Abstract
The study was designed to investigate the impact of First Principles of Instruction (FPI) with computer animation and chart, and their functional effect on gender to acquire expertise in science in Junior High School (JHS). The content for the treatments was selected from JHS integrated science syllabus. The contents of the instructional media were designed in line with the specifications of Principles of Multimedia Learning (PML). All the learning tasks for the two treatments were designed based on FPI. Eighty- seven second-year JHS students (male = 48, female = 39) with a mean age of 14.4 years were randomly assigned to the two treatments. Ateacher was trained to deliver the content. The main study consisted of three sessions, which were delivered in the regular classroom and computer laboratory. Paired-sample and independent t-tests and univariate analyses were used to analyze the data. The result showed that there was no statistically significant difference between the two treatments. In addition, the result revealed no significant interaction effect on gender and the two treatments for the acquisition of expertise in science at the basic school. The findings are novel and suggest that static/inexpensive instructional media (e.g.chart) carefully designed with specifications of PML integrated withFPI is equally effective as dynamic instructional media (e.g., computer animation) for the development of expertise in science in the context of traditional classrooms of basic schools irrespective of the gender of the students.
PUBLIC INTEREST STATEMENT
There is general realization that a strong foundation in science education will enhance analytical and problem-solving skills among learners to solve unfamiliar problems. Consequently, governments have placed much importance on science education; however, students are still performing abysmally. Several factors such as poor teaching methodologies and lack of teaching and learning materials have been attributed to the students’ low performance. There is growing scientific evidence on instructional design that suggests the instructional intervention of First Principles of Instruction (FPI) is the most effective means of promoting scientific expertise among the learners. Additionally, instructional technology literature indicates that the effective design of multimedia instruction will further enhance the development of scientific expertise. In this regard, this quasi-experimental study sought to provide empirical and new insights into the effectiveness of well-designed chart and computer animation instructional media integrated into FPI to develop scientific expertise among males and female students in the traditional basic school classroom.
1. Introduction
The complexityof the world has made teaching and learning of science at all levels ofthe educational system a vital issue to both developed and developing countries (Kelley & Knowles, Citation2016). There is a general realization that a strong foundation in the teaching and learning of science, especially at the basic level, enhance reasoning, analytical, and problem-solving skills to solve unconventional problems (National Academy of Sciences, Citation1998). The growing interest in Trends in International Mathematics and Science Studies (TIMSS) and Programme for International Student Assessment (PISA) is evidence of the global interest and importance placed on developing expertise in science among basic school students.
In Ghana, the science curriculum (also known as integrated science at the basic level of the educational system) is designed to help every learner to acquire general 21st century scientific literacy. In this respect, science is a compulsory subject in the basic schools in Ghana, and good (credit grade or better) performance at the basic level is the basic requirement for further education. That notwithstanding the critical importance placed on the teaching and learning of science at the basic schools, students performances in the subject are still low especially in Ghana (Buabeng, Acheaw & Danso, Citation2014; Coughlan, Citation2015; Gardner, Citation2011; Majo, Citation2016; West Africa Examination Council, Citation2018, Citation2019). Developingscience expertise, especially among girls, at the basic schools is also a problem in countries in sub-saharan and also developed countries (Cunningham, Citation2007; Gardner, Citation2011; UNESCO, Citation2017), including Ghana. In this study, expertise in science (in the basic schools) is defined as the coordinated and integrated set of knowledge, understanding and problem-solving skills required for basic education students to solve real-life problems related to diffusion and osmosis.
Students’ low performance in science at both international and local levels has activated much attention, debates, and discussions among government officials, educational practitioners, parents, employers and educational (technology) researchers. Several factorssuch as lack of adequate and well-designed teaching and learning materials, poor teaching methodology, and lack of fundinghave been attributed to students’ low performance in science(King’aru, Citation2014; Majo, Citation2016).
However, there is enough scientific evidence from the literature on instructional design that good design of intervention in line with FPI and Four Components Instructional Design Model (4 /ID) is the most effective means of promoting the development of expertise (Gardner, Citation2011; Merrill, Citation2004; Sarfo & Elen, Citation2007; Sarfo, Eshun, Elen & Adentwi, Citation2014; van Merriënboer, Citation1997). Similarly, the literature on teaching and learning of science indicates that the effective design of multimedia instruction will further enhance the development of expertise (Mayer, Citation2002, Citation2008). Thus, appropriate digital multimedia (e.g., computer animation) integrated intothe excellent design of intervention facilitates learning better than static media (e.g., chart) (Dziedzic & Barszcz, Citation2015; Gambani,Falode & Adegbenra, Citation2014). On the other hand, Clark (Citation2001) and Sarfo et al., (Citation2014) argued that a well-designed static media (e.g., chart) integrated intothe effective intervention would equally enhance learning as the computer animation. However, little is known about the effectiveness of FPI integrated with static media and computer animation to promote the development of expertise in science in the green context of traditional classrooms of the basic schools in Ghana.In line with these arguments, this study is experimentally designed to investigate the effectiveness of well-designed static instructional media (e.g., chart) and dynamic instructional media (e.g., computer animation) in the context of FPI (Merrill, Citation2004) and PML (Mayer, Citation2002, Citation2008) for the acquisition of expertise in science in basic schools. Furthermore, the study examines the impact of FPI with well-designed chart and computer animation (as instructional media) on male and female students in basic schools to develop expertise in integrated science.
is described as a set of interrelated principles that increase student’s learning when applied correctly in an instructional product or setting (Merrill, Citation2009). Similar principles have been identified by other authors (van Merrienboer & Kirschner, Citation2007). This FPI is always right under appropriate conditions; they promote more effective, efficient, or engaging learning (Merrill, Citation2009). Theyare 1) task centeredness, 2) activation, 3) demonstration, 4) application and 5) integration. highlights this.
