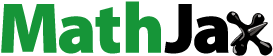
Abstract
It has been known that magnetic exposure of fuel prior to combustion can improve effectiveness of combustion process. However, the main reason of the phenomenon is still unclear. In this paper, characteristics of fuel as exposed to electromagnetic field was measured experimentally and inter-related appropriately in order to have preliminary insight to the clarification of the phenomenon. Fuel characteristics being investigated were viscosity, vibration of the fuel molecules, dipole moment, and refractive index. These experiments were performed using various blend compositions between fossil diesel (petrodiesel) and biodiesel fuel i.e. B0, B10, B40, B70 and B100. The electromagnetic field was generated by a galvanum tube wounded with 9,000 wire coil. The fuel characteristics of both prior and post electromagnetic exposures were then measured with time variation of 0–1,800 s. The experimental results revealed that electromagnetic exposure of the fuel increased vibrational frequency of its molecules significantly, which in turn weakened the attracting energy and caused uniform arrangement of dipole moment of the molecules. The experimental data also revealed that the fuel characteristics did not alter significantly after it was exposed to the electromagnetic field for more than 1,200 s. This information is considered to be useful for further research in order to resolutely clarify the phenomenon of efficient combustion process of fuel after exposure to magnetic field.
Public Statement Interest
Exposure of biodiesel to electromagnetic field yields in higher infrared absorption and lower viscosity. The higher infrared absorption indicates higher level of vibration of the function groups and resulted in lower viscosity of the fuel. This phenomena is regarded as the reason for more effective combustion reaction of the fuel.
The influence of the magnetization was found to be temporary, which will be vanished after 20 minutes release from the electromagnetic field.
1. Introduction
Energy is very important in supporting human’s activity since the earliest era of civilization. This dependency is getting more and more substantial, allowing humans to run the infrastructures on a much larger scale. The highest energy consumption is on fossil fuel, which is non-renewable and hence leading to its rapid declining supply. Several measures are therefore necessary, such as reducing fuel consumption, improving fuel quality and using alternative sources. Biodiesel has great potential as an alternative energy resource because it is considered as renewable fuel, which can be produced continuously (Bünger, Krahl, Schröder, Schmidt, & Götz, Citation2012; Maeda et al., Citation2008).
A number of studies have been conducted in regard to energy conservation by improving combustion efficiency. Mixing additives may be beneficial for that purpose, given that it could increase octane and cetane number, resulting in improving engine power and lowering fly ash emission (Ali, Abdullah, Mamat, & Adam, Citation2015; Fayyazbakhsh & Pirouzfar, Citation2016; Rahhal, Ghata, & Hourieh, Citation2009). Similarly, fuel magnetization can generate efficient combustion, in which permanent magnets applied on the fuel channel to the combustion chamber was reported for a reduction on fuel consumption (9–30%) and exhaust gas emissions such as hydrocarbon (HC) (5–32%) and carbon monoxide (CO) (5–34.3%) (Faris et al., Citation2012; Govindasamy & Dhandapani, Citation2007; Patel, Rathod, & Patel, Citation2014; Ugare, Dhoble, Lutade, & Mudafale, Citation2014). Each of these methods, however, has their own disadvantages. While most chemical additives contain metals which are harmful to humans, permanent magnets depletes over the application time.
An electromagnetic device has also been introduced to overcome the decrease in magnetic intensity of a permanent magnet. When applied on gasoline and diesel operated engines, the device was reported to reduce fuel consumption in the range of 12.8 to 30%, as well as reduce HC emission in the range of 44 to 58% and CO emission in the range of 35 to 80% (Chaware, Citation2015; Fatih & Saber, Citation2010; Patel et al., Citation2014).
While the effect of magnetic exposure in reducing the fuel consumption has been proven, the explanation on the phenomenon is still unclear. Many researchers claim that the magnetic exposure can cause ionization in the fuel (Chaware, Citation2015; Okoronkwo, Nwachukwu, Ngozi, & Igbokwe, Citation2010; Singh & Solanki, Citation2015). Others claim the de-clustering effect and the reduction in size of a fuel’s molecule constellation as shown in Figure (Attar, Tipole, Bhojwani, & Desmukh, Citation2013; Jain & Deshmukh, Citation2012; Jundale, Citation2015). Accordingly, thorough study on the magnetic exposure on the fuel and logical explanation to the phenomenon is indispensably required. The clear explanation to the phenomenon will contribute to the development of the combustion technology that will significantly increase the effectiveness of combustion process.
