Abstract
How are the domains of space and time related? One approach, A Theory of Magnitude (ATOM), proposes an undifferentiated system of magnitude representation in the brain, predicting that space and time are symmetrically related, while Conceptual Metaphor Theory (CMT) proposes that we represent time using spatial metaphors, predicting asymmetrical interactions between domains. Tau and Kappa effects are perceptual phenomena that arise when observers judge the distance/duration between consecutive stimuli in sequence: Timing affects the perception of space (Tau) and spacing affects the perception of time (Kappa). Interference bidirectionality has been taken as evidence for ATOM. CMT proponents argue that interference may result from the perceiver imputing velocity to the stimuli. Here, the Tau and Kappa paradigm was modified to reduce the illusion of imputed velocity by manipulating stimuli parameters. In favor of CMT, we found that when the illusion of imputed velocity is reduced, asymmetrical interference arises: the Tau effect is eliminated while the Kappa effect remains intact.
PUBLIC INTEREST STATEMENT
How are space and time related in our mind/brain? Two theories with opposite predictions have motivated a discussion around the nature of this relationship. According to one theory, space, time and quantity are represented by a common analog magnitude system. According to the other, time is an abstract concept that is not directly grounded on our physical experience, so we borrow spatial representations to think about it. Here we replicate a classic experiment where participants are asked to compare the duration/distance of two spatiotemporal intervals defined by three horizontally aligned lights that flash in succession. The original experiment was modified to pitch the predictions of the two theories against each other. The results are consistent with the view that the concept of time may be constructed in our minds through the metaphorization of the space domain.
Competing Interest
The authors report no conflicts of interest. The authors alone are responsible for the content and writing of the paper.
1. Introduction
A bulk of work has shown that the concepts of space and time are related in the mind/brain. Two theories with comparatively opposite predictions have motivated a discussion around the nature of this relationship: A Theory of Magnitudes (ATOM; Walsh, Citation2003) and the Conceptual Metaphor Theory (CMT; Casasanto, Citation2010; Lakoff & Johnson, Citation1990).
According to ATOM, space, time and quantity are represented by a common analog magnitude system. In other words, a specific module in the brain is proposed, where time, space, and other domains are processed as a common magnitude or neural metric. Numerous experiments with adults and children show cross-dimensional interference between domains such as space, time and quantity (Dehaene, Bossini, & Giraux, Citation1993; de Hevia & Spelke, Citation2010; Dormal & Pesenti, Citation2007; Henik & Tzelgov, Citation1982; Piaget, Citation1927/1969, Citation1941/1952, Citation1948/1956; Srinivasan & Carey, Citation2010; Xuan, Zhang, He, & Chen, Citation2007). Additional evidence for shared representation comes from neuroimaging studies showing an overlapping activity of some areas in the parietal lobe in response to the processing of different domains (Bueti & Walsh, Citation2009; Walsh, Citation2003).
Although bidirectional interference is expected under both approaches, ATOM´s and CMT´s predictions differ in whether space/time interference should be symmetric (ATOM) vs. asymmetric (CMT). If a common and undifferentiated magnitude system is in place, a symmetrical interference between space and time processing is expected: the amount of spatial interference on temporal judgments should be comparable to the amount of temporal interference on spatial judgments. According to CMT, abstract concepts result from metaphorization of more concrete concepts. Time is an abstract concept that is not directly grounded on our physical experience, so we borrow spatial representations to think about it. So, contrary to ATOM, what CMT predicts is that representations of time depend asymmetrically on representations of space (Lakoff & Johnson, Citation1999).