Figure 1. Phases for effective instruction by Merrill (Citation2002)
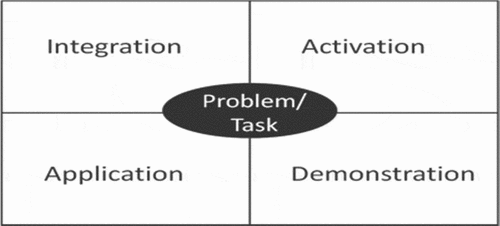
The first principle (task centeredness) states that the most effective learning environments are task-centred. Thus, learning is promoted if learners are engaged in solving challenging realistic problems (tasks). Merrill used the word’problem’to include a wide range of activities, with the most critical characteristics being that the activity is the whole task rather than only components of a task, and that the task is authentic and representative of those the learners will encounter in the real world after instruction. Problem-based learning is well represented by several recent instructional models, including van Merriënboer’s (Citation1997) whole task practice in 4 C/ID Model.
The second prescriptive principle (activation) emphasizes that learning is promoted when relevant prior knowledge is activated. Activation involves stimulating those existing knowledge structures of the learners that can be modified or tuned to enable learners incorporate the new information. Stimulating the learners’ prior knowledge and making it active for use in the working memory as a foundation for new information is the key to productive and successful learning.
The third prescriptive principle indicates that demonstration, which can show learners what to do, is an effective ingredient of powerful learning environments. Merrill (Citation2002) postulated that learning is facilitated when the demonstration is consistent with the learning goal; (a) examples and non-examples for concepts, (b) demonstrations for procedures, (c) visualizations for processes, and (e) modelling for behaviour. van Merriënboer (Citation1997) reported that showing a learner what to do through worked-out and modelling examples that illustrate the learner how to solve the problem and practice are essential dimensions for successful learning.
The fourth prescriptive principle application asserts that learning is promoted when learners use their new knowledge to solve problems. Another method of effective instruction concerningthe application is scaffolding (van Merriënboer, Citation1997). The assumption is that learners need intensive and considerable support at the beginning of learning, but as the learning progresses and the learners achieve expertise, this support is gradually taken away, eventually leaving the learners to solve the problem independently. Providing feedback to learners while solving design problems (applying knowledge and skills) has long been recognized as the most important form of guidance.
The fifth prescriptive principle focuses on integration. Learning is facilitated when learners are encouraged to integrate (transfer) the new knowledge or skill into their everyday life. This happens when learners can publicly reflect on, discuss, and defend their new knowledge or skill, and (learners) are highly motivated when they can do this (Merrill, Citation2002).
The FPI is general in the sense that it applies to any delivery system (e.g., pictures, models, charts, and computer) or any instructional architecture (e.g., tutorial method, exploratory method; Merrill, Citation2009).Wilujeng, Kusumah and Darhim (Citation2019) applied Merrill’s first principles of instruction to develop students’ achievement of algebraic thinking ability. The study adopted a posttest-only, control quasi-experimental design, and it was found that students who experienced FPI learning environment performed better than those who experienced a conventional learning environment. In applying the first principles of instruction in a short-term, high volume, rapid production of online professional development modules, Klein and Mendenhall (Citation2018) found that project requirements, personnel, physical setting, time, designer experience, training and team meetings were influenced by the use of the FPI. Similarly, Frick, Chadha, Watson, Wang and Green (Citation2007) surveyed 140 students in 89 higher education institutions to investigate the correlation between academic learning time and FPI and student mastery of course objectives. The study results indicated that students were nine times more likelytoreport that they had mastered course content when FPI was used. In another research, Gardner (Citation2011) demonstrated that FPImay increase students’ learningifused in instructional strategy to teach undergraduate biology courses. Recently, other scientific studies of Anderson, Tredway and Calice (Citation2015), Badali, Derakhshi, Bagheri and Ansari (Citation2016), Emamiyan-Kheshti, Ghasemi, Mehraji, Kazem Banihashelu and Badali (Citation2016), Gardner and Belland (Citation2017), Gardner, Barclay, Kong and LeVally (Citation2019), Jacob (Citation2018), Kuba, Rahimi, Smith, Shute and Dai (Citation2021), Mendenhall, (Citation2012), Truong, Elen and Clarebout (Citation2019), Yorganci (Citation2020) and Zarei, Badali and Amir (Citation2014)have equally demonstrated the effectiveness of FPI in developing complex skills like scientific problem-solving skills among the students.
There is research evidence in the literature to support the First Principles of Instruction’s effectiveness in teaching and learning science. However, most of these studies (e.g., Anderson et al., Citation2015; Badali et al., Citation2016; Emaniyan-Kheshi et al.,Citation2016; Frick et al., Citation2007; Gardner, Citation2011; Gardner et al., Citation2019; Mendenhall, Citation2012; Yorganci, Citation2020) were conducted in higher institutions and therefore, the research evidence on the impact of FPI in teaching and learning of science at the basic level is lacking.As indicated, the present study’s terminal objective is focused on developing expertise to solve real-life problems related to diffusion and osmosis. To achieve this objective in the traditional classroom of basic schools in the context of innovative instruction/teaching designed in line with FPI, the teacher should engage the learners with: 1) authentic, realistic problems or tasks; 2) activation 3) presentation of general information and demonstrations (examples); and 4) application related to diffusion and osmosis; then, support the learners to use the expertise acquired in the classroom to solve real-life problems.
In this study (and in the context of FPI), the demonstrationshowsworking examples of the problems or the task(s) related to diffusion and osmosis. Thus, it shows how the information presented on diffusion and osmosis is applied to the specific context. The theory behind this is that when only the general information is given, it is stored in the learners’ associative memory; they can only recall but can further apply if they construct/create the schema for the information. Without demonstration, learners may not be capable of building schema or building an inadequate schema (Merrill, Citation2012). A demonstration or showing of the specific steps involved in the process of osmosis and diffusion and information presentation helps learnersto create a good schema or mental model to enable them to apply the information in new situations.