In this paper, the characteristics of biodiesel blends under electromagnetic exposure were experimentally measured and analyzed. Several parameters, which is considered to be important for clarification of the phenomenon, such as viscosity, molecular vibration of the fuel, magnetic moment, refractive index, and fuel spray characteristics, were experimentally investigated both prior and post exposure to electromagnetic field. Furthermore, the alteration of fuel characteristics due to the electromagnetic exposure was comprehensively discussed. The characteristics to be obtain in this experiment are expectedly can be useful to the clarification of the effect of magnetic exposure to combustion efficiency which has been experimentally proven by many researcher as explained above.
2. Materials and method
Schematic diagram of the experiment is given in Figure . The detail of the experimental materials and method is given in the following sub-sections.
2.1. Generation of electromagnetic field
Electromagnetic field was generated by using a galvanum tube with diameter of 15 mm and length of 100 mm wounded with 0.20 mm diameter of copper wire. The tube was wounded with 9,000 wire coils, and 12 volts DC voltage were used to generate the electromagneticity. The intensity of the electromagnetic field was then measured by using Digital Teslameter Model MG-801, following equation of Biot Savart’s law as shown in Equation (1).(1)
(1)
Here μ is material permeability (Gauss cm/amp), μ0 is vacuum permeability (Gauss cm/amp), L is length of galvanum tube (cm), B is magnetic field (Tesla), N is coil number inductor, and i is DC electric current (amp).
2.2. Sample collection and preparation
Fuel samples consisting of biodiesel and petrodiesel were collected from Watt Co. Ltd and Pertamina Co. Ltd, respectively. The biodiesel was blended with petrodiesel in proportions of 0% (B0), 10% (B10), 40% (B40), 70% (B70), and 100% (B100). Biodiesel used for the blend was checked in laboratory to confirm its conformity to Indonesian National Standard (INS) as shown in Table . A 45 ml sample of each blend was placed in the galvanum tube. The samples were exposed to the electromagnetic field in time variable of 0, 10, 20, 30, 60, 180, 600, 1,200 and 1,800 s at room temperature.
Table 1. The biodiesel property values of INS
2.3. Fuel characteristics
2.3.1. Viscosity
Fuel sample viscosities of B0, B10, B40, B70, and B100 were measured by using a modified Oswald viscometer equiped with magnetic ball and censoring coils connected to sound data detector as shown in Figure . Time of magnetic ball in fluid (tb) was calculated as soon as the ball passed the first coil and the second coil with the distance between these coils was 107 mm. The device was calibrated by using distillate water in order to obtain time accuracy of 1 μs. The fuel viscosity measurement was conducted with 5 replications and used the average value for discussion in order to abate the data uncertainty caused from the measurement. The viscosity was calculated by using Equation (Equation2(2)
(2) ).
(2)
(2)
Here r is radius of magnetic ball (0.961 mm), g is acceleration of gravity (9.8 m/s2), ρb is density of the ball (310,467 kg/m3), and ρf is density of the fuel. The ρf was measured separately as shown in Table .
Table 2. Measured magnetic ball density and fuel density
2.3.2. Vibration of the fuel molecules
Molecular interactions of fuel samples were investigated by analyzing their infrared spectra. The Infra-red spectra were obtained using Fourier Transform Infrared (FTIR) spectroscopy (IR Prestige-21, Shimadzu Co. Ltd). The FTIR spectroscopy is equipped with L-alanine-doped triglycine sulfate (DLATGS) detector. The equipment was set at 4 cm−1 resolution, 20 scans accumulation, and absorbance (%A) measurement mode with wave number ranging from 4,000 to 400 cm−1 in order to determine the functional groups which were formed in the fuel. Samples of 1 μL were mixed with 0.5 g KBr. The FTIR measurements were carried out at room temperature.
