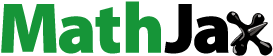
Abstract
This study presents the preparation and characterization of chemically activated carbonaceous materials obtained from waste tyres and the prepared adsorbent was applied for the adsorptive removal of Cd(II) and Pb(II) in the domestic wastewater samples. The activation efficiency of phosphoric acid (H3PO4) and hydrogen peroxide (H2O2) were evaluated and H2O2 was chosen as the appropriate activating agent as compared to phosphoric acid. The developed adsorbent was characterized using the scanning electron microscope (SEM), Brunnuer Emmet Teller (BET) which helped to decide which adsorbent material is best in terms of the surface area and Fourier transform infrared spectroscopy (FT-IR). Two-level fractional factorial design was used for the optimization of parameters affecting the adsorptive removal of Cd(II) and Pb(II). The optimum conditions were found to be 6.5, 0.2 g, 32.5 min and 55 mg L−1, for sample pH, mass of adsorbent, contact time and initial concentration, respectively. Amongst every adsorption isotherms that were used, Langmuir model was preferred due to the highest value of the correlation coefficient (r2). Therefore, the adsorption capacities of Cd(II) and Pb(II) were 201 and 196 mg g−1, respectively. The regeneration studies were performed and the adsorbent was capable of been used ten times and the material was stable with only a slight decrease in the removal efficiency of Cd(II) and Pb(II). Furthermore, the prepared adsorbent prepared was successfully applied for the removal of Cd(II) and Pb(II) in real environmental samples and the inductively coupled plasma optical emission spectroscopy was used for the analysis.
Public Interest Statement
Africa is facing serious challenges with outbreaks of deadly diseases that originates from consumption of unclean water. However, water is an essential resource that almost everything on earth depends on. The transparent colour of water does not mean it is not contaminated. The need of clean water necessitates research that helps with the understanding of adsorbents (material designed to remove pollutants in water) that are economic and greener for simple removal of pollutants in water. In this study, motor vehicle rubber tyres are treated for the extraction of carbon material that is beneficial in removing contaminants in water. This work was encouraged by the fact that tyres that are worn out, thrown away and eventually becomes a health hazard to the environment. Therefore, the outcome of this work showed that waste tyres can be of good use to clean water and to avoid throwing away tyres that pollutes landfills.
1. Introduction
Environmental pollution is a major challenge in the entire world (Fu & Wang, Citation2011). In recent years, contamination of the environment (water, soil and air) by persistent pollutants from different activities has become an increasingly serious problem (Fu & Wang, Citation2011). Toxic pollutants such as cadmium and lead are released from various activities such as mining operations, agricultural runoffs and paint industries, among others (Chan, Cheung, & McKay, Citation2012; Fu & Wang, Citation2011). In ensuring that the lives of flora and fauna are safe, proper techniques for the removal of various toxic contaminants from the environment are required (Fu & Wang, Citation2011). However, it is usually a daunting task for many industries to find an economically feasible method to remove contaminants such as trace metals from the environmental samples (Fung, Cheung, & McKay, Citation2012). On the other hand, less research has been conducted and reported on different wastewater samples generated from the wastewater treatment plants (Robles-Molina, Gilbert-López, García-Reyes, & Molina-Díaz, Citation2013). The purpose of wastewater treatment systems are to eliminate toxic pollutants and to maintain the cleanliness of water to protect the environment and humans and organisms that depend on it (Muga & Mihelcic, Citation2008). Industrialization has a serious negative impact in the environment, this is evident due to the slow deterioration of the environment and most importantly the quality of water (Chan, Chong, Law, & Hassell, Citation2009). Most WWTPs are not designed to remove trace elements, for this reason, effluent discharges with relatively high levels of trace metals are released into rivers and oceans. Therefore, it of paramount importance that trace metals should be constantly monitored and removed from the environment most especially in the WWTPs that releases wastewater to different water bodies.