Research evidence by Clark and Mayer (Citation2003) and Mayer (Citation2002) shows that relevant media (or multimedia) integrated into demonstration further promote learning efficiency, effectiveness and engagement. Multimedia is the presentation of material using both words (e.g.,printed and spoken text) and pictorial (e.g., static graphic including illustrations, charts or dynamic graphics including animation, video)(Mayer, Citation2002). Employing words and graphics to show examples (or to demonstrate) and explanations of the process and the concepts (such as high concentration area, low concentration area, concentration gradient, weaker solution, movement of molecules) of diffusion and osmosis enable students to understand and effectively apply the process of diffusion and osmosis inspecific real-life situations. As depicted in the task analysis above, the process of osmosis and diffusion is involved in topics/objectives, such as understanding and application of concepts, such as the movement of invisible molecules. Mayer (Citation2002) and Ainsworth and VanLabeke (Citation2008) indicated that in instructional settings, invisible concepts or processes could be more appropriately represented by dynamic media (e.g., animation). Animation is described as a form of representation that displays a process that changes over time (Ainsworth & VanLabeke, Citation2008). Taking into consideration its processing and multimedia capabilities, the computer can be argued to animate and present dynamic graphics illustrating or showing examples on the concepts and movement of molecules related to the processes of diffusion and osmosis more clearly, realistically, and effectively than chart (static graphic) (Gambani et al., Citation2014). This is because animation shows many elements’ mode of functioning (Dziedzic & Barszcz, Citation2015). Using computer animation in teaching facilitates students’ understanding of the materials andgives them a general idea of a given issue (Altipormark, Citation2014; Dziedzic & Barszcz, Citation2015). However, as demonstrated by Clark (Citation2001) and depicted in the numerous “non-significant-research results (http://www.nonsignificandifference.org), computers in themselves will not lead to better learning gain. Clark (Citation2001) demonstrated that the necessary condition or active ingredient of intervention which is sufficient to cause learning from instruction, is best characterized as an instructional method, not media or computer. The instructional method activates, compensates, or supplants the cognitive processes necessary for learning (Clark, Citation2001).All methods (e.g., demonstration, examples) required for learning can be effectively delivered by the diversity of media (both low-cost {e.g., chart} and high-cost {e.g., computer). Following from the above argument, it is proposed/hypothesized that if both chart and computer are expertly designed in line with five PML (Mayer, Citation2002, Citation2008) in the context of FPI to support the delivery of demonstration/example, both can equally and effectively promote the achievement of the terminal objective in the green context of the traditional classroom of basic schools. The five PML are the multimedia principle, the contiguity principle, the coherency principle, the modality principle, and the redundancy principle (Mayer, Citation2002).
Another issue of concern is gender disparity in the learning of science-oriented courses. According to UNESCO (Citation2017), the gender disparity in the Science, Technology, Engineering, and Mathematics (STEM) disciplines is disturbing and still requires urgent attention at various educational levels. Most of the time, girls believe that STEM subjects are “masculine” ones, and in this regard, female ability in STEM is innately inferior to that of the male. According to UNESCO (Citation2017), this conception undermines girls’ confidence, interest, and attitude to engage in STEM subjects such as science. Research shows that male and female students are qualitatively different in learningscience subjects (Greefield, Citation1997).Empirically, several studies conducted to examine the effect of gender on students’ performance in STEM revealed some conflicting results. Some research revealed no gender effect on the students’ performance (Ajayi & Ogbeba, Citation2017; Kola & Taiwo, Citation2013; Obadiaru, Citation2020).
On the other hand, some investigations reported a significant difference between gender and students’ performance (Filgona & Sababa, Citation2017; Musa & Samuel, Tile, Citation2013). Even those studies that found differences between gender and students’ performance are inconclusive. Some studies indicated that males performed better than their female counterparts (Gipps, Citation2004; Musa & Samuel, Citation2019) whereas other studies found results to the contrary (Soyibo, Citation2009; Tile, Citation2013). Based on these results, it can be argued that it is not clear that the findings on the effect of gender on students’ performance in STEM is conclusive. Supporting this view, Potvin and Hasni (Citation2014) concluded that there are no clear patterns on gender gaps in students’ performance internationally. The inclusion of gender analysis in this study will therefore help to have a better understanding of the possible differences in students’ performance,particularly within the powerful (innovative) learning environment. In this wise, this current study was propelled by two major reasons. First, globally, studies investigating the effect of gender on students’ academic achievement in science within the FPI frameworkare scarce. Second, the findings from previous studies on the impact of gender and students’ academic achievement in science are fuzzy. Thus, the study also sought to ascertain the influence of gender and students’ academic achievement in science. This current study would provide valuable insights and information for educational authorities and policymakers on factors that promote the development of problem-solving skills within Merrill’s FPI. Consequently, the present study addresses the issue again within the instructional intervention of FPI, which is a new and different setting within the Ghanaian context.
Overthe years, gender differences in the use of technology have attracted much attention in educational research and practice. Extant literature indicates that males and females have been characterized by a range of social and biological differences (Joiner,et al., Citation2011). The role of gender differences in using media and technology has been also explored (Kahveci, Citation2010; Sarfo, Amankwah & Konin, Citation2017). Some previous studies found that using technology for learning is a dominant activity for males as males have positive attitudes towards using technology for learning, especially in developing countries (Mariscal, Aneja & Sorgner, Citation2019; Rashid, Citation2016). Additionally, the literature has established that when equal access is provided to all students, females are less likely to use (computer) technologies than males because females perceive that using technology is predominately a male activity (Rashid, Citation2016; Stoet & Geary, Citation2018). The literature suggests that the possible reasons for such gender imbalances are socially constructed and are not related to innate ability (Joiner et al., Citation2011). As a result,Wu and Anderson (Citation2015) suggested that to make STEM education more relevant to girls and boys, educational technology such as digital games, simulation or animation, and more innovative intervention should be essential topics for STEM education educational technology researchers. This, therefore, necessitates further systematic investigation, especially within FPI with chart and computer animation as relatively little is known about gender effect within this learning environment.
This study aims at providing empirical evidence and new insights into the effectiveness of well-designed chart and computer animation instructional media integrated into FPI to develop expertise in science among males and female students in the traditional classroom of basic schools. To achieve this purpose, the following research questions are generated: (1) What is effect of learning environments designed in line with FPI with computer animation (high cost and dynamic) and chart (low cost and static) instructional media for the acquisition of expertise in science in the traditional classroom of basic school? (2) What is the interaction effect of gender on learning environments designed in line withFPI integrated with computer animation and chart for development of scientific expertise at the basic schools?
