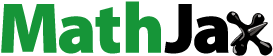
Abstract
The main advantage of biodiesel to fossil-diesel is attributed to the ester functional group in its structure. Many researchers have affirmed that the functional group assists the diesel engine to function at its peak. However, the efficiency of the biodiesel to keep the mechanical piston-strokes in the diesel engine is experimentally presumed low especially if the engine works for a long time. In this research, it was proposed that the cyclic break down of the ester functional group of biodiesel is a major disadvantage. Butanol, ethanol and methanol biodiesel were produced in the laboratory using waste vegetable oil from restaurant. The Ultraviolet-visible spectroscopy or spectrophotometer (UV–Vis) was used to investigate the cyclic break down in the Butyl-, Methyl- and Ethyl- biodiesel. The Ultraviolet-visible spectroscopy or spectrophotometer (UV–Vis) was used to probe the various biodiesel samples with a start and stop wavelength of 500 and 900 nm respectively. The wavelength interval was 1 nm at a high scanning speed. Between 500–550 and 800–850 nm, the Ethyl- and Butyl- biodiesel had an absorbance of 2.7 and 1.6 respectively with 3 peaks each. Methyl-biodiesel had the highest absorbance of 1.7 at 600–650 nm with 1 peak. The computational extrapolation of all outcomes affirms that cyclic breaking is highest in Butyl-biodiesel. The most resilient biodiesel type was the Ethyl-biodiesel. However, it was recommended that cyclic breaking may be preserved by ratio-mixing of biodiesel with fossil-diesel.
Public Interest Statement
This paper seeks ways of converting waste to a more efficient product. However, the efficient adaptation of biodiesel obtained from waste vegetable oil (WVO) to run an automobile engine is not known. The objective of this work is to adopt an original technique to obtain the best quality of bio-diesels from Waste vegetable oil (WVO). Based on the results gotten from the UV spectrophotometry analysis, biodiesel gotten from Ethyl-esters are the most resilient. Hence, cyclic breaking may be preserved by ratio-mixing of biodiesel with fossil-diesel.
1. Introduction
Biodiesel varies according to the production method. There are different blends of biodiesel, ranging from 2% biodiesel, 98% petro-diesel or B2, to the purest form yet, 100% biodiesel or B100. New research on biodiesel has repositioned it among the renewable energy option (Emetere, Akinyemi, & Edeghe, Citation2016; Uno, Emetere, Fadipe, & Oyediji, Citation2015) in the world today. Recently, automobile companies are making cars that run with biodiesel (Table ). According to ASTM (Citation2016), there are a few parameters that have to be met before biodiesel can be used in engines without any damage. Some of these properties include the cloud point, cetane rating, pour point, viscosity, higher heating value (HHV), its density, type of emission, etc. The cloud point, pour point, viscosity and density variability, are all known as the cold-flow properties of the biodiesel. These properties affect the performance of the biodiesel at lower temperatures. Biodiesels that have higher viscosity values perform poorer due to an increased difficulty in its vaporization and atomization. Eventually, higher emissions due to poorer combustion are expected in the long run. The HHV of biodiesels is the energy that resides in the fuel, which is readily converted into thermal energy, taking into account the latent heat of vaporization of water. The cetane rating of biodiesel is a rating of the ignition time for diesels. A typical biodiesel molecule, like the image shown below (Figure ) consists mostly of a long chain of carbon atoms, with hydrogen atoms attached, with an ester at one end. Diesel engines can burn biodiesels typically. However, it comes with its peculiar challenges which have been resolved by replacing of some of the rubber tubing in the engine structure because it is weakened by the biodiesel (Biofuels, Citation2016; Haas, Scott, Alleman, & McCormick, Citation2001). Diesel fuel, is not much different from biodiesel, chemically, the only difference between the two of them (diesel fuel and biodiesel) being the additional ester at one end of the biodiesel in its structure. Biofuels have also been described as solvents. Reports have been filed about the blockage of the fuel filters for engines that switched from conventional diesel to biodiesel. This blockage is because biodiesel clears up the gunk in the fuel transmission line and the engine. This challenge was resolved by the replacement of the blocked oil filters (Biofuels, Citation2016; Haas et al., Citation2001).
Table 1. Automobiles company that has adopted the biodiesel engine
Figure 1. (a) Butyl ester, (b) Ethyl ester, (c) Methyl-ester and (d) process of drying the oil.
Most vegetable oils belong to a category of compounds called esters. In the transesterification process, Methanol (which is turned to sodium methoxide) is used commonly to produce Methyl esters, which are also called FAME’s (Fatty Acid Methyl Esters) because it is a very cheap form of alcohol (Yusoff, Xu, & Guo, Citation2014). Ethanol is also used sometimes to produce Ethyl esters, which are also commonly referred to as the lesser-known Fatty Acid Ethyl Esters (FAEE) (Sebastian, Muraleedharan, & Santhiagu, Citation2016; Yusoff et al., Citation2014). Alcohols higher than methanol and ethanol such as isopropanol and butanol have also been used. The efficiency of the transesterification reaction varies inversely with the molecular weight of the alcohol was used. Also, it is directly related to the cold flow properties of the resulting ester. In the production of FAMEs, the methanol added, breaks the bond of the hydrocarbon chain with glycerol, which acts like a backbone to the vegetable oils. As the glycerol forms, the former bonds between the glycerol and hydrocarbon chain are replaced with bonds to methoxy-groups, which came from the methanol. There are also many other processes and steps involved in making biodiesel, the first of which is removing the water from the vegetable oil, which if not conducted will result in saponification.
