 mg/dm3 Cu(II) and 0 mg/dm3 Pb(II). When the Pb(II) concentration was increased from 0 to 60 mg/dm3, the results show that the adsorption capacity for Cu(II) actually increased from 26.8 to 39.1 mg/g at 140 mg/dm3. Therefore, the results would then suggest that adsorption of Cu(II) ions was actually enhanced in the presence of Pb(II). Results published in a separate paper by Yan et al. (Citation2010) also emphasizes the need for authors to thoroughly analyse their biosorption results. The authors reported that the biosorbent had no preference for Cu(II) and Pb(II) in spite of the differences in physicochemical properties of the metal ions. However, an analysis of results reported by the authors shows that the equilibrium sorption capacities were ≈ 0.15 and 0.17 mmol/g for Pb(II) and Cu(II), respectively. Although these quantities seem to be comparable (in mmol/g), the difference is significant when converted to mg/g, i.e., 31.1 and 11.1 mg/g for Pb(II) and Cu(II), respectively. This would actually lead to different conclusions, i.e., that the biosorbent had preferential sorption for Pb(II). Overall, therefore, these findings are significant in that despite the differences in biosorbents, experimental conditions, and models used to analyse the data, all biosorption systems analysed showed that Pb was preferentially removed from solution compared to the other metals. Such a general conclusion is an important contribution to the knowledge base on biosorption technology and has great potential to influence the design of the industrial process.
2.5. Application of response surface methodology
Further insights into biosorbent preference for metal sorption can be obtained by analysing findings from statistical methods such as Surface Response Methodology (SRM), which seeks to statistically explore the relationships between several explanatory variables and one or more response variables. It is evident from Table that most of the reported findings confirm the dominance of Pb over other metal ions during in biosorption studies. However, a few authors showed that some biosorbents preferentially adsorbed other metals in the presence of Pb. Rao and Khatoon (Citation2017) reported that for single-metal sorption using aluminate treated Casuarina equisetifolia leaves, adsorption capacities followed the order: Cu(II) (44.0 mg/g)>Ni(II) (39.0 mg/g)>Pb(II) (28.0 mg/g). It was also shown that adsorption capacities for single-metal sorption using Trichoderma viride decreased in the order of Cu(II) (0.360 mg/g)>Cd(II) (0.139 mg/g)>Pb(II) (0.103 mg/g) (Singh et al., Citation2010). Elsewhere, it was also reported that adsorption capacities for single-metal sorption followed the trend Cu(II) (204.1 mg/g)>Pb(II) (188.7)>Hg(II) (181.8 mg/g) (Alipanahpour Dil, Ghaedi, Ghezelbash, Asfaram, & Purkait, Citation2017).
Table 9. Major conclusions from selected studies applying Response Surface Methodology
2.6. Current and future perspectives
It was observed that a lot of data has been generated on multi-metal systems over the years. In particular, there exist published material on multi-metal systems with Pb(II) co-existing with other heavy metals, to allow reasonable conclusions on the influence of metal on sorption dynamics to be made. However, an exhaustive discussion of these systems can be hindered by limited data available for more complex systems, especially the quaternary system. More insights into the behaviour of the multi-metal systems would be realized if experiments on a given biosorbent are carried out under a combination of conditions indicated in Table , and also covering single, binary, ternary and quaternary sorption. This would allow more comprehensive and objective data analysis, e.g., how the total adsorption capacities would compare across different systems.