2. Methods
2.1. Research design
Considering the purpose of the study, a quasi-experimental design was utilized.This design was adopted since it involves selecting groups upon which a variable is tested without any random pre-selection processes. According to Cohen, Manion and Morrison (Citation2018), quasi-experimental design is used where true experimental research is not feasible, mostly in educational research, and involves intact classes. As was the case with this study, it was extremely difficult to reorganize all the classes to accommodate randomized controlled trial. Specifically, pretest-posttest consisting of two treatment designs were utilized. For this quasi-experimental design, second-year JHS students fromone school were selected and randomly assigned to each of the two experimental groups. Experimental group one received treatment of First Principles of Instruction with the chart (FPIC) or First Principles of Instruction with low-cost media (FPILM),and experimental group two received treatment of First Principles of Instruction with computer animation (FPICA) or First Principles of Instruction with high-cost media (FPIHM). The two treatments were designed and validated by an expert instructional designer and a subject matter expert/teacher. Both groups responded to pretest and posttestto measure changes in students’ performance in the two treatments.
2.2. Participants
The study participants were second-year junior high school students selected from one of the junior high schools in the Kumasi Metropolis of the Ashanti Region in Ghana. The choice of the population was purposive because this target population for the current study (JHS 2) constituted a better source for obtaining the accurate data needed for the study.This is supported by Dyel (Citation2011) who argued that JHS 2 students are considered suitable for this type of study by their academic experience since they had spent one year already studying the subject. In this study, form one students of JHS could not be used because they had justbeing introduced to the subject. In comparison, form three students were busy with their preparation towardstheir Basic Education Certificate Examination (BECE). To reduce maturation and history effects on the study, the researchers had discussions with the school authorities, including the science teachers in the school, upon which some timelines for the study was developed. Based on the timelines developed, it was agreed that topics like “osmosis and diffusion” should be taught as indicated in the school’s academic calendar. Therefore, the choice of these topics was just coincidental. Thus, no other factors influenced the researchers in terms of the choice of osmosis and diffusion. In all, eighty-seven (87) students (mean age = 14.4 years and SD = 1.3) consisting of 48 males and 39 females were involved in the study. A science (subject) teacher who was computer literate also participated in the study.
2.3. Research materials
The research materials were made up of 1) materials and teachers’ guidelines for the two treatments (FPIC and real object; and FPICA and real object), and 2) Pre- and post- assessment tasks identical for the two treatments. The two treatments were designed to help learners achieve the terminal objective: applying their knowledge and understanding of diffusion and osmosis’s concepts and principles to solve real-life problems.The content of the two treatments was identical and based on four topics selected from the syllabus. The information on the topics were chosen from the required textbook and some provided by the subject matter expert and subject teacher with guidelines from the instructional designer (Principal researcher). Again, all the two treatments were designed based on FPI: 1) learning tasks in the form of the case study; 2) activation, 3) demonstration/examples, and 4) application. In addition, both information on the charts and information on the computer was designed based on PML (Mayer, Citation2002, Citation2008) ( highlights this).
Table 1. Principles of multimedia learning
Body spray as a real object was used in both treatments. Identical teaching methods such as case study, discussion, role play, demonstrations/examples were employed in each treatment. Each treatment consisted of one lesson of 75 minutes (this is a reasonable time for two teaching periods in JHS in Ghana).
The treatments varied in terms of the instructional media provided by the teacher. In the FPI learning environment with charts, the information in the form of static graphics was designed, based on Mayer’s five PML, on some cardboard with supervision, explanation, or narration provided by the teacher during the teaching. In the FPI with computer animation learning environment, the information in the form of static graphics and dynamic graphic (animation) with narration or explanation was designed on a computer-based on the same five PML by Mayer (Citation2008); the teacher provided supervision, additional narration/explanation were necessary during the teaching. Both the chart and computer animation were used during the teaching to deliver or support the delivering ofdemonstrations/examples ofthe illustration of the 1) process of diffusion in gas, 2) the process of diffusion in liquid, 3) process of osmosis and 4) effect of plant cells and animal cells on different solutions (see Appendices). It is important to note that the redundancy principle, which involves animation (e.g., the movement of molecules in gas and liquid and the effect of plant cells and animal cells in different solutions related to diffusion and osmosis)and narration from the teachercould not fully apply under the FPI with the chart learning environment. The teacher did his best during the teaching to provide narration/explanation to support the static graphics on the chart. Still, neitherthe teacher nor the chart could provide the animation (e.g., the movement of moleculesin gas and liquid and the effect of plant cells and animal cells in different solutions related to diffusion and osmosis). However, the teacher and computer animation fully applied the redundancy principle under FPI with the computer learning environment (refer to ). This is due to the multimedia capabilities and processing capacities of the computer.
The assessment test consisted of 20 pretest and posttest items assessing knowledge, understanding and problem solving related to diffusion and osmosis. There were: 1) 10 knowledge items consisting of five pretest knowledge items (e.g., when sugar is mixed with water, equilibrium is reached when …) and their equivalent five posttest knowledge items (e.g., equilibrium is reached when salt is put in water, at a point …) to measure students’ ability to define and recall facts, principles, and procedures related to diffusion and osmosis; 2) four pretest understanding items (e.g., explain what will happen to animal cell when it is placed in a solution whose concentration is higher than that of the cell) and their equivalent four posttestunderstanding items (e.g., explain what will happen to a plant cell when it is placed in a solution whose concentration is lower than that of the cell) to measure students’ ability to explain with examples the concepts and processes related to diffusion and osmosis, and 3) one pretest problem solving item and its equivalent posttest similar to the terminal objective to measure students’ ability to solve real-life problems related to diffusion and osmosis.
2.4. Procedure
After thepilot test, the materials were reviewed by the researchers, including the expert instructional designer and the teacher was retrained to be able to deliver the lessons systematically as required for treatment fidelity (Krathwohl, Citation1995). The main study consisting of three sessions was conducted after the pilot test.
