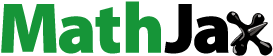
Abstract
This paper highlighted measuring the contact angle, the wetting heat and the IR spectrum before and after interactions between eight alkanes and coals to investigate the influence of the alkane structure and the number of carbon atoms on the coal surface hydrophobicity. The coal fines flotation tests were conducted with alkanes as collectors. The results show that after different alkanes acted on the coal samples, when the carbon numbers are same, both the contact angle and the wetting heat of isoparaffins were higher than those of the normal alkane. When the number of carbon atoms was 12, the contact angle and the wetting heat reached the maximum values after alkanes interacted with coals. The yield of the clean coal, the combustible material recovery and flotation perfect indicators of the isododecane were higher than those of the dodecane by 3.62, 4.08 and 0.96% respectively under the same condition of flotation. The ash content of the clean coal was also higher than that of the dodecane by 0.91%. This study shows that the collecting ability of the isoparaffin was superior to that of normal alkanes, while the flotation selectivity of the normal alkane was better than that of the isoparaffin.
Public Interest Statement
Fine coal is generally high in ash content and cannot be used directly. Flotation is one of the most important methods to reduce the ash content of fine coal. In order to improve the flotation efficiency, collectors need to be added to fine coal in the flotation process. The kinds of collectors are various, the aim of this paper is to find the optimum flotation collector. The study chooses eight kinds of different structure and different number of carbon atoms of the collector to research their flotation effect. The results show that the flotation effect was optimum when the number of carbon atoms of the isomeric alkane collector was 12.
1. Introduction
With the changes in geological conditions and improvement of coal mining mechanization degree, the proportion of the coal fines in the raw coal is increasing. The flotation, as one of the most effective methods for coal fines separation, draws more and more attention in the coal washing (Kong & Kang, Citation2011). In the flotation process, the wettability variation of coal and waste rock surface is expanded by flotation reagents to improve the selectivity and speed of flotation, and achieve the effective separation of coal and gangue (Li, Cui, Zhang, & Yang, Citation2010). In order to improve the flotation efficiency and gain better economic benefits, researchers focus more on the flotation reagent. Naik, Reddy, and Misra (Citation2005) studied the effect of three most important reagents for coal flotation, namely sodium meta silicate (regulator), kerosene (collector) and methyl isobutyl carbinol (frother). Hadler, Aktas, and Cilliers (Citation2005) found that an increase in the initial concentration of sodium isobutyl xanthate (SIBX), and an increase in the conditioning time yielded a higher concentrate grade by measuring the concentration of non-adsorbed reagents on a laboratory scale. Bradshaw, Oostendorp, and Harris (Citation2005) studied the performance of reagents and found that the collectors, namely sodium ethyl xanthate (SEX) and SIBX, were used to provide an increase in collector chain length to cause an increase in particle hydrophobicity. Erol, Colduroglu, and Aktas (Citation2003) carried out the froth flotation tests of bituminous coal fines using only one of the three non-ionic reagents; Triton x-100, Brij-35, methyl isobuthyl carbinol (MIBC) and sodium dodecyl sulfate (SDS), and the mixture of Triton x-100 or Brij-35 with MIBC in various ratios and found that the combustible solid recovery and the ash rejection strongly depended on the finer particle fraction in the feed. Jia, Harris, and Fuerstenau (Citation2002) studied tetrahydrofuran (THF) series collectors and found that the tetrahydrofuran connecting with fatty chain agent was suitable for the coal flotation with high oxidation degree and less aromatic content. The research by Sis, Ozbayoglu, and Sarikaya (Citation2003) showed that relatively low ash clean coal with high yield could be obtained by the flotation of high ash coal with the Pamak anionic collector. Kang, Hu, and Wang (Citation2014), Kang, Liu, and Zhang (Citation2011), Kang and Wang (Citation2014) and Xun, Kang, and Liu, (Citation2012) used linear alkanes, cycloalkanes, aromatics and the surface active agents to prepare composite agent as the collector for the flotation of the difficult-to-float coal. The study found that the collector composite had the advantages of higher floatation speed and stronger collecting ability and it might be a kind of ideal collector for difficult-to-float coals. Flotation effects of the different molecular structure of coal flotation reagents were compared. The C12 collector developed by Zhao, Wu, and Ma (Citation2010) with non-polar hydrocarbon oil, fatty acids, fatty ketones and surface active agent was applicable to the flotation of coarse coal. Shi, Li, Ma, and Zhang (Citation2009) used the wash oil as the collector with the mixture of aromatic hydrocarbons as its main component and showed that the wash oil had the high collecting ability and certain foaming properties compared with the light diesel oil. Chen, Song, Cao, Feng, and Huang (Citation2011) and Huang et al. (Citation2012) studied the components of waste tyre pyrolysis oil and found that a large number of alkanes, aromatics, olefins and aromatic substances, and some amide polar substances contained in it made the pyrolysis oil have two characters of collecting and foam properties, thus it could be used as a composite flotation agent. The study by Zhou, Qi, Liu, and Ning (Citation2007) showed that the emulsified oil made of the hydrocarbon oil, emulsifier and water compared with the light diesel oil, made the flotation perfection degree higher and the total sulfur removal rate reached 40%. The above mentioned literature focused more on the preparation of compound reagents with alkanes, olefins, aromatic hydrocarbons, polar functional groups and surface active agents and the flotation results, while only a little information is available on the collecting ability of flotation reagents of alkanes.