The methanol used in most biodiesel production processes is made using fossil fuel inputs. However there are sources in which bio-methanol (methanol made using non-conventional or renewable methods) can be obtained using carbon(IV) oxide or biomass feedstock making the production process of that biodiesel fossil fuel free. In producing biodiesel, glycerol is a product and the amount of biodiesel produced to glycerol is normally 10:1.
In the world today, the production of biodiesel is increasing steadily every year, however the feedstock being used are either too expensive or farfetched e.g. alligator fat (AAA World Magazine, Citation2011). Also, the feedstock may not be obtained locally. Nigeria is an emerging country with growing energy demands. The increasing awareness in the rate of destruction of ecosystems of the earth through pollution (Emetere, Citation2016; Emetere, Akinyemi, Oladimeji, Citation2016a, Citation2016b) is the basic reason renewable energy is preferred. This reason raises deep concern that little work is being done locally, to facilitate the production of energy from locally verified means. The little work which has been done however on the production of biodiesel in Nigeria, focuses on using seeds or feedstock. Recently, the Nigerian National Petroleum Corporation have embarked on farming Jathropa as the preferred source of biodiesel production. The few biodiesels that have been mass-produced, have some level of Nitrogen and/or Sulphur, although not as much as petroleum products. The aim of this research is to investigate the best way to preserve energy using the most contaminated feedstock i.e. ‘Waste Vegetable Oils (WVO)’ that can be used to produce biodiesel.
There have also been researches in the area of the efficiency of biodiesels and the cost effects of their implementation. In this light, some research has adopted catalyzed production of biodiesel from feedstock i.e. hastening the process of production via other means like sonic bombardment of the oil (Korshavi, Shariati, & Nikou, Citation2016).
Ayalasomayajula et al. (Citation2012) observed that a greater percentage of the 700 million gallons of biodiesel produced was from Soybean oil. This rate of production however is smaller than the required demand, hence the need for the development of other sources of oils that can be used in the production of biodiesel. Unlikely sources of oil from non-food sources have evolved since the search for non-food sources of biodiesel began. Some of these unconventional sources include WVO and Sewage Sludge (American Chemical Society, Citation2016; Ayalasomayajula et al., Citation2012; Thanh, Okitsu, Boi, & Maeda, Citation2012). WVO has a high potential as a very sustainable feedstock. Recently Dansie (Citation2016) was able to demonstrate the production of biodiesel from WVO’s exposing it (WVO) to direct ultrasonic irradiation with Lye (Concentrated Sodium or Potassium Hydroxide) as catalyst. The addition of methanol to the production process was able to boost the production or conversion efficiency by up to 99%, whilst reducing reaction time to ten seconds. With as little as 0.75 or 1.25% Sodium or Potassium Hydroxide respectively, the conversion can be completed, if the pellets of the aforementioned sodas are grounded and mixed completely with the methanol and WVO.
From experimental point of view, the same preparation technique is not recommended for Butyl-biodiesel, Methyl-biodiesel and Ethyl-biodiesel because it undergoes intramolecular esterification which leads to cyclic esters. The formation of Butyl-ester (Figure (a)), Methyl-ester (Figure (b)) and Ethyl-ester (Figure (c)) can only exist at certain laboratory conditions, for example, Butyl-ester production are more sensitive to molar ratio and reaction temperature (Jha, Gupta, & Kumar, Citation2007); Methyl-ester and Ethyl-ester are more sensitive to molar ratio than temperature (Boocock, Konar, Mao, & Sidi, Citation1996; Zhou, Konar, & Boocock, Citation2003). Hence, the transesterification is expected to be maximum at a reaction time of one hour to reach the highest yield of biodiesel. However, in the real sense, the excessive volume of glycerin produced in the batch process suggests that the different alkyl ester may likely undergo cyclic break down at reaction time beyond two hours due to its instability. This is a major disadvantage to the progress made so far on the biodiesel. In this paper, we examined the reasons of cyclic breaking using the UV spectrophotometry. The choice of the UV spectrophotometry is hinged from the photochemistry concept. With the UV spectrophotometry, the inner-shell photoexcitation foster an indepth knowledge of the selective chemical bond breaking (Eberhardt et al., Citation1983; Wada, Kizaki, Matsumoto, Sumii, & Tanaka, Citation2006) that had been propose in the preparation of biodiesel. The success of this research would assist automobile companies on the design and construction of diesel engine to drive the successful use of biodiesel.
2. Theory of the UV absorption technique
The absorption process of the UV electromagnetic radiation is dependent on the atomic structure and energy level associated with its position in the atom. The two common regions based on the light absorption wavelength are the UV region (190–380 nm) and visible region (380–900 nm). Chemical groups e.g. chromophores can be detected by UV–Vis spectrophotometers because of its multiple bond (such as C=C, C=O or C≡N). The biodiesel possess the multiple bond (C=O) that is peculiar with chromophore. In this study, it was proposed that the absorption of this multiple bonds during absorption spectroscopy affords salient information about the biodiesel (Lewis, Lee, & Kidder, Citation2004). Zawadzki, Shrestha, and He (Citation2007) quantified the absorption spectroscopy in form of absorbance index that originally measures the shape of the absorbance in the UV absorbance spectra.(1)
(1)
where AI = absorbance index, Axxx = absorbance at the xxx nm wavelength. The error due to noise (%) of the UV absorbance spectra is given by Owen (Citation2000) as(2)
(2)
where At is the true absorbance and Am is the measured absorbance.
The absorbance and wavelength in the UV spectroscopy is related as(3)
(3)
where A is absorbance, λ is wavelength, n is the order of the relationship between absorbance and wavelength, and “a” is a constant.