Many techniques that are extensively employed to remove trace metals include ion-exchange, adsorption, membrane filtration, chemical precipitation, and chemical treatment technologies (Al-Saadi, Saleh, & Gupta, Citation2013). Amongst the abovementioned techniques, the adsorption techniques are usually preferred because of their flexibility in operation and design. Adsorption techniques are known to be effective methods for removing toxic contaminants (Al-Saadi et al., Citation2013). Adsorbents such as carbon nanotubes and activated carbon have been largely explored by scientist for the removal of toxic contaminants in the environment (Al-Saadi et al., Citation2013).
In the past decades, an extensive research studies have been conducted to prepare activated carbon because of their usefulness in terms of application, more especially as adsorbents (Acevedo & Barriocanal, Citation2015). Over the past few years, a decrease in accessibility and mass production expenditure has led to increments in prices of commercial activated carbon (Chan et al., Citation2012). Currently, the utilization of waste materials that have, takes an increasing importance (Betancur, Martínez, & Murillo, Citation2009). The most common waste materials used for the production of low-cost adsorbents includes fly ashes, sawdust, paper mill sludge, agricultural by-products and scrap tyres (Acevedo, Citation2015; Gupta, Nayak, Agarwal, & Tyagi, Citation2014; Min & Harris, Citation2006). Used tyres is an alternative source of activated carbon due to the carbon content it has (Min & Harris, Citation2006). Waste tyres accounts for about 2% of the overall amount of waste. Furthermore, they are of concern in developed and under-developed countries due to the environmental problem (pollution) they may generate through inappropriate management (López et al., Citation2009). Therefore, the conversion of waste tyres to a useful carbonaceous material is beneficial to the environment because it can be used as adsorbents for removal of toxic trace elements (Belgacem, Belmedani, Rebiai, & Hadoun, Citation2013).
The aim of the research project study was to prepare the chemically activated carbon material and to characterize carbonaceous materials obtained from waste tyres. The prepared tyre-based activated carbon was applied for the adsorptive removal of Cd(II) and Pb(II) in wastewater (influent and effluent) collected from the domestic WWTP. The factorial design statistical analysis software was utilized to optimize the variables affecting the adsorptive removal of Cd(II) and Pb(II). The chosen parameters that are most likely to affect the results were contact time, mass of adsorbent pH and initial concentration for the adsorption of Cd(II) and Pb(II) in wastewater samples.
2. Experimental
2.1. Sampling-study area
The waste tyre was collected from the landfill in a stockpile near Lenasia (South of Gauteng). Only the sides of tyres were cut from the whole tyre since the side-walls are thread-free. Wastewater samples were obtained from a WWTPs facility (Northern Works (domestic)) which receives wastewater from the neighboring households across the city (Johannesburg, Gauteng). The raw (influent) and treated (effluent) wastewater samples were collected and refrigerated upon arrival in the lab. The lower, middle and upper stream (river water) sampling points were chosen as surface water collected from Soweto (Johannesburg).
2.2. Apparatus and instruments
The digital hot plate (DigiBlock ED36S, LabTech Africa (South Africa)) was used as heating sources for the activation of the carbon powder in this study. The inductively coupled plasma optical emission spectrometer (Thermoscientific (iCAP 6500 duo)) was used to determine trace metals. The ultrasound sonicator (BRANSON 5800, Lasec (South Africa)) was used for adsorption studies. The pH of solutions was tested using a pH meter of (Mettler-Toledo FE20, Switzerland). The solid phase extraction experiments were carried out using the VacMaster-24 sample SPE station (Biotage®, Uppsala, Sweden). The tubular electric furnace was used to perform the pyrolytic process under inert conditionsusing nitrogen.
2.3. Materials and reagents
The chemical activation process was performed using acid reagents which are, H3PO4 (85%) supplied by Sigma Aldrich (Fluka) and H2O2 (30%) which were purchased from Sigma Aldrich (Fluka). Acids such as HNO3 (69%), and HCl (37%) were also purchased from Sigma Aldrich were used to adjust the pH of samples and 10% HCl was prepared from HCl (37%), which was used for removing unwanted metals in the prepared material. The pH of were adjusted with 1.0 mol L−1 NaOH and 1.0 mol L−1 HNO3. The stock solutions of Cd(II) and Pb(II) were prepared from lead nitrate (Pb(NO)3, and cadmium from CdCl2 salt purchased from Sigma Aldrich. The wastewater standard reference material (CWW-TM-B) purchased from High-Purity Standards, Charleston, SC, USA. The acrodisc membrane cellulose acetate (Separations Scientific SA (Pty) Ltd) filters (0.45 um) were used to separate the activated carbon powder material from model solutions and wastewater samples. The SpexCertiPrep company (Industrial Analytical (Pty) Ltd, South Africa) supplied a multi-elemental standard solution containing Pb and Cd elements.
