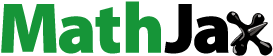
Abstract
Mine tailings are the byproducts of the mining industry. For many decades these tailings used to be stored in specially formed tailings ponds. This method of storage has caused several environmental disasters, coupled in some instances with human fatalities. These catastrophes prompted a change in the management strategy of tailings. This change started with attempting to solidify the tailings to immobilize them and reduce their hazard. As part of this new strategy, and in an effort to investigate the applicability of using mine tailings as a construction material, this study is an attempt to investigate the porosity dependent strength and leachability of newly formed mine tailings matrices. And to evaluate the applicability of using four different theoretical models to predict the strength of these matrices. For this purpose, two mine tailings, Musselwhite and Mont Wright, from the Eastern parts of Canada have been selected. In this study, solidification of the newly formed tailings matrices was investigated using Ordinary Portland cement, fly ash, slag and the new binder Calsifrit. Results show that using Calsifrit as a partial cement replacement, in addition to slag and fly ash, reduced the porosity of the tailings matrices. The leaching test showed that several types of heavy metals have a high percentage of retention in these matrices. Compressive strength results indicate that the tailings matrices are suitable as construction materials for certain structural applications. However, current theoretical models overpredict their strength and overprediction increases when fly ash or slag are added to the mix.
PUBLIC INTEREST STATEMENT
With the advent of new communication technologies and the proliferation of knowledge in all sectors, the public is more aware now of the challenges facing the environment. As such more emphasis has now been placed on regulatory bodies to establish guidelines for the enforcement of sustainable practices. The mining industry in Canada, which is one of the biggest in the world, is one such industry that has been implementing sustainable practices in its field. Sustainable construction materials made from mine waste is a must, if we are to move forward with reducing environmental pollution. Hence, this paper discusses the potential characteristics of such materials in view of their strength and environmental characteristics.
Competing Interests
The authors declare no competing interests.
1. Introduction
Traditionally, mine tailings have been treated as a waste material that requires landfilling inside specially created ponds surrounded by tailings dams. This approach has been practiced for many decades until these dams started breaking up and causing substantial environmental damages, associated in several instances with human fatalities (Fourie et al., Citation2001; Fourie & Papageorgiou, Citation2001). In the last two decades or so there has been a new trend towards the sustainable use of these tailings rather than treating them as a waste that requires dumping. Examples include the works of Garand et al. (Citation2000), Benzaazoua et al. (Citation2002), Theriault et al. (Citation2003), Roy et al. (Citation2007), Helinski et al. (Citation2010), Zhang (Citation2012), Ugama et al. (Citation2014), Sabat et al. (Citation2015), Manjarrez and Zhang (Citation2018), Mahmood and Elektorowicz (Citation2018a, Citation2018b, Citation2018c), Lottermoser (Citation2019), Davis and Cumbers (Citation2019), Lake (Citation2019), Xue et al. (Citation2020), Han et al. (Citation2020), and Obenaus-Emler et al. (Citation2020).
Therefore, as an approach to prevent mine tailings disasters from taking place, solidification of mine tailings is investigated using several binders and additives. This approach is part of an integrated experimental-computational program to determine their index and engineering properties and analyze their characteristics as construction materials. In this study, a novel binder called Calsifrit™ was applied in comparison to traditionally used Portland cement, fly ash, and slag to solidify two different types of mine tailings from Eastern Canada, namely, Mont Wright and Musselwhite. The objective of this study is to evaluate the potential use of the newly formed binders-tailings matrices as construction materials by evaluating the applicability of using theoretical models to predict their porosity dependent strength. This will be done through the incorporation of a new additive called Calsifrit, and comparing it with Portland cement, fly ash and slag in terms of strength, leachability and porosity.
2. Methodology
2.1. Materials
2.1.1. The tailings
Two types of mine tailings were selected for experimentation: Musselwhite tailings from Placer Dome (gold) mine, in Musselwhite, Ontario, and Mont Wright tailings from Quebec Cartier mineral company, from the Mont Wright (iron) mine, in Quebec.