It can be noted that in more recent times, green engineered nanoscale zero-valent metals (NZVMs) have emerged as novel adsorbent materials offering unique advantages (Al-Qahtani, Citation2017; Fazlzadeh et al., Citation2017; Lu et al., Citation2016; Malacas et al., Citation2019). However, very limited research has been published on the application of the NZVMs in multi-metal systems. Mahmoud, Abdou, Mohamed, and Osman (Citation2016) showed that engineered Staphylococcus aureus immobilized on magnetic Fe3O4-phthalate nanoparticles showed high selectivity for Pb(II) compared to Ni(II) and Cu(II) ions. In related studies, non-competitive and competitive trials revealed that nanoscale zero-valent iron (NZVI) functionalized Posidonia oceanica marine biomass had a higher affinity for Cu(II) and Pb(II) compared to Cd(II). Given the potential for commercialization shown by NZVI-based biosorbents, it is projected that an increasing number of papers will be published in testing application of these systems for more complex multi-metal sorption. Some authors have already published such research, exemplified by Ojima et al. (Citation2019) who investigated recovery of metals from complex systems including rare earth metal ions such as Ce3+, Dy3+, Gd3+, La3+, Nd3+, Y3+, and Yb3+.
3. Conclusion
Unravelling the behaviour of Pb(II) is important in understanding sorption dynamics given its widely reported dominance in multi-metal systems. In this study, probes based on ratios of adsorption capacities revealed the tendency of Pb(II) ions to outcompete co-existing metal ions. It was shown that despite the differences in biosorbents and experimental conditions used, the ratio: was consistently less than unity, whereas
>
, thus confirming that adsorption was depressed due to co-existence of the metal ions in solution. An in-depth analysis using the Pb-Cu system showed that the ratios
and
were consistently greater than unity, thus confirming the superiority of Pb(II) as a sorbate across a wide range of biosorbents. Furthermore, Pb(II) contributed to more than 70% and 77% of the total adsorption capacity for the binary and ternary systems reviewed, and it was shown that the % change in adsorption capacity (
) was negative for all systems studied. For most biosorbents, Pb(II) is effectively removed from solution even in the presence of other heavy metals. However, the removal of Cu(II), Cd(II), Zn(II), Ni(II) and Cr(III) would be significantly suppressed in the presence of Pb(II). Such general conclusions are important in the designing and optimization of sorption processes utilizing the biosorption technology.
Conflict of Interest
The author has no conflict of interest.
Acknowledgements
The Author acknowledges training conducted by WIPO which enabled use of Research4Life resources.
Additional information
Funding
Notes on contributors
Courtie Mahamadi
Prof Courtie Mahamadi holds a Doctor of Philosophy Degree from the University of Zimbabwe. The author’s research interests are on studying alternative technologies for the removal of metals and organic pollutants from water through use of low-cost adsorbents. The author's work contributes to knowledge on production of green, low cost biosorbents using renewable materials such as abundant agricultural wastes, aquatic macrophytes and engineered nanoscale zero-valent metals (NZVMs). Emphasis is placed on the characterization of the adsorbents in terms of their physico-chemical properties and determination of their effectiveness in the removal of pollutants from water. Specifically, this study examines the superior behaviour of Pb(II) during removal from multi-metal batch systems using biosorbents.
References
- Abdulaziz, M., & Musayev, S. (2017). Multicomponent biosorption of heavy metals from aqueous solutions: A review. Polish Journal of Environmental Studies, 26, 1433–32. doi:10.15244/pjoes/67975
- Ahmad, R., & Haseeb, S. (2017). Adsorption of Pb(II) on Mentha piperita carbon (MTC) in single and quaternary systems. Arabian Journal of Chemistry, 10, S412–S421. doi:10.1016/j.arabjc.2012.09.013
- Akar, T., & Tunali, S. (2006). Biosorption characteristics of Aspergillus flavus biomass for removal of Pb(II) and Cu(II) ions from an aqueous solution. Bioresource Technology, 97, 1780–1787. doi:10.1016/j.biortech.2005.09.009
- Alipanahpour Dil, E., Ghaedi, M., Ghezelbash, G. R., Asfaram, A., & Purkait, M. K. (2017). Highly efficient simultaneous biosorption of Hg2+, Pb2+ and Cu2+ by Live yeast Yarrowia lipolytica 70562 following response surface methodology optimization: Kinetic and isotherm study. Journal of Industrial and Engineering Chemistry, 48, 162–172. doi:10.1016/j.jiec.2016.12.035
- Al-Qahtani, K. M. (2017). Cadmium removal from aqueous solution by green synthesis zero valent silver nanoparticles with Benjamina leaves extract. Egyptian Journal of Aquatic Research, 43, 269–274. doi:10.1016/j.ejar.2017.10.003
- Al-Rub, F. A. A., El-Naas, M. H., Ashour, I., & Al-Marzouqi, M. (2006). Biosorption of copper on Chlorella vulgaris from single, binary and ternary metal aqueous solutions. Process Biochemistry (barking, London, England), 41, 457–464. doi:10.1016/j.procbio.2005.07.018
- Amini, M., & Younesi, H. (2009). Biosorption of Cd(II), Ni(II) and Pb(II) from aqueous solution by dried biomass of aspergillus niger: Application of response surface methodology to the optimization of process parameters. Clean: Soil Air Water, 37, 776–786.
