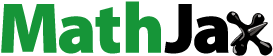
Abstract
This study evaluated the hyperaccumulation potential of four indigenous plant species: Chromolaena ordorata (CO), Paspalum viginatum (PV), Chrysopogon zizanioides (CZ) and Cynodon dactylon (CD) using soil polluted by the illegal gold mining activities. Nine treatments were used in this study: Control, CO, CZ, PV, CD, CO+PV, CO+CD, CZ+PV and CZ+CD samples. The soils’ physico-chemical properties and the concentration of some selected heavy metals and metalloid (As, Cd, Cu, Ni and Pb) were determined. Plant growth and dynamics of pH, electrical conductivity and heavy metals and metalloid in the gold ore mined soil were monitored for nine weeks. Accumulation, bioaccumulation and translocation potentials were determined. The mined soil was loamy textured and acidic with pH ranging between 5.71 and 6.24. Soil organic carbon, total nitrogen and phosphorous contents were relatively low (0.21 %, 0.09 % and 4.79 mg/kg soil, respectively). Concentrations of Pb, Cu, Ni, As and Cd (40.22, 30.54, 23.58, 6.18 and 0.27 mg/kg, respectively) in the area were below the WHO permissible limits. CO+PV combination resulted in higher reductions in As, Cd, Cu, Ni and Pb (40.1, 53.1, 36.8, 29.3 and 41.2%, respectively) from the mined soil. All the investigated plant species recorded BR > 1 for Cd and BR < 1 for As, Cu, Ni and Pb. Therefore, all studied plant species are good phytoextractors for Cd.
PUBLIC INTEREST STATEMENT
The growth of artisan gold mining in Ghana is negatively affecting the mined soil and plant growth in areas where these activities take place. Various mechanisms are being used to remediate these mined soils. This study looked at using selected plant species in phytoremediating the mined soils. The study has shown that introduction of specific vegetation in a heavy metals/metalloid-polluted area can help prevent erosion or contaminant leaching. In addition, it could be expected that the vegetation will help with the improvement of the soil’s biological properties by increasing the soil’s health and yield. The process is also environmental friendly while the cost of the phytoremediation exercise is much lower than those of traditional processes that can serve same purpose.
1. Introduction
Exploitations of natural resources have played diverse roles in enhancing the economies of both developing and developed countries. In as much as they end up improving the economies of these countries, their negative impact on the environment cannot be underemphasized. The negative impact from the mining activities on the environment is felt in the surrounding air, land, and water resources (Rangel et al., Citation2017; Vodouhe & Khasa, Citation2015). In Ghana, mining activities are mainly focused on the exploitation of gold due to large gold deposits existing across the country. Gold processing is done by government-regulated large-scale mining companies and several unregulated artisanal mining companies in the country. Discharge from the large-scale mining companies is adequately treated before been released into the environment and therefore less dangerous to living organisms. However, artisanal mining companies discharge their effluents directly into the environment poising danger to plants and animals (Karikari et al., Citation2020; I. Mensah et al., Citation2020). These effluents are associated with various contaminants ranging from heavy metals, hydrocarbons and toxic gases (Kortei et al., Citation2020). Extraction techniques employed in the processing of the gold deposits discharges varying levels of contaminants into the environment. Most of these contaminants are inorganic and remain indefinitely in the environment because of their non-degradable nature. These inorganic contaminants are bioaccumulative when taken up by living things and could result in diverse medical complications in humans (Khan, Citation2005). These bioaccumulative contaminants are mainly heavy metals/metalloids such as arsenic, copper, lead, zinc, iron, lead, cadmium, cyanide, and nickel (Cunningham & Berti, Citation1993).
Anthropogenic activities such as mining, fertilizer application and pesticide introduce additional amounts of these heavy metals into the top soil, flowing and underground water, and the surrounding air (Ali et al., Citation2013). According to Agrawal and Sharma (Citation2006), elevated concentrations of heavy metals in soils show possible toxicification that do affect the general plant development. In addition, the metabolic rate of plant life, and biological buildup of these lethal metals inside plants pose risk to anthropoid and animal wellbeing (Embrandiri et al., Citation2016). Increasing awareness of the risks caused by environmental pollution has led to several studies being conducted to mitigate their effect on anthropoid and animal (Hakami, Citation2015; Henry et al., Citation2013; Paull et al., Citation2019; Singh et al., Citation2020). One of these studies focuses on finding suitable methods of reducing heavy metal concentrations in the soil. This in turn will reduce food contamination through intake of the heavy metals from the soil (Cunningham & Berti, Citation1993). Recently, Almomani et al. (Citation2020) reported the successful removal of heavy metals from wastewater using novel magnetic nanoparticles. Their work showed that adsorption of heavy metals on magnetic nanoparticles is fast, effective and spontaneous. Over the years, numerous physico-chemical and biological remediation approaches like thermal handling, stabilization, precipitation and biological remediation have also been employed in the elimination of pollutants from soils (Kumarathilaka et al., Citation2020; Peng et al., Citation2018; Yao et al., Citation2012). Physico-chemical approaches for decontamination are suitable for comparatively smaller fields since they are too costly to be employed over large areas such as the ones contaminated by industrial substances, oil products and mining sites (Chekol et al., Citation2004).
Traditional environmental decontamination methods like pneumatic fracturing, soil flushing, solidification, vitrification, electrophoresis, chemical reduction, soil washing and excavation have been used over the years (Ashraf et al., Citation2019; Ernst, Citation1996; Saxena et al., Citation2020). However, these traditional methods are limited in their application and even in some situations result in the formation of secondary pollutants (Wuana & Okieimen, Citation2011). More often, these traditional physical and chemical remediation methods of heavy metal-contaminated soils are costly, time consuming and environmentally unsustainable (Saxena et al., Citation2020). Hence the need to ascertain for some progressive, eco-friendly and cost-effective technology.
An emerging technology that uses various plants such as rhizofiltration, phytostabilization, phytoextraction, phytovolatilization and phytodegradation to immobilize contaminants in the environment is phytoremediation (Chatterjee et al., Citation2013; Lee, Citation2013; Liu et al., Citation2018). Unfortunately, some of the plant species extensively studied and adopted for phytoremediation are not easily available in most communities, hence the need to investigate alternative plant species for phytoremediation at the study site in question. Chromolaena odorata known locally in Ghana as “Acheampong weed” belongs to the Asteraceae family. It is a rapidly growing perennial herb that forms dense tangled bushes. C. odorata grows on every form of well-drained soils as well as soils having comparatively low fertility. A study by Velasco-Alinsug et al. (Citation2005) demonstrated that, C. odorata accumulated high amounts of Hg in its vegetative tissues, without exhibiting any toxicity symptoms. Hamzah et al. (Citation2012) also described C. odorata as a good potential for removal of Hg and Pb from artisanal gold mining sites, because of its ability to accumulate high concentrations of these metals without experiencing toxicity. Paspalum vaginatum is another plant species found locally in Ghana. Elsewhere, it is widely used for rehabilitation of salt-affected lands, restoration of coastal wetlands, and forage for livestock (Riefner & Columbus, Citation2008). There are evidence that, P. vaginatum can also be used as bioremediation agent in contaminated soils (Duncan & Carrow, Citation2000). Also, Alrawiq and Idris (Citation2015) have further demonstrated that P. vaginatum has the ability to survive in soils with Hg concentrations up to 2 mg/L.
Chrysopogon zizanioides is another indigenous plant species that is commonly found in Ghana. C. zizanioides is a densely tufted perennial grass which belongs to the Poaceae family. This species can endure harsh environmental conditions and it is tolerant to elevated concentrations of heavy metals/metalloid such as As, Cd, Cu, Cr, Pb, Hg, Ni, Se and Zn in the soil (Danh et al., Citation2009; Das & Maiti, Citation2009). C. zizanioides has been found as an agent of phytoremediation and has been shown to enhance the degradation of heavy (Kumar & Maiti, Citation2015). Results from (Nirola et al., Citation2016b) showed that, C. zizanioides is an effective accumulator of metals like Cr, Pb, Ni, and Zn. Short and long-term phytoaccumulation experiments for several heavy metals (Cu, Cr, Pb and Zn) performed by Antiochia et al. (Citation2007) revealed that the accumulation of heavy metals are mostly in the roots of C. zizanioides than in the shoots. However, few studies have been reported about the tolerance capacity of C. zizanioides grasses growing on low to moderately Cr contaminated waste and its metal bioaccumulation (Gholipour et al., Citation2020; Wasino et al., Citation2019). Cynodon dactylon commonly known as Bermuda grass is also abundantly available in Ghana. C. dactylon is a prostrate, creeping, stonoiferous perennial herb, which belongs to the Poaceae family, which can thrive on a wide range of soil types and conditions. Sainger et al. (Citation2011) has shown that C. dactylon can be used for bioaccumulation with translocation factors of Zn > Fe > Cu > Ni > Cr. Studies by Wong (Citation1985) on C. dactylon detected higher concentrations of Cu, Cd and Zn in roots than aerial parts while contents of Cd, Mn and Zn were higher in the foliage of second harvest than the first. No studies so far have been reported for the phytoremediation of high concentration of Cr rich waste using these local aromatic grasses.
This work therefore assesses the hyper-accumulation ability of indigenous plant species: C. odorata, C. zizanioides, P. viginatum and C. dactylon at the study site. Soil samples obtained from an artisanal gold mining site at Bontesso in the Amansie West District, in the Ashanti Region of Ghana were used for this study. The location is one of the fastest-growing sites in Ghana for artisanal gold mining. Although concentrations of heavy metals such as As, Cd, Cu, Ni and Pb in soils at the site are currently below the WHO guidelines, agricultural produce from this site however have been reported to contain high levels of these heavy metals (Kwaansa-Ansah et al., Citation2019). With the current rate of increase in artisanal gold mining in the area, it is estimated that the soil contaminants will rise above WHO guidelines within a short time frame (Gyamfi et al., Citation2019). Hence the need to put in place mechanisms to clean up the area before the situation gets worse. It is Hypothesized that Indigenous hyperaccumulators such as Chromolaena ordorata, Chrysopogon zizanioides, Paspalum viginantum and Cynodon dactylon grown on compost-amended mined soils has no significant influence on the removal of heavy metals and metalloid degradation.