3. General methodology
The general method for the production of biodiesel has evolved over the years. There are many processes, which over time, have proved to be very efficient in the production of biodiesel, however there have also been ingredients and processes alike, which have been proven to be equally unnecessary in the production of biodiesel.
WVOs are the remnant oils left from kitchens ranging from fast food outlet and restaurants to household kitchens. It is expected that WVO is normally darker than the usual or original vegetable oil, and in most cases contains food particles, residue, and in some cases water. In the preparation of biodiesel from WVOs, there are a few steps involved in the initial preparation stages, and these are:
3.1. Filtration
The first step to the production of biodiesel is to filter the WVO. The filtration process is to remove any food particles or residue that may be contained in the oil. This process does not involve any complex filtration process. Simple cheesecloth or filter would be sufficient for this process. Failure to filter a sample or batch of WVO may lead to very undesirable results.
3.2. Separation I
This separation is to remove water that may be in the oil, and is a simple process. It involves letting the filtered oil to stand for 8 h at least, to allow the water settle to the bottom of the container or tank. After this is done, the oil is to be pumped into another tank or container.
3.3. Heating of the oil
Even after the oil has been separated from the solvent, there will still be some impurities in the oil due to exposure. So, the oil is boiled again to about 110°C to make sure there is no water content present in the oil (Figure (d)). When the oil cooled down it was put in the set up below. A thermometer was placed carefully to make sure that a temperature of 60°C was obtained. Some researcher try to bleach the dark oil to change the colouration.
3.4. Mixing
The mixing process involves two stages:
(1) | Mixing the Alkanol with concentrated Potassium Hydroxide (KOH) or concentrated Sodium Hydroxide (NaOH), both are generally referred to as lye. | ||||
(2) | Mixing the resulting Potassium or Sodium X-Oxide (where X represents the type of alkanol used e.g. using Methanol, Ethanol or Buthanol would result in Methoxide, Ethoxide or Buthoxide respectively). |
In mixing the Alkanol, WVO and Lye, the standard ratio between the Alkanol and WVO is 1:4, while the Lye is 3.5 g for this ratio. When mixing, there are a few things to take cognizance of, and they are:
The mixture between Alkanol and Lye is an exothermic one, and is normally in the temperature range of 50–60°C, or depending on the boiling point of the alkanol. A precise example of this is when mixing the lye with ethanol, for example, if the oil is at a temperature, higher than the boiling point of ethanol, once the lye and ethanol mix is introduced into the oil, the ethanol will boil away, leaving an incomplete reaction. Hence before the Alkanol X-Oxide is mixed with the WVO, the WVO is meant to be treated, based on the kind of alkanol been used. Sodium and Potassium are group 1 elements, and group 1 elements react very vigorously with cold water, hence the preheating procedure. The oil is normally preheated before mixing with Sodium or Potassium X-Oxide, to loosen the oil and allow the proper penetration and breakage of the glycerin backbone of the oil, however care must be taken not to make the oil hotter than the boiling temperature of the alkanol to be used (Figure ).
(1) | The mixture between the Lye and Alkanol takes up to fifteen minutes of continuous stirring, because the mixture is meant to be stirred until it is clear, hence, patience is required (Figure ). |
3.5. Separation II
The second separation in this process is in two stages:
(1) | Separation of Glycerin from impure biodiesel: This involves waiting for 8–24 h, or more, depending on the amount of WVO used, for the glycerin, which has been disconnected from the oil (chemically), to settle. However, as paramount as it is to wait long enough for the glycerin to settle, it is, also worthy to remember not to allow the glycerin stay for too long, as it may cake, hence becoming very difficult to remove. A tap at the bottom of the container being used will solve this problem. | ||||
(2) | Separation of Impurities from impure biodiesel: This is where the initial filtration process pays of, as the initial filtration process, if done properly, would make the separation in this stage just involve washing. Washing in this context involves the use of hot water to run through the impure biodiesel to remove any other impurities, not already taken care of. This process is advised to be done at least twice. |
4. Results and discussion
The initial results of the WVO and the product i.e. Methyl-biodiesel are shown in Table . This result explains the difference between the properties of WVO and processed biodiesel.
Table 2. Initial properties of WVO and Methyl-biodiesel
The ultraviolet spectrometer makes use of visible spectrophotometry, this process is dependent on the absorption and reflection of the fluid. This is determined by the wavelength chosen to interpret the fluid. The more easily excited the electrons the lower energy gap between the HOMO and the LUMO, the longer the wavelength of light it can absorb. A range of wavelength of 500–900 nm was used. This was further divided into four i.e. 500–600, 600–700, 700–800, and 800–900. The biodiesels were mixed in the ratio of 1:10 of the catalyst i.e. methanol. Below are table presentations of values for the results of the UV spectrometer test conducted for biodiesels of the WVO in this research. The specification of the UV spectrometer used for this work is shown in Table .
Table 3. Specification of the UV spectrometer
The basic statistics of the different absorbance for WVO 1 (ethanol treatment i.e. Ethyl-biodiesel), WVO 2 (butanol treatment i.e. butyl-biodiesel) and WVO 3 (methanol treatment i.e. Methyl-biodiesel) are exhibited as shown in Tables – respectively. WVO 1 responded most to all the wavelength of the UV spectrophotometry. The wavelength 800–900 nm showed the highest magnitude and number of absorption, however, wavelength 500–600 nm showed the highest standard deviation and coefficient of variation. This may likely portend that while the wavelength 800–900 nm could capture the Ethyl-ester structure better than other wavelength range, the wavelength 500–600 nm captures the degree of cyclic breaking of the ester (Table ).
