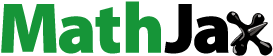
Abstract
In a Differential Chaos Shift Keying (DCSK) system, the reference and information chaotic bearing signals are transmitted in two consecutive time slots. This design requires twice the bit duration. In this paper, we present a system to double the data rate and enhance BER performance in Additive White Gaussian Noise (AWGN) channel. The system is based on adding reference signal and information bearing signal in one time slot without affecting its orthogonality. Information bearing signal is simply a time reversed and modulated version of the reference signal. The bit error performance of the proposed scheme, named Time Reversal Differential Chaos Shift Keying (TRDCSK), is studied analytically based on Gaussian Approximation (GA) for discrete time implementations. Excellent agreement between theoretical estimate and simulation results is obtained. Moreover, BER performance of the proposed scheme is compared with other differential coherent systems. Simulation results in AWGN channel environment show that TRDCSK outperforms the other standard systems at various and with wide range of spreading factor values.
Public Interest Statement
Recently, chaos-based communicants attract many researchers due to its unique properties like non-periodicity and its resistance to different channel condition. The main challenge in such systems is the ability to reproduce the same chaotic signal at the receiver. Moreover, noise makes this task more challenging. One way to overcome this complication is by sending two copy of the signal, one as a reference signal and other as an information carrying signal, which leads to reduce the efficiency of channel bandwidth. This paper suggests one system where the reference and information bearing is added together at same time with negligible effect of interference. Transmitter and receiver are described in details with its associated model. Theoretical performance is evaluated based on Gaussian model. Simulation results illustrate the efficiency of the proposed sequence compared with other systems. System requires to have the chaotic signal and its flipped version on the same time. This can be solved by adding one delay element at the beginning of the system.
1. Introduction
The basic idea of digital communication system using chaotic carrier is that the information bits are mapped to a sample function of chaotic signal from one or more chaotic attractors. Bits are mapped to actual non-periodic output of chaotic circuits and not to parameters of certain sample functions. Therefore, the major difference between a chaotic carrier and conventional carrier is that the sample function for a given symbol is non-periodic and different from one bit to another (Kolumban, Kennedy, & Chua, Citation1997). Based on the previous discussion, the receiver of chaotic communication systems can be broadly classified as coherent, non-coherent, and differentially coherent systems. In coherent systems, with chaotic synchronization, a local synchronized copy of each sample function has to be produced at the receiver. In realistic situations, the received signal is corrupted by noise which makes repeating of the exact copy a challenging task due to non-periodicity properties (Kolumban, Kennedy, & Chua, Citation1998). Non-coherent systems offer simple solutions by multiplying each received sample signal with itself to estimate the signal energy rather than generating a copy of sample function as in non-coherent Chaos Shift Keying and Chaos ON OFF keying(COOK) (Kis, Jako, Kennedy, & Kolumban, Citation1998). However, obtaining an optimum threshold to distinguish between signal sets does not depend only on signal power at the correlator output, but also on the noise power estimation which is the major drawback of non-coherent systems (Lau & Tse, Citation2003).
As discussed earlier, coherent correlation implementation requires an accurate prediction of one or more basic functions of non-periodic noisy chaos signal which is a problematic task for the receiver. Therefore, differential coherent system is suggested. In DCSK (Sushchik, Tsimring, & Volkovskii, Citation2000), every bit is presented by two sample functions. The first is sent as a reference and the second is sent as an information bearing signal. In the case of bit 1, the information is sent by transmitting two identical sample functions. For bit 0, the reference signal is transmitted and followed by an inverted copy of the sample function. In the receiver, the information bearing signal is correlated with the reference signal and the decision is made after passing the result of the correlation to the level comparator. BER performance is enhanced in noisy channel in comparison with other standard differentially coherent systems such as Corelation Delay Shift Keying (CDSK) and High Efficiency Differential Chaos Shift Keying (Yang & Ping Jiang, Citation2012) but data rate in DCSK is halved.