2. Materials and methods
2.1. Location and geology of study area
The study area is situated in the Wet Evergreen Forest Zone with dominant secondary forest and thick brush cover which is drained by the Bonte river. The Bonte river is bounded on both sides by steep hills that are about 500 m above mean sea level although the overall topography is considered to be low (Smith et al., Citation2016). The valleys created, as a result of the steep hills, have been extensively mined for placer deposits and now consist primarily of placer tailings. The soil in the area consists primarily of the Bekwai and Nzema Oda series and are generally deep to very deep, humus-laden, acidic with clay-loam to clay textures and well drained but occasionally gravelly and concretionary (Obiri-Danso et al., Citation2005).
The area has undergone extensive degradation in recent years. The main land uses of the area used to be subsistence and cash crop farming. Kwaansa-Ansah et al. (Citation2019) quite recently reported that artisanal gold mining is now the major land use in the study area. This is due to the surge in artisan, mostly illegal, gold mining operations currently been experienced in Ghana. The surge in artisan operations can be attributed to the growing migration of Chinese citizens to Ghana mainly to engage in the sector (Hilson et al., Citation2014).
The geology of Ghana is dominated by Birimian supergroup sequences overlain by clastic Tarkwaian Group (Davis et al., Citation1994) in synclinal troughs as shown in Figure ). The Birimian supergroup consists of narrow volcanic belts that are separated by wide anticlinal basins of marine clastic sediments. The rocks are mainly affected by greenschist metamorphic grade. Thin laterally extensive cherts and fine-grained manganiferous and graphitic sediments occupy transitional zones, while the belt margins contain fault zones that favour gold deposition. Conglomerates host palaeoplacer gold deposits in the Tarkwaian (Abouchami et al., Citation1990; Chudasama et al., Citation2016).
The study area contains gold-bearing quartz veins that are hosted by tightly folded Birimian sedimentary rocks that are intruded by dykes and granitoids (Figure )). In the southern portions, the intrusions are intensely brecciated and mineralised, while the weathering profiles strongly influence the topography (Chudasama et al., Citation2016). Weathering horizons consist of laterite, saprolite and oxidised bedrock, that weather away at higher elevations, while lower elevations are covered by alluvium or residual tailings from previous alluvial operations (Chudasama et al., Citation2016).
2.2. Samples preparation and ph determination of soil from study site
One hectare of land, divided into 25 subplots (20 m × 20 m) forming a sampling grid was demarcated. 9 of these subplots were systematically chosen with quadrants (1 m × 1 m) placed on each for soil sampling. The subplots were selected carefully such that each subplot contained the illegal mining activities. Three mined soil samples (which refer to the soil left behind after illegal mining activities) were randomly selected from each subplot and then mixed to provide a composite sample. In total, 90 kg of mined soil was obtained from the study site at Bontesso in in the Amansie West District, Ashanti Region of Ghana. The mined soil sample was homogenized and air dried at room temperature. The air dried sample was sieved using a 2 mm sieve mesh. Compost was also obtained from a local market. pH of the aqueous extract of the homogenized contaminated soil and compost were determined using a 1:1 (soil: water) ratio as reported by Black (Citation1965).
Four (4) selected indigenous plant samples growing on uncontaminated soil at the Botanical Gardens of Kwame Nkrumah University of Science and Technology, Kumasi (KNUST) were identified for the study. These indigenous plant species were C. odorata, C. zizanioides, P. vaginatum and C. dactylon The indigenous plant species were separated into roots and shoots and washed to remove any traces of soil particles. The cleaned species were air-dried for a week and oven-dried for 48 hours at 50°C to a constant weight.
Nine (9) treatments were used as phytoremediation agents in the study. Namely C. odorata, C. zizanioides, P. vaginatum and C. dactylon and their combinations in addition to the control (no plant species) as shown in Table .
Table 1. Treatments used in the study
2.3. Determination of soil organic carbon
Soil organic carbon was determined using the modified Walkley-Black wet oxidation procedure as described by Nelson and Sommers (Citation1996). EquationEquation (1)(1)
(1) was used to calculate the organic carbon content of the soil.
where M is the molarity of ferrous sulphate, V1 is the volume in mL of ferrous sulphate solution required for blank, V2 is the volume in mL of ferrous sulphate solution required for sample, w is the weight of moisture, and mcf = moisture correcting factor ((100 + % moisture)/100). Using the equivalent weight of carbon to be 3 and 1.3 for compensation factor for the incomplete oxidation of the organic carbon, a factor of 0.39 was obtained from the relation 3 × 0.001 × 100 % × 1.3. The procedure was repeated for all carbon determinations in the study.
2.4. Determination soil total nitrogen
Soil total nitrogen was determined by the Kjeldahl method as described in Sparks et al. (Citation1996). Soil total nitrogen was calculated as shown in EquationEquation (2)(2)
(2) :
where N is the concentration of HCl used in the titration, a is the volume in mL of HCl used in the titration, b is the volume in mL of HCl used in the blank titration, and w is the weight of air-dry soil sample. The 1.4 in the relation was obtained from 14 × 0.001 × 100 % where the 14 is the atomic weight of N. The procedure was repeated for all nitrogen determination in the study.
2.5. Determination of soil available phosphorus
Soil available phosphorus was extracted with Bray’s No.1 extracting solution (0.03 M NH4F and 0.025 M HCl) as described by Nelsen and Sommers (Citation1996). EquationEquation (3)(3)
(3) was used in computing the phosphorus content.
where a is the volume in mL of P in the sample extract, b is the volume in mL of P in the blank, and w is the sample weight in g. 15 ml of extracting solution was used with a 10 ml final sample solution. The procedure was repeated for all phosphorus determinations in the study.
2.6. Determination of some selected heavy metals/metalloid concentration of mined soil and plant species
Dried mined soil samples containing heavy metals and metalloid (As, Cd, Cu, Pb and Ni) were placed in crucibles and ashed at 500 °C for 2 hours. The dried samples were ground and sieved using a 2 mm sieve mesh. 2 g of the dried soil sample was weighed into a Kjeldahl digestion tube and mixed with perchloric, nitric and hydrochloric acids in the ratio 1:2:3, respectively. The mixture was then digested at a temperature of 450 °C until complete digestion was observed. The digested mixture was filtered and decanted into a 100 ml volumetric flask. The solution was finally topped to the 100 mL mark with distilled water. The unknown concentrations of the filtrates were then analysed using the Buck Scientific 210 VGP Atomic Absorption Spectrometer (AAS).
Determination of heavy metal and metalloid concentration in the selected dry plant samples (roots and shoots) were achieved by grinding these samples using a laboratory mill, placed into labelled crucibles and ashed for 3 hours at 500 °C. Aliquots of samples weighing 0.2 g were delivered into a beaker containing 3 and 1 mL of concentrated HCl and HNO3, respectively, and heated for 15 minutes on a hot plate at 100 °C to destroy any oxidizable and carbonaceous materials. The solutions were topped to the 30 mL mark with distilled water and filtered into 50 mL test tubes. Filtrates obtained were analysed for total As, Cd, Cu, Pb and Ni contents using a Buck Scientific 210 VGP AAS.
2.7. Determination of plants accumulation ratio and translocation factor
The ability of the plants to accumulate heavy metals from the soil was determined by the ratio of concentrations of heavy metals in the plant at harvest to the concentrations in the plant before transplanting (EquationEquation (4)(4)
(4) ) as reported by Mahar et al. (Citation2016).
Bioaccumulation factor (BF) was determined by taking the ratio of the concentration of heavy metal accumulated in plant tissue to the concentration of heavy metal present in the soil (EquationEquation (5)(5)
(5) ) as reported by Mahar et al. (Citation2016).
Translocation factor (TF), the ability of plants to transport heavy metals absorbed from the soil into the roots and shoots, was determined as the ratio of heavy metal concentration in plant shoot to that in plant root (EquationEquation (6)(6)
(6) ) as reported by Zacchini et al. (Citation2009).
2.8. Determination of contamination factor and degree of contamination
The soil’s contamination factor was computed using the relation proposed by Hakanson (Citation1980) as indicated in EquationEquation (7)(7)
(7) .
where Cf = contamination factor, Ci = measured concentration value for heavy metal i and Bi = background value of metal i.
The background values of As, Cd, Cu, Ni and Pb were obtained from the mean (arithmetic) values of background contents in Podzol, sandy soil (<20 % fraction and <0.02 mm) type on the worldwide scale (Kabata-Pendias & Mukherjee, Citation2007).
EquationEquation (8)(8)
(8) was used to compute for the degree of contamination (Cdeg) as reported by Hakanson (Citation1980);
where n is the number of elements (contaminants) in the soil.
2.9. Plant growth performance
Plants that are considered for phytoremediation must have high biomass production and also the ability to tolerate high levels of heavy metals in the soil. Growth performance of the selected plants used as phytoremediation agents were monitored for 9 weeks. The growth parameters measured were height, leaf area and chlorophyll content.
2.10. Statistical analysis of heavy metals analyses
Data obtained for heavy metal concentrations in soil and plants were subjected to analysis of variance (ANOVA) using R-Statistical Software, version 3.2.3 (2016). Mean differences between concentrations of heavy metals in soil and plants were compared using Tukey-B at 5 % significance level.