In coal fines flotation, non-polar hydrocarbon oil is the main collector, and the collecting ability of alkanes, olefin, aromatic hydrocarbons are different. The same kind of alkanes may have different flotation effects because of different structures and numbers of carbon atoms. This paper discusses the impact of alkane structure and the number of carbon atoms on the effect of coal flotation with eight alkanes including the normal alkanes and isoparaffins (naphthenic) as collectors.
2. Preparation and testing method
2.1. Test preparation
The coal sample was obtained from Qitaihe Xinxing Coal Mine in Heilongjiang Province, China. The size is less than 0.5 mm. The analysis of the coal is shown in Table .
Table 1. Coal sample analysis
According to the standard coal classification in China, the coal sample from Qitaihe Xinxing belongs to the 1/3 coking coal.
The tested collectors are: decane, dodecane, tridecane, tetradecane, isodecane, isododecane, isohexadecane, cycloalkane. Among them the cycloalkane is a mixture composed of cyclopentane, cyclohexane and its homologues, with the carbon number ranging from 5 to 10. The C6 naphthenic mass fraction accounts for more than 70%, so the C6 (cyclohexane) can be on behalf the naphthenic carbon atom number. In the paper, the cycloalkane was classified into isoalkanes and the secondary octyl alcohol served as a frother.To determine the effect of different collectors, not only the yield of the clean coal (γj) and the coal ash (Aj), but also the coal combustible recovery rate (ε) and flotation perfect index (ηωf) should be calculated (Zhou et al., Citation2007). The calculation formula for the clean coal yield is:(1)
(1)
where γj is the clean coal yield, Gj is the flotation clean coal weight and Gr is the flotation feed weight.
The calculation formula for the ash content is:(2)
(2)
where Aj is the ash content of the clean coal, G1 is the residual weight after burning and G is the coal sample weight before burning.
The calculation formula for the recovery of combustible material is:(3)
(3)
where ε is the combustible material recovery rate of the clean coal, γj is the clean coal yield, Aj is the ash content of the clean coal and Ay is the ash content of the raw coal.
The calculation formula for the flotation perfect index is:(4)
(4)
where ηωf is the flotation perfect index, γj is the clean coal yield, Ay is the ash content of the raw coal and Aj is the ash content of the clean coal.
2.2. Test method
2.2.1. Flotation test
The Model RK/FG50-100 hanging groove flotator with a 200 ml flotation tank was used in the tests. The scheme of the device is shown in Figure . About 20 g of the coal sample was used in each test. The concentration of pulp was 100 g/L. The dosage of the collector was 1.136 kg/t and the dosage of the frother was 0.114 kg/t with reagent proportion of 10:1. Firstly, the coal sample was mixed with water for 2 min. Then, the collector was added and mixed for 1 min. And then, the frother was added and mixed for 10 s. The air inlet switch was opened and the froth was collected for 3 min. Finally, the obtained coal samples were filtered and dried in the air.