- Apiratikul, R., & Pavasant, P. (2006). Sorption isotherm model for binary component sorption of copper, cadmium, and lead ions using dried green macroalga, Caulerpa lentillifera. Chemical Engineering Journal, 119, 135–145. doi:10.1016/j.cej.2006.02.010
- Cao, Y. R., Liu, Z., Cheng, G. L., Jing, X. B., & Xu, H. (2010). Exploring single and multi-metal biosorption by immobilized spent Tricholoma lobayense using multi-step response surface methodology. Chemical Engineering Journal, 164, 183–195. doi:10.1016/j.cej.2010.08.055
- Chang, J.-S., Law, R., & Chang, -C.-C. (1997). Biosorption of lead, copper and cadmium by biomass of Pseudomonas aeruginosa PU21. Water Research, 31, 1651–1658. doi:10.1016/S0043-1354(97)00008-0
- Chew, S. Y., & Ting, A. S. Y. (2016). Biosorption behaviour of alginate-immobilized Trichoderma asperellum, a common microfungi in single and multi-metal systems. Separation and Purification Technology, 51, 743–748. doi:10.1080/01496395.2015.1130059
- Chong, K.-H., & Volesky, B. (1995). Description of 2-metal biosorption equilibria by Langmuir-type. Biotechnology and Bioengineering, 47, 451–460. doi:10.1002/bit.260470406
- Costa, C. S. D., Da Silva, M. G. C., & Vieira, M. G. A. (2018). Investigation of the simultaneous biosorption of toxic metals through a mixture design application. Journal of Cleaner Production, 200, 890–899. doi:10.1016/j.jclepro.2018.07.314
- Deng, J., Liu, Y., Liu, S., Zeng, G., Tan, X., Huang, B., … Yan, Z. (2017). Competitive adsorption of Pb(II), Cd(II) and Cu(II) onto chitosan-pyromellitic dianhydride modified biochar. Journal of Colloid and Interface Science, 506, 355–364. doi:10.1016/j.jcis.2017.07.069
- El-Naas, M. H., Al-Rub, F. A., Ashour, I., & Al Marzouqi, M. (2007). Effect of competitive interference on the biosorption of lead(II) by Chlorella vulgaris. Chemical Engineering and Processing: Process Intensification, 46, 1391–1399. doi:10.1016/j.cep.2006.11.003
- Engl, A., & Kunz, B. (1995). Biosorption of heavy metals by Saccharomyces cerevisiae: Effects of nutrient conditions. Journal of Chemical Technology & Biotechnology, 63, 257–261. doi:10.1002/(ISSN)1097-4660
- Escudero-Oñate, C., Poch, J., & Villaescusa, I. (2017). Adsorption of Cu(II), Ni(II), Pb(II) and Cd(II) from ternary mixtures: Modelling competitive breakthrough curves and assessment of sensitivity. Environmental Science: Processes & Impacts, 4, 833–849. doi:10.1007/s40710-017-0262-7
- Fan, C., Li, K., Juexiu, L., Ying, D., Wang, Y., & Jia, J. (2017). Comparative and competitive adsorption of Pb(II) and Cu(II) using tetraethylenepentamine modified chitosan/CoFe2O4 particles. Journal of Hazardous Materials, 326, 211–220. doi:10.1016/j.jhazmat.2016.12.036