These two tailings were chosen due to their availability in northeastern Canada; in proximity to the area where Plan Nord will be employed. Table shows their physical properties. Where D10 = diameter corresponding to 10% finer, D50 = diameter corresponding to 50% finer, D60 = diameter corresponding to 60% finer, D30 = diameter corresponding to 30% finer, Cu = uniformity coefficient = D60/D10, Cz = coefficient of gradation = D30 2/(D10 D60), P4.75 mm (%) = percentage passing sieve no.4, P 0.075 mm (%) = percentage passing ASTM sieve # 200, SW-SM = well-graded sand with silt, SM = silty sand, SP = poorly graded sand, USCS (Das, Citation2000) = unified soil classification system. Specific gravity values are 2.76 and 3.26 for Mont Wright and Musselwhite tailings, respectively. It can be seen that according to the unified soil classification system (Das, Citation2000), Musselwhite is classified as sandy silts while Mont Wright tailings are poorly graded sand. Mont Wright tailings are coarse-textured grayish purple silty sand with traces of gravel.
Table 1. Physical properties of the tailings
The mineralogical composition of Mont Wright tailings is Sandstone (quartzite), Mica schist, Amphibolite, Gabroïque Granite, Gabroïque Feldsphate, Specular Hematite, Specular Magnetite, Quartz, Diopside, Tremolite, Actinolite and Gruenerite. While Musselwhite tailings are composed of Quartz (SiO2), Birnessite-syn (MnO2), Calcium manganese oxide hydrate (Ca2Mn14O27.xH2O) and Dannemorite (Fe,Mg,Mn)7Si8O22(OH)2. Loss on ignition test conducted for both Mont Wright and Musselwhite tailings revealed no indication of organic matter.
2.1.2. Binding materials
Type I ordinary Portland cement (OPC), fly ash, slag and Calsifrit are used as the binding materials in this study.
The fly ash used was obtained from St. Laurence Cement located in Longueuil, Quebec. Tests reveal that the unconfined compressive strength of fly ash lies between 1.38 and 6.895 MPa and its permeability is between 10−5 and 10−8 cm/s (Conner, Citation1990). Ground granulated blast furnace slag (GGBFS) used was obtained from Lafarge North America located in Montreal, Quebec. It was produced in accordance with ASTM C 989 (Citation2006). It is composed of (30–50)% Calcium Oxide (0–20)% Magnesium Oxide with less than 1% Crystalline Silica. Its specific gravity is between 2 and 3, water pH 8–11. Its boiling point is above 1000°C (Lafarge North America, Citation2007).
Calsifrit™ is defined as a totally amorphous siliceous material. It is composed of calcium and sodium fluoro-aluminosilicate. This homogeneous solid material has a blackish grey color, possessing high reactivity potential and cementitious properties when finely ground. This material was obtained from its manufacturer NovaFrit International. Calsifrit has a pH (1% solution in water) of 6.5–7.5, a melting point of 800°C. Its specific gravity is 1.3 and has a moisture content of less than 10% (NovaFrit International, Citation2006).
2.2. Experiments
2.2.1. Formulation of tailings matrices and compression of 5 cm3 cube specimens
Each type of tailings was mixed with the type I ordinary Portland cement, Calsifrit, fly ash and slag using a special procedure. Cement was present as the main binder in all mixes; however, a ratio of 5% to 25% of it was substituted with Calsifrit, Calsifrit and slag or Calsifrit and fly ash. This was done to maintain a solid matrix in all mixture types. These mixtures were, then, poured in the 50-mm cubes for curing in the moisture chamber for 1, 7 and 28 days. Then, they were subjected to compression testing according to ASTM C 109/C 109 M (Citation1999). Compression testing was performed on the 50-mm cubes through the application of monotonic compression loads until visual signs of failure were noticed.
2.2.2. Tailings metal content
Atomic absorption analysis (Table ) performed on the tailings prior to testing showed that both tailings contain a high amount of metals particularly iron (up to 270 g/kg) and copper (1 g/kg).
Table 2. Results of the atomic absorption analysis of the tailings
2.2.3. Toxicity characteristics leaching procedure (TCLP)
This is a regulatory test designed to assist in waste classification designated as EPA 1311 (USEPA Method Citation1311, 1992). After solidification of the tailings matrices, TCLP was conducted to find traces of either one of these heavy metals: Cr, Cu, Ni, Zn, Fe and Pb to determine the effect that solidification has on the new matrices. The metal content of the extract, for the series of heavy metals investigated, was determined using a Perkin-Elmer Analyst 100 atomic absorption spectrometer.
2.3. Equations
Several researchers attempted to investigate the effect porosity has on strength and the mathematical relation between them. Balshin (Citation1949), from his study of the tensile strength of metal ceramics, suggested the relation
where σ is the strength, σo is the strength at zero porosity, b is the empirical constant.
While Ryshkevitch (Citation1953), from a study of the compressive strength of Al2O3 and ZrO2, obtained the relation
where k is the empirical constant.