3. Results and discussion
3.1. Mine soil properties
The mined soil’s physical and chemical properties are presented in Table . The pH range of the study area was found to lie within the range 5.71–6.24. The mined soil being slightly acidic was not surprising, as A. K. Mensah et al. (Citation2015) has reported that low pH values is a feature for most mined soils in Ghana. Several other researchers have confirmed the acidic nature of mined soils in Ghana (Akabzaa et al., Citation2007; Amonoo-Neizer et al., Citation1996; Antwi-Agyei et al., Citation2009; Appiah & Thomas, Citation1982; Nsiah & Schaaf, Citation2019; Ofori-Sarpong & Amankwah, Citation2019).
Table 2. Physical and chemical properties of the mined soil used for the study
The average levels of organic carbon, total nitrogen and phosphorus in the mined soil were generally low as indicated in Table . This could be due to the mining activities, which have resulted in the removal of vegetation and consequently, loss of plant nutrients. Studies by other researchers do show similar trends in the disruption of ecosystem functioning and loss of litter layer other mining areas in Ghana (Appiah & Thomas, Citation1982; Tetteh et al., Citation2015). Variation in soil exchangeable bases contents were found to decrease in the order of Ca2+ > K+ > Mg2+ > Na+. This is an indication that both pH and ionic strength of the soil solution affect the cation exchange capacity especially in highly weathered soils. This finding is consistent with that presented by Appiah and Thomas (Citation1982).
The average levels of organic carbon, total nitrogen and phosphorus in the mined soil were generally low as indicated in Table . The average organic matter content in the mined soil is 0.3 % which implies that it cannot support plant growth (Landon, Citation2014). Landon (Citation2014) classified soil organic matter values below 4 %, between 4 and 10 %; and above 10 % as low, medium and high, respectively. He reported that nitrogen values below 0.2 %, between 0.2 % and 0.5 %; and above 0.5 % are considered low, medium and high, respectively. The low values could be attributed to the mining activities, which resulted in the removal of vegetation and consequently, loss of plant nutrients. Studies by other researchers do also show similar trends in other mining areas in Ghana (Appiah & Thomas, Citation1982; Tetteh et al., Citation2015). The low organic matter in the mined soil indicates the soil needs restoration measures when it is to be used for agricultural purposes (Mensah, Citation2015). Variation in soil exchangeable bases contents were found to decrease in the order of Ca2+ > K+ > Mg2+ > Na+. This is an indication that both pH and ionic strength of the soil solution affect the cation exchange capacity especially in highly weathered soils. This finding is consistent with that presented by Appiah and Thomas (Citation1982).
The results of this study reveal that, the heavy metals/metalloid contents of the study area was within the recommended limits as set up in WHO (Citation1996) guidelines. This is probably due to the nature of gold ore deposit that is being mined in the study area. Concentrations of trace elements in natural soil and contaminated soil from gold deposits sites show great variability and therefore it is imperative to use background values from worldwide scale for easy reproducibility and comparability of analytical data as reported by Kabata-Pendias and Mukherjee (Citation2007). Table presents the mean concentration of heavy metals/metalloid, the contamination factors in the mined soil at the study site and the respective background contents for the associated metals/metalloid. The mean (arithmetic) values of the background contents of As, Cd, Cu, Ni and Pb in Podzol, sandy soil (<20 % fraction and <0.02 mm) type on the worldwide scale are 4.4, 0.37, 13.0, 13.0 and 22.0 mg/kg, respectively (Kabata-Pendias & Mukherjee, Citation2007). The contamination factors (Cf) for all metals/metalloid considered in the study were greater than 1 except Cd indicating that mined soil is moderately contaminated by As, Cu, Ni and Pb and lowly contaminated by Cd as described by Hakanson (Citation1980).
Table 3. Mean concentration of heavy metals and metalloid in the soil at the study site
Although, the study area recorded heavy metals/metalloid concentrations, all values were below the permissible limit for heavy metals in soils according to WHO (Citation1996). The mean concentrations of the investigated heavy metals/metalloid were in the order of Pb (40.22 ± 4.80 mg/kg) > Cu (30.54 ± 3.54 mg/kg) > Ni (23.58 ± 1.54 mg/kg) > As (6.18 ± 0.67 mg/kg) > Ni (25.31 mg/kg) > Cd (0.27 ± 0.04 mg/kg). The low concentrations of the heavy metals/metalloid could be due to slow weathering of the waste rock which has been exposed as a result of the illegal mining activities. The concentrations of the heavy metals/metalloid in the mined soil in the study area were compared with the values reported from other illegal mining areas in Ghana (Armah et al., Citation2014). The low concentration of As was similar to values obtained in Ashanti and Western regions of Ghana by Akabzaa et al. (Citation2007) and (Hayford et al., Citation2009) respectively. Also, Bortey-Sam et al. (Citation2015) reported low concentrations of Cd (0.050 mg/kg), Cu (6.2 mg/kg), Ni (3.7 mg/kg) and Pb (7.2 mg/kg) for agricultural soils in 19 communities in Tarkwa, Western region of Ghana. The similar levels of heavy metals in the present study area and the study sites reported by Bortey-Sam et al. (Citation2015) were attributed to the similarity in the duration of the illegal mining activities and the type of ores mined in the two areas (Boateng et al., Citation2012). However, other studies in Ghana on heavy metals concentrations at illegal gold mining sites revealed high concentrations of these heavy metals/metalloids (Antwi-Agyei et al., Citation2009; Gyamfi et al., Citation2019). Shen et al. (Citation2019) attributed the high concentrations to abuse of pesticide and phosphate fertilizer on agriculture soil around the mining areas.
The degree of contamination (Cdeg) in the mined soil at the study site was 8.12. Loska and Wiechuła (Citation2003) reported the existence of four pollution levels of Cdeg < 5 for low pollution; 5 ≤ Cdeg < 10 medium pollution; 10 ≤ Cdeg < 20 high pollution; and with Cdeg ≥ 20 representing very high pollution). From the Cdeg of 8.12 obtained for this study, it was concluded that our study site was medially polluted. Though the heavy metals/metalloid were below the WHO permissible limits, these heavy metals/metalloid can be detrimental when the soil is used in the cultivation of edible plants. Also with time, the tailings from the illegal gold mining activities can lead to prolong accumulation of heavy metals/metalloid in the soil. With the current rate of increase in artisanal gold mining in the area, it is estimated that the soil contaminants will rise above WHO guidelines within a short time frame (Gyamfi et al., Citation2019; Hilson et al., Citation2014; Karikari et al., Citation2020). Therefore, these heavy metals might have potential of polluting the soils in the study area which will intend pose serious health problems in the future. Hence, the need to put in place mechanisms to clean up the area before the situation gets worse.
3.2. Analysis of plant biomass before transplanting
Table provides the average moisture content and percentage dry weight of the shoots and roots of the selected plant species before transplanting in the mined soil. The highest moisture content in the roots was obtained in C. odorata (55.08 ± 0.64%) followed by P. vaginatum (54.54 ± 1.2 %). C. zizanioides had the highest moisture contents in the shoot. C. odorata and C. zizanioides recorded the least root and shoot dry weights of 45.92 ± 0.55 and 29.18 ± 0.39 %, respectively. According to earlier researchers, plant species with high biomass production are ideal for phytoremediation (Cunningham & Ow, Citation1996; Kumar et al., Citation1995; Schnoor, Citation1997). The selected plant species satisfied the biomass requirement (Table ) and were among the 400 plant species that have been reported as hyperaccumulator species of heavy metals in the soil (Ghosh & Singh, Citation2005; Lasat, Citation1999; Salt & Krämer, Citation2000).
Table 4. Moisture content and percentage dry weight of plants before transplanting
3.3. Concentrations of heavy metals/metalloid in plants species before transplanting
The levels of heavy metals/metalloid in the plant species selected for the study before transplanting are presented in Table . All levels where below the permissible limits in plants according to WHO (Citation1996) guidelines in all the plants selected for the study except Cd. These observations could be attributed to the ability of the plant species to extract higher levels of Cd in soils where the species were taken from. The observations in the present study confirm that accumulation of metals by plants depends on the availability and solubility of metals in soils, their translocation potential and the type of plant species involved (Mahar et al., Citation2016; Zacchini et al., Citation2009).
Table 5. Concentrations of heavy metals/metalloid in plant species before transplanting
3.4. Biomass of plants at harvest
Table shows the results of the percentage dry weight and moisture content of plants at harvest. Higher moisture contents were observed in the shoots compared to the roots of all the plants species. P. vaginatum recorded the highest amount of moisture in the roots (63.59 %) while the highest in the shoots were observed in C. zizanioides (83.46 %). In both roots and shoots, C. dactylon recorded highest percentage dry weights (53.89 and 44.19 %, respectively) among the plants. The roots of the plants species however recorded higher dry weights than the shoots.
Table 6. Moisture content and percent dry weight of plants at harvest
3.5. Changes of pH and EC in soil after planting
Changes in pH and EC of the mined soil throughout the study were monitored at four different sampling periods (weeks 0, 3, 6 and 9) and the results presented in Figure . The mean pH of the soil throughout the study period ranged from 6.4 (slightly acidic) in the control treatment at week 9 to 7.2 (slightly basic) for the combined CO and PV plant species at week 6. Different dynamics were observed for each treatment. Generally, the control treatment recorded lower pH values from week 3 to week 9 than the other soil treatments. There were also general increases in soil pH in all treatments between the baseline and week 3. However, after week 3, a reduction in soil pH was observed under the control, CZ, CZ + PV and CZ + CD treatments up till week 9.