2.4. Pretreatment of waste tyre
The raw waste tyre was thoroughly washed with a detergent and rinsed with deionised water to ensure that there is no detergent or soil particles’ remaining on the surface of the tyre. Thereafter, the tyre material was oven-dried at 100°C. Small pieces of tyres were cut using a sharp knife before inserting them into an electric tubular furnace to obtain a powder with different particle sizes, under a continuous purging with nitrogen (Inert condition). The pyrolysis process was utilized to obtain the raw carbon powder at the temperature of 900°C. The powder was then made uniform by milling the particles to the same size (100–250 μm). This entire pyrolysis procedure was a standard method (no optimization) for obtaining the raw carbon powder (char) that will be further activated using different conditions.
2.5. Chemical activation of carbon
The preference of chemical activation over the physical activation has been well documented due to the porosity, pore size and the large surface area of the material after activation (Mui, Ko, & McKay, Citation2004).The disadvantage of physical activation is that it time and energy consuming. For this reason, chemical activation process is considered an alternative process to activate raw carbon from waste tyres. Chemical activation has an advantage over physical activation because it allows both pyrolysis and activation to occur in a single process (Mui et al., Citation2004). During the chemical activation, activating agents such as KOH, NaOH, HCl, H2SO4 have been extensively used to enhance the activation process (Fung et al., Citation2012) but in this study hydrogen peroxide (H2O2) will be explored due to its environmental friendly properties and it will be compared with phosphoric acid (H3PO4).
The chemical activation procedure was optimized by varying factors that can affect the production and effectiveness of the activated carbon. Factors such as temperature and time were fixed at 200°C and 2 h, respectively. Activating agents (acidic chemicals) and different ratios of raw carbon (fixed) to activating agent (varied), is important in obtaining attractive properties of the adsorbent (activated carbon) that are suitable in adsorbing inorganic pollutants. The most important aspect of the optimization was the choice of the activating agents (acidic chemicals) which were used to impregnate the raw carbon with, which is phosphoric acid (H3PO4) and hydrogen peroxide (H2O2). The temperature at which the activation was performed was set at 200°C using the digital block digestor. The ratios between the raw carbon and the activation agents were 1:5 and 1:10 but the raw carbon was fixed at 0.3 g. After the activation process, the end product (activated carbon) was allowed to cool down for a few minutes and it was mixed with 10% HCl to remove the inorganic impurities. The excess phosphoric acid in the adsorbent was eliminated with hot water and the hydrogen peroxide with cold water.
2.6. Multivariate optimization of influential factors for the application of activated carbon
The multivariate chemometric approach was used to minimize the experiments and to assess factors interaction and also investigate the most influential effects (Divrikli, Akdogan, Soylak, & Elci, Citation2009; Soylak, Narin, Bezerra, & Ferreira, Citation2005). The optimization process was performed using a two-level (24) fractional factorial design (Minitab 17 software). Four experimental variables affecting the adsorption of metals to the prepared adsorbent that include, pH, initial concentration, contact time, of the model solutions and the mass of the adsorbent added to the solution. Factors and levels (minimum, central point and maximum) of experimental designs used for the optimization for adsorption studies are presented in Table .