Some evidence for asymmetry comes from language use. Across cultures, people use spatial metaphors to describe time more frequently than temporal metaphors to describe space. The TIME IS SPACE (linguistic) metaphor has been found in languages as diverse as English, Mandarin Chinese, Hindi and Sesotho (Altverson, Citation1994). More recently, the asymmetrical dependence of time on space has been shown using experimental designs. Boroditsky (Citation2000) used linguistic temporal and spatial priming to study the cross-dimensional interference. Consistent with the CMT, the data showed that spatial primes affected the way people thought about time, but temporal primes had no influence over spatial thinking. Similarly, Casasanto and Boroditsky (Citation2008) performed a series of psychophysical tasks, which did not require the use of language, showing that the spatial stimuli interfered significantly with the temporal judgments, while the temporal stimuli had no effect on the spatial judgments.
Other studies (Casasanto, Citation2010; Casasanto, Fotakopoulou, & Boroditsky, Citation2010) also found the space-time asymmetric dependence in kindergartners and schoolchildren. These results suggest that the asymmetry appears in an early stage of children development and that its cause is prior to language acquisition. Further evidence in favor of asymmetry comes from neuroimaging data. Gijssels, Bottini, Rueschemeyer, and Casasanto (Citation2013) found asymmetric activation of the inferior parietal lobe while the participants were making spatial and temporal judgments on the same visual stimuli.
1.1. Tau and Kappa effects: why are they relevant?
Since symmetry of interference could be hard to demonstrate experimentally, bidirectional interference has been often taken as evidence in favor of ATOM (e.g. Lourenco & Longo, Citation2011). An example of bidirectional interference are the Tau and Kappa effects (Benussi, Citation1913; Bill & Teft, Citation1969; Cohen, Hansel, & Sylvester, Citation1953; Collyer, Citation1977; Helson, Citation1930; Jones & Huang, Citation1982; Price-Williams, Citation1954; Sarrazin, Giraudo, Pailhous, & Bootsma, Citation2004). In a classic experiment, participants are asked to compare the duration/distance of two spatiotemporal intervals defined by three horizontally aligned light bulbs that flash in succession. When participants are asked to compare the duration of both temporal intervals, the temporal judgments increase in function of the spatial separation of the light bulbs (Kappa effect). In a similar way, when they are asked to compare the distance between the light bulbs, the spatial judgments increase as a function of the temporal duration between light bulbs (Tau effect; e.g. Jones & Huang, Citation1982; Sarrazin et al., Citation2004).
Tau and Kappa effects have been found across many domains including spatial modalities, vision, hearing and touch (see Jones & Huang, Citation1982). Also these effects have been shown using different experimental paradigms including categorical judgments of two spatiotemporal intervals (Abe, Citation1935; Benussi, Citation1913; Helson & King, Citation1931; Jones & Huang, Citation1982) and reproduction tasks (e.g. Sarrazin et al., Citation2004).
The effect of time on space (Tau effect) has been interpreted as evidence against CMT (Lourenco & Longo, Citation2011). But asymmetry and bidirectional interference are not mutually exclusive, and bidirectionality is not ruled out by CMT. As a matter of the fact, “effects of time on space are not at odds with CMT so long as (under adequately controlled conditions) a greater or more frequent effect of space on time is also found” (Bottini & Casasanto, Citation2013, p. 6).
Moreover, proponents of CMT have argued that Tau and Kappa effects might be mediated by other factors besides domain interference. Specifically, Tau and Kappa effects might be mediated by participants computing movement (with a certain velocity) to the flashing light (Casasanto & Boroditsky, Citation2008; see also Bottini & Casasanto, Citation2013). According to the Imputed Velocity Hypothesis (Jones & Huang, Citation1982; Price-Williams, Citation1954) subjects tend to impute uniform motion to discontinuous displays. For example, Jones and Huang (Citation1982) proposed that if participants assumed a constant velocity of motion, they would ascribe a greater duration to the two points that are further apart spatially, and conversely, a greater spatial separation between the two points that are temporally farther apart.