2.3.3. Magnetic moment
Vibrating Sample Magnetometer (VSM) instrument (Oxford VSMI.2H) was used to measure the magnetic properties of the fuel samples as a function of magnetic field. The VSM had amplitudes of 1–1.5 mm. The fuel sample with volume of 10 μL was placed in the coiled tube.
2.3.4. Refractive index
The refractive index was then measured by using Atago 3T refractometer model RX-5000α-plus, which needs 3 μL of the fuel sample at room temperature. The instrument was capable for measuring refractive index at the range between 1.327 and 1.585. The measurement was conducted with 5 replications to take into account the possible data uncertainty.
2.3.5. Fuel spray characteristics
Spray characteristic of B10 fuel was firstly investigated in order to assure the effect of electromagnetic exposure of the fuels. It can be realized by using nozzle injector tester with testing pressure of 14.7 MPa. The spray characteristic was then captured by using high resolution camera.
3. Results and discussion
3.1. Effects of electromagnetic exposure on fuel spray performance
Figure shows spray performance of biofuel (B10) out of the injector after 0, 30, 60, 180, 600, 1,200, and 1,800 s of electromagnetic exposure. It appears that exposure of the fuel to electromagnetic field (b, c, d, e, f, g) have wider spray characteristics as compared to un-magnetized one (a). Technically, wider spray of fuel give advantages to combustion process (Avinash & Vipul, Citation2012; Casey et al., Citation2013), since the local viscosity is lower, as it is also confirmed by the measured viscosity. Again, wider spray of the fuel could increase its contact surface with oxygen, and hence improve the combustion process.
3.2. Effects on kinematic viscosity
Figure shows the viscosity of various fuel blends exposed to electromagnetic fields at time intervals. Even though the biodiesel used in the experiment met the INS, its viscosity was higher than petrodiesel, It is important to state the higher viscosity of biodiesel than petrodiesel, as it is also reported by other researcher (Borges, Díaz, Gavín, & Brito, Citation2011; Chavarria-hernandez & Pacheco-catalán, Citation2014; Gülüm & Bilgin, Citation2016; Kafui, Sunnu, & Parbey, Citation2015), since viscosity is closely related to combustion process. The electromagnetic exposure was proven to lower kinematic viscosity of the fuel samples (Marques et al.,Citation1997; Rosensweig, Kaiser, & Miskolczy, Citation1969; Tung, Vinh, Phong, Long, & Hung, Citation2003), and there by exposing the biodiesel blend to electromagnetic field will be advantageous for improving its combustion process. Furthermore, longer exposure time to electromagnetic field resulted in lower viscosity, but not significant anymore after 1,200 s of the exposure time. It is also noted that viscosity value is not only determined by the tensile strength of molecules but also by the state of molecular orientation at liquid (fuel sample) – solid (magnetic ball) interface (Nakano, Citation2003; Sengupta, Herminghaus, & Bahr, Citation2014; Tung et al., Citation2003). It can be expected that the effect of magnetic exposure to the viscosity can be continued for longer exposure time by changing distribution of the molecular orientation at the interface.
3.3. Effects of electromagnetic exposure on fuel molecule interactions
Figure shows the intensity of infrared absorption of petrodiesel and biodiesel fuels from FTIR observation at various wave numbers. Each peak in the graph shows the existence of functional groups. The figure showed that petrodiesel and biodiesel can be distinguished by peak existence at wave numbers 1,743 and 1,176 cm−1 in biodiesel absorbance curve, which represent C=O and C–O bonds (Berman, Meiri, Linder, & Wiesman, Citation2016; Ferrão et al., Citation2011; Furlan, Wetzel, Johnson, Wedin, & Och, Citation2012).
Figure 6. Infrared absorption intensity by petrodiesel and biodiesel fuel as function of wavenumber.