Table 4. The basic statistics of absorbance in WVO 1
Table 5. The basic statistics of absorbance in WVO 2
Table 6. The basic statistics of absorbance in WVO 3
For WVO 2, the highest absorption is in the range of wavelength 800–900 nm. Also, it had the highest number of absorption outcome, highest standard deviation and coefficient of variation. This result may be a trivial conclusion that the wavelength 800–900 nm could capture the butyl-ester structure and the degree of cyclic breaking of the ester better than other wavelength range (Table ).
The same result trend that was observed for WVO 2 was seen in WVO 3 i.e. Methyl-ester. However the initial difference can be seen in the highest number of values obtained for wavelength 500–600 nm and 800–900 nm in WVO 2 and WVO 3 respectively (Table ). Also, the highest number of absorption values of the biodiesel may partly support the idea of site desorption concept (Wada et al., Citation2003). The site absorption also can be one of the ways to affirm the role of inter and intra-molecular configurations of reactive sites.
The high absorbance of the Ethyl-ester in Figure (a), depicts many scenarios in science. First, it depicts the semi-quantitative test of identity which may be bond structure or deformation at a specified wavelength. According to Lucas et al. (Citation2006), absorbance of the Ethyl-ester reveals the likelihood that three cations of the Ethyl-ester would create peak shifts that may alter the positions of aromatic absorbance peaks. Hence, the five peaks noticed in Figure (a) may likely depict how many times the three cations of the Ethyl-ester have altered the chemical bonds. Antosiewicz and Shugar (Citation2016), suggested that the peaks shift of Ethyl ester suggests that one or more of the elements (in this case protein Tyr residues) are located near the surface.
Figure 4. (a) Absorbance of Ethyl-ester at wavelength of 500–600 nm, (b) absorbance of Methyl-ester at wavelength of 500–600 nm, (c) absorbance of butyl-ester at wavelength of 500–600 nm and (d) comparative of absorbance of Ethyl-, methy-, and butyl-ester at wavelength of 500–600 nm.
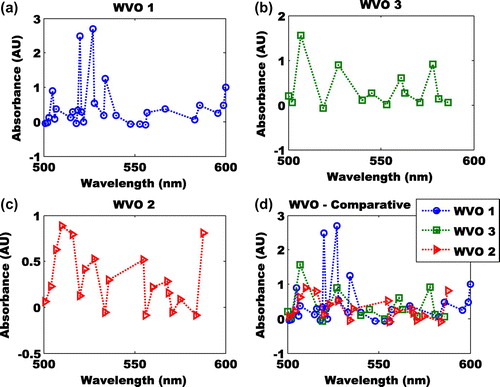
It had been suggested that in the chemistry of Methyl-ester, neither the C=C double bond nor the carbonyl on their own have intense maxima above 200 nm (RSC, Citation2016). A research conducted by Westbrook, Pitz, Sarathy, and Mehl (Citation2013) by combusting different Methyl-ester derived biodiesel showed that the components of Methyl ester may contain as high as three C=C double bonds. The number of the C=C double bonds reduce rate of low temperature reactivity and the cetane number. Hence, the absorption of the Methyl-ester may logically refer to the five structures of Methyl-ester (Methyl stearate, Methyl oleate, Methyl linoleate, Methyl linolenate, and Methyl palmitate) components in biodiesel fuels. We conducted the absorbance index and error analysis to understand the absorption of the Methyl bonds as it relates to length of the hydrocarbon chains, the number and position of the C=C bonds.
The butyl-ester is subject to hyperchromic effect because of the increase or decrease of the availability of “n” electrons in negatively charged ion shifts (Kumar, Citation2016). More revealing is the possibility of two symmetrical Methyl deformations when the presence of two or more Methyl groups on the t-butyl groups results in the splitting of its band. Hence, a comparative study of the butyl, Methyl and Ethyl esters may likely reveal bonds deformation.
The highest absorbance was found at 527 nm (Figure (a)). The absorbance of the Ethyl-ester between wavelengths of 500–600 nm revealed five peaks at 505, 520, 527, 534 and 600 nm. The absorbances of the peak shift are 1.583, 0.216, −1.453 and −0.241. These results are evidences of hyperchromic effect in the Ethyl-ester biodiesel. This affirms the possibilities of cyclic breaking of the Ethyl-ester, hence a reduction of cetane number.
The absorbance of the Methyl-ester between wavelengths of 500–600 nm revealed four peaks (Figure (b)) at 507, 527, 561 and 578 nm. The highest absorbance was found at 507 nm. The reduced value of the peak-shift reveals a lower presence of the C=C bond. The absorbances of the peak shift are −0.67, −0.294 and 0.018. Unlike the Ethyl-ester biodiesel, the absorbances of the peak shift of the Methyl-ester biodiesel consistently increased. We infer that the reduction of the length of the hydrocarbon chains occurred. Hence, the cyclic breaking was very minimal.
The highest absorbance was found at 510 nm (Figure (c)). The absorbance of the butyl-ester between wavelengths of 500–600 nm revealed four peaks at 507, 510, 516 and 588 nm. The absorbances of the peak shift are 0.261, −0.095 and 0.011. These results are evidences of hyperchromic effect that is common with the butyl-ester (Kumar, Citation2016) biodiesel. This affirms the possibilities of cyclic breaking of the butyl-ester to possibly Methyl-ester and a reduction of cetane number. The comparative study of the three esters shows that their absorbances were almost uniform between 535 and 600 nm (Figure (d)). Could this be an evidence of cyclic breaking to Methyl-esters?