The first day (first session) of the experiment was used to administer the pretest. All the 87 students were arranged in two classrooms in the school. Each student was given a code as an index number. The administration of the pretest took 45 minutes. After the pretest, the students wererandomly divided into two groups and randomly assigned to the two treatments: the FPIC group consisted of 44 students (26 males and 18 females), and the FPICM group consisted of 43 students (25 males and 18 females). On the second day (second session),both the principal researcher and teacher, together with the computer technician, made sure that the computer animation software was uploaded on all the functional computers in the computer laboratory and everything required for the teaching of the two treatments in the classroom and computer laboratory was intact. Then the teacher taught the FPIC group, followed by the FPICM group, per the school’s normal timetable. The FPIC group was taught in the normal classroom, while the FPICM group was taught in the school’s computer laboratory. The movement of the two groups was controlled to prevent them from interacting. The teacher delivered the two treatments systematically as designed and instructed, and the teachings were supervised by the principal researcher (expert instructional designer). Each teaching took 75 minutes, as has already been indicated. The posttest administration occurred (third session) in the same rooms where the pretest was administered; it took 45 minutes as the pretest. Scripts for the pre-and post- tests and the marking scheme were submitted to an independent subject matter expert for marking. The teacher remarked on them, and the discrepancies were discussed with the principal researcher.
2.5. Data analysis
Descriptive statistics, paired-sample t-test, independent sample t-test and univariate analysis were used to analyze the data.
3. Results
The dependent variable was the students’ performance on the two treatment conditions or the learning gains (students’ posttest scores minus students pretest scores). The independent variable was the two treatment conditions.
3.1. Students’ performance on pretest
The independent-sample t-test involving the pretest scores showed no statistically significant difference between the two groups of students t(86) = 1.177, p = . 243. highlights an overview of the mean scores on the pretest.
Table 2. Overview of mean scores on the pretest
3. 2. Students’performance on the pretest and posttest
A paired-sample t-test showed a statistically significant difference between students’ performance in the pre-test and posttest t (83) = −11.55, p = .000, eta2 = 0.88. This means that the two groups’ performance in the post-test was better than the performance of the two groups in the pretest. depicted the mean and standard deviation scores for the pretest and posttest.
Table 3. Overview of mean scores for pretest and posttest
3.3. Learning gains between the two treatment groups
An independent−samples t-test conducted for the learning gains revealed no statistically significant difference between the two treatment groups in terms of their learning gains t ((82) = −1.232, p = .221). The FPI with chart group and FPI with computer animation group equally performed better. highlights the results.
Table 4. Overview of mean scores of learning gains between the two groups
3.4. Gender effects on the two treatment groups for learning gains
Univariate analysis conducted revealed no statistically significant effects of gender (male and female) on the two treatments (FPIC and FPICA) to develop expertise in science at the basic school. [F (1, 80) = .886, p = .350]. There is an interaction effect but not significant. and highlight the results.
Table 5. Interaction effect of media and gender on FPI for learning gains
4. Discussion
The purpose of the study was to investigate: 1) the effect of learning environments designed in line with FPI with computer animation (high cost and dynamic) and chart (low cost and static) instructional media for the acquisition of expertise in science in the traditional classroom of basic school, and 2) the effect of gender on learning environment designed in line with FPI with computer animation and chart for development of expertise in science in the traditional classroom of basic school. The outcome of the study supports the hypothesis that in the context of well designed: 1) computer animation and 1) chart in line with principles of multimedia learning and FPI, both computer animation and chart can equally and effectively facilitate the development of expertise in science in the green context of the traditional classroom of basic school. This finding demonstrates that students inFPI with computer animation (dynamic media) learning environment and students inFPI with charts (static media) learning environments performed equally better in the acquisition of knowledge, understanding, and problem-solving skills in diffusion and osmosis in the traditional classroom of basic school. The result of the study shows that in well-designed learning environments in line with FPI, both animation (dynamic media) and chart (static media), if also well designed, equally stimulate the learners’ learning processes to construct an adequate mental model for the information, and apply it successfully in new situations. This finding contradicts the findings of Rusli and Negara (Citation2017) and Altipormark (Citation2014) who indicated that computer animation facilitates learning better than static graphic. It is important to note that, to some great extent, the participants of those studies were tertiary education students, while the participants of the present study were basic school students. However, the finding of this study supports research findings of Sarfo et al., (Citation2014) and Sarfo and Elen (Citation2007) as well as Smaldino, Lowther and Russell (Citation2008) that small and inexpensive media (e.g., chart, flashcard) if properly designed and used in the context of effective principles of instruction (e.g., FPI, 4 C/ID model) can make teaching and instruction as effective as expensive media (e.g., computer). The result of the study, further supports the finding of Clark (Citation2001) that instructional method activates, compensates or supplants the cognitive processes necessary for learning, and not computer animation or chart. The present study adds new insights to the effectiveness of FPI by extending its potential to teaching and learning of science at the basic level of education (in developing countries).
It seems from the present finding that the learners in the FPI with computer animation learning environment were fascinated with the animation and therefore concentrated more on the beauty and wonder of the animation instead of the understanding of the process of diffusion and osmosis depicted by the computer animation and also explained by the teacher. In contrast, the learners in the FPI with chart might not realize any beauty and wonder with the chart and therefore had to focus on the understanding of the process of diffusion and osmosis as depicted by the chart and also explained by the teacher. Then the question generated from the above is: Do the learners focus on the beauty and wonders of the emerging technologies or the understanding of the content that the emerging technologies depict? A further research study is required to investigate this.