Would Tau and Kappa effects remain when imputed velocity is reduced or factored out? This is the question that motivates this study. CMT advocates (e.g. Casasanto & Boroditsky, Citation2008) have proposed that when participants impute velocity to the flashing dots, symmetrical time-space interference may arise. This would explain classical Tau and Kappa effects. However, under conditions where motion and velocity imputation is suppressed, then ATOM and CMT predictions could be pitched against each other. Symmetrical interference would still be expected according to ATOM, but according to CMT, asymmetrical interference between time and space domains should emerge.
Then, if ATOM is right, then a comparable (symmetric) Tau and Kappa effects should be observed under conditions of suppressed imputed velocity. If, on the other hand, CMT is right, the reduction of imputed velocity would reveal the asymmetric dependence of time on space. In other words, Kappa effect (space-on-time interference) should be greater than the Tau effect (time-on-space interference).
1.2. Manipulation of imputed velocity
Anstis and Ramachandran (Citation1987) have explored the conditions under which the human perceptual system incurs in the processes of imputing velocity of discontinuous displays. Their experiment consisted of successive lightning of light bulbs (flashes). They found that the imputation of velocity (what they call visual inertia) gets significantly reduced by sudden changes of direction of the sequential lightening and that the imputation of velocity vanishes when the light bulbs do not flash and stay on (static) and always visible.
This article recreates the original Tau and Kappa experimental design while manipulating the effect of imputed velocity. To do so, three horizontally aligned flashes were displayed on a computer screen. We manipulate whether the circles (bulbs in the original study) remain static or, as in typical paradigms, they go off as in flashes. Following Anstis and Ramachandran (Citation1987) we assume that when the three consecutive circles do not flash (that is, they stay static), the imputed velocity will be reduced and the interference between space and time domains will be fleshed out.
1.3. Interval comparison experiment
The experiment was intended as a computerized variation of the classic Kappa and Tau experiment in one of its simplest version: three horizontally arranged sequential flashes define two spatial and two temporal intervals (e.g. Abe, Citation1935; Benussi, Citation1913; Helson & King, Citation1931; Jones & Huang, Citation1982). In the current version, flashes are instantiated by three white circles in a dark computer screen. We carried out a three-factor (2 × 3 × 2) design (type of response [space-judgments/time-judgments] × configuration of the spatiotemporal intervals [CC (space constant and time constant)/CV (space constant and time variable)/VC (space variable and time constant)] × lighting pattern [flashing circles/static circles]). The first two factors were varied within subjects, while the third one (Lighting Pattern), was varied between subjects. The assumption of the study is that sequentially flashing circles admit imputed velocity. However, imputed velocity is diminished when sequential circles remain static in the display.
2. Method
2.1. Participants
Fifty-six participants from the community of Universidad de Los Andes volunteered to participate (8) or participated in exchange of extra-credit for Psychology classes (48). Age range varied from 18 to 52 years; 14.3% (8) were male and 85.7% (48) were female.
2.2. Materials and procedure
The stimulus consisted of three horizontally aligned white circles, which appeared in succession on a black background in a computer screen. Each white circle had a 4 mm diameter. The whole configuration was 10 cm long (the three circles sequence display) and the sequence lasted 1000 ms in total. The displayed was centered on a 14´ PC monitor. The circles flashed from the left to the right, creating a one-dimensional spatial configuration, which consisted of two spatiotemporal intervals of distance length 1 and length 2 (henceforth L1 and L2), and duration time 1 and time 2 (henceforth T1 and T2). L1 and T1 were defined by the sequence of the first and the second circles, and L2 and T2 were defined by the sequence of the second and third circles. Each participant was exposed to 72 displays of the stimuli (trials) divided into two type-of-response blocks: the temporal judgment block (36 trials) and the spatial judgment block (36 trials). The order of presentation of the two blocks was randomized and counterbalanced across subjects.