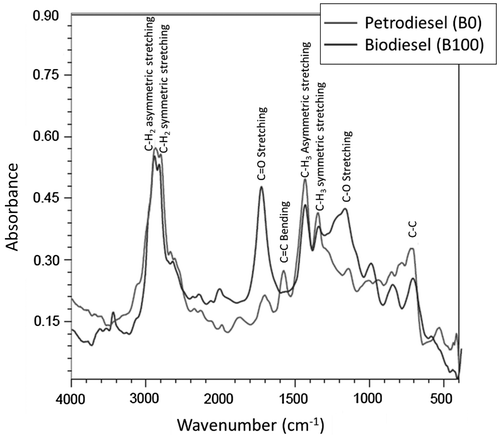
Figure shows FTIR observation of B0, B10, B40, B70, and B100 fuels at varied exposure time to various wave number of electromagnetic field. It can be seen that spectrum of each fuel have identical shape and peak positions regardless the exposure time. This means that the electromagnetic exposure time did not alter molecular structure of those fuels, which also proved that ionization might not occur. Furthermore, by comparing all of the fuel spectra after electromagnetic exposure to the original fuel spectra, the increment of the absorption intensity for each functional group was observed. Table is the summary of the comparison by taking the difference in absorption intensity at wavenumber 2,924 cm−1. The table revealed that extension of exposure time yielded in increasing intensity of infrared absorption, as it is also reported by Calabrò and Magazù (Citation2015) for lower electromagnetic intensity and longer exposure time. The absorption intensity can be correlated to molecular vibration of functional groups. Longer time of electromagnetic exposure causes more number of molecules to vibrate. This phenomenon was consistent with all functional groups existing in the fuel samples, and thereby consistent with all fuel samples regardless of the fuel’s structure.
Figure 7. The infrared spectra of biodiesel, petrodiesel and its blends after 0–1,800 s exposure to electromagnetic field at wavenumber of 400–4,000 cm−1.
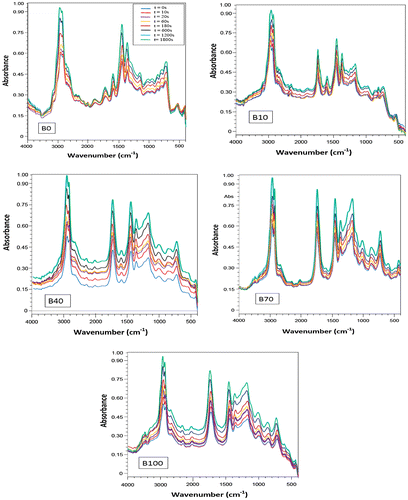
Table 3. Comparison of infrared absorbance at wavenumber 2,924 cm−1 by various fuel samples before and after exposure to 1,500 Gauss electromagnetic field
The vibrational increment of functional groups indicates that the polarization and transition of dipole moments of molecules occur due to the displacements of the fuel molecules and alteration of magnetic moment of those molecular interactions. Furthermore, molecular attracting energy of functional groups is determined by their vibrational frequency in which the higher frequency the lower the absolute value. This is the reason why the fuel viscosity, which is influenced by the molecular attracting energy (Faris et al., Citation2012), decrease after it is exposed to electromagnetic field. Higher absorption intensity, which indicates higher vibration frequency, yield in lower molecular affinity. This means less energy required to break the inter-atomic bonds. Therefore, the molecular affinity of the fuel molecules decreases after exposure to the electromagnetic field.
3.4. Effects on dipole moment
Petrodiesel, biodiesel and its blend are considered as paramagnetic material in which each molecule of the fuel has dipole moment influenced by electromagnetic field. Figure shows the dipole moment obtained from VSM measurement. It can be seen that the electromagnetic exposure causes the increase of the dipole moment, regardless of the biodiesel blending proportion. It means that the fuel molecules arranges themselves according to the direction of electromagnetic field or, in other word, its dipole direction was arranged properly (Kuwako et al.,Citation1997; Nittoh et al., Citation2012; Sheldon, Adjiman, & Cordiner, Citation2005). The main constituent molecule of the fuel sample is hydrocarbon (C–H) that has unpaired electron spin moments. When it is exposed to electromagnetic field, the induced magnetic moment becomes weak. A strong electromagnetic field exposing hydrocarbon molecules causes intermolecular hydrocarbons to repel each other (de-clustering), which creates an optimal distance between molecules of hydrocarbons and oxygen. The polarized molecules are relatively more active and oriented in accordance with the direction of the electromagnetic field.