Similarly, Schiller (Citation1971), on the basis of the study of set sulfate plasters, proposed the relation:
where n is the empirical constant, po is the porosity at zero strength.
While Hasselman (Citation1969) suggested the equation of a linear relationship between strength and porosity for different refractory materials:
where c is the empirical constant.
More recently, Chen et al. (Citation2013) studied several equations relating porosity to the compressive strength of cement-based materials. They proposed an equation based on the extended Zheng model (Zheng et al., Citation1992) to predict the compressive strength based on porosity for cement mortars:
where
P: porosity
Pc: percolation porosity at failure threshold
σo: Strength at zero porosity
Through experimentation, these researchers were able to come up with the following values for these coefficients:
Pc: 0.562, σo: 69.4 MPa(Chen et al., Citation2013)
σo = 70.8 MPa, b = 2.91 (Balshin, Citation1949)
σo = 77 MPa, k = 3.74 (Ryshkevitch, Citation1953)
n = 26.3, Po = 0.78 (Schiller, Citation1971)
σo = 62.6 MPa, c = 123.1 (Hasselman, Citation1969)
Porosity (P) of the solidified mine tailings matrices is calculated according to the following equation (Stegemann & Côté, Citation1991):
where
BD = bulk density,
w = moisture content,
Gs = specific gravity,
d = density of water (= 1 g/cm3).
These equations (1–5) have never been tested for mine tailings matrices; hence, the current study aims to explore their applicability in predicting the strength of mine tailings matrices. Details of the full experimental and computational program can be found elsewhere (Mahmood, Citation2012).
3. Results and discussion
3.1. Porosity
Porosity was calculated for the 5 cm3 cubes with curing periods of 1, 7 and 28 days. Porosity values are presented in Figures – for the Mont Wright and Musselwhite tailings matrices. Porosity for Mont Wright matrices ranged from 0.235 to 0.401 and porosity for Musselwhite matrices ranged from 0.32 to 0.427.
It can be seen in Figures – that Musselwhite matrices have higher porosity values than Mont Wright tailings matrices. Furthermore, these figures indicate a declining trend in the experimental strength values, with the increase in porosity. This can be explained by the fact that Musselwhite tailings, in comparison to Mont Wright, had higher initial water content as can be seen in Table . Conner (Citation1990) reported that as the water/cement ratio increased, the percentage of large pores and hence the permeability of the product increased. It is established that strength decreases as matrix porosity increases, and that porosity increases as the water to cement ratio increases above an optimal value (Lea, Citation1971; Neville, Citation1995). Increasing the Calsifrit ratio in the matrices had generally led to a decrease in the porosity of the Musselwhite tailings matrices. Whereby, for Mont Wright tailings matrices it seemed that the optimum Calsifrit percentage with the least porosity was 10% of the total percentage of binder used. A University of Sherbrook report established that using 25% Calsifrit as a Portland cement substitute decreased the permeability of concrete to chloride ion by 50%. Calsifrit does this by promoting a discontinuous pore structure (NovaFrit NovaFrit International, Citation2006). Although pozzolans are known accelerators of C3 S hydration (Massazza, Citation1998), they themselves hydrate slowly. Pozzolanic cements tend to gain strength more slowly than Portland cement unless an activator is used (Stegemann, Citation2001). Using Calsifrit in addition to slag and fly ash reduced the permeability and improved the strength and durability of the tailings matrices, in accordance with other research studies on pozzolans (Stegemann, Citation2001). Reduced permeability is a factor in materials performance since it will, in turn, reduce water infiltration to the groundwater aquifer, which leads to more sustainable construction materials. Water and leachates infiltration needs to go to a minimum, within regulatory standards, for any construction material to pass through the approval stage. So far, the use of Calsifrit, slag and fly ash has proven beneficial in this regard. Using these pozzolanic materials has the ultimate goal of reducing the dependence on Portland cement Production of Portland cement is known to be the biggest anthropogenic source of carbon dioxide (CO2) emissions into the environment (Andrew, Citation2018). Hence, the need for alternative materials with similar functions and less environmental pollution. Figure shows two microscopic images of (a) Mont Wright (75% OPC, 15% S, 10% CF (1day)), and (b) Musselwhite tailings matrices (75% OPC, 15% S, 10% CF (1 day)).