At a wavelength of 600–700 nm, a poor absorbance was observed except for the Ethyl-ester biodiesel (Figure (a)–(c)). Hence, a reliable comparative analysis (Figure (d)) cannot be obtained. The highest absorbance was found at 609 nm (Figure (a)). The absorbance of the Ethyl-ester between wavelengths of 600–700 nm revealed five peaks at 609, 624, 636, 641 and 661 nm. The absorbances of the peak shift are −0.241, 0.155, −0.232 and −0.117. These results i.e. comparing the absorbance of the three esters shows that large component of Ethyl-ester structure are maintained (Antosiewicz & Shugar, Citation2016). This observation reveals the low degree of cyclic breaking in Ethyl-ester. Hence, it may be more stable than Methyl- and butyl-ester biodiesels.
Figure 5. (a) Absorbance of Ethyl-ester at wavelength of 600–700 nm, (b) absorbance of Methyl-ester at wavelength of 600–700 nm, (c) absorbance of butyl-ester at wavelength of 600–700 nm and (d) comparative of absorbance of Ethyl-, methy-, and butyl-ester at wavelength of 600–700 nm.
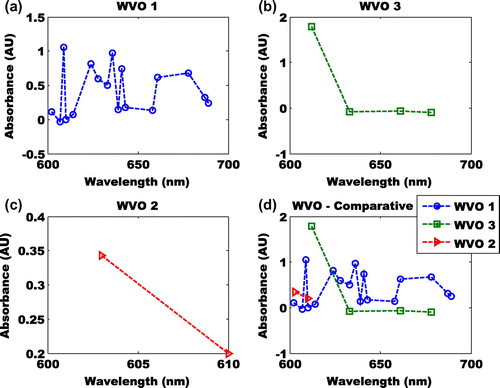
The highest absorbance was found at 771 nm (Figure (a)). The absorbance of the Ethyl-ester between wavelengths of 700–800 nm revealed nine peaks at 707, 709, 730, 747, 756, 770, 771, 781, and 786 nm. The absorbances of the peak shift are −0.084, 0.618, −0.183, 0.359, −0.722, 0.809, −0.727 and 0.149. These results are evidences of hyperchromic effect in the Ethyl-ester biodiesel. This result further affirms the possibilities of cyclic breaking of the Ethyl-ester, hence a reduction of cetane number.
Figure 6. (a) Absorbance of Ethyl-ester at wavelength of 700–800 nm, (b) absorbance of Methyl-ester at wavelength of 700–800 nm, (c) absorbance of butyl-ester at wavelength of 700–800 nm and (d) comparative of absorbance of Ethyl-, methy-, and butyl-ester at wavelength of 700–800 nm.
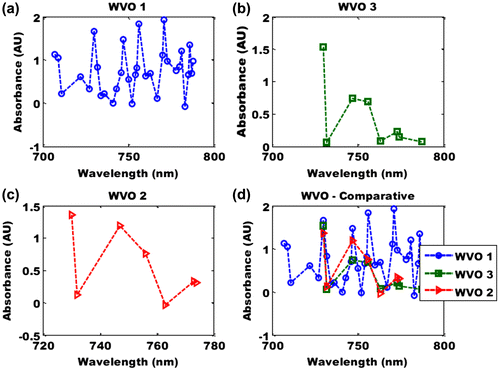
The absorbance of the Methyl-ester between wavelengths of 700–800 nm revealed two peaks (Figure (b)) at 747 and 756 nm. The highest absorbance was found at 730 nm. The absorbance of the peak shift is −0.05. Not enough information can be used to infer any event (Figure (b)). The same fate holds for WVO 2 (Figure (c)). However, its maximum absorbance is at wavelength 730 nm. The comparative absorbance features showed that the three esters (Methyl-, Ethyl- and butyl) had a convergence at about 730 and 753 nm (Figure (d)).
The highest absorbance was found at 871 nm (Figure (a)). The absorbance of the Ethyl-ester between wavelengths of 800–900 nm revealed eight peaks at 815, 824, 841, 857, 871, 875, 884 and 896 nm. The absorbances of the peak shift are −0.315, 0.590, 0.474, 1.022, −1.465, −0.282 and −0.423. These results are evidences of hyperchromic effect in the Ethyl-ester biodiesel. This result further affirms the possibilities of cyclic breaking of the Ethyl-ester, hence a reduction of cetane number.
Figure 7. (a) Absorbance of Ethyl-ester at wavelength of 800–900 nm (b) absorbance of Methyl-ester at wavelength of 800–900 nm (c) absorbance of butyl-ester at wavelength of 800–900 nm (d) comparative of absorbance of Ethyl-, methy-, and butyl-ester at wavelength of 800–900 nm.
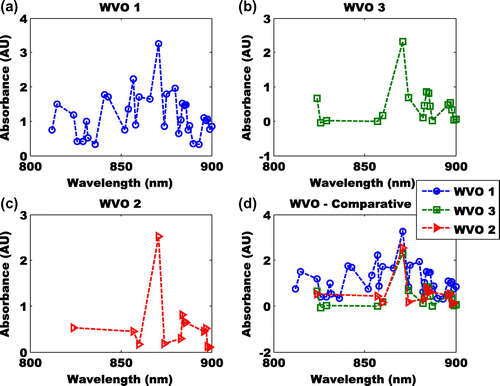
The absorbance of the Methyl-ester between wavelengths of 800–900 nm revealed three peaks (Figure (b)) at 871, 884 and 896 nm. The highest absorbance was found at 871 nm. The absorbance of the peak shift is −1.469 and −0.382. Not enough information can be used to infer any event (Figure (b)). The same fate holds for WVO 2 (Figure (c)). However, its maximum absorbance is at wavelength 871 nm. The comparative absorbance features showed that the three esters (Methyl-, Ethyl- and butyl) had a convergence at about 871 and 883 nm (Figure (c)). From the comparative results in Figures (d) and (d), it could be inferred that cyclic breaking coincide with one of the esters i.e. Methyl-ester.