2.2.2. Determination of contact angle
The measurement instrument DCA21 was used for measuring the contact angle before and after the effect of alkanes on coal samples. The instrument has a high precision scale, by which the weight of the solid powder can be measured in real time. The M2 − t relationship curve was plotted by professional software. The contact angle between ethanol and the coal sample was zero. Firstly, it plotted the M2 − t relationship curve between ethanol and the coal sample, then the curve between water and the coal sample. The contact angle between water and the coal sample was calculated according to the two curves. The scheme of the device is shown in Figure . Nine 10 g coal samples were prepared into the pulp in a concentration of 100 g/l. Then 8 kinds of collectors were added to the pulp respectively and stirred for 2 min. The consumption of the collector was 1.136 kg/t. A 10 g coal sample without the collector was also stirred for 2 min as a control. The pulp was filtered and naturally dried for further use.
2.2.3. Determination of the wetting heat
The wetting heat of the coal sample and collectors were measured by the C80-II Setaram micro calorimeter, which has both a measurement pool and a reference pool. Both the measurement and the reference pool have an upper room and a lower one. The upper room and the lower room are separated by a plastic film. The coal sample was arranged in the lower room of the measurement pool, and the upper room of it was filled with a wetting liquid. Only the upper room of the reference pool was filled with the same wetting liquid and the lower room was empty. When the measurement and the reference pool reached thermal equilibrium, the plastic films between two rooms were pierced at the same time. Consequently, the upper wetting liquids entered the lower room respectively. Minerals in the measurement pool were mixed with the wetting liquid and the heat value was calculated by the system. The scheme of the device is shown in Figure . The eight collectors served as the wetting liquids. In each test, a 0.2 g coal sample and a 2 mL collector were needed. The initial temperature was set at 30°C and the constant temperature lasted for 2 h. Then wetting heat data were collected for 3 h.
2.2.4. Determination of spectrum
The infrared spectrum was measured by the MB103 Fu Liye transform infrared spectrometer before and after the effect of alkanes on the coal sample. The KBr pellet method was used with a spectral range of 4,000–500 cm−1, a resolution of 1–64 cm−1, a wavenumber accuracy of ±0.04 cm−1 and a wavenumber repeatability of ±0.01 cm−1.
3. Experimental results and analysis
3.1. Flotation test
Flotation tests with all the eight collectors were carried out. Conditions of flotation tests were illustrated in Section 2.2.1. Flotation test results are shown in Figures –.
As can be seen from Figures –, isoparaffin curves are all above normal alkane curves, that is, the clean coal yield, the combustible material recovery and the flotation perfect index by the isoparaffin flotation are higher than those by the normal alkane, which shows that the collecting performance of the isoparaffin is better than that of the normal alkane. But that the ash content of the clean coal by the isoparaffin flotation is higher than that by the normal alkane, showing that the flotation selectivity of the isoparaffin is worse compared with that of the normal alkane. In Figures – , when the number of carbon atoms was 12, the maximum value could be obtained, while the ash content of the clean coal was relatively low. It shows that the flotation effect is optimum when the number of carbon atoms was 12 for alkanes collectors. When the number of carbon atoms was 12, the clean coal yield, the combustible material recovery and the flotation perfect index by the isoparaffin flotation were higher than that by the normal alkane flotation by 3.62, 4.08 and 0.96% respectively and the ash content of the clean coal by the isoparaffin flotation was higher than that by the normal alkane by 0.91%.
3.2. Changes of contact angle after alkanes interacting with the coal
The magnitude of the contact angle reflected the degree of the wetted mineral surface. The smaller the contact angle, the more easily the mineral surface was wetted by water and the stronger the hydrophilicity. Conversely, the larger the contact angle, the more difficult the mineral surface was wetted, and the stronger the hydrophobicity. The contact angle of coal and water is shown in Figure . From Figure , we can see that the larger the contact angle is, the more difficult it is to be wetted by water, the better the floatability of coal is. The changes after the interaction of alkanes with coals in the size of coal contact angle can infer the collector performance (Wang, Li, & Zhao, Citation2004; Zhou, Yan, & Liu, Citation2012). The measurement result of the coal contact angle before alkanes acting on the coal was 85.76°. Measurement results of coal contact angles after alkanes acting on the coal are shown in Table . The molecular structure of alkanes is shown in Table .