Table 1. Factors and level used in the factorial design
2.7. Characterization of the prepared activated carbon
The chemical activation process was chosen as the sample activation methods for attaining desirable properties of the activated carbon from waste tyres. The prepared adsorbent will be characterized using thescanning electron microscopy (Nano 2000 High ResolutionSEM (HRSEM), Jeol-Japan)to provide visual information of the top surface as well as cross-sectional morphology of the raw and activated carbon powders. The unmodified, modified activated carbonwill be studied to observe the difference in morphology. The Brunauer-Emmett-Teller (BET) was used to understand properties such as pore volume, pore size, surface area and distribution of powdered materials that are of utmost importance in many applications because it determines both reactivity and adsorptive ability of the activated carbons. The properties of the activated carbon will be studied by using Micromeritics ASAP 2020 surface and porosity analyser by N2 adsorption. Lastly, The fourier transform Infrared spectroscopy (Perkin Elmer 100 Spectrophotometer, United Kingdom) will be utilized to identify or confirm the presence of oxygen containing functional groups, hydroxyl, carbonyl and carboxylic groups and chemical bonds in a sample. The activated carbon was mixed with potassium bromide (KBr) at a ratio of 1:15 before characterization.
2.8. Adsorption/batch studies
The application of the prepared activated carbon on model solutions and wastewater was performed on a batch scale using the ultrasound sonicator. Eleven experimental runs were performed for each metal, of which in total 22 runs were performed according to Table . A model solution of 50 mL was placed in the ultrasound sonicator with the adsorbent in the solution under different conditions, according to the design matrix from the two level fractional design. The adsorption of metal ions by the developed adsorbent was calculated by subtracting the remaining concentrations of the metal ions in solution from the initial concentration.(1)
(1)
Where C0 and Ce are the initial and equilibrium concentrations of the metal ions in solution (mgL−1), respectively, V is the volume of the aqueous solution (L), and M is the mass of activated carbon used (g).
The trace metal percentage removal (R) is denoted by Equation (1), which determines the efficiency of the adsorbent. Ci is the initial concentration and Cf is the final concentration after adsorption.
2.9. Analysis of trace metals
The metals of interest in this study were lead and cadmium which are deemed toxic at high levels in wastewater and surface water. The concentration of the mentioned metals was determined by ICP-OES.
3. Results and discussions
3.1 Selection of the suitable activating agent according to surface properties
The chemical activation process was carried out using the digiblock (digital digestor block) for evaluatingthe effectiveness of H2O2 and H3PO4 in activating the carbon from waste tyres. The comparison between the H2O2 and H3PO4 was evaluated using the parameters such as time, temperature and the ratio of the raw carbon (fixed) to the varying volumes of activating agents. About four combination as shown in Table were prepared to evaluate the performance of the activating agent at different conditions.
Table 2. Selection of the suitable activating agent
The decision regarding which combination was the best amongst the four that were tested, was based on the BET results. The BET results (Table ) presents the activated carbon properties such as pore volume surface area, and pore size. These properties were used to select the suitable combination. The micropore volume, surface area, mesopore volume and total pore volume were evaluated according to different impregnation (activation) ratios shown in Table . These results (Table ) were used to determine which combinationobtained good surface properties that can be usedfor the selection of the appropriate acidic chemical (H2O2 and H3PO4) during the chemical activation process. According to the results, combination (WT-AC-4 (1:10)) was found to have the highest surface area compared to the others. The inclusion or rather the activation of the raw carbon by H2O2 proved to be more efficient than the activation with H3PO4. The doubling of the acidic chemicals played a role in obtaining good properties, however, it was evident that the raw carbon was greatly improved with regards to the inclusion of H2O2 at ratios of 1:10 (adsorbent: acid). The reason might be that with the increment in impregnation ratio, the activating agent percolates the interior of the particle of the developed tyre-based activated carbon to a point at which no further reaction could take place (Olorundare, Krause, Okonkwo, & Mamba, Citation2012; Olorundare, Msagati, Krause, Okonkwo, & Mamba, Citation2014). Similar phenomenon was observed for (WT-AC-4 (1:10)), the measurement of the total pore volume was measured from the nitrogen adsorption data as the volume of liquid nitrogen. The tyre-based activated carbon was found to be mesoporous, the mesopore volume was obtained by the difference between the total pore volume and micropore volume (Olorundare et al., Citation2012). The total pore volume and surface area the showed to increase with increasing in the impregnating ratios, especially for H2O2 as an activating agent. However, the other methods exhibited low porosity possibly because of the contraction of carbonaceous structures (Olorundare et al., Citation2012). Therefore, the combination with sample name WT-AC-4 (1:10) was used for further investigation.