Based on Figures , we performed the linear regression to comparatively see the impacts of absorbance index and error analysis of the three (Methyl-, Ethyl- and butyl). In this case, the absorbances for each wavelength group were compared to see if there any relationship which would reveal more features. The linear regression between absorbance index and error analysis of WVO 1 that was observed includes: absorbance index of 6.27% at a slope and interception of 0.034 and 2.95 respectively (AI 600 vs. AI 500 of Figure ), and absorbance error analysis of 3.42% at a slope and interception of 0.12 and 0.46 respectively (AE 600 vs. AE 500 of Figure ); absorbance index of 2.08% at a slope and interception of −0.07 and 8.0 respectively (AI 700 vs. AI 500 of Figure ), and absorbance error analysis of 1.9% at a slope and interception of −0.11 and 0.79 respectively (AE 700 vs. AE 500 of Figure ); absorbance index of 3.57% at a slope and interception of −0.31 and 23.5 respectively (AI 800 vs. AI 500 of Figure ), and absorbance error analysis of 0.1% at a slope and interception of −0.036 and 1.22 respectively (AE 800 vs. AE 500 of Figure ); absorbance index of 1.03% at a slope and interception of 0.29 and 6.46 respectively (AI 700 vs. AI 600 of Figure ), and absorbance error analysis of 3.08% at a slope and interception of −0.16 and 0.72 respectively (AE 700 vs. AE 600 of Figure ); absorbance index of 0.3% at a slope and interception of −0.67 and 27.96 respectively (AI 800 vs. AI 600 of Figure ), and absorbance error analysis of 10.41% at a slope and interception of −0.48 and 1.5 respectively (AE 800 vs. AE 600 of Figure ); absorbance index of 0.5% at a slope and interception of 0.24 and 19.34 respectively (AI 800 vs. AI 700 of Figure ), and absorbance error analysis of 2.32% at a slope and interception of 0.19 and 1.01 respectively (AE 800 vs. AE 700 of Figure ).
From the regression analysis of absorbance index and error analysis of WVO 1, all the results are reliable. The regression analysis of the error was less than six percent (>6%) except for 800/600 nm. This result makes the UV absorption technique more tolerable. Therefore the best sampling method to determine the quality of biodiesel are 600/500 nm, 800/500 nm and 700/500 nm. Despite the opposite trend (slope and intercept) between the absorbance index and error analysis, its reliability is non-questionable. The likely cause of opposite trend (slope and intercept) between the absorbance index and error analysis can be attributed to the reduced energy gap due to massive excitation of electron. The result further corroborated the evidence of cyclic breaking. This result can be corroborated with the findings of Lucas et al. (Citation2006). The chemistry of these results is also supported Sebastian et al., Citation2016.
Based on Figure , we performed the linear regression to comparatively see the impacts of absorbance index and error analysis of WVO 3 i.e. Figure . The linear regression between absorbance index and error analysis of WVO 3 that was observed includes: absorbance index of 40.45% at a slope and interception of 0.24 and 1.72 respectively (AI 700 vs. AI 500 of Figure ), and absorbance error analysis of 5.05% at a slope and interception of 0.18 and 0.36 respectively (AE 700 vs. AE 500 of Figure ); absorbance index of 3.71% at a slope and interception of −0.47 and 6.54 respectively (AI 800 vs. AI 500 of Figure ), and absorbance error analysis of 0.41% at a slope and interception of −0.10 and 0.35 respectively (AE 800 vs. AE 500 of Figure ); absorbance index of 3.07% at a slope and interception of 1.18 and 3.27 respectively (AI 800 vs. AI 700 of Figure ), and absorbance error analysis of 1.57% at a slope and interception of −0.24 and 0.37 respectively (AE 800 vs. AE 700 of Figure .
From the regression analysis of absorbance index and error analysis of WVO 3, all the results are reliable. The regression analysis of the error was less than six percent (>6%). This result makes the UV absorption technique more tolerable. Therefore the best sampling method to determine the quality of biodiesel is 700/500 nm.
The linear regression of absorbance index and error analysis of WVO 2 was performed as shown in Figure . The linear regression between absorbance index and error analysis of WVO 2 that was observed includes: absorbance index of 8.39% at a slope and interception of 1.59 and 0.88 respectively (AI 700 vs. AI 500 of Figure ), and absorbance error analysis of 0.86% at a slope and interception of −0.14 and 0.65 respectively (AE 700 vs. AE 500 of Figure ); absorbance index of 5.44% at a slope and interception of 4.48 and −1.48 respectively (AI 800 vs. AI 500 of Figure ), and absorbance error analysis of 23.21% at a slope and interception of 1.01 and 0.2 respectively (AE 800 vs. AE 500 of Figure ); absorbance index of 5.73% at a slope and interception of −1.16 and 17.51 respectively (AI 800 vs. AI 700 of Figure ), and absorbance error analysis of 1.29% at a slope and interception of 0.18 and 0.58 respectively (AE 800 vs. AE 500 of Figure ).