3.2. Theoretical models
Figures – show the strength as predicted by porosity values using the models of Chen et al. (Citation2013), Ryshkevitch (Citation1953), Schiller (Citation1971), Balshin (Citation1949), and Hasselman (Citation1969). The experimental test results are included, as well. It seems that these models generally overpredict the strength of mine tailings matrices based on porosity values. This could be due to several factors. One such factor is the heavy metals content of these tailings that acts to retard hydration, as was thoroughly discussed by Mahmood (Citation2012). It could also be due to the fact that porosity was indirectly calculated by the use of Stegemeann’s equation (Stegemann & Côté, Citation1991). Measured porosity might have different values. Another explanation lies in the fact that these models were developed for different materials. Ryshkevitch’s (Citation1953) model was developed from a study of the compressive strength of Al2O3 and ZrO2. Schiller’s (Citation1971) model was developed based on a study of set sulfate plasters, Hasselman’s (Citation1969) model was the result of a study on refractory materials, while Balshin’s (Citation1949) model was developed based on a study of metal ceramics. No account was given, in these models, on the effect impurities have on strength.
Figure 6. The strength test results of Mont Wright Tailings Matrices and the predicted strength using the models of Chen et al. (Citation2013), Ryshkevitch (Citation1953), Schiller (Citation1971), Balshin (Citation1949), and CitationHasselman(Citation1969)
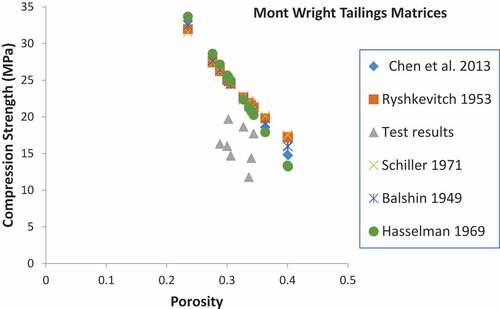
Figure 7. The strength test results of Mont Wright Pozzolanic Matrices and the predicted strength using the models of Chen et al. (Citation2013), Ryshkevitch (Citation1953), Schiller (Citation1971), Balshin (Citation1949), and CitationHasselman(Citation1969)
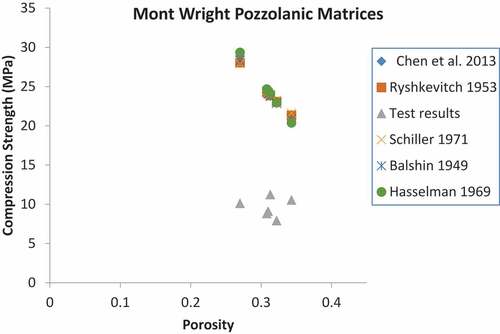
Figure 8. The strength test results of Musselwhite Tailings Matrices and the predicted strength using the models Chen et al. (Citation2013), Ryshkevitch (Citation1953), Schiller (Citation1971), Balshin (Citation1949), and CitationHasselman(Citation1969)
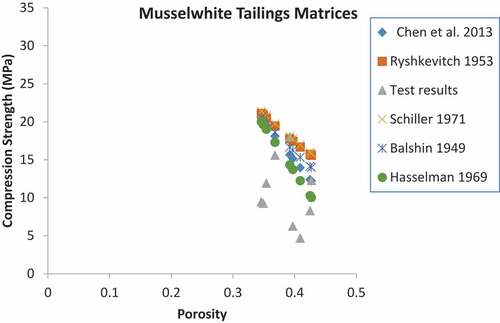
Figure 9. The strength test results of Musselwhite Pozzolanic Matrices and the predicted strength using the models of Chen et al. (Citation2013), Ryshkevitch (Citation1953), Schiller (Citation1971), Balshin (Citation1949), and CitationHasselman(Citation1969)
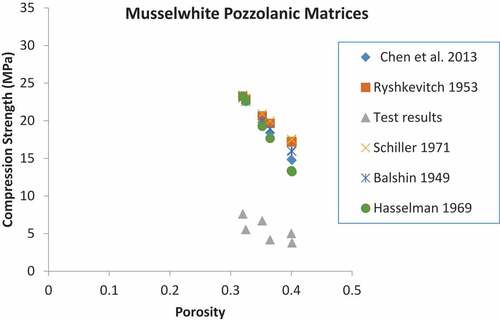
Although the model of Chen et al. (Citation2013) was developed based on a study of cement mortars, to overcome the shortcomings of the previous models, no account was given of the effect impurities and pozzolanic materials have on strength. Such effect is manifested in the current experimental results. It is seen in Figures – that the presence of fly ash and slag had a reducing effect on the overall compressive strength of the tailings matrices. While all the theoretical models discussed over predict the compressive strength of these matrices, it is shown that overprediction increases when these pozzolanic materials are added to the mix. Compression strength values for Calsifrit-cement tailings matrices seem to be reasonably close to the values predicted by these models. This suggests that the chemical and reaction properties of Calsifrit resemble those of cement to a great extent. Compressive strength values shown in Figures – matched reasonably well with published values in the literature (Fernández Olmo et al., Citation2003; Gervais & Ouki, Citation2002; Polettini et al. Citation2001, Citation2002; Stegemann et al., Citation2001), for a similar type of cement-hardened cubes containing heavy metal impurities. The Portland Cement Association (PCA) specifies a minimum compressive strength of 2.4 MPa for mortar cement type O used for bricks, blocks and stone masonry construction (PCA, Citation2002). Results in Figures – show that all tailings matrices pass this lower limit strength indicating their viability for certain structural applications.