Figure 2. (a) Changes of soil pH with time and (b) Dynamics of soil electrical conductivity with time
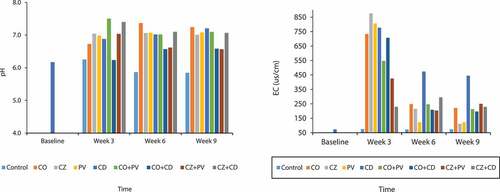
The pH recorded in CO plant species increased from 6.7 to 7.4 between weeks 3 and 6 and declined to 7.2 at week 9. The observed trend in CO + PV plant species was different from the other treatments as it increased to a pH of 7.5 in week 3, decreased to 7.0 in week 6 and increased again to 7.1 in week 9. This can be attributed to the behaviour of certain plant species which when cultivated in basic soils makes the heavy metals chemically unavailable but are sparingly available for plant use (Epstein, Citation1972).
Electrical conductivity (EC) of the soil through the study period ranged from 72 µs/cm in the control treatment at week 6 to 877 µs/cm in the CZ plant species at week 3 (Figure )). With the exception of CZ + CD plant species, EC recorded in all the treatments generally increased from the beginning of the study to the 3rd week then decrease thereafter. The increment could be as a result of salinity and irrigation of the soil which is an important indicator of the health of polluted soil. At week 3, PV plant species recorded the highest EC while the control recorded the least. However, at weeks 6 and 9, ECs were highest in the CD treatment (473 and 445 µs/cm, respectively). According to Harter (Citation1983) some plants are more susceptible to EC with each species having a EC threshold, beyond which the plant’s yield decreases.
Soil properties affect metal availability in different ways as reported by Harter (Citation1983). The increase in the pH and EC values observed relative to the baseline values could be due to the addition of compost to the soil. The compost used to amend the soil had relatively high amount of organic matter (48.6 %) which acts mainly as the source of exchangeable cations. These cations could displace H+ in the soil thereby altering the pH and the EC of the soil as noted in the study. This is in agreement with the studies by Noll (Citation2003).
3.6. Dynamics of heavy metals/metalloid in soil with time
Figure shows the dynamics of heavy metals/metalloid concentrations in mined soil across the study period. The results show general reductions in the levels of metals from their initial concentrations to the end of the study in all treatments. Among all the treatments, excluding the baseline, control samples at week 3 recorded the highest concentration of As (6.08 mg/kg) and CO + PV plant species at week 9 recorded the least (3.69 mg/kg). The levels of Cd ranged from 0.12 mg/kg in CO + PV plant species at week 9 to 0.25 mg/kg in the control at week 3. The control recorded the highest concentrations of Cd at all sampling periods. At week 3, CO plant species recorded the least level of Cd (0.21 mg/kg). However, the trend changed in weeks 6 and 9 with CO + PV plant species recording the least concentrations of 0.17 and 0.12 mg/kg, respectively. Cu concentrations ranged from 19.29 mg/kg in CO+PV plant species at week 9 to 30.06 mg/kg in the control at the week 3.
Figure 3. Dynamics of studied heavy metals/metalloid concentration in soil with time for (a) As, (b) Cd, (c) Cu, (d) Ni and (e) Pb
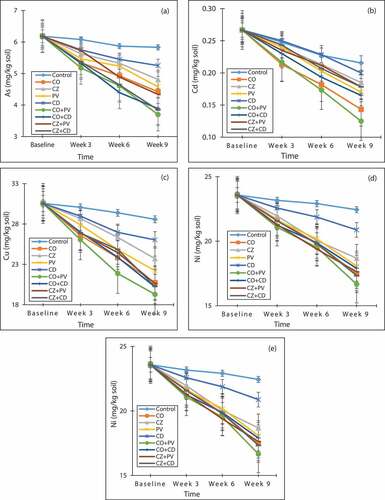
In all the sampling periods (weeks 3, 6 and 9), highest levels of Cu were observed in CO+PV combination. Similar to the other metals, a general decreased with time was observed in Ni concentrations in all treatments (Figure )). The control recorded highest concentrations of Ni at all sampling periods while CO+PV test sample recorded least concentrations ((21.08, 19.52 and 16.66 mg/kg soil) at all periods of sampling during weeks 3, 6 and 9, respectively. Pb concentrations ranged from 23.69 mg/kg in CO+PV sample at week 9 to 39.51 mg/kg in the control at week 3. The least Pb concentrations in the soil at weeks 3, 6 and 9 were 34.56, 30.73 and 23.69 mg/kg in CO+PV, CO and CO+PV plant species, respectively. The general reduction of metals observed is an indication that, plant species used for the study were effective in extracting metals from the soil. However, slight reductions were observed in the control treatments but these reductions were not statistically significant.
The levels of heavy metals in soil decreased with plant growth (i.e. as the plant species produce more biomass, more metals were extracted from the soil thereby reducing the levels of the metals in the soil). These observations affirm the importance of high biomass production to the success of phytoremediation (Cunningham & Ow, Citation1996; Kumar et al., Citation1995; Schnoor, Citation1997). Again, the significant reductions of metals in soils treated with the plant species than those of the control soil could also be attributed to the active contribution of plants in circumstances when heavy metals are available. This is done by the secretion of phytosidophores into the rhizosphere that chelate and solubilize metals that are soil bound (Kinnersley, Citation1993). Two of the mechanisms used by plants to enhance metal accumulation are acidification of the rhizosphere and exudation of carboxylates as reported by Zhao et al. (Citation2001). Yang et al. (Citation2005) reported that acidification of rhizosphere soil in numerous plant types accumulating metals such as Zn, Cu, Cd and Ni. Also, Kinnersley (Citation1993) validates this claim as his findings support our results. His studies reported that the amount of heavy metals that enter plants is determined by their mass flow, which is based on their concentration in the surrounding soil solution. This process he claims is driven by transpiration. Hence, the amount of heavy metal uptake by root interception is dependent on the amount of available metal in the soil volume occupied by the root which corroborates our results.
3.7. Percentage reduction and concentrations of heavy metals/metalloid in soil at harvest
Metal concentrations reduced in all the mined soils after phytoremediation, as presented in Table . Percentage reductions for all metals in all treatments were below 50 % except for Cd reduction in CO+PV samples which recorded a 53.1 % reduction. Least percentage reductions were recorded in the control samples for all As, Cd, Cu, Ni and Pb metals as 5.7, 19.1, 6.4, 4.8 and 5.4 %, respectively. Highest percentage reductions for all As, Cd, Cu, Ni and Pb metals were recorded in CO+PV samples (40.1, 53.1, 36.8, 29.3 and 41.2 %, respectively). Reductions for all metals in the sole plant treatments were highest in CO treated soils indicating the potential of the species as phytoremediator for these metals. Followed closely to the CO in metals reduction was PV which exhibited higher phytoremediating potential compared to the CZ and CD. The evidence of the potential of these two species as phytoremediators was further confirmed when combined. The combination CO+PV resulted in highest reductions of all metals at harvest. This shows that for efficient reclamation of a polluted area, there is the need to combine these two species on the land.
Table 7. Mean percentage reduction and concentrations of heavy metals in soil at harvest
3.8. Plant growth performance
Growth patterns were observed in all plant species investigated (Tables –1). There was a general increase in height and leaf area in the treatment with time (Tables and ). At all the sampling periods, CZ+CD samples recorded the highest plant height (36.24 ± 5.69, 45.01 ± 4.6 and 55.23 ± 4.18 cm at weeks 3, 6 and 9, respectively) while CO+CD samples recorded the least height at all sampling periods (13.86 ± 2.24, 18.66 ± 2.34 and 21.42 ± 1.95 cm at weeks 3, 6 and 9, respectively). CO+PV samples recorded the largest leaf area of 47.99 ± 9.32 cm2 among the treatments at week 3. However, at weeks 6 and 9, largest leaf areas of 68.51 ± 14.78 and 80.64 ± 9.23 cm2 respectively were observed in CO samples. PV sample recorded the largest leaf area at week 3 among the sole plant treatments but the trend changed in weeks 6 and 9 with CO sample recording the largest at both sampling periods. For the combinations, CO+PV recorded largest leaf area at all sampling periods. The general plant growth patterns observed for the plant species could be due to the pH conditions at the study site. According to Sheoran et al. (Citation2010), plant growth on mine soil is sustained by pH conditions between 6.0 and 7.5 and at pH below 5.5, reduced plant growth can occur.
Table 8. Mean height of plants species with time
Table 9. Mean leaf area of plant species with time
Table 10. Mean chlorophyll contents in plant species with time
Generally, chlorophyll contents observed in all treatments increased from week 3 to week 6 and then decreased in the 9th week with the exception of CO, which recorded a general decreased from weeks 3 to 9. CO recorded the highest chlorophyll content (10.92 µmol/m2) at week 3 while CZ sample recorded the least chlorophyll (1.26 µmol/m2). At weeks 6 and 9, highest chlorophyll contents were observed in CO+CD sample. CO sample among the sole plant treatments recorded highest chlorophyll contents at all sampling periods while the highest among the combinations were observed in CO+CD plant species at periods of sampling.
Plant height and leaf area increased with time until harvest while chlorophyll contents peaked at week 6 and reduced due to yellowing as plants reach maturity. These observations in Tables –1 could be as a result of the addition of compost which might have supplied nutrients for the plant. Soil amendments such as fertilizer, compost, and manure and sewage sludge have been reported (Hartley et al., Citation2009) to help enhance plant growth. The normal growth by the plant species could also be due the low levels of heavy metals in the soil, which were below the WHO permissible limits.
Table 11. Concentration of heavy metals/metalloid in plant at harvest
Table 12. Bioaccumulation factor for heavy metals/metalloid
3.9. Accumulation (extractive) potential of plants for heavy metals/metalloid
The mean concentrations of heavy metals in plants species and their extractive potentials (accumulation ratio) at harvest are presented in Table . Concentration of As in the roots were highest in CO (0.44 mg/kg), which was 69, 33 and 83 % higher than CZ, PV and CD, respectively. CO significantly recorded higher concentration of As (0.77 mg/kg) in the whole plant than CZ and CD (0.55 and 0.52 mg/kg, respectively). The highest concentrations of Cd in roots, shoots and whole plant were significantly (P < 0.05) recorded by CD (0.55, 0.75 and 1.29 mg/kg, respectively).