Instructions were given in participants´ native language, Spanish. At the end of each trial, participants were asked to compare the spatial or temporal length depending on Type-of-Response block. In the temporal-judgment condition, the participants´ task was to judge whether T2 was equal to, greater than, or less than T1. In the spatial-judgment condition, the task was to judge whether L2 was equal to, greater than, or less than L1. They were indicated to press the key “First” (in Spanish “Primero”, labeled on a sticker on the key 1 in the keyboard) or “Second” (in Spanish “Segundo”, labeled on a sticker on the key 0 in the keyboard) to indicate first or second interval. The time lapse between each response and the following trial was 1500 ms, and during this lapse, the screen remained black.
The factor Configuration-of-Interval refers to the arrangements of longitude and duration of the intervals. A block contained 12 trials of each of the three possible configurations. In the CC (Constant-Constant) condition, the duration and the length of the two spatiotemporal intervals were equal in length (L1 = L2 = 5 cm) and duration between circles (T1 = T2 = 500 ms). In the CV (Constant-Variable) condition, the length of the intervals remained constant (L1 = L2 = 5 cm), but the duration of the intervals varied. In half of the CV trials in a block (6) the first temporal interval lasted longer than the second one (T1 = 600 ms and T2 = 400 ms) and in the other half of the trials the second interval lasted longer (T1 = 400 ms and T2 = 600 ms) than the first one. Finally, in the third condition VC (Variable-Constant), the duration of the two intervals remained constant (T1 = T2 = 500 ms) and the length varied. In half the trials in each block (6) the first spatial interval was longer than the second (L1 = 6 cm, L2 = 4 cm), and in the other half, the second interval was longer (L1 = 4 cm and L2 = 6 cm) than the first. The order of Configuration-of-Intervals (CC, CV, and VC) was randomized within each block. Display configurations of conditions are shown in Figure .
Figure 1. A schematic outline of the three display conditions. The upper panel corresponds to the CC (Constant-Constant) condition (L1 = L2 = 5 cm and T1 = T2 = 500 ms). The middle panel represents the CV (Constant-Variable) condition, where the length of the intervals remained constant (L1 = L2 = 5 cm), but the duration of the intervals varied (T1 = 600 ms/T2 = 400 ms, and T1 = 400 ms/T2 = 600 ms). The lower panel represents the VC (Variable-Constant) condition, where the interval remained constant (500 ms), but the length varied (L1 = 4 cm/L2 = 6 cm and L1 = 6 cm/L2 = 4 cm)
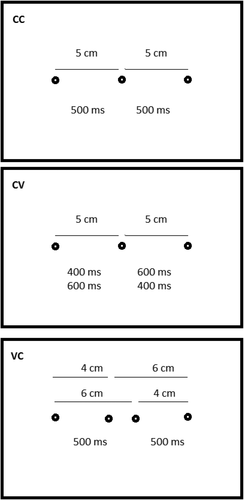
The range of L1 and L2 (4, 5, and 6 cm) and T1 and T2 (400, 500, and 600 ms) was based on previous studies (see Jones & Huang, Citation1982 for a review). For example, Price-Williams (Citation1954) argued that the Kappa effect is found when T1 and T2 range between 600 ms and 6000 ms. Jones and Huang (Citation1982), reported an optimum T1+T2 between 160 and 1500 ms. Abe (Citation1935) used three flashes similar to the current study. The two spatial intervals varied between 3 and 5 cm.
The third factor, the Lightening-Pattern refers to the way dots appeared on the display. In the Flashing condition, the dots flashed and went off in succession as in the classical Tau and Kappa paradigm. Circles appeared one by one and stayed visible for 100 ms. In the Static condition, dots remained on after appearing on the screen for the remaining of the trial, after which the three circles went off and the response was elicited from the participant while the screen remained black. Half of the participants (n = 28) were assigned to the Flashing condition and the other half were assigned to the Static condition.
The experimental stage was preceded by a familiarization stage in which participants learned the task and became familiarized with the interface. The participants were able to start the experimental stage after succeeding in four practice comparisons (two temporal and two spatial judgment responses). Participants were seated at a desk with a laptop computer in front of them. The experiment was conducted using E-prime experimental software package (2.0) (Schneider, Eschman, & Zuccolotto, Citation2002) on a PC.