Table 3. Surface and pore structure of the developed tyre-based activated carbon
3.2. Characterization of the adsorbent
The carbon based materials were further characterized to evaluate a number of parameters such the surface morphology, pore size distribution, type of material and surface functional groups that are present due to the chemical activation process that was carried out. The abovementioned properties were investigated by employing characterization techniques such as SEM and FT-IR.
3.2.1. Scanning electron microscope (SEM)
The morphology of the surface of activated carbon is shown in Figure . According to the Figure (a), showing the micrograph of a raw carbon exhibits a slightly porous morphology structure with small pores of various shapes and sizes. The porous morphology of the activated carbon (decorated) (Figure (b)), shows that indeed the activation using H2O2 plays a critical part in improving the properties of the raw carbon in terms of the highly increased porous structure. The porosity of the adsorbent was evident on the outer surface that is full of cavities, proving to have high surface area with more or less uniform pore distribution and the size and shape of the pores are the same. The uniform structures of the produced activated carbon are mesoporous and microporous in nature.
3.2.2. Fourier transform infrared spectroscopy (FT-IR)
The spectra of FT-IR (figure not included) for raw and activated carbon were compared. The difference was quite evident as the raw material showed less functional group in terms of peaks/spectrum as compared to the hydrogen peroxide-activated carbon. The FT-IR spectrum of the preparedactivated carbon was generated to identify the possible functional groups, especially the oxygen functional group that are expected to uptake the trace metals (Cd(II) and Pb(II)) of interest in water. The range at which the spectrum was generated was from 4,000 to 450 cm−1. It was discovered that peaks where mostly centered around 3,400, 1,700, 1,627 and 1,580 cm−1which were due to the presence of the OH group of phenols and C-O bonds (Al-Saadi et al., Citation2013). At 3,400 cm−1 the band that appeared is associated with O-H vibrations of the hydroxyl groups (Al-Saadi et al., Citation2013), the appearance of the peak at 1,700 cm−1 is characteristic of stretching vibrations of the carboxyl groups, which substantiates the occurrence of C-O. The aromatic skeletal stretching vibrations were observed at peaks, 1,627 and 1,580 cm−1. The peak (1,180 cm−1) revealed the presence of the carbonyl to an ester.
3.3. Optimization strategy
The optimization strategy was carried out by evaluating factors that can possibly affect the performance of the prepared material for the removal of Cd(II) and Pb(II). The two-level factorial (fractional) design was utilized as a statistical tool for optimization of factors involving central points with three replicates. Factors such as concentration of the adsorbate, pH of the solution, mass of the adsorbent and the contact time, were considered asmain factors affecting the removal percentage (analytical response) of Cd(II) and Pb(II). The temperature was fixed at temperature (25°C) using the ultrasonicator. The experimental runs were performed according to the design matrix shown in Table . The results of the design matrix were reported in terms of the removal percentage of both Cd and Pb. According to the results in Table , experimental number 9, 10 and 11 (center points), gave relatively quantitative removal percentages of Cd(II) and Pb(II).
Table 4. The design matrix and the results of the two-level (24−1) fractional factorial design
The highest removal percentage of Cd(II) and Pb(II) were ranging at 86–90%, hence it was chosen as the effective combination for the removal of Cd(II) and Pb(II). The results of the factorial design were further evaluated using the analysis of variance (ANOVA) at 95% confidence level presented in terms of Pareto charts (Figure ) (Fung et al., Citation2012). Figure (a), presents the effects of factors and their interactions in the removal of Cd(II) and Figure (b) explains the effects of factors in removing Pb from water. Factors such as IC, pH, CT and the interaction of IC and pH showed to a negative effect in the % Removal (response) of Cd(II) and Pb(II). Figure (a) also confirms the significance of the pH value having a negative effect, which means that an increase in pH leads to a decreasein metal removal percentage and this is also evident by observing the dotted reference line that cuts through the pH bar. The length of the bar in all presented Pareto charts represent the relative importance of comparative effects which are proportional to the absolute value of the estimated effects (Nomngongo & Ngila, Citation2015). The large negative effect of the pH on the analytical response implies that, the pH of the solution should decrease from a higher level to the middle or lower level. These findings are true because at high pH, values metal ions tend to complex in different matrices and form precipitates and this will lead to the metals not being adsorbed by the prepared adsorbent. The contact time, initial concentration and their interaction (Figure ) showed to have an insignificant effect in the adsorption of both metals, suggesting that the time it takes for the adsorbent to take-up metals can be possible in a matter of few minutes. The combination of pH 6.5, concentration (55 mg L−1), contact time of 32.5 min and mass of adsorbent (0.2 g), proved to be the effective combination of factors to remove Cd(II) and Pb(II) in water.