Mean ± SD in same column with different letters in superscripts are significantly different (P < 0.05).
Similar levels of Cd were recorded in the roots of CO, CZ and PV. Concentration of Cu in the roots was highest in CO (0.94 mg/kg) and least in CZ (0.24 mg/kg). Analyses on the shoots and whole plant of CO recorded the highest concentrations of Cu (0.92 and 1.86 mg/kg) while CZ recorded the least (0.27 and 0.51 mg/kg, respectively). CZ and CD significantly recorded highest concentrations of Ni in the roots, shoots and whole plants (as shown in Table ).
Lead (Pb) concentrations were significantly highest in CO in the roots (0.087 mg/kg), CO and PV (0.072 and 0.069 mg/kg) in the shoots and CO (0.16 mg/kg) in the whole plant. According to Lăcătuşu et al. (Citation2009), concentrations of metals in plants vary from species to species due to plant response under different environmental conditions as noted in Table . Concentrations of As and Cd in all the species were above the permissible limits of metals in the plant (WHO, Citation1996).
Highest accumulation ratios of As were observed in both roots and shoots of PV (37.6 and 34.4, respectively), with the highest in the whole plant recorded by CO (33.2). CO and PV showed the highest accumulating capabilities for Cd by recording highest accumulation ratios in both plant organs and whole plant as well (12.9 and 13.3 in the roots, 12.9 and 12 in shoot and 11.3 and 11.2 in the whole plant). This suggests that, CO and PV are good candidates for phytoremediating As (Terry & Banuelos, Citation1999). Generally, CZ and PV were seen as best plants for the accumulation of Cu, Ni and Pb as they recorded highest accumulation ratios for these metals in both plant organs and whole plant. Ability of CZ to accumulate heavy metals in the present study affirms the findings of Nirola et al. (Citation2016a) who also reported that CZ is an effective accumulator of metals like Cr, Pb, Ni, and Zn.
3.10. Bioaccumulation (hyperaccumulation) potential of plants for heavy metals/metalloid
Bioaccumulation factors (BF) of all plant species (in both parts and whole plant) for Cd were greater than 1.00 as shown in Table . CD recorded the highest factors in both the organs and the whole plant (2.05 in the root, 2.80 in the shoot and 4.85 in the whole plant). Since the BF > 1, it indicates that all the plant species used in the study are suitable for phytoextraction of Cd. This is also an indication of the species high selective affinity for the uptake of Cd, where all species recorded levels of Cd above the permissible limits of metals in plants (WHO, Citation1996). Although bioaccumulation factors for all plant species for As, Cu, Ni and Pb in both plant parts and whole plant observed were less than 1, these plant species are potential accumulators for these metals based on the accumulation factors.
3.11. Translocation factors for heavy metals/metalloid
Table shows the results of concentrations of metals in roots compared to concentrations in shoots (translocation ratio). The species generally showed selective translocations for metals. Transport of metals within plant organs is dependent on the type of metal involved. According to Kabata-Pendias (Citation2000), metals such as Ag, B, Li, Mo, and Se are very mobile and therefore easily transported from roots to above grounds parts; Cd, Mn, Ni, and Zn are moderately mobile; and Co, Cr, Cu, Fe, Hg and Pb are strongly bound in root cells. Plants with high translocation factor (TF > 1) are considered good phytotranslocators (Zacchini et al., Citation2009). CZ and CD recorded TFs greater than 1 for As indicating that, the species are good phytostabilizers for As. CO, CZ and CD were all observed to be good phytostabilizers for Cd (Table ). Only CZ showed good phytostabilizing potential for Cu and CZ and PV for Pb. Ni was strongly bound in the root cells as all plant species recorded TF values less than 1.
Table 13. Translocation factors for heavy metals concentrations in root compared to concentrations in shoot of plants
3.12. Correlation analysis
The results of Pearson correlation coefficient matrix for heavy metals/metalloid (As, Cd, Cu, Ni and Pb), EC and pH of the soil samples are presented in Table . The computed statistical results showed that As had strong significant positive correlations with Cd, Cu, Ni and Pb (r = 0.7114, 0.7959, 0.7208 and 0.7657). Cd had strong positive and significant relationships with Cu and Ni (r = 0.7589 and 0.7380) and very strong significant positive correlation with Pb (r = 0.8142). Cu on other hand had very strong significant positive correlations with Ni and Pb (r = 0.8710 and 0.8483). There was also a very strong positive and significant relationship between Ni and Pb (r = 0.8600). However, all metals had weak negative correlations with pH (r = −0.1377, −0.1061, −0.1651, −0.2093 and −0.1876 for As, Cd, Cu, Ni and Pb, respectively) and very weak negative insignificant correlations with EC (r = −0.0812, −0.0785, −0.0838, −0.0734 and −0.0932 for As, Cd, Cu, Ni and Pb, respectively). The correlations between pH and As as well as Cd were insignificant while with Cu, Ni and Pb were significant.
Table 14. Pearson correlation coefficient matrix for the heavy metals in soil samples
3.13. Implication of results
Heavy metals/metalloids pollution in the environment surrounding mining areas have been reported to be one of most serious environmental problems in Ghana (Amonoo-Neizer et al., Citation1996; Bempah et al., Citation2013; Ewusi et al., Citation2013; Kim et al., Citation2003). The heavy metals/metalloids are generated from waste rocks by mining activities which highly deteriorate the ecosystems around these mines. These pollutants easily disperse into the surrounding environment and are taken up by plants (Wongsasuluk et al., Citation2014).
Developing countries are faced with many challenges especially illegal mining in those countries endowed with natural mineral resources. Although most of them have regulations regarding artisan mining operations, enforcement have been a challenge. Ghana has been reported to be at the forefront in the fight against such illegal mining activities (Banchirigah, Citation2008; Hilson et al., Citation2014; Hilson & Potter, Citation2003). Impact on the environment in such illegal mining areas is expected to be very high. In Ghana, majority of the studies (Amonoo-Neizer et al., Citation1996; Bempah et al., Citation2013; Ewusi et al., Citation2013; Kim et al., Citation2003) reported on the impact of mining activities on heavy metal pollution have focused mainly in the Tarkwa and Obuasi communities which is historically noted for numerous large scale and small-scale mining activities. Impact of illegal mining activities in other areas such as Bonteso in the Amansie West District of the Ashanti Region, Ghana, has not been studied and hence there is limited or no reported data on the heavy metals concentrations and their effect on the environment in that District. The analysis of heavy metals/metalloid (As, Cu, Ni, Pb and Cd) concentrations in the present study site will serve as the basis for further studies in the understanding of the distribution of heavy metals/metalloids and their impact on vegetation in the area. The degree of contamination of heavy metals/metalloid on mined soil generated by illegal mining activities in the area has now been determined. The concentrations of the heavy metals/metalloid in the study area imply that the mined soil is moderately polluted by As, Cu, Ni and Pb except Cd. The results will help inform decision on how the soil in the surrounding environment can be amended for improved agricultural practices.
Furthermore, researchers (Broadley et al., Citation2007; Sreelal & Jayanthi, Citation2017) have shown that the mobility or accumulation of these heavy metals in soils is affected by the properties of the soil such as pH, cations exchange and others chemical elements. The results on the potential of indigenous plant species to remove heavy metals (As, Cu, Ni, Pb and Cd) from amended tailings of illegal gold mining activities have shown that C. odorata has a good potential for the removal of Cd. The findings from this study also imply that addition of compost to mined soil could enhance the phytoaccumulation potentials of the plant species used in the study. This can guide environmentalist on how to reclaim and remove heavy metals/metalloid from polluted soil in the study site.
The findings further indicated that based on the soil’s physico-chemical properties, combined use of C. odorata and P. vaginatum resulted in higher reductions for all metals from the soil. C. odorata was the preferred species for remediating mined soils high in As. The study also showed that P. vaginatum could be used to accumulate heavy metals from reclaimed mined soil than Cd than C. Odorata. C. dactylon is preferred to C. zizanoides in the removal of Cu from reclaimed mined soil. The bioaccumulation ratios which were less than one (BR < 1) for As, Cu, Ni and Pb in all the plant species studied implied that the species are not suitable for phytoextraction of these metals. All the plant species had BRs greater than 1 (BR >1) for Cd showing they are good phytoextractors for Cd.
Again, combinations of the plant species showed reduced concentrations of the heavy metals/metalloid when compared to the use of individual plant species. This implies that the use of combined plant species may be more promising for reducing heavy metals/metalloid concentrations in mined soil. C. zizanioides and P. vaginatum were seen as best plants for the accumulation of Cu, Ni and Pb as they recorded highest accumulation ratios for these metals in both plant organs and whole plant.
4. Conclusions
The results of the study showed that, mined soil in the study area was moderately acidic with pH ranging between 5.71 and 6.24. The mined soil had a loamy sand texture. Soil organic carbon content, total nitrogen and available phosphorus were low (0.21 %, 0.09 % and 4.79 mg/kg soil). Levels of Pb, Cu, Ni, As and Cd in the area were also low (40.22, 30.54, 23.58, 6.18 and 0.27 mg/kg, respectively). Contamination factors and degree of contamination showed that, the study area was moderately contaminated with the metals considered in the study and was also within WHO permissible limits. Addition of compost enhanced the phytoaccumulation potentials of the plant species used in the study. Combined use of C. odorata and P. vaginatum resulted in higher reductions for all As, Cd, Cu, Ni and Pb metals from the soil (40.1, 53.1, 36.8, 29.3 and 41.2 %, respectively). C. odorata was the most effective species among the selected species in reducing the concentrations of all As, Cd, Cu, Ni and Pb metals in the soil (28.4, 46.2, 32.1, 25.9 and 37.7 %, respectively).