Recently, many techniques have been developed to improve the data rate of DCSK, first is made in Kolumban, Jako, and Kennedy (Citation1999) using single sample function as a reference to modulate multiple information bits. The developed scheme requires additional delay and synchronization circuits compared with standard DCSK. Another method which is based on combining reference signal and information bearing signal at the same time is analyzed in Yang and Jiang (Citation2013), Xu, Wang, and Kolumbn (Citation2011), Xu, Wang, Member, and Chen (Citation2014) and Xu and Leung (Citation2010). Separation between reference and information bearing signal is performed using Walsh code, initial condition modulation, or phase separation. In these schemes, more delay elements are required in DCSK transmitter which is considered as major technical drawback. DCSK BER is further enhanced using repeated sub segments in both signal parts: reference signal and information bearing signal as in Kaddoum and Soujeri (Citation2016), while Escribano, Kaddoum, Wagemakers, and Giard (Citation2016) offers sending reference sample followed directly by information bearing sample to enhance signal resistance against fading effect of the channel. Efficient utilization of the transmitted signal energy using Multicarrier Modulation for DCSK (MC-DCSK) is introduced in Kaddoum, Richardson, and Gagnon (Citation2013). However, the penalty which needs to be paid to implement MC-DCSK is the design of a bank of narrow band modulators with a high degree of accuracy to maintain sub carrier synchronization. The work in Chen, Wang, and Lau (Citation2013) uses Space-Time Block Code DCSK (STBC-DCSK) to enhance BER performance in multipath fading channel. STBC-DCSK enhances the noise performance of the standard DCSK: the data rate and the average energy for each bit are similar to that in DCSK with additional STBC encoder at the transmitter. Permutation of chaotic samples is used to build M-Ary modulation scheme for DCSK and enhance the security as in Cheong, Lau, and Tse (Citation2003). In this paper, a time reversal technique used in many acoustic and wireless communications systems (Albassam, Citation2015; Albassam & Jerew, Citation2016; Chang, Fetterman, Newberg, & Panaretos, Citation1998; Forest & Hoefer, Citation1995; Fouda, Teixeira, & Yavuz, Citation2012; Sorrentino, Roselli, & Mezzanotte, Citation1993) is considered to generate a complete DCSK signal (reference part and information bearing part) with the same bit duration and without any additional signals to maintain the orthogonality. The basic difference between the proposed scheme and the one which has been proposed in Albassam (Citation2015) is the reduction of integration time which reduces the number of intra signal interference in the correlator output. Therefore, the modified version of the time reversal technique can be implemented by:
(1) | Generating a chaotic sample function for one bit duration. | ||||
(2) | Recording a copy of the same segment to establish a time reversal (mirrored) version of the chaotic signal. | ||||
(3) | Adding the forward signal with its time reversed version to create a new signal with time symmetry at the middle of bit duration. | ||||
(4) | Considering the first part as reference signal and the second part as an information bearing signal. |
The remainder of this paper is organized as follows; In Section 2, transmitter and receiver functional block diagram of the proposed system is described and analyzed. An expression to evaluate BER in AWGN is developed. BER simulation results of TRDCSK vs. DCSK, CDSK are illustrated and discussed in Section 4. Paper is concluded in Section 5.
2. System description
Baseband scheme of TRDCSK transmitter consists of two stages: signal generator and modulator as shown in Figure . In the signal generator stage, the source generates M chaotic sequence in each bit duration, where M is the spreading factor. Copy of the segment will be delivered to the Time Reversal (TR) unit where the signal is sampled, recorded, and exactly reproduced in reverse order over one bit duration. This requires an initial delay of one bit which will be ignored in our analysis and we will assume that both signals can be produced at any ith instant. Therefore, the output of TR unit for the first information bit can be given by
. Then both signals; the generated one and its ideal reversed version are added instantaneously. The output represents a symmetric signal around M / 2. The first part serves as a reference signal, while the second part, which is a mirrored version of the first part, will serve as an information bearing signal.
In the data modulator stage, which is a version of DCSK modulator (Sushchik et al., Citation2000), the reference signal in the first half of bit duration is passed directly to the output while the information bearing signal is multiplied by the information bit b and delivered to the output through the switch in the second half of bit duration.
Without loss of generality, we will consider the analysis of the single information bit for the rest of the analysis. Hence, the transmitted signal can be formulated as:
(1)
(1)
The channel under investigation is AWGN and the received signal can be written as:(2)
(2)
where is the noise signal modeled as a stationary random process with power spectral density =
At the receiver, which is shown in Figure , each incoming noisy segment undergoes time reversal process. Hence, the output after the time reversal unit can be given as,
(3)
(3)
With the assumption that the perfect bit synchronization has already been established, each incoming signal is correlated with time reversed version
and the correlator output is not integrated over M as in DCSK and CDSK (Sushchik et al., Citation2000). However, the integration is done over half bit duration only to avoid the effect of redundant signal components in the second half
. The correlator output Z at the end of first bit duration can be given as:
(4)
(4)
The first and second term in (4) represent the effective components which contain significant parts of the modulated signal energy. The third term is the correlation between the chaotic segment and its flipped version over finite time length which can affect the correlator positively or negatively. The latter ones, on the other hand, are the zero-mean disturbance components, which are formed by the noise part of the correlator input. Data are decoded according to the following rule.(5)
(5)
3. BER performance evaluation of TRDCSK
In this section, a theoretical estimation of BER for the proposed system is produced. GA method, which is valid for chaotic systems operating with moderate and large spreading factor is adapted (Sushchik et al., Citation2000). This is due to the fact that at low spreading factors, average energy of each bit is a fluctuated value. Therefore, Probability Density Function (PDF) of bit energy has to be determined as in the integration method (Kaddoum, Chargé, & Roviras, Citation2009; Kaddoum, Chargé, Roviras, & Fournier-Prunaret, Citation2009). Let us recall the standard assumptions and properties of chaos signal which states (Yang & Ping Jiang, Citation2012):
(1) | Chaotic signal | ||||
(2) | The correlation between each chaotic segment with its time reversed version vanishes with sufficient value of M. | ||||
(3) | Statistical independence is assumed between |
The mean of the correlator when +1 is transmitted can be computed as:(6)
(6)
Similarly, we can show that(7)
(7)
Variance of the correlator output at +1 transmission can be calculated as:(8)
(8)
where is the variance operator.