- Fazlzadeh, M., Rahmani, K., Zarei, A., Abdoallahzadeh, H., Nasiri, F., & Khosravi, R. (2017). A novel green synthesis of zero valent iron nanoparticles (NZVI) using three plant extracts and their efficient application for removal of Cr(VI) from aqueous solutions. Advanced Powder Technology, 28, 122–130. doi:10.1016/j.apt.2016.09.003
- Fiol, N., Villaescusa, I., Martinez, M., Miralles, N., Poch, J., & Serarols, J. (2006). Sorption of Pb(II), Ni(II), Cu(II) and Cd(II) from aqueous solution by olive stone waste. Separation and Purification Technology, 50, 132–140. doi:10.1016/j.seppur.2005.11.016
- Fomina, M., & Gadd, G. M. (2014). Biosorption: Current perspectives on concept, definition and application. Bioresource Technology, 160, 3–14. doi:10.1016/j.biortech.2013.12.102
- Fourest, E., & Roux, J.-C. (1992). Heavy metal biosorption by fungal mycelial by-products: Mechanisms and influence of pH. Applied Microbiology and Biotechnology, 37, 399–403. doi:10.1007/BF00211001
- Fritz, W., & Schluender, E. U. (1974). Simultaneous adsorption equilibria of organic solutes in dilute aqueous solutions on activated carbon. Chemical Engineering Science, 29, 1279–1282. doi:10.1016/0009-2509(74)80128-4
- Gupta, V. K., & Rastogi, A. (2008). Biosorption of lead from aqueous solutions by green algae Spirogyra species: Kinetics and equilibrium studies. Journal of Hazardous Materials, 15, 407–414. doi:10.1016/j.jhazmat.2007.07.028
- Hammaini, A., Ballester, A., Blázquez, M. L., González, F., & Munõz, J. (2002). Effect of the presence of lead on the biosorption of copper, cadmium and zinc by activated sludge. Hydrometallurgy, 67, 109–116. doi:10.1016/S0304-386X(02)00157-3
- Hawari, A. H., & Mulligan, C. N. (2007). Effect of the presence of lead on the biosorption of copper, cadmium and nickel by anaerobic biomass. Process Biochemistry (barking, London, England), 42, 1546–1552. doi:10.1016/j.procbio.2007.08.009
- Holan, Z. R., & Volesky, B. (1994). Biosorption of lead and nickel by biomass of marine algae. Biotechnology and Bioengineering, 43, 1001–1009. doi:10.1002/bit.260431102
- Hossain, M. A., Ngo, H. H., Guo, W. S., Nghiem, L. D., Hai, F. I., Vigneswaran, F. I., & Nguyen, T. V. (2014). Competitive adsorption of metals on cabbage waste from multi-metal solutions. Bioresource Technology, 160, 79–88. doi:10.1016/j.biortech.2013.12.107
- Jiang, L., Zhou, W., Liu, D., Liu, T., & Wang, Z. (2017). Biosorption isotherm study of Cd2+, Pb2+ and Zn2+ biosorption onto marine bacterium Pseudoalteromonas sp. SCSE709-6 in multiple systems. Journal of Molecular Liquids, 24, 7230–7237.
- Kamar, F. H., Nechifor, A. C., Nechifor, G., Al-Musawi, T. J., & Mohammed, A. H. (2016). Aqueous phase biosorption of Pb(II), Cu(II), and Cd(II) onto cabbage leaves powder. International Journal of Chemical Reactor Engineering, 15, 2194–5748.
- Kapoor, A., & Viraraghavan, T. (1995). Fungal biosorption-an alternative treatment option for heavy metal bearing wastewaters: A review. Bioresource Technology, 53, 195–206.