Table 2. Contact angles after alkanes acting on coal
Table 3. Molecular structure diagram of alkanes
As can be seen from Table , after the alkanes interacted with the coal, the contact angle of the coal increased to some different degrees. With the same number of carbon atoms, the contact angle of the coal after interacting with the isoparaffin was larger, compared with the normal alkane. It shows that the isoparaffin can improve the hydrophobicity of the coal more than the normal alkane. No matter it was the normal alkane or the isoparaffin, when the number of carbon atoms was 12, the contact angle of the coal was greater, compared with the alkanes with same structures, but with other different numbers of carbon atoms. That is to say, the C12 alkane had the strongest ability to enhance the hydrophobicity of the coal surface. This agrees with flotation tests. When the hydrophobicity is the strongest, the floatability of coal is the best.
3.3. The wetting heat of alkanes interacting with the coal
The heat released by the interaction of the solid with the liquid is called the wetting heat, whose numerical value is used to characterize the wetting property of the solid–liquid system (Shen & Wang, Citation1997). The magnitude of the wetting heat can reflect the affinity strength of the solid and the liquid. Measurement results of the wetting heat of alkanes interacting with the coal are shown in Table .
Table 4. Wetting heat of alkanes interacting with the coal
According to the physical meaning of the wetting heat, the bigger the numerical value of the wetting heat is, the stronger the affinity of the liquid and the solid. In the flotation process, the greater the wetting heat value after the interaction of alkanes with coals, the firmer the combination of alkanes and coals, and the better alkanes spreading on the coal surface, then the hydrophobic property of the coal surface can be improved effectively (Xun et al., Citation2012). As can be seen from Table , the wetting heat of the isoparaffin with the number of carbon atoms such as 10 and 12 interacting with coals were higher than that of the normal alkane with the same number of carbon atoms. Therefore, the isoparaffin with the number of carbon atoms such as 10 and 12 was better than the normal alkane in improving the coal surface hydrophobicity. With the number of carbon atoms increasing, the wetting heat of the normal alkane and the isoparaffin at first increased and then decreased, and the maximum value appeared at C12. This agrees with the contact angle analysis reported as above mentioned.
3.4. Spectral study of alkanes interacting with the coal
Infrared spectroscopy is one of the effective means of studying the interaction between reagents and minerals and it can infer the effect of reagents and minerals, according to the changes of shape and intensity of mineral spectral peak before and after the interaction between reagents and minerals (Zhu, Citation2009). The infrared spectroscopy of alkanes interacting with the coal is shown in Figures and .
Figure 9. The infrared spectroscopy of normal alkane before and after actting on coal.
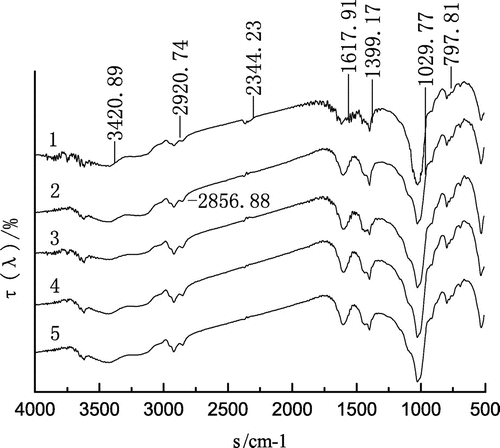
Figure 10. The infrared spectroscopy of isoparaffin before and after actting on coal.
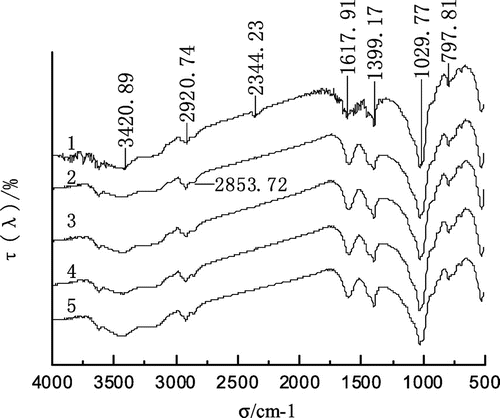
In Figures and , the infrared spectroscopy of the raw coal showed multiple sharp peaks in the range of 3,700–3,500 cm−1. The hydroxyl (–OH−) was in a free state. After the normal alkane and the isoparaffin interacted with the coal, the absorption peak of hydroxyl decreased and broadened. This showed that the hydrophobicity of the coal enhanced after the alkane had been adsorbed onto the coal surface (Lu & Du, Citation2003). As can be seen from Figures and , after the alkane interacted with the coal, not only was the intensity of the absorption peak at 2,920.74 cm−1 enhanced, but also the absorption peak of the asymmetric stretching vibration of –CH2 of cycloalkanes or fatty hydrocarbons became significant at the point of 2,856.88 and 2,853.72 cm−1. After the absorption peaks at 2,920.74–2,856.09 cm−1 in Figures and were compared, it could be seen that the absorption peak of the isoparaffin interacting with the coal sample was sharper than that of the normal alkane.