From the regression analysis of absorbance index and error analysis of WVO 2, all the results are reliable. The regression analysis of the error was less than six percent (>6%) except for 800/500 nm. This result makes the UV absorption technique more tolerable. Therefore the best sampling method to determine the quality of biodiesel are 700/500 nm and 800/700 nm.
Comparatively, the UV absorption spectroscopy has shown that WVO 3 has a higher quality than WVO 1 and WVO 2. It was also noted that different biodiesel may analyzed using different wavelength combination as shown in the study. This assertion was also supported by Lucas et al. (Citation2006). Hence more excitation of electron is expected for all the samples because of the cyclic breaking that is common to all. Hence, for optimization experimentation, preparation methods may be preferred for an improved result.
5. Conclusion
Based on the results gotten from the UV spectrophotometry analysis, biodiesel gotten from Methyl-, Ethyl- and Butyl-ester undergoes cyclic breaking which reduce the cetane number and performance in the diesel engine. Against traditional knowledge that butyl-ester is subject to hyperchromic effect, it was discovered in this text that Ethyl-ester is highly subject to hyperchromic effect. Hence the subjectivity of any ester to hyperchromic effect largely depends on the laboratory preparation method. It was suggested that hyperchromic effect in Ethyl-ester was majorly due to cyclic breaking. The absorbances of the peak shift of Ethyl-ester are −0.241, 0.155, −0.232 and −0.117. For Methyl-ester, the reduced value of the peak-shift reveals a typical evidence that there are lower presence of the C=C bond depicting hydrocarbon chain reduction. The absorbances of the peak shift are −0.67, −0.294 and 0.018. The absorbances of the peak shift for butyl-ester are 0.261, −0.095 and 0.011. The peaks position shows a likely transition from butyl-ester to possibly Methyl-ester and a reduction of cetane number. Comparing the absorbance of the three types of esters (i.e. butyl-ester, Ethyl-ester and Methyl-ester), it was proposed that large component of Ethyl-ester structure are maintained. This observation reveals a low degree of cyclic breaking in Ethyl-ester. Hence, it may be more stable than Methyl- and butyl-ester biodiesels.
From the regression analysis of absorbance index and error analysis of WVO 1, all the results are reliable. The regression analysis of the error was less than six percent (>6%) except for 800/600 nm. Hence, the best sampling method to determine the quality of biodiesel are 600/500 nm, 800/500 nm and 700/500 nm. From the regression analysis of absorbance index and error analysis of WVO 3, all the results are reliable. The regression analysis of the error was less than six percent (>6%). Therefore the best sampling method to determine the quality of biodiesel is 700/500 nm. From the regression analysis of absorbance index and error analysis of WVO 2, all the results are reliable. The regression analysis of the error was less than six percent (>6%) except for 800/500 nm. Therefore the best sampling method to determine the quality of biodiesel are 700/500 nm and 800/700 nm. Comparatively, the UV absorption spectroscopy has shown that WVO 3 has a higher quality than WVO 1 and WVO 2.
Cyclic breaking may be preserved by ratio-mixing of biodiesel with fossil-diesel. Also, the glycerin gotten from the production of the Methyl-ester, Ethyl-ester and butyl-ester biodiesels is much as shown in our laboratory pictures. Hence, the glycerin can be used in the production of soap or dynamite. It simply means we have succeeded in converting a total waste to a more efficient product.
Acknowledgement
The authors acknowledge Covenant University for the partial sponsorship of the project.
Additional information
Funding
Notes on contributors
M.E. Emetere
M.E. Emetere had his doctorate degree (PhD) in Industrial Physics. He has authored two textbooks and over hundred peer-reviewed international papers listed on the Thompson Reuters indexed journals and Scopus indexed journals. He won the prestigious AU/TWAS Young Scientist National Award (Earth and Life Sciences) in 2015. He has won few travel grants to Italy, Malaysia, South Africa, Germany, United Kingdom, United State of America and Taiwan. Emetere is involved in collaborative research with scientists from Nigeria, Malaysia, Greece, South Africa and Iran.
References
- AAA World Magazine. (2011, November–December). AAA World Magazine, p. 19.
- American Chemical Society. (2016). Gator in your tank: Alligator fat as a new source of biodiesel fuel. Retrieved March 18, 2016, from Science Daily: www.sciencedaily.com/releases/2011/08/110817094926.htm
- Anonymous. (2016a). Retrieved September 26, from http://biofuelsassociation.com.au/biofuels/biodiesel/biodiesel-and-my-vehicle/
- Anonymous. (2016b). Volvo/Mack engines are certified to comply with U – GSA. Retrieved September 26, from www.gsa.gov/portal/getMediaData?mediaId=210911
- Anonymous. (2016c). Retrieved September 26, from http://biodiesel.org/using-biodiesel/oem-information/oem-statement-summary-chart
- Antosiewicz, J. M., & Shugar, D. (2016). UV–Vis spectroscopy of tyrosine side-groups in studies of protein structure. Part 2: Selected applications. Biophysical Reviews, 8, 163–177.10.1007/s12551-016-0197-7
- ASTM. (2016). Retrieved September 26, from https://www.astm.org/Standards/D6751.htm
- Ayalasomayajula, S., Subramaniam, R., Gallo, A., Dufreche, S., Zappi, M., & Bajpai, R. (2012). Potential of alligator fat as source of lipids for biodiesel production. Industrial & Engineering Chemistry Research, 51, 2166–2169.10.1021/ie201000s
- Biofuels. (2016). BioFuel oasis. Retrieved September 26, from http://biofueloasis.com/faq/
- Boocock, D. G. B., Konar, S. K., Mao, V., & Sidi, H. (1996). Fast one-phase oil-rich processes for the preparation of vegetable oil methyl esters. Biomass Bioenergy, 11, 43–50.10.1016/0961-9534(95)00111-5
- Dansie, M. (2016). Bio-diesel in just 10 s. Retrieved October 3, 2016, from http://revolution-green.com/biodiesel-in-just-10-seconds/
- Eberhardt, W., Sham, T. K., Carr, R., Krummacher, S., Strongin, M., Weng, S. L., & Wesner, D. (1983). Site-specific fragmentation of small molecules following soft-X-ray excitation. Physical Review Letters, 50, 1038.10.1103/PhysRevLett.50.1038
- Emetere, M. E. (2016). Statistical examination of the aerosols loading over Mubi-Nigeria: The satellite observation analysis. Geographica Panonica, 20, 42–50.