Procedures followed were in accordance with the ethical standards of the responsible committee on human experimentation (institutional and national) and with the Helsinki Declaration of 1975, as revised in 2000.
3. Results
Table shows the proportion of responses “first interval longer” (in duration or distance). This measure was used following the original study of Jones and Huang (Citation1982). In the flashing condition, rows and columns decrease monotonically for both spatial and temporal judgments. This suggests that in the flashing condition, space context interferes with temporal judgments and that temporal context interferes with spatial judgments, consistent with typical Tau and Kappa effects previously described in the literature. However, when circles are static and imputation of velocity is presumably reduced, the data suggests space-on-time interference (Kappa effect) remains while time-on-space interference (Tau effect) vanishes. Next, we describe the results in more detail, first for the case of temporal judgments and next for spatial judgments.
Table 1. Proportion of responses “First interval longer”
3.1. Kappa effect
Temporal judgments Participants were instructed to select the interval that was longer in time—either the first or the second. The frequency with which they selected the intervals varied depending on the space/time configuration. In the CC condition (T1 = T2 = 500 ms and L1 = L2 = 5 cm) the results were the following: participants exposed to flashing circles, in average, selected the first interval 6.8 (SD = 2.4) out of 12 times (56%), while participants exposed to static circles selected the first interval 6.3 (SD = 2.7) out of 12 times (52.3%). Interval selection in the CC condition did not differ significantly from chance in neither group of participants (p’s > .05). In the CV condition (T1/T2 = 600 ms/400 ms and T1/T2 = 400/600 ms; L1 = L2 = 5 cm), in average, participants correctly selected more the longer lasting interval most of the time: participants exposed to flashing circles, selected the 600 ms interval 8.32 (SD = 2.4) out of 12 times (70%), while participants exposed to static circles selected the 600 ms interval 8.1 (SD = 2.1) out of 12 times (67.5%). The preference for the longer lasting interval was significantly higher than chance in both groups of participants (Flashing condition: t(27) = 5.17, p < .0001; Static condition: t(27) = 5.27, p < .0001).
In the VC condition, the duration of both intervals was the same (T1 = T2 = 500 ms) but the spatial length of intervals was variable (L1/L2 = 4 cm/6 cm or L1/L2 = 6 cm/4 cm). Results are depicted in Figure ). The Kappa effect was observed in both the flashing and static condition. Participants consistently selected longer intervals (6 cm) more frequently as long-lasting. Subjects exposed to flashing circles, chose the 6 cm-interval as “longer lasting” in average 8.68 (SD = 2.2) out of 12 times (72.3%), a frequency significantly higher than chance (t(27) = 6.5, p < .0001). Subjects exposed to static circles, chose the 6 cm-interval as “longer lasting” 7.93 (SD = 2.1) out of 12 times (66.1%), a frequency significantly higher than chance (t(27) = 4.88, p < .0001).
In order to investigate whether the Kappa effect was significantly stronger in the flashing condition, comparisons were conducted between the frequencies of selection of the 6 cm interval in the VC condition, across participants exposed to the flashing vs. static circles. An independent sample t-test comparison revealed that the difference did not reach significance (t(54) = 1.3, p = .19)
L1 = length of the first interval; L2 = length of the second interval; T1 = duration of the first interval; T2 = duration of the second interval.