Accumulation of As was higher in both roots and shoots of P. vaginatum with accumulation ratios values of 37.6 and 34.4, respectively. P. vaginatum accumulated more Cd in the root with ratio 13.3 and C. odorata more in the shoot and whole plant (ratios: 12.9 and 11.3). For Cu, accumulations were higher in C. dactylon in the roots (ratio; 3.2), C. zizanoides in the shoots (ratio; 1.6) and C. zizanoides and C. zizanioides in the whole plant (ratio; 1.4 each). C. zizanioides accumulated higher amount of Pb in both shoot and whole (ratios 28.2 and 21.8) and P. vaginatum accumulated higher in the root (17.9). Bioaccumulation ratios for As, Cu, Ni and Pb in all the plant species were less than 1 which shows that the species are unsuitable for phytoextraction of the metals. All the species had BRs greater than 1 for Cd, indicating that they are potential phytoextractors for Cd. However, it is recommended that all the plant species should be investigated in areas where the concentrations of heavy metals/metalloids are relatively high so as to ascertain the phytoextractive potential of these indigenous plant species.
Statistically, As had strong significant positive correlations with Cd, Cu, Ni and Pb (r = 0.7114, 0.7959, 0.7208, and 0.7657). Cd had strong positive and significant relationships with Cu and Ni (r = 0.7589 and 0.7380) and very strong significant positive correlation with Pb (r = 0.8142). Cu, on other hand, had very strong significant positive correlations with Ni and Pb (r = 0.8710 and 0.8483). There was also a very strong positive and significant relationship between Ni and Pb (r = 0.8600).
In this aspect, the study has shown that introduction of specific vegetation in a Cd polluted area can help to prevent erosion or contaminant leaching. In addition, it could be expected that the vegetation will help the improvement of soil biological properties by increasing the soil’s health and yield. The process is also environmental friendly while the cost of the phytoremediation exercise is much lower than that of traditional processes that can serve same purpose.
Additional information
Funding
Notes on contributors
Emmanuel Gikunoo
Philipa Opoku holds a BSc degree in Renewable Natural Resources and an MPhil degree in Environmental Resources Management both from Kwame Nkrumah University of Science and Technology (KNUST), Kumasi, Ghana. She works with the Ghana Forest Commission and has over five years working experience as a forester.
Dr. Emmanuel Gikunoo and Dr. Emmanuel Kwesi Arthur are lecturers at the Materials Engineering Department, KNUST, Ghana. Their areas of publication include surface engineering, mechanical metallurgy, advanced composite materials, ceramic processing, materials selection in mechanical design, waste utilisation, point-of-care devices, and environmental pollution and heavy metal removal from soils and water.
Dr. Gordon Foli is a lecturer with the Department of Geological Engineering, KNUST, Ghana. He has written over 32 peer reviewed publications on the topics: mining impacts and risk assessment, mineral deposit assessment, waste dumpsite and earth dam characterisation, phytoremediation and bioaccumulation of trace metals, mine water remediation, and geochemical classification of some paleoproterozoic rocks.
References
- Abouchami, W., Boher, M., Michard, A., & Albarede, F. (1990). A major 2.1 Ga event of mafic magmatism in West Africa: An early stage of crustal accretion. Journal of Geophysical Research: Solid Earth, 95(B11), 17605–28. https://doi.org/10.1029/JB095iB11p17605
- Agrawal, V., & Sharma, K. (2006). Phytotoxic effects of Cu, Zn, Cd and Pb on in vitro regeneration and concomitant protein changes in Holarrhena antidysenterica. Biologia Plantarum, 50(2), 307–310. https://doi.org/10.1007/s10535-006-0027-z
- Akabzaa, T., Banoeng-Yakubo, B., & Seyire, J. (2007). Impact of mining activities on water resources in the vicinity of the Obuasi mine. West African Journal of Applied Ecology, 11(1), 101-109. DOI: 10.4314/wajae.v11i1.45719
- Ali, H., Khan, E., & Sajad, M. A. (2013). Phytoremediation of heavy metals—concepts and applications. Chemosphere, 91(7), 869–881. https://doi.org/10.1016/j.chemosphere.2013.01.075
- Almomani, F., Bhosale, R., khraisheh, M., & Almomani, T. (2020). Heavy metal ions removal from industrial wastewater using magnetic nanoparticles (MNP). Applied Surface Science, 506, 144924. https://doi.org/10.1016/j.apsusc.2019.144924
- Alrawiq, H. S., & Idris, M. (2015). Preliminary test of mercury exposure on Paspalum vaginatum in phytoremediation process. International Journal of ChemTech Research, 7, 310–315. https://www.semanticscholar.org/paper/Preliminary-
- Amonoo-Neizer, E., Nyamah, D., & Bakiamoh, S. (1996). Mercury and arsenic pollution in soil and biological samples around the mining town of Obuasi, Ghana. Water, Air, and Soil Pollution, 91(3–4), 363–373. https://doi.org/10.1007/BF00666270
- Antiochia, R., Campanella, L., Ghezzi, P., & Movassaghi, K. (2007). The use of vetiver for remediation of heavy metal soil contamination. Analytical and Bioanalytical Chemistry, 388(4), 947–956. https://doi.org/10.1007/s00216-007-1268-1
- Antwi-Agyei, P., Hogarh, J. N., & Foli, G. (2009). Trace elements contamination of soils around gold mine tailings dams at Obuasi, Ghana. African Journal of Environmental Science and Technology, 3(11), 353-359. http://www.academicjournals.org/ajest
- Appiah, M., & Thomas, R. (1982). Inositol phosphate and organic phosphorus contents and phosphatase activity of some Canadian and Ghanaian soils. Canadian Journal of Soil Science, 62(1), 31–38. https://doi.org/10.4141/cjss82-004
- Armah, F. A., Quansah, R., & Luginaah, I. (2014). A systematic review of heavy metals of anthropogenic origin in environmental media and biota in the context of gold mining in Ghana. International Scholarly Research Notices, 2014, 1–37. https://doi.org/10.1155/2014/252148
- Ashraf, S., Ali, Q., Zahir, Z. A., Ashraf, S., & Asghar, H. N. (2019). Phytoremediation: Environmentally sustainable way for reclamation of heavy metal polluted soils. Ecotoxicology and Environmental Safety, 174, 714–727. https://doi.org/10.1016/j.ecoenv.2019.02.068
- Banchirigah, S. M. (2008). Challenges with eradicating illegal mining in Ghana: A perspective from the grassroots. Resources Policy, 33(1), 29–38. https://doi.org/10.1016/j.resourpol.2007.11.001
- Bempah, C. K., Ewusi, A., Obiri-Yeboah, S., Asabere, S. B., Mensah, F., Boateng, J., & Voigt, H.-J. (2013). Distribution of arsenic and heavy metals from mine tailings dams at Obuasi Municipality of Ghana. American Journal of Engineering Research, 2(5), 61–70. http://citeseerx.ist.psu.edu/viewdoc/download?doi=10.1.1.683.3570
- Black, C. A. (1965). Methods of soil analysis Part 1. American Society for, Testing Materials, American Society of, Agronomy.
- Boateng, E., Dowuona, G., Nude, P., Foli, G., Gyekye, P., & Jafaru, H. (2012). Geochemical assessment of the impact of mine tailings reclamation on the quality of soils at AngloGold concession, Obuasi, Ghana. Research Journal of Environmental and Earth Sciences, 4(4), 466–474.
- Bortey-Sam, N., Nakayama, S. M., Akoto, O., Ikenaka, Y., Baidoo, E., Mizukawa, H., & Ishizuka, M. (2015). Ecological risk of heavy metals and a metalloid in agricultural soils in Tarkwa, Ghana. International Journal of Environmental Research and Public Health, 12(9), 11448–11465. https://doi.org/10.3390/ijerph120911448
- Broadley, M. R., White, P. J., Hammond, J. P., Zelko, I., & Lux, A. (2007). Zinc in plants. New Phytologist, 173, 677–702. DOI: 10.1111/j.1469-8137.2007.01996.x
- Chatterjee, S., Mitra, A., Datta, S., & Veer, V. (2013). Phytoremediation protocols: An overview. Plant-Based Remediation Processes. Springer. 1-18. https://doi.org/10.1007/978-3-642-35564-6_1
- Chekol, T., Vough, L. R., & Chaney, R. L. (2004). Phytoremediation of polychlorinated biphenyl-contaminated soils: The rhizosphere effect. Environment International, 30(6), 799–804. https://doi.org/10.1016/j.envint.2004.01.008
- Chudasama, B., Porwal, A., Kreuzer, O. P., & Butera, K. (2016). Geology, geodynamics and orogenic gold prospectivity modelling of the Paleoproterozoic Kumasi Basin, Ghana, West Africa. Ore Geology Reviews, 78, 692–711. https://doi.org/10.1016/j.oregeorev.2015.08.012
- Cunningham, S. D., & Berti, W. R. (1993). Remediation of contaminated soils with green plants: An overview. In Vitro Cellular & Developmental Biology-Plant, 29(4), 207–212. https://doi.org/10.1007/BF02632036
- Cunningham, S. D., & Ow, D. W. (1996). Promises and prospects of phytoremediation. Plant Physiology, 110(3), 715. https://doi.org/10.1104/pp.110.3.715
- Danh, L. T., Truong, P., Mammucari, R., Tran, T., & Foster, N. (2009). Vetiver grass, vetiveria zizanioides: a choice plant for phytoremediation of heavy metals and organic wastes. International Journal of Phytoremediation, 11(8), 664–691. https://doi.org/10.1080/15226510902787302
- Das, M., & Maiti, S. K. (2009). Growth of cymbopogon citratus and vetiveria zizanioides on Cu mine tailings amended with chicken manure and manure-soil mixtures: A pot scale study. International Journal of Phytoremediation, 11(8), 651–663. https://doi.org/10.1080/15226510802568547
- Davis, D., Hirdes, W., Schaltegger, U., & Nunoo, E. (1994). U Pb age constraints on deposition and provenance of Birimian and gold-bearing Tarkwaian sediments in Ghana, West Africa. Precambrian Research, 67(1–2), 89–107. https://doi.org/10.1016/0301-9268(94)90006-X
- Duncan, R. R., & Carrow, R. N. (2000). Seashore paspalum: The environmental turfgrass. John Wiley & Sons.