3.5. Effects on refractive index
In order to clarify that fuel molecules have been arranged uniformly following the electromagnetic field, the refractive index of each fuel samples under electromagnetic exposure was investigated. Theoretically, when fuel molecules arrange uniformly the value of refractive index will be high (Kaur & Pal, Citation2015; Nita, Geacai, Neagu, & Geacai, Citation2013; Zabala, Arzamendi, Reyero, & Gandía, Citation2014). Figure shows the refractive index of biodiesel and its blend with petrodiesel measured by refractometer. It can be seen that longer exposure to electromagnetic fields yields higher refractive index. This result proves that uniform arrangement of the fuel molecules occurs after the fuel was exposed to electromagnetic field.
4. Conclusion
Exposure to electromagnetic fields on petrodiesel fuel, biodiesel, and its blends caused lower viscosity, wider spray characteristic, higher infrared absorption that cause higher vibrational frequency of the functional groups and weaker attracting energy, and higher refractive index values which indicated larger dipole moment. The result also revealed that the effect of electromagnetic field to the fuel was saturated when it was exposed longer than 1,200 s. These information are considered to be useful for further research in order to resolutely clarify the phenomenon of efficient combustion process of fuel after exposure to magnetic field.
Funding
This research is granted by State Educational Scholarship fund 2017, Directorate General of Higher Education, Ministry of Research, Technology and Higher Education.
Additional information
Notes on contributors
Tatun Hayatun Nufus
Tatun Hayatun Nufus is currently a postgraduate student of Agricultural Engineering Sciences at Bogor Agricultural University, Indonesia. She is a lecturer at the Department of Mechanical Engineering, Politeknik Negeri Jakarta. Her reseach interest is energy saving, and has conducted a series of experiment on fuel magnetisasion.
Radite Praeko Agus Setiawan
Radite Praeko Agus Setiawan is an associate professor at the Department of Mechanical and Biosystem Engineering, Bogor Agricultural University, with research interest in mechatronic especially for application in agricultural mechanization.
Wawan Hermawan
Wawan Hermawan is an associate professor at the Department of Mechanical and Biosystem Engineering, Bogor Agricultural University, with research interest in agricultural power and machinery.
Armansyah Halomoan Tambunan
Armansyah Halomoan Tambunan is professor at the Department of Mechanical and Biosystem Engineering, Bogor Agricultural University, with research interest in renewable energy and energy analysis.
References
- Ali, O. M., Abdullah, N. R., Mamat, R., & Adam, A. (2015). Comparison of the effect of different alcohol additives with blended fuel on cyclic variation in diesel engine. Energy Procedia, 75, 2357–2362. doi:10.1016/j.egypro.2015.07.490
- Attar, R. A., Tipole, P., Bhojwani, V., & Desmukh, S. (2013). Experimental investigation of effect of magnetic field on hydrocarbon refrigerant in vapor compression cycle. International Journal of Engineering Research & Technology, 2, 2106–2112.
- Avinash, K. A., & Vipul, H. C. (2012). Spray characteristics of biodiesel/blends in a high pressure constant volume spray chamber. Experimental Thermal and Fluid Science, 42, 212–218.
- Berman, P., Meiri, N., Linder, C., & Wiesman, Z. (2016). H low field nuclear magnetic resonance relaxometry for probing biodiesel autoxidation. Fuel, 177, 315–325. doi:10.1016/j.fuel.2016.03.002
- Borges, M. E., Díaz, L., Gavín, J., & Brito, A. (2011). Estimation of the content of fatty acid methyl esters (FAME) in biodiesel samples from dynamic viscosity measurements. Fuel Processing Technology, 92, 597–599. doi:10.1016/j.fuproc.2010.11.016
- Bünger, J., Krahl, J., Schröder, O., Schmidt, L., & Götz, A. (2012). Potential hazards associated with combustion of bio-derived versus petroleum-derived diesel fuel. Critical Reviews in Toxicology, 8444 ( June 2017). https://doi.org/10.3109/10408444.2012.710194
- Calabrò, E., & Magazù, S. (2015). FTIR spectroscopy analysis of molecular vibrations in gasoline fuel under 200 mT static magnetic field highlighted structural changes of hydrocarbons chains. Petroleum Science and Technology, 33, 1676–1684. doi:10.1080/10916466.2015.1089282
- Casey, A., Elisa, T., Daniel, T., Harold, S., Dennis, M., & Tonghun, L. (2013). Characterization of the effect of fatty ester composition on the ignition behavior of biodiesel fuel sprays. Fuel, 111, 659–669.