The proximity of the theoretical models with the experimental results of the mine tailings matrices, with no pozzolanic materials, is quite encouraging. It is suggested that more tests, with more tailings matrices’ mixtures, should be conducted to measure experimentally the porosity of mine tailings matrices to further evaluate these theoretical models. Also, it seems that there is a need for a new equation that describes the porosity-strength relation for tailings matrices, taking into account pozzolanic additions. This proposed equation should take into account the effect heavy metals content has on the strength properties of these matrices.
3.3. Toxicity characteristics leaching procedure (TCLP)
TCLP tests were carried out for the crushed hardened matrices at 1, 7 and 28 days in order to determine the amounts of heavy metals that can be extracted. Specimens of tailings matrices were crushed to powder consistency before extraction with acetic acid for 18 h at a 20:1 liquid to solid ratio with an initial pH of 5. Then, they were agitated in a rotary extractor for 18 h at 30 rpm and 22ºC. After 18 h of agitation, the specimens were filtered through a 0.6–0.8 µm glass fiber filter. Results in Table show high retention of heavy metals in the hardened cement-pozzolan phases. The heavy metals with a high percentage of retention are Cr, Ni, Cu, Zn and Pb. Values shown are the average of three determinations.
Table 3. TCLP test results for Mont Wright and Musselwhite matrices
The US EPA specifies a regulatory level for Zn, Cr and Pb of 5 mg/L in the TCLP extract. For Cu, the limit specified by EPA is 1 mg/L and for Fe 0.3 mg/L (US Environmental Protection Agency [USEPA], Citation2011). For the other metals, it specifies a value of 100 times the drinking water standards (LaGrega et al., Citation1994). The 100 factor is to account for dilution into the environment.
Through the addition of fly ash-Calsifrit or slag-Calsifrit, the leachates did not contain heavy metals including Cr and Ni. This might be due to the adsorption mechanism of fly ash and slag. Similar results for fly ash were previously reported by Singhal et al. (Citation2008) and Zhang et al. (Citation1999).
All tested mixtures had lower concentrations than the EPA toxicity threshold levels with the exception of Fe. It is thought that the binding techniques investigated function on a lower Fe tailings concentration. The initial high Fe concentrations need to be further investigated using higher binder concentrations. The retention of heavy metal molecules in the hydrated Portland cement matrix appears to be a result of more than one process. It is thought that these processes might include ionic adsorption to the hydrated C-S-H in the hydrated cement paste, ionic incorporation into the crystalline network of some compounds of the hydrated cement such as sulfates in the ettringite hydrate and physical retention in the porous structure (Cheilas et al., Citation2007).
TCLP results are encouraging for the short term considering medium to low exposure mediums. However, for high leaching mediums, more tests need to be conducted for longer periods of time to better assess the full potential of the mine tailings matrices and their environmental effect. A further assessment should include a functionality test to determine the best function these matrices are capable of fulfilling.
4. Conclusion
Porosity results showed that Musselwhite matrices have higher porosity values than Mont Wright matrices. Using Calsifrit, in addition to slag and fly ash as partial cement replacements, reduced, in general, the porosity of the tailings matrices. Increasing the percentage of Calsifrit in the matrices generally led to a decrease in the porosity of the Musselwhite tailings matrices. As for Mont Wright tailings matrices, the optimum Calsifrit percentage with the least porosity is 10% of the total weight of binder used.