Since Tent map, which has an average value of and computed variances
, is selected to generate the chaotic sequence, it can be easily shown that
(9)
(9)
For signal correlation with its mirrored version, the variance can be found as:(10)
(10)
For other statistically independent terms, variance can be calculated as:(11)
(11)
where is the average noise power with power spectrum density =
, adding Equations (9)–(11) yields:
(12)
(12)
Similarly, we can calculate the variance of the correlator output at transmission. As described earlier, is regarded Gaussian and error can occur if the correlator output goes below the threshold while +1 is transmitted or is above the threshold while
is transmitted. Then BER can be found as:
(13)
(13)
This can be simplified to(14)
(14)
where
4. Results and discussion
In this section, BER performance of TRDCSK is simulated and compared with DCSK, CDSK, and HE-DCSK in AWGN environment and for different levels. To compare with the derived expression in Section 3, simulated BER performance for TRDCSK is first shown in Figure , which illustrates the relationship between theoretical BER of the proposed system and its simulated performance at various M. Apparently, disagreement between theoretical predictions and simulation results is observed at small value of M in Figure . This is due to variable energy value at each bit which clearly shows the limitation of the Gaussian-approximation method. Fortunately, clear agreement between analytic estimates and numerical results can be evidently observed at
in Figure , that positively helps to confirm the validity of Equation (13) with large value of M.
Obviously when , as shown in Figure , TRDCSK always seems 5 dB better than CDSK and 2 dB worse than DCSK. This can be explained by the fact that at low M, orthogonality between the chaotic signal and its time reversed version is not complete. When
, it is very interesting to notice that the proposed system outperforms DCSK by 1 dB and CDSK by 4 dB at BER
due to orthogonality enhancement as shown in Figure . Figures and display the simulation results for TRDCSK, DCSK, and CDSK at
and
which clearly shows the effectiveness of the system in terms of bit error performance. The reason behind this improvement, as compared to other systems, can be ascribed to the effect of intra signal interference in TRDCSK correlator which is decreased due to reduction in the integration period. Consequently, there is reduction in noise variance; particularly the last term in (11) that increases the performance of TRDCSK significantly.
The proposed scheme is tested against another advanced scheme named HE-DCSK (Yang & Ping Jiang, Citation2012). An average advantage of 1 dB can be easily noticed in Figures – at various value of M. As M increases to 600, BERs for all systems becomes worse, which can be attributed to the increasing of negative contribution from noise cross terms in their corresponding correlator outputs as shown in Figure .
5. Conclusion
A baseband TRDCSK is developed and its BER performance is studied. In each bit duration, the chaotic sample function and its mirrored version are used to represent one bit. Hence, bandwidth requirement is reduced in comparison with other standard systems. System performance is tested and compared with other differentially coherent systems. Results reveal that TRDCSK outperforms standard differentially coherent systems at considerable range of M in different scenarios. Additionally, the system offers double bit rate compared to DCSK. Major drawback is the processing time required to provide time reversed version of the sample function.
Additional information
Funding
Notes on contributors
Nizar Albassam
Nizar Albassam received his PhD from Al-Nahrain University, 2004 and MSc Almustansyiah University, 2000. His research interests include wireless communications. Namely Spread Spectrum Communications and Chaos-based Communications and their applications. Currently, He is a faculty member in the Middle East College, Muscat, Oman.
References
- Albassam, N. N. (2015). Reference flipped-differential chaos shift keying scheme for chaos- based communications. International Journal of Engineering & Technology, 4, 320–326.
- Albassam, N. N., & Jerew, O. (2016). Design and implementation of a chaotic scheme in additive white gaussian noise channel. Journal of Computer Networks and Communications. n/a-n/a.