- Kavand, M., Soleimani, M., Kaghazchi, T., & Asasian, N. (2016). Competitive separation of lead, cadmium, and nickel from aqueous solutions using activated carbon: Response surface modeling, equilibrium, and thermodynamic studies. Chemical Engineering Communications, 203, 123–135. doi:10.1080/00986445.2014.962691
- Khajeh, M., Laurent, S., & Dastafkan, K. (2013). Nanoadsorbents: Classification, preparation, and applications (with emphasis on aqueous media). Chemical Reviews, 113, 7728–7768. doi:10.1021/cr400086v
- Kongsuwan, A., Patnukao, P., & Pavasant, P. (2009). Binary component sorption of Cu(II) and Pb(II) with activated carbon from Eucalyptus camaldulensis Dehn bark. Journal of Industrial and Engineering Chemistry, 15, 465–470. doi:10.1016/j.jiec.2009.02.002
- Krishnani, K. K., Meng, X., Christodoulatos, C., & Boddu, V. M. (2008). Biosorption mechanism of nine different heavy metals onto biomatrix from rice husk. Journal of Hazardous Materials, 153, 1222–1234. doi:10.1016/j.jhazmat.2007.09.113
- Krstic´, V., Uroševic´, T., & Pešovski, B. (2018). A review on adsorbents for treatment of water and wastewaters containing copper ions. Chemical Engineering Science, 192, 273–287. doi:10.1016/j.ces.2018.07.022
- Kumar, D., Singh, A., & Gaur, J. P. (2008). Mono-component versus binary isotherm models for Cu(II) and Pb(II) sorption from binary metal solution by the green alga Pithophora oedogonia. Bioresource Technology, 99, 8280–8287. doi:10.1016/j.biortech.2008.03.008
- Lu, H., Wang, J., Stoller, M., Wang, T., Bao, Y., & Hao, H. (2016). An overview of nanomaterials for water and wastewater treatment. Advances in Materials Science and Engineering, ID 4964828, 10. doi:10.1155/2016/4964828
- Lu, W.-B., Kao, W.-C., Shi, -J.-J., & Chang, J.-S. (2008). Exploring multi-metal biosorption by indigenous metal-hyperresistant Enterobacter sp. J1 using experimental design methodologies. Journal of Hazardous Materials, 153, 372–381. doi:10.1016/j.jhazmat.2007.08.059
- Mahamadi, C., & Nharingo, T. (2010). Competitive adsorption of Pb2+, Cd2+ and Zn2+ ions onto Eichhornia crassipes in binary and ternary systems. Bioresource Technology, 101, 859–864. doi:10.1016/j.biortech.2009.08.097
- Mahdi, Z., Yu, Q. J., & El Hanandeh, A. (2018). Competitive adsorption of heavy metal ions (Pb2+, Cu2+, and Ni2+) onto date seed biochar: Batch and fixed bed experiments. Separation and Purification Technology. doi:10.1080/01496395.2018.1523192
- Mahmoud, M. E., Abdou, A. E., Mohamed, S. M., & Osman, M. M. (2016). Engineered Staphylococcus aureus via immobilization on magnetic Fe3O4-phthalate nanoparticles for biosorption of divalent ions from aqueous solutions. Journal of Environmental Chemical Engineering, 4, 3810–3824. doi:10.1016/j.jece.2016.08.022
- Maity, J., & Ray, S. K. (2018). Chitosan based nano composite adsorbent-Synthesis, characterization and application for adsorption of binary mixtures of Pb(II) and Cd(II) from water. Carbohydrate Polymers, 182, 159–171. doi:10.1016/j.carbpol.2017.10.086
- Malacas, M., Balberan, M. C., Bederi, N. A. J., Ramos, C. J., Rato, M., Salazar, A. G., & Roque, E. (2019). The removal of copper (II) and lead (II) from aqueous solution using Fuller’s earth and Fuller’s earth-immobilized nanoscale zero valent iron (FE-NZVI) by adsorption. MATEC Web of Conferences, 268, 05006. doi:10.1051/matecconf/201926805006