- Embrandiri, A., Kiyasudeen, S. K., Rupani, P. F., & Ibrahim, M. H. (2016). Environmental xenobiotics and its effects on natural ecosystem. Plant Responses to Xenobiotics. Springer.
- Epstein, E. (1972). Mineral nutrition of plants: Principles and perspectives, pp 412, ISBN : 047124340X.
- Ernst, W. (1996). Bioavailability of heavy metals and decontamination of soils by plants. Applied Geochemistry, 11(1–2), 163–167. https://doi.org/10.1016/0883-2927(95)00040-2
- Ewusi, A., Obiri-Yeboah, S., Voigt, H.-J., Asabere, S. B., & Bempah, C. K. (2013). Groundwater quality assessment for drinking and irrigation purposes in Obuasi municipality of Ghana, a preliminary study. Research Journal of Environmental and Earth Sciences, 5(1), 6–17. https://doi.org/10.19026/rjees.5.5633
- Gholipour, M., Mehrabanjoubani, P., Abdolzadeh, A., Raghimi, M., Seyedkhademi, S., Karimi, E., & Sadeghipour, H. R. (2020). Facilitated decrease of anions and cations in influent and effluent of sewage treatment plant by vetiver grass (Chrysopogon zizanioides): The uptake of nitrate, nitrite, ammonium, and phosphate. Environmental Science and Pollution Research, 27, 21506–21516. https://doi.org/10.1007/s11356-020-08677-5
- Ghosh, M., & Singh, S. (2005). A review on phytoremediation of heavy metals and utilization of it’s by products. Asian Journal on Energy & Environment, 6(4), 214 - 231.
- Gyamfi, E., Appiah-Adjei, E. K., & Adjei, K. A. (2019). Potential heavy metal pollution of soil and water resources from artisanal mining in Kokoteasua, Ghana. Groundwater for Sustainable Development, 8, 450–456. https://doi.org/10.1016/j.gsd.2019.01.007
- Hakami, B. A. (2015). Impacts of soil and water pollution on food safety and health risks. Technology, 6(11), 32–38. http://www.iaeme.com/IJCIET/issues.asp?JType=IJCIET&VType=6&IType=11
- Hakanson, L. (1980). An ecological risk index for aquatic pollution control. A sedimentological approach. Water Research, 14(8), 975–1001. https://doi.org/10.1016/0043-1354(80)90143-8
- Hamzah, A., Kusuma, Z., Utomo, W., & Guritno, B. (2012). Siam weed (Chromolaena odorata L.) for phytoremediation of artisanal gold mine tailings. Journal of Tropical Agriculture, 50(10), 88–91.
- Harter, R. D. (1983). Effect of soil pH on adsorption of lead, copper, zinc, and nickel 1. Soil Science Society of America Journal, 47(1), 47–51. https://doi.org/10.2136/sssaj1983.03615995004700010009x
- Hartley, W., Dickinson, N. M., Riby, P., & Lepp, N. W. (2009). Arsenic mobility in brownfield soils amended with green waste compost or biochar and planted with Miscanthus. Environmental Pollution, 157(10), 2654-2662. doi:10.1016/j.envpol.2009.05.011
- Hayford, E., Amin, A., Osae, E., & Kutu, J. (2009). Impact of gold mining on soil and some staple foods collected from selected mining communities in and around Tarkwa-Prestea area. West African Journal of Applied Ecology, 14(1), 1-12. https://doi.org/10.4314/wajae.v14i1.44708
- Henry, H. F., Burken, J. G., Maier, R. M., Newman, L. A., Rock, S., Schnoor, J. L., & Suk, W. A. (2013). Phytotechnologies – preventing exposures, improving public health. International Journal of Phytoremediation, 15(9), 889–899. https://doi.org/10.1080/15226514.2012.760521
- Hilson, G., Hilson, A., & Adu-Darko, E. (2014). Chinese participation in Ghana’s informal gold mining economy: Drivers, implications and clarifications. Journal of Rural Studies, 34, 292–303. https://doi.org/10.1016/j.jrurstud.2014.03.001
- Hilson, G., & Potter, C. (2003). Why is illegal gold mining activity so ubiquitous in rural Ghana? African Development Review, 15(2–3), 237–270. https://doi.org/10.1111/j.1467-8268.2003.00073.x
- Kabata-Pendias, A. (2000). Trace elements in soils and plants. CRC press.
- Kabata-Pendias, A., & Mukherjee, A. B. (2007). Trace elements from soil to human. Springer Science & Business Media.
- Karikari, E., Castro-Sotomayor, J., & Asante, G. (2020). Illegal mining, identity, and the politics of ecocultural voice in Ghana. In Tema, M., José, C. (Eds.), Routledge Handbook of Ecocultural Identity (pp. 240–259).
- Khan, A. G. (2005). Role of soil microbes in the rhizospheres of plants growing on trace metal contaminated soils in phytoremediation. Journal of Trace Elements in Medicine and Biology, 18(4), 355–364. https://doi.org/10.1016/j.jtemb.2005.02.006
- Kim, I. S., Kang, K. H., Johnson-Green, P., & Lee, E. J. (2003). Investigation of heavy metal accumulation in Polygonum thunbergii for phytoextraction. Environmental Pollution, 126(2), 235–243. https://doi.org/10.1016/S0269-7491(03)00190-8
- Kinnersley, A. (1993). The role of phytochelates in plant growth and productivity. Plant Growth Regulation, 12(3), 207–218. https://doi.org/10.1007/BF00027200
- Kortei, N. K., Heymann, M. E., Essuman, E. K., Kpodo, F. M., Akonor, P. T., Lokpo, S. Y., Boadi, N. O., Ayim-Akonor, M., & Tettey, C. (2020). Health risk assessment and levels of toxic metals in fishes (Oreochromis noliticus and Clarias anguillaris) from Ankobrah and Pra basins: Impact of illegal mining activities on food safety. Toxicology Reports, 7, 360–369. https://doi.org/10.1016/j.toxrep.2020.02.011
- Kumar, A., & Maiti, S. K. (2015). Effect of organic manures on the growth of cymbopogon citratus and chrysopogon zizanioides for the phytoremediation of chromite-asbestos mine waste: A pot scale experiment. International Journal of Phytoremediation, 17(5), 437–447. https://doi.org/10.1080/15226514.2014.910174
- Kumar, P. N., Dushenkov, V., Motto, H., & Raskin, I. (1995). Phytoextraction: The use of plants to remove heavy metals from soils. Environmental Science & Technology, 29, 1232–1238.
- Kumarathilaka, P., Seneweera, S., Ok, Y. S., Meharg, A. A., & Bundschuh, J. (2020). Mitigation of arsenic accumulation in rice: An agronomical, physico-chemical, and biological approach–A critical review. Critical Reviews in Environmental Science and Technology, 50(1), 31–71. https://doi.org/10.1080/10643389.2019.1618691
- Kwaansa-Ansah, E. E., Armah, E. K., & Opoku, F. (2019). Assessment of total mercury in hair, urine and fingernails of small–scale gold miners in the Amansie West District, Ghana. Journal of Health and Pollution, 9(21), 190306. https://doi.org/10.5696/2156-9614-9.21.190306
- Lăcătuşu, R., Cîtu, G., Aston, J., Lungu, M., & Lăcătuşu, A. R. (2009). Heavy metals soil pollution state in relation to potential future mining activities in the Roşia Montană area. Carpathian Journal of Earth and Environmental Sciences, 4(2), 39–50.
- Landon, J. R. (2014). Booker tropical soil manual: A handbook for soil survey and agricultural land evaluation in the tropics and subtropics. Routledge.
- Lasat, M. (1999). Phytoextraction of metals from contaminated soil: A review of plant/soil/metal interaction and assessment of pertinent agronomic issues. Journal of Hazardous Substance Research, 2(1), 5. https://doi.org/10.4148/1090-7025.1015
- Lee, J. H. (2013). An overview of phytoremediation as a potentially promising technology for environmental pollution control. Biotechnology and Bioprocess Engineering, 18(3), 431–439. https://doi.org/10.1007/s12257-013-0193-8
- Liu, J., Xin, X., & Zhou, Q. (2018). Phytoremediation of contaminated soils using ornamental plants. Environmental Reviews, 26(1), 43–54. https://doi.org/10.1139/er-2017-0022
- Loska, K., & Wiechuła, D. (2003). Application of principal component analysis for the estimation of source of heavy metal contamination in surface sediments from the Rybnik Reservoir. Chemosphere, 51(8), 723–733. https://doi.org/10.1016/S0045-6535(03)00187-5
- Mahar, A., Wang, P., Ali, A., Awasthi, M. K., Lahori, A. H., Wang, Q., Li, R., & Zhang, Z. (2016). Challenges and opportunities in the phytoremediation of heavy metals contaminated soils: A review. Ecotoxicology and Environmental Safety, 126, 111–121. https://doi.org/10.1016/j.ecoenv.2015.12.023
- Mensah, A. K. (2015). Role of revegetation in restoring fertility of degraded mined soils in Ghana: A review. International Journal of Biodiversity and Conservation, 7(2), 57–80. https://doi.org/10.5897/IJBC2014.0775
- Mensah, A. K., Mahiri, I. O., Owusu, O., Mireku, O. D., Wireko, I., & Kissi, E. A. (2015). Environmental impacts of mining: A study of mining communities in Ghana. Applied Ecology and Environmental Sciences, 3(3), 81–94.