It can be seen from the results above that these tailings matrices are suitable as construction materials for certain structural applications. The theoretical models of Chen et al. (Citation2013), Ryshkevitch (Citation1953), Schiller (Citation1971), Balshin (Citation1949), and Hasselman (Citation1969) generally overpredict the strength of these matrices. This is due, to a large extent, to the presence of pozzolanic additives and contaminants since overprediction increases with their presence. Also, the theoretical models did not take into account the curing period of the specimens. In the absence of a credible theoretical model that predicts porosity-based strength for tailings-cement-pozzolanic matrices with contaminants, the theoretical models detailed herein can be used with caution. Initial leaching tests, using the TCLP test, showed that these matrices have a high retention capacity for these heavy metals: Cr, Ni, Cu, Zn and Pb. However, long term leaching tests need to be conducted to establish their suitability for the long term.
In the future, it is advised to incorporate Calsifrit with other mine tailings in more strength tests to further establish its viability. This can lead in the end to the formulation of a theoretical model that predicts strength based on porosity for tailings-cement-Calsifrit-pozzolanic additives. Experimental results detailed herein can be used as a stepping stone in the development of this proposed theoretical model.
Acknowledgements
The authors would like to acknowledge with gratitude the support that Dr. Michelle Nokken and Dr. Catherine Mulligan of Concordia University (Montreal, Canada) have provided for this study.
Additional information
Funding
Notes on contributors
Ali A. Mahmood
Dr Ali A. Mahmood obtained his PhD from Concordia University in Montreal, Canada. He has more than 12 years of experience in research, teaching and construction in the field of civil engineering. His research interests include: sustainable engineering materials, discrete element and numerical methods and soil stabilization and reinforcement. He has been a member and chair in several committees in international journals and conferences.
Maria Elektorowicz
Prof. Maria Elektorowicz obtained her M.Eng. and Ph.D. degrees from Warsaw Technical University, Poland. Her research interests are bio-physico-chemical interaction phenomena in soil/groundwater/contaminant matrix, contaminated site remediation, as well as contaminated sediments and biosolids management. She joined Concordia University, in Montreal, in 1993. Since then she has been active in teaching Environmental Engineering and related subjects. Her research portfolio is rife with tens of papers and several patents.
References
- Andrew, R. M. (2018). Global CO2 emissions from cement production. Earth System Science Data, 10(1), 195–14. https://doi.org/10.5194/essd-10-195-2018
- ASTM C 109/C 109 M. (1999, January 4). Standard test method for compressive strength of hydraulic cement mortars (using 2-in. or 50 mm cube specimens). Annual Book of ASTM Standards, American Society for Testing and Materials.
- ASTM C 989. (2006, February 4). Standard specification for ground granulated blast-furnace slag for use in concrete and mortars. Annual Book of ASTM Standards, American Society for Testing and Materials Philadelphia Pennsylvania.
- Balshin, M. Y. (1949). Relation of mechanical properties of powder metals and their porosity and the ultimate properties of porous-metal ceramic materials. Dokl Akad SSSR, 67(5), 831–834.
- Benzaazoua, M., Belem, T., & Bussiere, B. (2002). Chemical factors that influence the performance of mine sulphidic paste backfill. Cement and Concrete Research, 32(7), 1133–1144. https://doi.org/10.1016/S0008-8846(02)00752-4
- Cheilas, A., Katsioti, M., Georgiades, A., Malliou, O., Teas, C., & Haniotakis, E. (2007). Impact of hardening conditions on to stabilized/solidified products of cement–sewage sludge–jarosite/alunite. Cement and Concrete Composites, 29(4), 263–269. https://doi.org/10.1016/j.cemconcomp.2006.12.005
- Chen, X., Wu, S., & Zhou, J. (2013). Influence of porosity on compressive and tensile strength of cement mortar. Construction and Building Materials, 40(2013), 869–874. https://doi.org/10.1016/j.conbuildmat.2012.11.072
- Conner, J. (1990). Chemical fixation and solidification of hazardous wastes. Van Nostrand Reinhold.
- Das, B. M. (2000). Fundamentals of geotechnical engineering. Brooks/Cole Publishing Company California.
- Davis, M., & Cumbers, J. (2019). Expanding tailings stewardship to include towards sustainable mining verification. Proceedings of Tailings and Mine Waste 2019, Vancouver, Canada: Published by the University of British Columbia.
- Fernández Olmo, I., Somarriba, P., & Irabien, A. (2003). Mechanical and environmental properties of cement/foundry waste systems. In Proceedings of WASCON, International Conference on the Environmental and Technical Implication of Construction with Alternative Materials. San Sebastian, Spain.