3.4. Adsorption capacities of metal ions and the regeneration of the adsorbent
The adsorption capacity is an essential part of adsorption studies in order to get a good understanding with regards to the relationship of an adsorbent and an adsorbate. The adsorption capacity for each metal was investigated using optimum conditions. The prepared activated carbon was applied on ten (ranging from 10 to 400 mg L−1) different concentration levels in a 50 mL of Cd(II) and Pb(II) solutions. The adsorption capacity was performed using a sonicator and the metals were determined by ICP-OES.(2)
(2)
where Ce is the equilibrium concentration of metals in solution, qe (amount of metals adsorbed), qmax (the maximum capacity of adsorption, mg g−1) and KL (constant related to affinity of the binding sites, Lmg−1) is the Langmuir isotherm constant.
The Langmuir and Freundlich linear isotherm models were used to explain adsorption behavior of the analyte onto the adsorbent, however, two isotherms were compared according to the correlation coefficient (r2). When the experimental data was fitted into both equations, Langmuir equation presented in Equation (2) gave the r2 value (r2 = 0.98) that is better than the Freundlich isotherm (r2 = 0.84) (equation not shown). The adsorption capacity of analytes (Cd(II) and Pb(II)) were calculated. The results obtained from a model assume a uniform adsorption due to the uniformity of the adsorbent surface. Therefore, the maximum adsorption capacities of Cd(II) and Pb(II) were determined to be 201 and 196 mg g−1, respectively.
3.5. Regeneration of the adsorbent
The regeneration study was investigated to check the reusability of the prepared material in adsorbing Cd(II) and Pb(II), using optimum conditions. This study was performed using the solid phase extraction (SPE) and ten cycles for each metal (Cd(II) and Pb(II)) were ran and analyzed with ICP-OES. The retained metals were flushed with 3 mol L−1 HNO3. However, the optimized activated carbon was able to be reused ten times and the regeneration was stable with only slight decrease in the removal efficiency of Cd(II) and Pb(II). The developed activated carbon showed more stability with Cd(II) adsorption compared to Pb(II).
3.6. Validation and application of the optimized tyre-based activated carbon in wastewater
The developed activated carbon method was validated by applying the adsorbent for the analysis of a wastewater SRM (CWW-TM-B) to remove Cd and Pb. The results shown in Table displayed satisfying removal efficiency as compared to the certified values of the standard reference material (SRM) for Cd and Pb. The percentage of the removal efficiency was 99 and 98% for Cd(II) and Pb(II) respectively. The outcome of the validation confirmed that the developed activated carbon can be used to remove metals in known certified concentrations. In addition, these results proved that the activated carbon is a suitable adsorbent for removing Cd(II) and Pb(II) in water samples and has a potential to be applied in real samples.
Table 5. Validation and application of the optimized tyre-based activated carbon in domestic wastewater and surface water
The carbon adsorbent was applied in influent and effluent wastewater collected from the WWTP and surface water collected from Soweto (township in Gauteng) for the removal of Cd(II) and Pb(II). The results obtained are presented in Table . The wastewater (influent and effluent)and surface water was first treated (digested) using the digital hotplate with the dilute HNO3 in order to break the metal free from sample matrix. Wastewater (influent and effluent) and surface water were firstly thoroughly analyzed to understand the content of the samples in terms of levels of Cd(II) and Pb(II) in the WWTP and surface water. The %removal (Eqn 1) of trace metals were calculated by subtracting the remaining concentrations of the metal ions in solution from the initial concentration. Thus, according to the results in Table , the optimized tyre-based activated carbon demonstrated a potential to be successfully used for the removal of Cd(II) and Pb(II)in wastewater and surface water samples.