- Chang, Y., Fetterman, H. R., Newberg, I. L., & Panaretos, S. K. (1998). Microwave phase conjugation using antenna arrays. IEEE Transactions on Microwave Theory and Techniques, 46, 1910–1919.
- Chen, P., Wang, L., & Lau, F. (2013). One analog stbc dcsk transmission scheme not requiring channel state information. IEEE Transactions on Circuits and Systems I: Regular Papers, 60, 1027–1037.
- Cheong, K. Y., Lau, F. C., & Tse, C. K. (2003). Permutation-based m-ary chaotic-sequence spread-spectrum communication systems. Circuits, Systems & Signal Processing, 22, 567–577.
- Escribano, F. J., Kaddoum, G., Wagemakers, A., & Giard, P. (2016). Design of a new differential chaos-shift-keying system for continuous mobility. IEEE Transactions on Communications, 64, 2066–2078.
- Forest, M., & Hoefer, W. J. R. (1995). A novel synthesis technique for conducting scatterers using tlm time reversal. IEEE Transactions on Microwave Theory and Techniques, 43, 1371–1378.
- Fouda, A. E., Teixeira, F. L., & Yavuz, M. E. (2012). Time-reversal techniques for miso and mimo wireless communication systems. Radio Science, 47(6), n/a–n/a. RS0P02.
- Kaddoum, G., Chargé, P., & Roviras, D. (2009). A generalized methodology for bit-error-rate prediction in correlation-based communication schemes using chaos. IEEE Communications Letters, 13, 567–569.
- Kaddoum, G., Chargé, P., Roviras, D., & Fournier-Prunaret, D. (2009). A methodology for bit error rate prediction in chaos-based communication systems. Circuits, Systems and Signal Processing, 28, 925–944.
- Kaddoum, G., Richardson, F., & Gagnon, F. (2013). Design and analysis of a multi-carrier differential chaos shift keying communication system. IEEE Transactions on Communications, 61, 3281–3291.
- Kaddoum, G., & Soujeri, E. (2016). Nr-dcsk: A noise reduction differential chaos shift keying system. IEEE Transactions on Circuits and Systems II: Express Briefs, 63, 648–652.
- Kis, G., Jako, Z., Kennedy, M., & Kolumban, G. (1998). Chaotic communications without synchronization. In Proceeding of the International Conference on Telecommunications, IEEE (pp. 49–53). Edinburgh.
- Kolumban, G., Jako, Z., & Kennedy, M. (1999). Enhanced versions of dcsk and fm dcsk data transmission systems. In Proceeding of the International Conference on Circuits and Systems ISCAS ’99 (Vol. 4, pp. 475–478). Orlando, FL: IEEE.
- Kolumban, G., Kennedy, M., & Chua, L. (1998). The role of synchronization in digital communications using chaos II chaotic modulation and chaotic synchronization. IEEE Transactions on Circuits and Systems I: Fundamental Theory and Applications, 45, 1129–1140.
- Kolumban, G., Kennedy, M. P., & Chua, L. O. (1997). The role of synchronization in digital communications using chaos. fundamentals of digital communications. IEEE Transactions on Circuits and Systems I: Fundamental Theory and Applications, 44, 927–936.
- Lau, F. C. M., & Tse, C. K. (2003). Chaos-Based digital communication systems: Operating principles, analysis methods, and performance evaluation. Berlin: Springer.
- Sorrentino, R., Roselli, L., & Mezzanotte, P. (1993). Time reversal in finite difference time domain method. IEEE Microwave and Guided Wave Letters, 3, 402–404.
- Sushchik, M., Tsimring, L., & Volkovskii, A. (2000). Performance analysis of correlation-based communication schemes utilizing chaos. IEEE Transactions on Circuits and Systems I: Fundamental Theory and Applications, 47, 1684–1691.
- Xu, M., & Leung, H. (2010). A novel high data rate modulation scheme based on chaotic signal separation. IEEE Transactions on Communications, 58, 2855–2860.
- Xu, W. K., Wang, L., & Kolumbn, G. (2011). A novel differential chaos shift keying modulation scheme. International Journal of Bifurcation and Chaos, 21, 799–814.
- Xu, W., Wang, L., Member, S., & Chen, G. (2014). Performance analysis of the CS-DCSK / BPSK communication system. IEEE Transactions on Circuits and Systems I: Regular Papers, 61, 2624–2633.
- Yang, H., & Jiang, G.-P. (2013). Reference modulated DCSK: A novel chaotic communication scheme. IEEE Transactions on Circuits and Systems II: Express Briefs, 60, 232–236.
- Yang, H., & Ping Jiang, G. (2012). High-efficiency differential-chaos-shift-keying scheme for chaos-based noncoherent communication. IEEE Transactions on Circuits and Systems II: Express Briefs, 59, 312–316.