- Fourie, A. B., Blight, G. E., & Papageorgiou, G. (2001). Static liquefaction as a possible explanation for the Merriespruit tailings dam failure. Canadian Geotechnical Journal, 38(4), 707–719. https://doi.org/10.1139/t00-112
- Fourie, A. B., & Papageorgiou, G. (2001). Defining an appropriate steady state line for Merriespruit gold tailings. Canadian Geotechnical Journal, 38(4), 695–706. https://doi.org/10.1139/t00-111
- Garand, P., Vezina, S., & Bocking, K. (2000). Effects of flocculent on deposition of tailings sludge for upstream raise of impoundment dykes. In Proceedings of the 53rd Canadian Geotechnical Conference, Canadian Geotechnical Society (pp. 633–640). Montreal, Quebec.
- Gervais, C., & Ouki, S. K. (2002). Performance study of cementitious systems containing zeolite and silica fume: Effects of 4 metal nitrates on the setting time, strength and leaching characteristics. Journal of Hazardous Materials, 93(2), 187–200. https://doi.org/10.1016/S0304-3894(02)00005-5
- Han, F., Luo, A., Liu, J., & Zhang, Z. (2020). Properties of high-volume iron tailing powder concrete under different curing conditions. Construction and Building Materials, 241, 118108. https://doi.org/10.1016/j.conbuildmat.2020.118108
- Hasselman, D. P. H. (1969). Griffith flaws and the effect of porosity on tensile strength of brittle ceramics. Journal of the American Ceramic Society, 52(8), 457. https://doi.org/10.1111/j.1151-2916.1969.tb11982.x
- Helinski, M., Fahey, M., & Fourie, A. (2010). Coupled two-dimensional finite element modeling of mine backfilling with cemented tailings. Canadian Geotechnical Journal, 47(11), 1187–1200. https://doi.org/10.1139/T10-020
- Lafarge North America. (2007). 12950 worldgate dr ste. 500 Herndon Virginia 20170.
- LaGrega, M. D., Buckingham, P. L., & Evans, J. C. (1994). Hazardous waste management. McGraw-Hill, Inc.
- Lake, D. (2019). Stabilization of copper porphyry tailings with tailings-glass geopolymer solidification. Proceedings of Tailings and Mine Waste 2019, Vancouver, BC. Published by the University of British Columbia.
- Lea, F. M. (1971). The chemistry of cement and concrete. Chemical Publishing Company, Inc.
- Lottermoser, B. G. (2019). Valorization of phosphate mine wastes: the challenge of additional resource recovery from phosphate rock. Proceedings of Tailings and Mine Waste 2019, Vancouver, BC. Published by the University of British Columbia.
- Mahmood, A. A. (2012). Experimental and computational assessment of tailings binder matrices for construction purposes in cold regions. [Ph. D. thesis] Concordia University Department of Building Civil and Environmental Engineering.
- Mahmood, A. A., & Elektorowicz, M. (2018a). Experimental and computational assessment of the strength properties of Mont Wright tailings matrices for use as road materials. Journal of Physics: Conference Series, 1049, 012038. Retrieved from https://iopscience.iop.org/article/10.1088/1742-6596/1049/1/012038
- Mahmood, A. A., & Elektorowicz, M. (2018b). Freezing/thawing weathering resistance of solidified mine tailings matrices. Journal of Physics: Conference Series, 1049, 012103. Retrieved from https://iopscience.iop.org/article/10.1088/1742-6596/1049/1/012103
- Mahmood, A. A., & Elektorowicz, M. (2018c). The computer program Tailings-DEM™ modeling the strength properties of Musselwhite tailings matrices. Journal of Physics: Conference Series, 1049, 012104. Retrieved from https://iopscience.iop.org/article/10.1088/1742-6596/1049/1/012104
- Manjarrez, L., & Zhang, L. (2018). Utilization of copper mine tailings as road base construction Material through Geopolymerization. ASCE Journal of Materials in Civil Engineering, 30(9), 04018201. https://doi.org/10.1061/(ASCE)mt.1943-5533.0002397
- Massazza, F. (1998). Pozzolana and pozzolanic cements. In Leas’s chemistry of cement and concrete (4th ed.). (P.C. Hewlett edited by). Butterworth-Heinemann.
- Neville, A. M. (1995). Properties of concrete (4th ed.). Longman Scientific and Technical.
- NovaFrit International. (2006). 1200 garnier street ville ste-Catherine, Quebec J0L 1E0.
- Obenaus-Emler, R., Falah, M., & Illikainen, M. (2020). Assessment of mine tailings as precursors for alkali-activated materials for on-site applications. Construction and Building Materials, 246, 118470. https://doi.org/10.1016/j.conbuildmat.2020.118470
- PCA. (2002). The Portland cement association, masonry information, mortar cement product datasheet.