4. Concluding remarks
In this study, the waste tyre-based activated carbon was successfully developed and exhibited great effectiveness in the removal of Cd(II) and Pb(II) in wastewater. For optimization purposes, factors such as pH, contact time, mass of adsorbent and concentration of the solution were effectively studied using the two level fractional factorial design. The prepared tyre-based activated carbon was characterized by SEM, BET and FT-IR to confirm the modification of the raw carbon employing the chemical activation using the H2O2 as the activating agent over H3PO4. A decision was made from the detailed infrared spectrum, that the prepared tyre-based activated carbon material includes oxygen-containing functional groups that provide additional active sites for the adsorption process. The study of adsorption capacity of the activated carbon using Langmuir model was preferred due to the highest value of the correlation coefficient (r2). The prepared activated carbon was validated and successfully applied for the removal of Cd(II) and Pb(II) in influent and effluent wastewater collected from the wastewater treatment plant and surface water collected from Soweto (township in Gauteng). In removing Cd(II) and Pb(II), the developed adsorbent was evaluated in terms of %removal of Cd(II) and Pb(II) in influent, effluent and surface water samples. It turned out that the %removal of both metal ions in influent and effluent wastewater were less than 90% as compared to surface water samples. In terms of precision (repeatability) the percentage RSD for both metals was satisfactory with values less than 4%. Therefore, according to the obtained results, metal ions from surface water were easily removed (due to the complexicity of the sample) by the tyre-based activated carbon as compared to wastewater collected from the WWTP. The ability of the prepared adsorbent proved to be efficient in removing Cd(II) and Pb(II) from domestic wastewater (influent and effluent) and surface water.
Funding
This work was supported by the National Research Foundation (NRF) [grant number SFH14073184214] and South African Agency for Science and Technology Advancement [grant number SFH1407318421].
Acknowledgement
The authors would like to thank University of Johannesburg for making this study possible by making laboratory facilities available.
Additional information
Notes on contributors
K. Mogolodi Dimpe
The research group that Philiswa N. Nomngongo is currently working under the analytical/environmental chemistry research group. Our group focuses on the method development; application of different adsorbents to remove pollutants, the use of sample preparation techniques that helps in the preconcentration of samples that are low in concentration to be enable the sensitive accurate quantification of different pollutants. The application of the current material in real samples will be tested to investigate its performance in the bench scale and ultimately the large scale. The reported research is in line with other research projects in a sense that all projects are more analytical and the use of greener adsorbent to alleviate and to free the environment from toxic pollutants. The key research activities of analytical/environmental chemistry research group deals with the identification of the problem, quantification of pollutants and eventually to mitigate the problem by implementing the developed techniques and materials in a more simpler and effective way.
References
- Acevedo, B. (2015). Properties and performance of mesoporous activated carbons from scrap tyres, bituminous wastes and coal. Fuel, 151, 83–90. doi:10.1016/j.fuel.2015.01.010.
- Acevedo, B., & Barriocanal, C. (2015). Texture and surface chemistry of activated carbons obtained fromtyre wastes. Fuel Process Technology, 134, 275–283. doi:10.1016/j.fuproc.2015.02.009
- Al-Saadi, A. A., Saleh, T. A., & Gupta, V. K. (2013). Spectroscopic and computational evaluation of cadmium adsorption using activated carbon produced from rubber tires. Journal of Molecular Liquids, 188, 136–142.10.1016/j.molliq.2013.09.036
- Belgacem, A., Belmedani, M., Rebiai, R., & Hadoun, H. (2013). Characterization, analysis and comparison of activatedcarbons issued from the cryogenic and ambient grindingof used tyres. Chemical Engineering Transactions, 32, 1705–1710.