- Martín-Lara, M. A., Blázquez, G., Calero, M., Almendros, A. I., & Ronda, A. (2016). Binary biosorption of copper and lead onto pine cone shell in batch reactors and in fixed bed columns. International Journal of Mineral Processing, 148, 72–82. doi:10.1016/j.minpro.2016.01.017
- Medellin-Castillo, N. A., Padilla-Ortega, E., Regules-Martínez, M. C., Leyva-Ramos, R., Ocampo-Pérez, R., & Carranza-Alvarez, C. (2017). Single and competitive adsorption of Cd(II) and Pb(II) ions from aqueous solutions onto industrial chili seeds (Capsicum annuum) waste. Sustainable Environment Research, 27, 61–69. doi:10.1016/j.serj.2017.01.004
- Módenes, A. N., Espinoza-Quiñones, F. R., Colombo, A., Geraldi, C. L., & Trigueros, D. E. G. (2015). Inhibitory effect on the uptake and diffusion of Cd2+ onto soybean hull sorbent in Cd-Pb binary sorption systems. Journal of Environmental Management, 154, 22–32. doi:10.1016/j.jenvman.2015.02.022
- Muthusamy, S., & Venkatachalamb, S. (2015). Competitive biosorption of Cr(VI) and Zn(II) ions in single- and binary-metal systems onto a biodiesel waste residue using batch and fixed-bed column studies. RSC Advances, 5, 45817–45826. doi:10.1039/C5RA05962C
- Naja, G., Vanessa, M., & Volesky, B. (2010). Biosorption, metal, encyclopedia of industrial biotechnology: Bioprocess, bioseparation, and cell technology. New York, NY: Wiley.
- Ofomaja, A. E, Naidoo, E, & Modise, S. (2010a). Biosorption of copper(ii) and lead(ii) onto potassium hydroxide treated pine cone powder. Journal of Environmental Management, 91(8), 1674-1685.
- Ofomaja, A. E., Unuabonah, E. I., & Oladoja, N. A. (2010b). Competitive modeling for the biosorptive removal of copper and lead ions from aqueous solution by Mansonia wood sawdust. Bioresource Technology, 101, 3844–3852. doi:10.1016/j.biortech.2009.10.064
- Ojima, Y., Kosako, S., Kihara, M., Miyoshi, N., Igarashi, K., & Azuma, M. (2019). Recovering metals from aqueous solutions by biosorption onto phosphorylated dry baker’s yeast. Scientific Reports, 9(1). doi:10.1038/s41598-018-36306-2
- Park, J. H., & Chon, H.-T. (2016). Characterization of cadmium biosorption by Exiguobacterium sp. isolated from farmland soil near Cu-Pb-Zn mine. Environmental Science and Pollution Research, 23, 11814–11822. doi:10.1007/s11356-016-6335-8
- Rao, R. A. K., & Khatoon, A. (2017). Aluminate treated Casuarina equisetifolia leaves as potential adsorbent for sequestering Cu(II), Pb(II) and Ni(II) from aqueous solution. Journal of Cleaner Production, 165, 1280–1295. doi:10.1016/j.jclepro.2017.07.160
- Ray, J., Jana, S., Bhanja, S. K., & Tripathy, T. (2018). Efficient removal of Co(II), Ni(II), and Zn(II) metal ions from binary and ternary solutions using a pH responsive bifunctional graft copolymer. Colloid and Polymer Science, 296, 1275–1291. doi:10.1007/s00396-018-4345-4
- Rodrigues, M. S., Ferreira, L. S., de Carvalho, J. C. M., Lodi, A., Finocchio, E., & Converti, A. (2012). Metal biosorption onto dry biomass of Arthrospira (Spirulina) platensis and Chlorella vulgaris: Multi-metal systems. Journal of Hazardous Materials, 217–218, 246–255. doi:10.1016/j.jhazmat.2012.03.022
- Ronda, A., Martín-Lara, M. A., Dionisio, E., Blázquez, G., & Calero, M. (2013). Effect of lead in biosorption of copper by almond shell. Journal of the Taiwan Institute of Chemical Engineers, 44, 466–473. doi:10.1016/j.jtice.2012.12.019