- Emetere, M. E., Akinyemi, M. L., & Edeghe, E. B. (2016). A simple technique for sustaining solar energy production in an active convective coastal regions. International Journal of Photoenergy, 2016, 3567502.
- Emetere, M. E., Akinyemi, M. L., & Oladimeji, T. E. (2016a). Statistical examination of the aerosols loading over Kano-Nigeria: The satellite observation analysis. Scientific Review Engineering and Environmental Sciences, 72, 167–176.
- Emetere, M. E., Akinyemi, M. L., & Oladimeji, T. E. (2016b). A conceptual mathematical examination of the aerosols loading over Abuja-Nigeria. Communications in Mathematics and Applications, 7, 47–54.
- Haas, M. J., Scott, K. M., Alleman, T. L., & McCormick, R. L. (2001). Engine performance of biodiesel fuel prepared from soybean soapstock: A high quality renewable fuel produced from a waste feedstock. Energy & Fuels, 15, 1207–1212.10.1021/ef010051x
- Jha, M. K., Gupta, A. K., & Kumar, V. (2007). Kinetics of transesterification on jatropha curcas oil to biodiesel fuel. In Proceedings of the World Congress on Engineering and Computer Science 2007,WCECS 2007 (pp. 1–4). ISBN 978-988-98671-6-4.
- Korshavi, E., Shariati, A., & Nikou, M. R. K. (2016). Instant biodiesel production from waste cooking oil under industrial ultrasonic radiation. International Journal of Oil, Gas and Coal Technology, 11, 308–317.
- Kumar, S. (2016). Spectroscopy of organic compounds (pp. 1–36). Guru Nanak Dev University Lecture Note.
- Lewis, E. N., Lee, E., & Kidder, L. H. (2004). Near-infrared chemical imaging. Microscopy Today, 12, 6–11.
- Lucas, L. H., Ersoy, B. A., Kueltzo, L. A., Joshi, S. B., Brandau, D. T., Thyagarajapuram, N., … Middaugh, C. R. (2006). Probing protein structure and dynamics by second-derivative ultraviolet absorption analysis of cation-π interactions. Protein Science, 15, 2228.10.1110/ps.062133706
- Owen, T. (2000). Fundamentals of modern UV-visible spectroscopy (p. 51). Germany: Primer Publishing.
- RSC. (2016). Ultraviolet/visible spectroscopy—Royal Society of Chemistry. Retrieved October 3, 2016, from media.rsc.org/.../MCT4%20UV%20and%20visible%20spec.pdf
- Sebastian, J., Muraleedharan, C., & Santhiagu, A. (2016). A comparative study between chemical and enzymatic transesterification of high free fatty acid contained rubber seed oil for biodiesel production. Cogent Engineering, 3, 1178370.
- Thanh, L. T., Okitsu, K., Boi, L. V., & Maeda, Y. (2012). Catalytic technologies for biodiesel fuel production and utilization of glycerol: A review. Catalysts, 2, 191–222.10.3390/catal2010191
- Uno, U. E., Emetere, M. E., Fadipe, L. A., & Oyediji, J. (2015). Progress on mangiferaindica as dye sensitized solar cell. International Journal of Applied Engineering Research, 10, 17685–17694.
- Wada, S.-I., Kizaki, H., Matsumoto, Y., Sumii, R., & Tanaka, K. (2006). Selective chemical bond breaking characteristically induced by resonant core excitation of ester compounds on a surface. Journal of Physics: Condensed Matter, 18, S1629.
- Wada, S.-I., Sumii, R., Isari, K., Waki, S., Sako, E. O., S., Tetsuhiro, … Tanaka, K. (2003). Active control of chemical bond scission by site-specific core excitation. Surface Science, 528, 242–248.10.1016/S0039-6028(02)02639-0
- Westbrook, C. K., Pitz, W. J., Sarathy, S. M., & Mehl, M. (2013). Detailed chemical kinetic modeling of the effects of CC double bonds on the ignition of biodiesel fuels. Proceedings of the Combustion Institute, 34, 3049–3056.10.1016/j.proci.2012.05.025
- Yusoff, M. F. M., Xu, X., & Guo, Z. (2014). Comparison of fatty acid methyl and ethyl esters as biodiesel base stock: A review on processing and production requirements. Journal of the American Oil Chemists’ Society, 91, 525–531.10.1007/s11746-014-2443-0
- Zawadzki, A., Shrestha, D. S., & He, B. (2007). Biodiesel blend level detection using ultraviolet absorption spectra. Transactions of the ASABE, 50, 1349–1353.10.13031/2013.23612
- Zhou, W., Konar, S. K., & Boocock, D. G. V. (2003). Ethyl esters from the single-phase base-catalyzed ethanolysis of vegetable oils. Journal of the American Oil Chemists' Society, 80, 367–371.10.1007/s11746-003-0705-1