Moreover, the study found no significant interaction effect of gender on the learning environment designed in line with FPI with computer animation and chart for development of expertise in science in the traditional classroom of the basic school. This shows that gender does not affecta properly designed FPI learning environment integrated with computer animation (dynamic media) or chart (static media) to acquire knowledge, understanding and problem-solving skills in science in the green context of the traditional classroom of basic school. This finding partially does not support the position of Wu and Anderson (Citation2015) that effective education in STEM subjects for boys and girls should critically consider computer simulation or animation instruction. Rather, the finding partially adds novel and more understanding to Wu and Anderson by clarifying and specifying the kind of intervention relevant for successful instruction of STEM subjects for girls and boys at the basic school. Even though the finding expands and adds new insights to the effectiveness of FPI by Merrill (Citation2012), it is important to emphasize that the generalisability power of the present study is limited to “Science” (S in STEM). Further research is suggested to investigate the gender effect on the two innovative interventions for developing expertise in the remaining three subjects,”Technology, Engineering and Mathematics” (TEM in STEM). In addition, since the present finding in connection with gender, computer animation and chart, FPI, and learning of science is a novelty, further research is suggested to validate the current finding.
5. Conclusion and recommendations
What is of critical importance, especially in the context of financial constraints and learning environments with limited ICT resources, is the appropriate, effective and efficient solution to learning problems in science in the classrooms of basic schools. The results of the present study provided sound empirical evidence that expensive dynamic instructional media (e.g., computer animation) and inexpensive static instructional media (e.g., chart), when expertly designed in line with PML and integrated into properly designed FPI,will equally, effectively and efficiently provide the solution to learning problems in science,especially at the basic level. This suggests that the kind of instructional media (dynamic, static) and gender (male, female) do not influence the instructional potentials of carefully and adequately designed learning environments in line with FPI to develop expertise in science at the basic level. These results, together with Clark (Citation1994, Citation2001); Sarfo et al., (Citation2014); Sarfo and Elen (Citation2007); Smaldino et al., (Citation2008) and many others strongly suggest that expensive instructional media, such as a computer, is not a guarantee for or solution to, achieving quality education (quality teaching and learning) in the traditional classrooms of 21st century. It is just a tool (see Sarfo et al. , Citation2014), like low cost and static instructional media such as a chart, to aid the instructional strategy’s delivery. The pedagogy or effective design of learning tasks based on real principles of instruction such as FPI and 4C/ID matters, not the kind of delivery system or gender. The findings also confirm and re-echo the position of Sarfo et al., (Citation2014) that in the context of constraints in hard technology, soft technology together with innovative instructional strategies based on effective principles of instruction can achieve learning results (e.g., in science at the basic level of education, building design at the secondary technical education) as effectively as instructional strategies integrated with hard technology. This is an excellent message to countries such as Ghana, Nigeria, Tanzania and others with financial constraints and limited ICT resources. In the context of financial constraints, let us spend and utilize the little we have to train teachers/instructors to acquire instructional design expertise rather than spending it on expensive instructional media to promote quality classroom teaching in schools.
Based on the findings and conclusions, the following recommendations are made. First, FPI learning environment should be adopted by the National Council for Curriculum and Assessment (NaCCA) as one of the instructional interventions for teaching STEM subjects that require complex skills. Second, scientific problems or questions posed by teachers and examiners should reflect real-life experiences so that students will not find it difficult to transfer what is learnt from the classroom to a real-life setting. Third, the Ghana Education Service should provide training on applying FPI learning environment to basic school teachers so that this instructional model could be effectively used in all Ghanaian JHS classrooms to realise its full potential on students’ performance. For future research, it is suggested that a similar study should be conducted within the instructional framework of FPI with computer animation and chart as there was no control group in this study. It is, therefore, envisioned that the recommendations and conclusions reached from this study will provide valuable information and insights to improve students’ low performance in science at the basic level of education.
Disclosure statement
No potential conflict of interest was reported by the author(s).
Additional information
Funding
Notes on contributors
Francis Amankwah
Frederick Kwaku Sarfo (PhD) is a Professor of Instructional Technology and Vice-Chancellor of AAMUSTED, Ghana. His research interest is focused on: Integration of ICT into education, Instructional design for learning, Designing powerful (innovative) learning environments for the development of technical and vocational expertise, and curriculum development
Patrick Debrah is a professional science teacher in Ghana. He received his bachelor’s and master’s degrees from University of Education, Winneba (UEW), Ghana. His research interests include the use of technologies in science education, science pedagogies and science curriculum.
Francis Amankwah is a doctoral student and lecturer at AAMUSTED, Ghana. He received his master’s degrees in Computer Education and Technology and Educational Leadership from Ohio University and UEW, respectively. His research interests include media use in education, teachers’ efficacies and ICT curriculum.
Prof. Francis Owusu-Mensah (PhD) is an Associate Professor in distance education. Currently, he is Ag. Principal of CoDeL, UEW. His research focuses on open and distance education, educational psychology and curriculum development.
References
- Ainsworth, S., & VanLabeke, N. (2008). Multiple forms of dynamic representation. Learning and Instruction, 14(3), 241–23. https://doi.org/10.1016/j.learninstruc.2004.06.002
- Ajayi, V. O., & Ogbeba, J. (2017). Effect of gender on senior secondary chemistry students’ achievement in stoichiometry using hands-on activities. American Journal of Educational Research, 5(8), 839–842. https://doi.org/10.12691/education-5-8-1
- Altipormark, K. (2014). Impact of computer animations in cognitive learning: Differentiation. International Journal of Mathematical Education in Science and Technology, 4 (8), 1146–1166. Retrieved May 4, 2018, from https://doi.org/10.1080/0020739x.2014.914256
- Anderson, G., Tredway, C., & Calice, C. (2015). A longitudinal study of nursing students’ perceptions of online course quality. Journal of Interactive Learning Research, 26(1), 5–21.
- Badali, M., Derakhshi, S. A., Bagheri, P., & Ansari, M. R. (2016). The effect of team-based learning coupled with Merrill’s first principles of instruction on the achievement of learning objectives in nursing students. Interdisciplinary Journal of Virtual Learning Medical Sciences, 7(4), 1–6. https://doi.org/10.5812/ijvlms.10812
- Buabeng, I., Acheaw, K. O., & Danso, F. N. (2014). TIMSS 2011 Science assessment result: An overview of Ghana’s performance. Journal of Curriculum and Teaching, 3(2), 1- 12. Retrieved April 3, 2018, from http://www.sceied.ca./journal/index/php/jct doi:10.5430/jct.v3n2p1
- Clark, R. C., & Mayer, R. E. (2003). E-learning and the science of instruction. Jossey-Bass.