By the analysis of the IR spectra before and after alkanes was adsorbed to the coal sample, it can be seen that the alkane as a collector not only changed the hydrophilic groups on the coal surface to hydrophobic groups, but also could strengthen the alkyl hydrophobic groups on the coal surface, making the coal more hydrophobic. The isoparaffin has more side chains and occupies more space, so it was adsorbed more firmly onto the coal surface than the normal alkane and it also could increase the hydrophobicity of the coal more than the normal alkane.
3.5. Analysis of alkanes interacting with the coal
It can be seen from the flotation test results that the clean coal yield, combustible material recovery and flotation perfect index by the isoparaffin flotation were all higher than those by the normal alkane, but the ash content of the clean coal by the isoparaffin flotation was also higher than that by the normal alkane. Because the isoparaffin has more side chains, when the same molar mass of alkanes was adsorbed onto the coal surface, the area covered by the isoparaffin was larger than that covered by the normal alkane, thus the isoparaffin increased the yield of clean coal in the flotation, but meanwhile, the hydrophilic gangues were received easily (Xie, Citation2001). Therefore, the isoparaffin increased the clean coal yield, but in the meantime, it relatively decreased the collector selectivity and resulted in an increase in the ash content of the clean coal.
The collecting ability of alkane collectors for the coal depended not only on the molecular structure of the alkane, but also on its carbon chain length. The longer the carbon chain length of the alkane, the stronger its hydrophobic ability. But every time a –CH2 was added, the alkane viscosity increased accordingly (Ding, Citation1987). The alkane with a long carbon chain was not easily dispersed in water, so its collecting function declined. But if the carbon chain is too short, the hydrophobic ability of the alkane is weak. It may not improve the hydrophobicity of the coal and cannot obtain the optimal flotation effect. In this experiment, the flotation effect was optimum when the number of carbon atoms of the alkane collector was 12.
4. Conclusions
(1) | Either normal alkanes or isomeric alkanes, with the increase of the number of carbon atoms, the flotation perfect index increased gradually. The flotation perfect index reached the maximum value when the number of carbon atoms is 12. When the number of carbon atoms exceeds 12 or is too large, the perfect index of flotation decreased. | ||||
(2) | The studies on the contact angle, wetting heat and infrared spectrum of different alkanes before and after interacting with coal samples proved that the isoparaffin could enhance the hydrophobicity of the coal more than the normal alkane. | ||||
(3) | The alkanes collecting ability for coal not only depended on their molecular structures, but also on their carbon chain length. In this experiment, when the number of carbon atoms was 12, the contact angle and the wetting heat of alkanes interacting with the coal were the maximum and the flotation effect of alkanes was optimum. | ||||
(4) | In the same flotation conditions, the clean coal yield, the combustible material recovery and the flotation perfect index by the isododecane flotation were higher than those by the dodecane by 3.62, 4.08 and 0.96% respectively, and the ash content was also higher than that by the dodecane by 0.91%, so the collecting ability of the isoparaffin is superior to that of the normal alkane, while the selectivity of the normal alkane flotation was better than that of the isoparaffin. |
Funding
The financial support from China National Natural Science Fund [grant number 51144012] is gratefully acknowledged.
Additional information
Notes on contributors
Wenze Kang
Wenze Kang is a Professor at Heilongjiang University of Science and Technology, China. He holds a Dr (2006) degree in Mineral Engineering from China University of Mining and Technology. His interests are in non metallic minerals such as coal and graphite. He has had his more than 60 articles published in journals, such as Fuel Processing Technology, Mining Science and Technology, and other journals and has published three monographs textbooks.
Jun Hu
Jun Hu holds a ME (2013) degree in Mineral Processing from Heilongjiang University of Science and Technology. Her interest is coal flotation.
References
- Bradshaw, D. J., Oostendorp, B., & Harris, P. J. (2005). Development of methodologies to improve the assessment of reagent behaviour in flotation with particular reference to collectors and depressants. Minerals Engineering, 18, 239–246.10.1016/j.mineng.2004.09.012
- Chen, K., Song, C. A., Cao, X., Feng, D. X., & Huang, B. (2011). Experiment study on waste tyre pyrolysis to prepare collector of slime floatation. Coal Science and Technology, 2, 115–118.