3.2. Tau effect
Spatial Judgments Participants were instructed to select the longer interval in distance—either the first or the second. The frequency with which they selected intervals varied depending on the space/time configuration. In the CC condition the results were the following: participants exposed to flashing circles, in average, selected the first interval 5.6 (SD = 2.3) out of 12 times (46.2%), while participants exposed to static circles selected the first interval 5.9 (SD = 2.6) out of 12 times (49.2%). Interval selection in the CC condition did not differ significantly from chance in neither group of participants (p’s > .3). Similar to the case of temporal judgments, participants´ choices in the CC condition did not differ significantly from chance. This suggests an absence of primacy effects in our data. In the VC condition (length variable, L1/L2 = 6 cm/4 cm or L1/L2 = 4 cm/6 cm and time constant T1 = T2 = 500 ms), in average, participants correctly selected the longer interval (6 cm) most of the time: participants exposed to flashing circles, selected the 6 cm interval 11.3 (SD = 0.8) out of 12 times (91.4%), while participants exposed to static circles selected the 6 cm interval 11.8 (SD = 0.3) out of 12 times (98.3%). The preference for the longer interval was significantly higher than chance in both groups of participants (Flashing condition: t(27) = 24, p < .0001; Static condition: t(27) = 86, p < .0001).
The Tau effect was observed among participants exposed to flashing circles, but it was not observed among participants exposed to static circles. Results are depicted in Figure ). In the CV condition, the length of both intervals was the same (L1 = L2 = 5 cm) but the duration of intervals was variable (T1/T2 = 400 ms/600 ms or T1/T2 = 600 ms/400 ms). In the flashing condition, as predicted by the Tau effect, participants consistently selected more longer lasting intervals (600 ms) as “longer in distance” an average of 7 (SD = 1.5) out of 12 times (58.4%). This frequency was significantly higher than chance (t(27) = 3.53, p = .001). However, subjects exposed to static circles, chose intervals randomly. The 600 ms interval was chosen as “longer in distance” an average of 5.92 (SD = 1.65) out of 12 times (49.3%), a frequency that was not significantly different from chance (t(27) = 0.22, p = .82).
In order to investigate whether the Tau effect was significantly stronger in the flashing condition compared to the static condition, comparisons were conducted between the frequencies of selection of the 600 ms interval in the CV condition, across participants exposed to the flashing circles vs. static circles. An independent sample t-test comparison revealed a significant difference between groups (t(54) = 2.27, p < .03).
Taken together, the results are consistent with the presence of asymmetry in space/time interference as predicted by CMT. Kappa effect was observed both when circles were flashing and static, but Tau effect was only observed when dots were flashing as in typical paradigms. This suggests that, when the imputed velocity is reduced (by making circles static), space interference on temporal judgments remains, but time-on-space interference does not.
4. Discussion
The human perceptual system tends to interpret discontinuous visualizations as moving objects that will continue to move at a constant speed. Imputation of velocity to flashing lights, has been proposed as one candidate cause of the Tau and Kappa effects (see Jones & Huang, Citation1982 for a review). The characteristics of the stimuli were manipulated so as to reduce the imputation of velocity (Anstis & Ramachandran, Citation1987) in the hope of influencing the Tau and Kappa effects. The results suggest that such manipulation uncovered an asymmetrical pattern: Tau but not Kappa was significantly reduced when circles remain static.
Kappa and Tau effects have been recently interpreted as evidence in favor of ATOM theory and against the predictions of CMT (Lourenco & Longo, Citation2011). The results reported here suggest that these effects are coherent with CMT. In line with what has been previously shown in the literature (Boroditsky, Citation2000; Bottini & Casasanto, Citation2013; Casasanto & Boroditsky, Citation2008; Casasanto et al., Citation2010; Gijssels et al., Citation2013; Merritt, Casasanto, & Brannon, Citation2010), our data provides evidence in favor of a clear influence of the space domain over temporal judgments, even when display of the stimuli is such that participants may not impute motion to the circles in the screen. Temporal context, on the other hand, although exerting a measurable influence on spatial judgments (consistent with previously reported Tau effect) failed to prevail when imputation of motion was reduced by stimuli manipulation, suggesting that the Tau effect depends on the imputation of motion. Such an asymmetrical dependence on perceived velocity, goes along the prediction of CMT and presents a challenge for ATOM.