- Saeed, A., Iqbal, M., & Akhtar, M. W. (2005). Removal and recovery of lead(II) from single and multimetal (Cd, Cu, Ni, Zn) solutions by crop milling waste (black gram husk). Journal of Hazardous Materials, 117, 65–73. doi:10.1016/j.jhazmat.2004.09.008
- Saeid, A., & Chojnacka, K. (2015). Multi-cation biosorption by Chlorella kessleri. Open Chemistry, 13, 959–966. doi:10.1515/chem-2015-0117
- Sari, A., & Tuzen, M. (2009). Kinetic and equilibrium studies of biosorption of Pb(II) and Cd(II) from aqueous solution by macrofungus (Amanita rubescens) biomass. Journal of Hazardous Materials, 164, 1004–1011. doi:10.1016/j.jhazmat.2008.09.002
- Sellaoui, L., Franco, D. S. P., Dotto, G. L., Lima, É. C., & Lamine, A. B. (2017). Single and binary adsorption of cobalt and methylene blue on modified chitin: Application of the Hill and exclusive extended Hill models. Journal of Molecular Liquids, 233, 543–550. doi:10.1016/j.molliq.2016.10.079
- Şengil, I. A., & Özacar, M. (2009). Competitive biosorption of Pb2+, Cu2+ and Zn2+ ions from aqueous solutions onto Valonia tannin resin. Journal of Hazardous Materials, 166, 1488–1494. doi:10.1016/j.jhazmat.2008.12.071
- Şener, M., Reddy, D. H. K., & Kayan, B. (2014). Biosorption properties of pretreated sporopollenin biomass for lead(II) and copper(II): Application of response surface methodology. Ecological Engineering, 68, 200–208. doi:10.1016/j.ecoleng.2014.03.024
- Sheintuch, M., & Rebhun, M. (1988). Adsorption isotherms for multi-solute systems with known and unknown composition. Water Research, 22, 421–430. doi:10.1016/0043-1354(88)90036-X
- Shirsath, D. S., & Shirivastava, V. S. (2015). Adsorptive removal of heavy metals by magnetic nanoadsorbent: An equilibrium and thermodynamic study. Applied Nanoscience, 5, 927–935. doi:10.1007/s13204-014-0390-6
- Singh, R., Chadetrik, R., Kumar, R., Bishnoi, K., Bhatia, D., Kumar, A., … Singh, N. (2010). Biosorption optimization of lead(II), cadmium(II) and copper(II) using response surface methodology and applicability in isotherms and thermodynamics modeling. Journal of Hazardous Materials, 174, 623–634. doi:10.1016/j.jhazmat.2009.09.097
- Sulaymon, A. H., Ebrahim, S. E., & M-Ridha, M. J. (2014). Competitive biosorption of Pb(II), Cr(III), and Cd(II) from synthetic wastewater onto heterogeneous anaerobic biomass in single, binary, and ternary batch systems. Desalination and Water Treatment, 52, 5629–5638. doi:10.1080/19443994.2013.813008
- Sulaymon, A. H., Mohammed, A. A., & Al-Musawi, T. J. (2013). Competitive biosorption of lead, cadmium, copper, and arsenic ions using algae. Environmental Science and Pollution Research, 20, 3011–3023. doi:10.1007/s11356-012-1208-2
- Suzaki, P. Y. R., Munaro, M. T., Triques, C. C., Kleinübing, S. J., Klen, M. R. F., Jorge, L. M. M., & Bergamasco, R. (2017). Biosorption of binary heavy metal systems: Phenomenological mathematical modelling. Chemical Engineering Journal, 313, 364–373. doi:10.1016/j.cej.2016.12.082
- Uogintė, I., Lujanienė, G., & Mažeika, K. (2019). Study of Cu (II), Co (II), Ni (II) and Pb (II) removal from aqueous solutions using magnetic Prussian blue nano-sorbent. Journal of Hazardous Materials, 369, 226–235. doi:10.1016/j.jhazmat.2019.02.039
- Vijayaraghavan, K., & Raja, F. D. (2014). Experimental characterisation and evaluation of perlite as a sorbent for heavy metal ions in single and quaternary solutions. Journal of Water Process Engineering, 4, 179-184.