- Chavarria-hernandez, J. C., & Pacheco-catalán, D. E. (2014, February). Predicting the kinematic viscosity of FAMEs and biodiesel: Empirical models. Fuel, 1–9. doi:10.1016/j.fuel.2014.01.105
- Chaware, K. (2015). Review on effect of fuel magnetism by varying intensity on performance and emission of single cylinder four stroke diesel engine. International Journal of Engineering and General Science, 3, 1174–1178.
- Faris, A. S., Al-Naseri, S. K., Jamal, N., Isse, R., Abed, M., Fouad, Z., … Abas, A. (2012). Effects of magnetic field on fuel consumption and exhaust emissions in two-stroke engine. Energy Procedia, 18, 327–338. doi:10.1016/j.egypro.2012.05.044
- Fatih, F. A., & Saber, G. M. (2010). Effect of fuel magnetism on engine performance and emissions. Australian Journal of Basic and Applied Sciences, 4, 6354–6358.
- Fayyazbakhsh, A., & Pirouzfar, V. (2016). Investigating the influence of additives-fuel on diesel engine performance and emissions: Analytical modeling and experimental validation. Fuel, 171, 167–177. doi:10.1016/j.fuel.2015.12.028
- Ferrão, M., Viera, M. S., Pazos, R. E. P., Fachini, D., Gerbase, A. E., & Marder, L. (2011). Simultaneous determination of quality parameters of biodiesel/diesel blends using HATR-FTIR spectra and PLS, iPLS or siPLS regressions. Fuel, 90, 701–706. doi:10.1016/j.fuel.2010.09.016
- Furlan, P. Y., Wetzel, P., Johnson, S., Wedin, Y., & Och, A. (2012). Investigating the oxidation of biodiesel from used vegetable oil by FTIR spectroscopy : Used vegetable oil biodiesel oxidation study by FTIR. Spectroscopy Letters: An International Journal for Rapid Communication, 43, 580–585. doi:10.1080/00387010.2010.510708
- Govindasamy, P., & Dhandapani, S. (2007). Performance and emissions achievements by magnetic energizer with a single cylinder two stroke catalytic coated spark ignition engine. Journal of Scientific and Industrial Research, 66, 457–463.
- Gülüm, M., & Bilgin, A. (2016). Two-term power models for estimating kinematic viscosities of different biodiesel-diesel fuel blends. Fuel Processing Technology, 149, 121–130. doi:10.1016/j.fuproc.2016.04.013
- Jain, S., & Deshmukh, P. (2012). Experimental investigation of magnetic fuel conditioner (MFC) in IC engine. IOSJR Journal of Engineering, 2, 27–31.
- Jundale, V. A. (2015). Analysis of fuel and exhaust gases of SI engine by using magnet. The International Journal of Science & Technoledge, 3, 155–159.