Previous studies on Tau and Kappa had focused on the bidirectionality of the interactions and not on their symmetrical or asymmetrical character (e.g. Benussi, Citation1913; Bill & Teft, Citation1969; Cohen et al., Citation1953; Collyer, Citation1977; Helson, Citation1930; Jones & Huang, Citation1982; Price-Williams, Citation1954; Sarrazin et al., Citation2004). Interference bidirectionality has been the basis of using Tau and Kappa effects as evidence in favor or an undifferentiated magnitude system in the brain (ATOM) and against CMT. But CMT is not at odds with bidirectional interactions, as long as the influence of concrete concepts on abstract ones is greater than the reverse. On top of that, evidence of an asymmetry in the strength of the Tau and Kappa effects (under conditions in which imputation of velocity is perturbed), as the one reported here, further aligns with CMT predictions.
Acknowledgements
We thank the members of the Language and Cognition lab at Universidad de los Andes for their help on the data recollection process. This research was funded by the Fondo de Apoyo para Profesores Asistentes (FAPA), Vicerrectoría de Investigaciones, Universidad de Los Andes, Bogotá, Colombia.
Additional information
Funding
Notes on contributors
Florencia Reali
Florencia Reali is an Associate Professor in the Psychology Department at the Universidad de los Andes in Colombia. She currently teaches subjects such as perception, psychology of language, psycholinguistics and methods in experimental psychology. Her major research interests cover language evolution, cognitive linguistics and human language acquisition and processing.
References
- Abe, S. (1935). Experimental study on the co-relation between time and space. Tohoku Psychologica Folia, 3, 53–11.
- Altverson, H. (1994). Semantics and experience: Universal metaphors of time in English, Mandarin, Hindi, and Sesotho. Baltimore: Johns Hopkins University Press.
- Anstis, S., & Ramachandran, V. S. (1987). Visual inertia in apparent motion. Visual Research, 27(5), 755–764.
- Benussi, V. (1913). Versuche zur Analyse taktil erweckter Scheinbewegungen [Analysis attempts of apparent motion induced by tactile stimulation]. Archiv fur die gesamte Psychologie, 36, 58–135.
- Bill, J. C., & Teft, L. W. (1969). Space–Time relations: Effects of time on perceived visual extent. Journal of Experimental Psychology, 81, 196–199. doi:10.1037/h0027425
- Boroditsky, L. (2000). Metaphoric structuring: Understanding time through spatial metaphors. Cognition, 75, 1–25.
- Bottini, R., & Casasanto, D. (2013). Space and time in the child’s mind: Metaphoric or ATOMic?. Frontiers in Psychology, 4, 1–9. doi:10.3389/fpsyg.2013.00001
- Bueti, D., & Walsh, V. (2009). The parietal cortex and the representation of time, space, number, and other magnitudes. Philosophical Transactions of the Royal Society B, 364, 1831–1840. doi:10.1098/rstb.2009.0028
- Casasanto, D. (2010). Space for thinking. In V. Evans & P. Chilton (Eds.), Language, cognition, and space: State of the art and new directions. London: Equinox Publishing, 453–478.