- Polettini, A., Pomi, R., & Sirini, P. (2002). Fractional factorial design to investigate the influence of heavy metals and anions on acid neutralization behavior of cement-based products. Environmental Science and Technology, 36(7), 1584–1591. https://doi.org/10.1021/es010002z
- Polettini, A., Pomi, R., Sirini, P., & Testa, F. (2001). Properties of portland cement stabilized mswi fly ashes. Journal of Hazardous Materials, 88(1), 123–138.
- Roy, S., Adhikari, G. R., & Gupta, R. N. (2007). Use of gold mill tailings in making bricks: A feasibility study. Waste Management and Research, 25(5), 475–482. https://doi.org/10.1177/0734242X07076944
- Ryshkevitch, R. (1953). Compression strength of porous sintered alumina and zirconia. Journal of the American Ceramic Society. American Ceramic Society, 36(2), 65–68. https://doi.org/10.1111/j.1151-2916.1953.tb12837.x
- Sabat, V., Shaikh, M., Kanap, M., Chaudhari, M., Suryawanshi, S., & Knadgouda, K. (2015). Use of iron ore tailings as a construction material. International Journal of Conceptions on Mechanical and Civil Engineering, 3(2), 1–6. Retrieved from wairco.org/IJCMCE/August2015Paper2.pdf
- Schiller, K. K. (1971). Strength of porous materials. Cement and Concrete Research, 1(4), 419–422. https://doi.org/10.1016/0008-8846(71)90035-4
- Singhal, A., Tewari, V. K., & Prakash, S. (2008). Utilization of treated spent liquor sludge with fly ash in cement and concrete. Building and Environment, 43(6), 991–998. https://doi.org/10.1016/j.buildenv.2007.02.002
- Stegemann, J. A. (2001). Neural network analysis of the effects of contaminants on properties of cement pastes. [PhD Thesis]. Imperial College of Science, Technology and Medicine.
- Stegemann, J. A., Butcher, E. J., Irabien, A., Johnston, P., de Miguel, R., Ouki, S. K., Polettini, A., & Sassaroli, G., Eds. (2001). Neural network analysis for prediction of interactions in cement/waste systems. Final Report. (Contract No. BRPR-CT97-0570). Commission of the European Community.
- Stegemann, J. A., & Côté, P. (1991). Investigation of test methods for solidified waste evaluation - a cooperative program. (Environment Canada Report EPA 3/HA/8).
- Theriault, J. A., Frostiak, J., & Welch, D. (2003). Surface disposal of paste tailings at the Bulyanhulu gold mine, Tanzania. In Proceedings of Sudbury 2003 Mining and the Environment, Sudbury, Ontario.
- Ugama, T. I., Ejeh, S. P., & Amartey, D. Y. (2014). Effect of tailings on the properties of concrete. IISTE, 6(10), 7–13. Retrieved from https://www.iiste.org/Journals/index.php/CER/article/view/16375
- USEPA. (2011). US environmental protection agency, website info. http://www.epa.gov. Ariel Rios Building, 1200 Pennsylvania Ave. NW, Washington, DC. 20004.
- USEPA Method 1311. (1992). Toxicity characteristic leaching procedure. US Environmental Protection Agency.
- Xue, G., Yilmaz, E., Song, W., & Cao, S. (2020). Fiber length effect on strength properties of polypropylene fiber reinforced cemented tailings backfill specimens with different sizes. Construction and Building Materials, 241, 118113. https://doi.org/10.1016/j.conbuildmat.2020.118113
- Zhang, L. (2012). Recycling and utilization of mine tailings as construction material through Geopolymerization. In Proceedings U.S. EPA Hardrock Mining Conference 2012: Advancing Solutions for a New Legacy. Denver, CO.
- Zhang, Y., Wang, Z., Xu, X., Chen, Y., & Qi, T. (1999). Recovery of heavy metals from electroplating sludge and stainless steel pickle waste liquid by ammonia leaching method. Journal of Environmental Sciences, 11 (3), 381–384. English edition, China. Retrieved from www.jesc.ac.cn/jesc_en/ch/reader/view_abstract.aspx?file_no=19990323&flag=1
- Zheng, M., Zheng, X., & Luo, Z. J. (1992). Fracture strength of brittle porous materials. International Journal of Fracture, 58(3), 51–55. https://doi.org/10.1007/BF00015623