- Vijayaraghavan, K., Rangabhashiyam, S., Ashokkumar, T., & Arockiaraj, J. (2016). Mono- and multi-component biosorption of lead(II), cadmium(II), copper(II) and nickel(II) ions onto coco-peat biomass. Separation and Purification Technology, 51, 2725–2733. doi:10.1080/01496395.2016.1212889
- Vijayaraghavan, K., & Yun, Y.-S. (2008). Bacterial biosorbents and biosorption. Biotechnology Advances, 26, 266–291. doi:10.1016/j.biotechadv.2008.02.002
- Wang, J. L., & Chen, C. (2006). Biosorption of heavy metals by Saccharomyces cerevisiae: A review. Biotechnology Advances, 24, 427–451. doi:10.1016/j.biotechadv.2006.03.001
- Wang, J. L., & Chen, C. (2009). Biosorbents for heavy metals removal and their future a review. Biotechnology Advances, 27, 195–226. doi:10.1016/j.biotechadv.2008.11.002
- Wang, S., Vincent, T., Faur, C., & Guibal, E. (2017). Modeling competitive sorption of lead and copper ions onto alginate and greenly prepared algal-based beads. Bioresource Technology, 231, 26–35. doi:10.1016/j.biortech.2017.01.066
- Wang, T., & Sun, H. (2013). Biosorption of heavy metals from aqueous solution by UV-mutant Bacillus subtilis. Environmental Science and Pollution Research (international), 20, 7450–7463. doi:10.1007/s11356-013-1767-x
- Wang, X., Guo, Y., Yang, L., Han, M., Zhao, J., & Cheng, X. (2012). Nanomaterials as sorbents to remove heavy metal ions in wastewater treatment. Journal of Environmental & Analytical Toxicology, 2, 2–7. doi:10.4172/2161-0525.1000154
- Xie, P., Hao, X., Mohamad, O. A., Liang, J., & Wei, G. (2013). Comparative study of chromium biosorption by Mesorhizobium amorphae strain CCNWGS0123. Applied Biochemistry and Biotechnology, 169, 570–587. doi:10.1007/s12010-012-9976-1
- Yan, C., Li, G., Xue, P., Wei, Q., & Li, Q. (2010). Competitive effect of Cu(II) and Zn(II) on the biosorption of lead(II) by Myriophyllum spicatum. Journal of Hazardous Materials, 179, 721–728. doi:10.1016/j.jhazmat.2010.03.061
- Yan, G., & Viraraghavan, T. (2001). Heavy metal removal in a biosorption column by immobilized M. rouxii biomass. Bioresource Technology, 78, 243–249.
- Yu, K., Ho, J., McCandlish, E., Buckley, B., Patel, R., Li, Z., & Shapley, N. C. (2013). Copper ion adsorption by chitosan nanoparticles and alginate microparticles for water purification applications. Colloids and Surfaces A: Physicochemical and Engineering Aspects, 425, 31–41. doi:10.1016/j.colsurfa.2012.12.043
- Zhu, Y., Hu, J., & Wang, J. (2012). Competitive adsorption of Pb(II), Cu(II) and Zn(II) onto xanthate-modified magnetic chitosan. Journal of Hazardous Materials, 221–222, 155–161. doi:10.1016/j.jhazmat.2012.04.026