- Ding, L. Q. (1987). The theory and practice of flotation (pp. 79–80). Beijing: Coal Industry Press.
- Erol, M., Colduroglu, C., & Aktas, Z. (2003). The effect of reagents and reagent mixtures on froth flotation of coal fines. International Journal Mineral Processing, 71, 131–145.10.1016/S0301-7516(03)00034-6
- Hadler, K., Aktas, Z., & Cilliers, J. J. (2005). The effects of frother and collector distribution on flotation performance. Minerals Engineering, 18, 171–177.10.1016/j.mineng.2004.09.014
- Huang, B., Gong, X. W., Dong, X., Pan, X., Shi, W. J., & Zhu, Y. (2012). Study on flotation performance of different fractions from waste tire pyrolysis oil. Coal Technology, 5, 112–114.
- Jia, R., Harris, G. H., & Fuerstenau, D. W. (2002). Chemical reagents for enhanced coal flotation. Coal Preparation, 22, 123–149.10.1080/07349340213847
- Kang, W. Z., Hu, J., Wang, & G. Y. (2014). Experimental study of slime flotation using dodecyl benzene and dodecane. Coal Preparation Technology, 2, 19–22.
- Kang, W. Z., Liu, S. Y., & Zhang, Y. G. (2011). Flotation experiment of scare difficult-to-float fine coals floated by collector AO. Journal of Heilongjiang Institute of Science and Technology, 2, 85–88.
- Kang, W. Z., & Wang, G. Y. (2014). Effect of reagents of different molecular structure on coal flotation. Journal of Heilongjiang University of Science and Technology, 4, 375–378.
- Kong, X. H., & Kang, W. Z. (2011). Study on effect of reagent mixtures in flotation of coal fines. Guangzhou Chemical Industry, 6, 50–52.
- Li, Y. J., Cui, G. W., Zhang, J. Q., & Yang, S. (2010). The current situation and development of coal slime flotation agent. Symposium of 2010 China National Coal Preparation Academic Conference, 154–156.
- Lu, C. X., & Du, H. G. (2003). Organic chemistry (p. 162). Beijing: Science Press.
- Naik, P. K., Reddy, S. R., & Misra, V. N. (2005). Interpretation of interaction effects and optimization of reagent dosages for fine coal flotation. International Journal Mineral Processing, 75, 83–90.10.1016/j.minpro.2004.05.001
- Shen, Z., & Wang, G. T. (1997). Colloid and surface chemistry (pp. 96–108). Beijing: Chemical Industry Press.
- Shi, C. S., Li, G. Z., Ma, R. X., & Zhang, Y. (2009). Study on wash oil collector to coal flotation results. Coal Engineering, 2, 80–82.
- Sis, H., Ozbayoglu, G., & Sarikaya, M. (2003). Comparison of non-ionic and ionic collectors in the flotation fines. Minerals Engineering, 16, 399–401.10.1016/S0892-6875(03)00047-5
- Wang, B. J., Li, M., & Zhao, Q. Y. (2004). Relationship between surface potential and functional groups of coals. Journal of Chemical Industry and Engineering (China), 8, 1329–1333.
- Xie, G. Y. (2001). Study of mineral processing (pp. 435, 440, 516). Xuzhou: China University of Mining and Technology Press.
- Xun, H. X., Kang, W. Z., & Liu, S. Y. (2012). Study on collecting results of AO to scarce and difficult floated coal slime. Coal Science and Technology, 8, 118–120.
- Zhao, Y. Z., Wu, Z. Y., & Ma, Z. S. (2010). Application study of new collector C12 in Jinyang coal preparation plant. Coal Preparation Technology, 6, 18–20.
- Zhou, C. C., Yan, B., & Liu, J. T. (2012). Effects of the structure of aromatic collectors on flotation performance. Journal of China University of Mining and Technology, 1, 82–85.
- Zhou, H. W., Qi, X., Liu, B. S., & Ning, W. R. (2007). Experimental research on emulsive collector for coal flotation. Coal Quality Technology, 4, 52–54.
- Zhu, Y. (2009). Research on XY complex flotation reagents preparation and application. Harbin: Heilongjiang Institute of Science and Technology.