- Kafui, G., Sunnu, A., & Parbey, J. (2015). Effect of biodiesel production parameters on viscosity and yield of methyl esters : Jatropha curcas, elaeis guineensis and cocos nucifera. Alexandria Engineering Journal. doi:10.1016/j.aej.2015.09.011
- Kaur, R., & Pal, B. (2015). Physicochemical and catalytic properties of Au nanorods micro- assembled in solvents of varying dipole moment and refractive index. Materials Research Bulletin Journal, 62, 11–18.10.1016/j.materresbull.2014.11.012
- Kuwako, A., Nittoh, K., Ikehara, T., Yoshida, T., Yoguchi, I, & Watanabe, T. (1997). Measurement of electric-dipole moment by polarization laser spectroscopy. Journal of Nuclear Science and Technology, 34, 360–366. doi:10.1080/18811248.1997.9733675
- Maeda, H., Hagiwara, S., Nabetani, H., Sagara, Y., Soerawidjaya, T. H., Tambunan, A. H., & Abdullah, K. (2008). Biodiesel fuels from palm oil via the non-catalytic transesterification in a bubble column reactor at atmospheric pressure : A kinetic study. Renewable Energy, 33, 1629–1636. doi:10.1016/j.renene.2007.08.011
- Marques, L. C. C., Rocha, N. O., Machado, A. L. C., Neves, G. B. M., Vieira, L. C., Dittz, C. H. 1997. Study of paraffin crystalization proess under the influence of magnetic field on chemical. Society of Petroleum Engineers Latin American and Caribbean Petroleum Engineering Conference. Rio de Janeiro, 1–8. doi:10.2118/38990-MS
- Nakano, K. (2003). Scaling law on molecular orientation and effective viscosity of liquid-crystalline boundary films. Tribology Letters, 14, 17–24.10.1023/A:1021710131040
- Nita, I., Geacai, S., Neagu, A., & Geacai, E. (2013). Estimation of the refractive index of diesel fuel + biodiesel blends. Ovidius University annals of Chemistry, 24, 24–26.
- Nittoh, K., Kuwako, A., Ikehara, T., Yoshida, T., Nittoht, K., Kuwako, A., … Yoshidatt, T. (2012). Measurement of dipole-moment in atomic transitions under strong external magnetic field. Journal of Nuclear Science and Technology, 33, 569–576. doi:10.1080/18811248.1996.9731957
- Okoronkwo, A. C., Nwachukwu, C., Ngozi, O. L., & Igbokwe. (2010). The effect of electromagnetic flux density on the ionization and the combustion of fuel. American Journal of Scientific and Industrial Research, 1, 527–531. doi:10.5251/ajsir.2010.1.3.527.531
- Patel, P. M., Rathod, G. P., & Patel, T. M. (2014). Performance and emission analysis of single cylinder diesel engine under the influence of magnetic fuel energizer. IOSR Journal of Mechanical and Civil Engineering (IOSR-JMCE), 11, 34–39. 10.9790/1684
- Rahhal, M., Ghata, A., & Hourieh, Y. (2009). Utilizing chemical additives to reduce the side effects caused by combustion of fuel oil. Petroleum & Coal, 51, 150–159.
- Rosensweig, R. E., Kaiser, R., & Miskolczy, G. (1969). Viscosity of magnetic fluid in a magnetic field. Journal of Colloid and Interface Science, 29, 680–686.10.1016/0021-9797(69)90220-3
- Sengupta, A., Herminghaus, S., & Bahr, C. (2014). Liquid crystal microfluidics : Surface, elastic and viscous interactions at microscales. Liquid Crystals Reviews, 2, 1–47. doi:10.1080/21680396.2014.963716
- Sheldon, T. J., Adjiman, C. S., & Cordiner, J. L. (2005). Pure component properties from group contribution : Hydrogen-bond basicity, hydrogen-bond acidity, Hildebrand solubility parameter, macroscopic surface tension, dipole moment, refractive index and dielectric constant. Fluid Phase Equilibria, 231, 27–37. doi:10.1016/j.fluid.2004.12.017
- Singh, K. A., & Solanki, R. M. (2015). Investigation of fuel saving in annealing lehr through magnetic material fuel saver. International Journal of Science and Research (IJSR), 4, 178–180.
- Tung, N. P., Vinh, N. Q., Phong, N. T. P., Long, B. Q. K., & Hung, P. V. (2003). Perspective for using Nd – Fe – B magnets as a tool for the improvement of the production and transportation of vietnamese crude oil with high paraffin content. Physica B: Condensed Matter, 327, 443–447.10.1016/S0921-4526(02)01788-X
- Ugare, V., Dhoble, S., Lutade, S., & Mudafale, K. (2014). Performance of internal combustion (CI) engine under the influence of stong permanent magnetic field. Journal of Mechanical and Civil Engineering, 11–17.
- Zabala, S., Arzamendi, G., Reyero, I., & Gandía, L. M. (2014). Monitoring of the methanolysis reaction for biodiesel production by off-line and on-line refractive index and speed of sound measurements. Fuel, 121, 157–164. doi:10.1016/j.fuel.2013.12.056