- Mensah, I., Boakye-Danquah, J., Suleiman, N., Nutakor, S., & Suleiman, M. D. (2020). Small-scale mining, the SDGs and human insecurity in Ghana. Africa and the Sustainable Development Goals. Springer. https://doi.org/10.1007/978-3-030-14857-7_8
- Nelson, D. W., & Sommers, L. E. (1996). Total carbon, organic carbon, and organic matter. Methods of Soil Analysis: Part 3 Chemical Methods, 5, 961–1010. https://doi.org/10.2136/sssabookser5.3.c34
- Nirola, R., Megharaj, M., Aryal, R., & Naidu, R. (2016a). Screening of metal uptake by plant colonizers growing on abandoned copper mine in Kapunda, South Australia. International Journal of Phytoremediation, 18(4), 399–405. https://doi.org/10.1080/15226514.2015.1109599
- Nirola, R., Megharaj, M., Beecham, S., Aryal, R., Thavamani, P., Vankateswarlu, K., & Saint, C. (2016b). Remediation of metalliferous mines, revegetation challenges and emerging prospects in semi-arid and arid conditions. Environmental Science and Pollution Research, 23(20), 20131–20150. https://doi.org/10.1007/s11356-016-7372-z
- Noll, M. R. (2003). Trace elements in terrestrial environments: Biogeochemistry, bioavailability, and risks of metals. Journal of Environmental Quality, 32(1), 374. https://doi.org/10.2134/jeq2002.3740
- Nsiah, P. K., & Schaaf, W. (2019). The potentials of biological geotextiles in erosion and sediment control during gold mine reclamation in Ghana. Journal of Soils and Sediments, 19(4), 1995–2006. https://doi.org/10.1007/s11368-018-2217-7
- Obiri-Danso, K., Weobong, C., & Jones, K. (2005). Aspects of health-related microbiology of the Subin, an urban river in Kumasi, Ghana. Journal of Water and Health, 3(1), 69–76. https://doi.org/10.2166/wh.2005.0007
- Ofori-Sarpong, G., & Amankwah, R. (2019). Potential of mine waste rock to generate acid mine drainage–a case study in South-Western Ghana. New Frontiers in Natural Resources Management in Africa. Springer. https://doi.org/10.1007/978-3-030-11857-0_6
- Paull, N. J., Krix, D., Irga, P. J., & Torpy, F. R. (2019). Airborne particulate matter accumulation on common green wall plants. International Journal of Phytoremediation, 1–13. https://doi.org/10.1080/15226514.2019.1696744
- Peng, W., Li, X., Xiao, S., & Fan, W. (2018). Review of remediation technologies for sediments contaminated by heavy metals. Journal of Soils and Sediments, 18(4), 1701–1719. https://doi.org/10.1007/s11368-018-1921-7
- Rangel, W. M., Thijs, S., Janssen, J., Oliveira Longatti, S. M., Bonaldi, D. S., Ribeiro, P. R. A., Jambon, I., Eevers, N., Weyens, N., & Vangronsveld, J. (2017). Native rhizobia from Zn mining soil promote the growth of Leucaena leucocephala on contaminated soil. International Journal of Phytoremediation, 19(2), 142–156. https://doi.org/10.1080/15226514.2016.1207600
- Riefner, R. E., JR, & Columbus, J. T. (2008). Paspalum vaginatum (Poaceae), a new threat to wetland diversity in southern California. Journal of the Botanical Research Institute of Texas, 2(1), 743–759. https://www.jstor.org/stable/41971700
- Sainger, P. A., Dhankhar, R., Sainger, M., Kaushik, A., & Singh, R. P. (2011). Assessment of heavy metal tolerance in native plant species from soils contaminated with electroplating effluent. Ecotoxicology and Environmental Safety, 74(8), 2284–2291. https://doi.org/10.1016/j.ecoenv.2011.07.028
- Salt, D. E., & Krämer, U. (2000). Mechanisms of metal hyperaccumulation in plants. Phytoremediation of Toxic Metals: Using Plants to Clean up the Environment, 231-245. John Wiley & Sons. http://hdl.handle.net/11858/00-001M-0000-0014-3525-3
- Saxena, G., Purchase, D., Mulla, S. I., Saratale, G. D., & Bharagava, R. N. (2020). Phytoremediation of heavy metal-contaminated sites: Eco-environmental concerns, field studies, sustainability issues, and future prospects. Reviews of Environmental Contamination and Toxicology, 249, 71–131. https://doi.org/10.1007/398_2019_24
- Schnoor, J. 1997. Phytoremediation. Technology evaluation report (E Series TE 98–101). Ground-Water Remediation Technologies Analysis Center.
- Shen, F., Mao, L., Sun, R., Du, J., Tan, Z., & Ding, M. (2019). Contamination evaluation and source identification of heavy metals in the sediments from the Lishui River Watershed, Southern China. International Journal of Environmental Research and Public Health, 16(3), 336. https://doi.org/10.3390/ijerph16030336
- Sheoran, V., Sheoran, A., & Poonia, P. (2010). Soil reclamation of abandoned mine land by revegetation: A review. International Journal of Soil, Sediment and Water, 3(2), 13. https://scholarworks.umass.edu/intljssw/vol3/iss2/13
- Singh, J., Yadav, P., Pal, A. K., & Mishra, V. (2020). Water pollutants: Origin and status. Sensors in Water Pollutants Monitoring: Role of Material, 5-20. Springer. https://doi.org/10.1007/978-981-15-0671-0_2
- Smith, A. J., Henry, G., & Frost-Killian, S. (2016). A review of the Birimian Supergroup-and Tarkwaian Group-hosted gold deposits of Ghana. Episodes, 39(2), 177–197.
- Sparks, D., Page, A., Helmke, P., Loeppert, R., Soltanpour, P., Tabatabai, M., & Johnston, C. (1996). Methods of soil analysis, part 3. Chemical Methods, 1085–1121.
- Sreelal, G., & Jayanthi, R. (2017). Review on phytoremediation technology for removal of soil contaminant. Indian Journal of Scientific Research, 14(1), 127–130.
- Terry, N., & Banuelos, G. S. (1999). Phytoremediation of contaminated soil and water. CRC Press.
- Tetteh, E. N., Logah, V., Twum-Ampofo, K., & Partey, S. T. (2015). Effect of duration of reclamation on soil quality indicators of a surface–mined acid forest oxisol in South–Western Ghana. West African Journal of Applied Ecology, 23(2), 63–72.
- Velasco-Alinsug, M. P., Rivero, G. C., & Quibuyen, T. A. O. (2005). Isolation of mercury-binding peptides in vegetative parts of Chromolaena odorata. Zeitschrift für Naturforschung C, 60(3–4), 252–259. https://doi.org/10.1515/znc-2005-3-409
- Vodouhe, F. G., & Khasa, D. P. (2015). Local Community perceptions of mine site restoration using phytoremediation in abitibi-temiscamingue (Quebec). International Journal of Phytoremediation, 17(10), 962–972. https://doi.org/10.1080/15226514.2014.981238
- Wasino, R., Likitlersuang, S., & Janjaroen, D. (2019). The performance of vetivers (Chrysopogon zizaniodes and Chrysopogon nemoralis) on heavy metals phytoremediation: Laboratory investigation. International Journal of Phytoremediation, 21(7), 624–633. https://doi.org/10.1080/15226514.2018.1546275
- WHO. (1996). Permissible limits of heavy metals in soil and plants. Geneva, Switzerland: World Health Organisation.
- Wong, M. H. (1985). Heavy metal contamination of soils and crops from auto traffic, sewage sludge, pig manure and chemical fertilizer. Agriculture, Ecosystems & Environment, 13(2), 139–149. https://doi.org/10.1016/0167-8809(85)90056-8
- Wongsasuluk, P., Chotpantarat, S., Siriwong, W., & Robson, M. (2014). Heavy metal contamination and human health risk assessment in drinking water from shallow groundwater wells in an agricultural area in Ubon Ratchathani province, Thailand. Environmental Geochemistry and Health, 36(1), 169–182. https://doi.org/10.1007/s10653-013-9537-8
- Wuana, R. A., & Okieimen, F. E. (2011). Heavy metals in contaminated soils: A review of sources, chemistry, risks and best available strategies for remediation. Isrn Ecology, 2011. https://doi.org/10.5402/2011/402647
- Yang, X., Feng, Y., He, Z., & Stoffella, P. J. (2005). Molecular mechanisms of heavy metal hyperaccumulation and phytoremediation. Journal of Trace Elements in Medicine and Biology, 18(4), 339–353. https://doi.org/10.1016/j.jtemb.2005.02.007
- Yao, Z., Li, J., Xie, H., & Yu, C. (2012). Review on remediation technologies of soil contaminated by heavy metals. Procedia Environmental Sciences, 16, 722–729. https://doi.org/10.1016/j.proenv.2012.10.099
- Zacchini, M., Pietrini, F., Mugnozza, G. S., Iori, V., Pietrosanti, L., & Massacci, A. (2009). Metal tolerance, accumulation and translocation in poplar and willow clones treated with cadmium in hydroponics. Water, Air, and Soil Pollution, 197(1–4), 23–34. https://doi.org/10.1007/s11270-008-9788-7
- Zhao, F., Hamon, R., & Mclaughlin, M. J. (2001). Root exudates of the hyperaccumulator Thlaspi caerulescens do not enhance metal mobilization. New Phytologist, 151(3), 613–620. https://doi.org/10.1046/j.0028-646x.2001.00213.x