- Betancur, M., Martínez, J. D., & Murillo, R. (2009). Production of activated carbon by waste tire thermochemical degradation with CO2. Journal of Hazardous Materials, 168, 882–887.10.1016/j.jhazmat.2009.02.167
- Chan, O. S., Cheung, W. H., & McKay, G. (2012). Single and multicomponent acid dye adsorption equilibrium studies on tyre demineralised activated carbon. Chemical Engineering Journal, 191, 162–170.10.1016/j.cej.2012.02.089
- Chan, Y. J., Chong, M. F., Law, C. L., & Hassell, D. G. (2009). A review on anaerobic–aerobic treatment of industrial and municipal wastewater. Chemical Engineering Journal, 155, 1–18.10.1016/j.cej.2009.06.041
- Divrikli, U., Akdogan, A., Soylak, M., & Elci, L. (2009). Factorial design for multivariate optimization of preconcentration system for spectrophotometric phosphorus determination. Talanta, 79, 1287–1291.10.1016/j.talanta.2009.05.046
- Fu, F., & Wang, Q. (2011). Removal of heavy metal ions from wastewaters: A review. Journal of Environmental Management, 92, 407–418.
- Fung, P. P. M., Cheung, W. H., & McKay, G. (2012). Systematic analysis of carbon dioxide activation of waste tire by factorial design. Chinese Journal of Chemical Engineering, 20, 497–504.10.1016/S1004-9541(11)60211-5
- Gupta, V. K., Nayak, A., Agarwal, S., & Tyagi, I. (2014). Potential of activated carbon from waste rubber tire for the adsorption of phenolics: Effect of pre-treatment conditions. Journal of Colloid and Interface Science, 417, 420–430.10.1016/j.jcis.2013.11.067
- López, G., Olazar, M., Artetxe, M., Amutio, M., Elordi, G., & Bilbao, J. (2009). Steam activation of pyrolytic tyre char at different temperatures. Journal of Analytical and Applied Pyrolysis, 85, 539–543.10.1016/j.jaap.2008.11.002
- Min, A., & Harris, A. T. (2006). Influence of carbon dioxide partial pressure and fluidization velocity on activated carbons prepared from scrap car tyre in a fluidized bed. Chemical Engineering Science, 61, 8050–8059.10.1016/j.ces.2006.09.047
- Muga, H. E., & Mihelcic, J. R. (2008). Sustainability of wastewater treatment technologies. Journal of Environmental Management, 88, 437–447.10.1016/j.jenvman.2007.03.008
- Mui, E. L. K., Ko, D. C. K., & McKay, G. (2004). Production of active carbons from waste tyres-a review. Carbon, 42, 2789–2805.10.1016/j.carbon.2004.06.023
- Nomngongo, P. N., & Ngila, J. C. (2015). Multivariate optimization of dual-bed solid phase extraction for preconcentration of Ag, Al, As and Cr in gasoline prior to inductively coupled plasma optical emission spectrometric determination. Fuel, 139, 285–291.10.1016/j.fuel.2014.08.046
- Olorundare, O. F., Krause, R. W. M., Okonkwo, J. O., & Mamba, B. B. (2012). Potential application of activated carbon from maize tassel for the removal of heavy metals in water. JPCE, 50, 104–110.
- Olorundare, O. F., Msagati, T. A. M., Krause, R. W. M., Okonkwo, J. O., & Mamba, B. B. (2014). Steam activation, characterisation and adsorption studies of activated carbon from maize tassels. Chemistry and Ecology, 30, 473–490.10.1080/02757540.2013.877001
- Robles-Molina, J., Gilbert-López, B., García-Reyes, J. F., & Molina-Díaz, A. (2013). Comparative evaluation of liquid–liquid extraction, solid-phase extraction and solid-phase microextraction for the gas chromatography-mass spectrometry determination of multiclass priority organic contaminants in wastewater. Talanta, 117, 382–391.10.1016/j.talanta.2013.09.040
- Soylak, M., Narin, I., Bezerra, M. A., & Ferreira, S. L. C. (2005). Factorial design in the optimization of preconcentration procedure for lead determination by FAAS. Talanta, 65, 895–899.10.1016/j.talanta.2004.08.011