- Clark, R. E. (1994). Media will never influence learning. Educational Technology Research and Development, 42(2), 21–29. https://doi.org/10.1007/BF02299088
- Clark, R. E. (2001). What is next in the media and methods debate? In R. E. Clark (Ed.), Learning from media: Argument, analysis, and evidence (pp. 327–337). Information Age Publishing.
- Cohen, L., Manion, L., & Morrison, K. (2018). Research methods in education (8th ed.). Taylor and Francis.
- Coughlan, S. (2015). Asia tops biggest global school rankings. Retrieved May 2, 2018, from http://www.bbc.com/news/business-32608772
- Cunningham, C. (2007). Implications of Recent Contributions to Research on K-12 Engineering and Technology Education on STEM Education. Conference Proceedings, 2007 DR-K12 PI Meeting. September 2007, Arlington, VA.
- Dyel, B. D. (2011). Effect of cooperative learning strategy on academic performance and attitude of basic science students in large classes [ Unpublished M.Ed Thesis]. Ahmadu Bello University.
- Dziedzic, K., Barszcz, M., Paśnikowska-Łukaszuk, M., & Jankowska, J. (2015). The role of computer animation in teaching technical subjects. Advances in Science and Technology Research Journal, 9(28), 134–138. https://doi.org/10.12913/22998624/60801
- Emamiyan-Kheshti, M., Ghasemi, M., Mehraji, N., Kazem, K., Banihashelu, K., &Badali, M. (2016). Effects of blending Merrill's basic principles of instruction and team-based learning in retention and application in medicine students. Medicine Education, 1, 62–71.
- Filgona, J., & Sababa, L. S. (2017). Effect of gender on senior secondary school students’ academic achievement in geography in ganye educational Zone, Nigeria. European Journal of Education Studies, 3(4), 394–410. doi:10.13140/RG.2.2.31068.33921
- Frick, T., Chadha, R., Watson, C., Wang, Y., & Green, P. (2007). Theory-based course evaluation: Nine scales for measuring teaching and learning quality. Retrieved June 5, 2017, from http://www.indiana.edu/~tedfrick/TALQ.pdf
- Gambani, A. I., Falode, C. O., & Adegbenro, D. A. (2014). Effectiveness of computer animation and geometrical instructional model on mathematics achievement and retention among. JSS studentsEuropean Journal of Science andMathematicsEducation, 2(2), 127–146.
- Gardner, J. (2011). Testing the efficacy of Merrill’s first principles of instruction in improving student performance in introductory biology courses.[ Doctoral dissertation, Utah State University]. Retrieved June 6, 2017 from http://digital-commons.usu
- Gardner, J., Barclay, M., Kong, Y., & LeVally, C. (2019). Designing an accelerated graduate evaluation course using the first principles of instruction and interactive media. Journal of Educational Technology Systems, 1–25. https://doi.org/10.1177/0047239519893049
- Gardner, J., & Belland, B. R. (2017). Problem-centred supplementary instruction in biology: Influence on content recall, content understanding and problem-solving ability. Journal of Science Education and Technology, 26(4), 383–393. https://doi.org/10.1007/s10956-017-9686-0
- Gipps, K. S. (2004). Gender inequality. In H. Therney (Ed.), Women’s studies encyclopedia. Peter Bedrick Books. (pp. 36-49) .
- Greefield, T. A. (1997). Gender-and grade-level differences in science interest and participation. Science Education, 81 (3), 259–276. Retrieved December 20, 2018, from. https://doi.org/10.1002/(SICI)1098-237X(199706)81:3≤259::AID-SCE1≥3.0.CO;2-C
- Jacob, A. H. (2018). Flipping with the first principles of instruction: An examination of preservice teachers’ technology integration development. Journal of Digital Learning in Teacher Education, 34(4), 201–218. https://doi.org/10.1080/21532974.2018.1494520
- Joiner, R., Iacovides, J., Owen, M., Gavin, C., Clibbery, S., Darling, J., & Drew, B. (2011). Digital games, gender and learning in engineering: Do females benefit as much as males? Journal ofScience Educational Technology, 20, 178–185.
- Kahveci, M. (2010). Student’s perceptions to use technology for learning: Measurement integrity of the modified fennema-sherman attitudes scales. The Turkish Online Journal of EducationalTechnology, 9(1), 185–201.
- Kelley, T. R., & Knowles, G. (2016). A conceptual framework for integrated STEM education. International Journal of STEM Education, 3(11), 1–11. https://doi.org/10.1186/s40594-016-0046-z
- King’aru, J. M. (2014). Factors contributing to poor performance in science subjects: A case of secondary schools in KAWE Division A dissertation submitted in partial fulfillment of the requirements for the degree of master of project management of the Open University of Tanzania.
- Klein, J. D., & Mendenhall, A. (2018). Applying the first principles of instruction in a short-term, high volume rapid production of online professional development modules. Journal of Computing in Higher Education, 30(1), 93–110. https://doi.org/10.1007/s12528-017-9166-9
- Kola, A. J., & Taiwo, A. K. (2013). Analysis of gender performance in physics in Colleges of Education, Nigeria. Journal of Education and Practices, 4(6), 1–5.
- Krathwohl, D. R. (1995). Methods of educational and social sciences research: An integrated approach. Longman.
- Kuba, R., Rahimi, S., Smith, G., Shute, U., & Dai, C. P. (2021). Using the first principles of instruction and multimedia learning principes of design and develop in-game learning videos. Educational Technology Resercah and Development, 69(2), 1201–1220. https://doi.org/10.1007/s11423-021-09994-3
- Majo, S. (2016). Factors influencing poor performance in science subjects in secondary schools in Shinyanga Municipality GRIN Verlag. Retrieved May 3, 2018, from https://www.grin.com/document/383487
- Mariscal, J., Mayne, G., Aneja, U., & Sorgner, A. (2019). Bridging the gender digital gap. Economics: The Open-Assessment E-Journal, 13(1), 1–12. https://doi.org/10.5018/economics-ejournal.ja.2019-9
- Mayer, R. E. (2002). Multimedia learning. Psychology of Learning and Motivation, 41, 85–139.