- Casasanto, D., & Boroditsky, L. (2008). Time in the mind: Using space to think about time. Cognition, 106, 579–593. doi:10.1016/j.cognition.2007.03.004
- Casasanto, D., Fotakopoulou, O., & Boroditsky, L. (2010). Space and time in the child’s mind: Evidence for a cross-dimensional asymmetry. Cognitive Science, 34, 387–405. doi:10.1111/cogs.2010.34.issue-3
- Cohen, J., Hansel, C. E. M., & Sylvester, J. D. (1953). A new phenomenon in time judgement. Nature, 172, 901. doi:10.1038/172901a0
- Collyer, C. E. (1977). Discrimination of spatial and temporal intervals defined by three light flashes: Effects of spacing on temporal judgments. Perception & Psychophysics, 21, 357–364. doi:10.3758/BF03199487
- de Hevia, M. D., & Spelke, E. S. (2010). Number-space mapping in human infants. Psychological Science, 21(5), 653–660. doi:10.1177/0956797610378686
- Dehaene, S., Bossini, S., & Giraux, P. (1993). The mental representation of parity and number magnitude. Journal of Experimental Psychology: General, 122, 371–396. doi:10.1037/0096-3445.122.3.371
- Dormal, V., & Pesenti, M. (2007). Numerosity-length interference: A Stroop experiment. Experimental Psychology, 54(4), 289–297. doi:10.1027/1618-3169.54.4.289
- Gijssels, T., Bottini, R., Rueschemeyer, S. A., & Casasanto, D. (2013). Space and time in the parietal cortex: FMRI evidence for a neural asymmetry. Proceedings of the 35th annual conference of the Cognitive Science Society (pp. 495–501). Austin, TX: Cognitive Science Society.
- Helson, H. (1930). The tau effect: An example of psychological relativity. Science, 71, 536–537. doi:10.1126/science.71.1844.450
- Helson, H., & King, S. M. (1931). The Tau effect: An example of psychological relativity. Journal of Experimental Psychology, 14, 202–217. doi:10.1037/h0071164
- Henik, A., & Tzelgov, H. (1982). Is three greater than five: The relation between physical and semantic size in comparison tasks. Memory & Cognition I, 10, 389–395. doi:10.3758/BF03202431
- Jones, B., & Huang, Y. L. (1982). Space–Time dependencies in psychological judgment of extent and duration: Algebraic model of the tau and kappa effects. Psychological Bulletin, 91, 128–142. doi:10.1037/0033-2909.91.1.128
- Lakoff, G., & Johnson, M. (1990). Metaphors we live by. Chicago: Chicago University Press.
- Lakoff, G., & Johnson, M. (1999). Philosophy in the flesh. New York, NY: Basic Books.
- Lourenco, S. F., & Longo, M. R. (2011). Origins and the development of generalized magnitude representation. In S. Dehaene & E. Brannon (Eds.), Space, time, and number in the brain: Searching for the foundations of mathematical thought. Amsterdam, NL: Elsevier, 225–244.
- Merritt, D. J., Casasanto, D., & Brannon, E. M. (2010). Do monkeys think in metaphors? Representations of space and time in monkeys and humans. Cognition, 117, 191–202. doi:10.1016/j.cognition.2010.08.011
- Piaget, J. (1927/1969). The child’s conception of time. New York, NY: Ballantine Books.
- Piaget, J. (1941/1952). The child´s conception of number. London: Routledge & Kegan.
- Piaget, J. (1948/1956). The child’s conception of space. London: Routledge & Kegan Paul.
- Price-Williams, D. R. (1954). The kappa effect. Nature, 173, 363–364. doi:10.1038/173363a0
- Sarrazin, J. C., Giraudo, M. D., Pailhous, J., & Bootsma, R. J. (2004). Dynamics of balancing space and time in memory: Tau and kappa effects revisited. Journal of Experimental Psychology: Human Perception and Performance, 30, 411–430.
- Schneider, W., Eschman, A., & Zuccolotto, A. (2002). E-Prime reference guide. Pittsburgh, PA: Psychology Software Tools.
- Srinivasan, M., & Carey, S. (2010). The long and the short of it: On the nature and origin of functional overlap between representations of space and time. Cognition, 116, 217–241. doi:10.1016/j.cognition.2010.05.005
- Walsh, V. (2003). A theory of magnitude: Common cortical metrics of time, space and quantity. Trends in Cognitive Sciences, 7(11), 483–488.
- Xuan, B., Zhang, D., He, S., & Chen, X. (2007). Larger stimuli are judged to last longer. Journal of Vision, 7(10), 1–5. doi:10.1167/7.10.2