- Mayer, R. E. (2008). Applying the science of learning: Evidence-based principles for the design of multimedia instruction. American Psychologist, 63(8), 760–769. https://doi.org/10.1037/0003-066X.63.8.760
- Mendenhall, A. M. (2012). Examining the use of first principles of instruction by instructional designers in a short-term, high volume, rapid, production of online K-12 teacher professional development modules. Unpublished Doctoral Dissertation, Florida State University.
- Merrill, M. D. (2002). A pebble in the pond model for instructional design. Performance Improvement, 41(7), 41–46. https://doi.org/10.1002/pfi.4140410709
- Merrill, M. D. (2004). Firstprinciples of instruction. Educational Technology Research and Development, 50(3), 43–59. https://doi.org/10.1007/BF02505024
- Merrill, M. D. (2012). First principles of instruction. Pfeiffer.
- Merrill, M. D. (2009). First principles of instruction. In C. M. Reigeluth, and A. Carr (Eds.), Instructionaldesign theories and models: Building a common knowledge base. (Vol. III). Routledge Publishers. 1–26 .
- Musa, C. D., & Samuel, R. I. (2019). Influence of gender and school location on science and mathematics students’ achievement in Western Senatorial District of Nasarawa State, Nigeria. East African Scholars Multidisciplinary Bulletin, 2(3), 259–264.
- National Academy of Sciences. (1998). Teaching about evolution and the nature of science. The National Academies Press.
- Obadiaru, I. (2020). Trends in academic achievement of senior secondary school students in computer studies in Rivers State. Journal of the Nigerian Academy of Education, 16(2), 281–293.
- Potvin, P., & Hasni, A. (2014). Interest, motivation and attitude towards science and technology at K-12 levels: A systematic review of 12 years of educational research. Studies in Science Education, 50(1), 85–129. https://doi.org/10.1080/03057267.2014.881626
- Rashid, A. T. (2016). Digital inclusion and social inequality: Gender differences in ICT access and use in five developing countries. Gender, Technology and Development, 20(3), 306–332. https://doi.org/10.1177/0971852416660651
- Rusli, M., & Negara, I. (2017). The effect of animation in multimedia computer-based learning and learning style to the learning results. Turkish Online Journal of Distance Education, 18(4), 177–190.
- Sarfo, F. K., Amankwah, F., & Konin, D. (2017). Computer self-efficacy among senior high school teachers in Ghana and functionality of demographic variables on their computer self-efficacy. The Turkish Online Journal of Educational Technology, 16(1), 19–31.
- F. K., & Elen, J. (2007). Developing technical expertise in secondary technical schools: The effect of 4C/ID learning environments. Learning Environment Resource, 10(3) ,207–221. https://doi.org/10.1007/s10984-007-9031-2
- Sarfo, F. K., & Elen, J. (2007). Developing technical expertise in secondary technical schools: The effect of 4C/ID learning environments. Learning Environments Research, 10(3), 207–221. .https://doi.org/10.1007/s10984-007-9031-2
- Sarfo, F. K., Eshun, G., Elen, J., & Adentwi, K. I. (2014). Towards the solution of the abysmal performance in mathematics in junior high schools: Comparing the pedagogical potential of two designed interventions. Electronic Journal of Research in Educational Psychology, 12(3), 763–784. https://doi.org/10.25115/ejrep.34.14028
- Smaldino, S. E., Lowther, D. L., & Russell, J. D. (2008). Instructional technology and media for learning (9th ed.). Pearson/Merrill/Prentice Hall.
- Soyibo, K. (2009). Gender differences in Caribean students’ performance on a test of errors in biology lebelling. Research in Science and Technological Education, 17(1), 75–82. https://doi.org/10.1080/0263514990170106
- Stoet, G., & Geary, D. C. (2018). The gender-equality paradox in ccience, technology, engineering, and mathematics education. Psychological Science, 29(4), 581–593. https://doi.org/10.1177/0956797617741719
- Tile, M. T. (2013). Effect of activity-based on psychomotor skills acquisition and interest of senior secondary two in Biology [ Unpublished M.Ed Dissertation, Benue State University].
- Truong, M. T., Elen, J., & Clarebout, G. (2019). Implementing Merrill’s first principles of instruction: Practice and identification. Journal of Educational and Instructional Studies in the World, 9(2), 14–28.
- UNESCO. (2017). Girls’ and women’s education in science, technology,engineering and mathematics.
- van Merrienboer, J. J. G., & Kirschner, P. A. (2007). Ten steps to complex learning. Erlbaum.
- van Merriënboer, J. J. G. (1997). Training complex cognitive skills: A four-component instructional design model for technical training. Educational TechnologyPublications.
- West Africa Examination Council. (2018). Basic education certificate examination: Chief examiner’s report. WAEC
- West Africa Examination Council. (2019). Basic education certificate examination.Chief examiner’s report. WAEC
- Wilujeng, H., Kusumah, Y. S., & Darhim, D. (2019). The students’ achievement of algebraic thinking using Merrill’s first principles of instruction. Journal of Physics: Conference Series, 1188, 1–8. https://doi.org/10.1088/1742-6596/1188/1/012039
- Wu, Y. T., & Anderson, O. R. (2015). Technology-enhanced Stem (science, technology, engineering and mathematics education). Journal of Computers in Education, 2 (3), 245–249. Retrieved June 7, 2017, from. https://doi.org/10.1007/s40692-015-0041-2
- Yorganci, S. (2020). Integrating flipped learning approach of first principles of instruction in mathematics courses. Journal of Computer Assisted Learning, 36(5), 763–776. https://doi.org/10.1111/jcal.12448
- Zarei, Z. E., Badali, M., & Amir, T. M. H. (2014). Investigating the efficacy of Merrill’s first principles of instruction on students’ learning and retention. New Thoughts on Education, 9(4), 57–75. https://doi.org/10.22051/jontoe.2014.355.