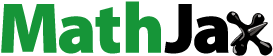
Abstract
There exist a discrepancy between the usage of the expensive standard accelerometer and the cheap and accessible consumer device accelerometer for the measurement of whole-body vibration (WBV). This study investigated the validity of accelerations (g-forces) obtained from a consumer device accelerometer by simultaneously using the consumer device accelerometer with a standard accelerometer during evaluation of WBV experienced by earthmoving equipment operators. The consumer device accelerometer is a software (WBV) compatible with iOS devices from operating system 5 and above (LIS331DLH, 3-axis, ±2 g acceleration range, MEMS type) while the standard accelerometer used during this study is a GCDC tri-axial accelerometer (GCDC X16-4 3-Axis, G-Force Data logger, ±18 g acceleration range, MEMS type). The two accelerometers were mounted following the basicentric axes of the seated operators and put on before earthmoving operation. Data from both the accelerometers were analysed using MATLAB. Results gotten from both accelerometers revealed extreme values of WBV. Some fell within the Health Guidance Caution Zone (HGCZ i.e. 0.47 m/s2 and 0.93 m/s2) while some were above 0.93 m/s2. There were however differences between values gotten from the two accelerometers indicating inaccuracy from the consumer device accelerometer. This research created ground for improvement of accelerometers available on mobile devices and perhaps waken the need for developing new and economic methods of WBV measurement and evaluation.
Public Interest Statement
Whole-body vibration (WBV) is often encountered during the operation of locomotive vehicle ranging from light cars to heavy earthmoving equipment. The International Organization for Standardization (ISO) and other international standards have stipulated certain limits of WBV dosage. They have also highlighted methods of WBV evaluation and the appropriate equipment (accelerometer and data acquisition devices) which are very expensive. Due to economy of evaluation, consumer device accelerometers compactible which android, apple and window devices were invented. This study investigated the inaccuracy of these consumer device accelerometers and suggests adequate and better technology to foster the attempt of WBV evaluation at an affordable cost. The accelerometers available on consumer devices are recommended for WBV monitoring rather than evaluation, they can however be used for evaluation upon great improvement.
1. Introduction
There often exist discrepancy between the usage of the standard and consumer device (available on mobile phone) whole-body vibration (WBV) measuring devices i.e. accelerometers. The standard accelerometers are expensive and difficult to procure while the consumer device accelerometers on android, window and apple phones from operating system (OS) 5 and above are readily available and easily used. Measuring WBV requires cost between 4,500 USD and 10,000 USD (Burgess-Limerick, Citation2014; Stein, Chmurny, & Rosik, Citation2011). Apart from the cost of procuring a standard accelerometer, data obtained from it are expensive and difficult to analyse. The case is not so for the consumer device accelerometers available on mobile devices.
An accelerometer is a device that measures “proper” acceleration. The acceleration measured by an accelerometer is not the same as the coordinate acceleration (i.e. rate of change). An accelerometer at rest will measure 9.81 m/s2 and will measure zero when falling freely due to gravity. The accelerations measured by accelerometers are often times termed g-forces. Accelerometers are essentially used in measuring WBV and hand-arm vibration in workplaces where vibrations are encountered.
WBV measurement is an important aspect of workplace ergonomics because workplaces with vibration values exceeding limits stipulated by the International Organization of Standard (International Standards Organization [ISO 2631-1], Citation1997) and European Parliament and the Council of the European Union (Citation2002/44/EC) are the causes of musculoskeletal disorders (MSDs) (Bodunde, Citation2016). ISO 2631-1 (Citation1997) is an evaluation done when the WBV is without shock either in one direction or multiple direction. It uses frequency-weighted RMS acceleration and vibration dose values for its evaluation. The Health Guidance Caution Zone (HGCZ) is the interval between the lower and upper bound 0.47 and 0.93 m/s2 respectively (McPhee, Foster, & Long, Citation2009) in terms of an eight hour energy equivalent acceleration and corresponding vibration dose value (VDV) of 8.5 and 17 m/s1.75 respectively (Wolfgang & Burgess-Limerick, Citation2014a). The European parliament, on the other hand, via the directive 2002/44/EC suggested the exposure action and limit values for frequency-weighted RMS acceleration and VDV for both hand-arm and whole-body vibrations as shown in Table .
Table 1. European parliament recommended action and limit values
Low Back Pain (LBP) is a type of MSD that is linked with occupational exposure to WBV. An operator is proned to LBP when his body is exposed to vibration especially for prolong duration (Bodunde, Citation2016; Bovenzi, Citation2009; Darby, Citation2008; Gallais & Griffin, Citation2009). The injuries to heart and lungs probably result from the beating of these organs against each other and against the rib cage due to excessive vibration. The brain injury, which is a superficial hemorrhage, may be due to relative motion of the brain within the skull, to mechanical action involving the blood vessels directly, or to secondary mechanical effects. An increase in body temperature is also observed after exposure to intense vibration (Joubert, Citation2009).
Workplaces with high and intense level of vibration magnitude include seats of Earthmoving Equipment (EME) (Smith & Leggat, Citation2005; Zhao & Schindler, Citation2014). The operators of EME are exposed to WBV, which may be extremely severe depending on factors such as nature of equipment, task to be performed, speed of travel condition of the working site etc.
ISO and other international standards recommends the use of seat-pad accelerometer and other data acquisition devices for the evaluation of WBV (McPhee et al., Citation2009). Some researchers have used the seat-pad accelerometers for evaluating WBV and values gotten from their researches predict accurately the behaviours of WBV accelerations in x, y, and z axes (Azizan, Fard, Azari, & Jazar, Citation2017; Deboli, Calvo, & Preti, Citation2017; Liu, Qiu, & Griffin, Citation2017; Mayton, Porter, Xu, & Weston, Citation2017).
A research laboratory (CRA-ING) in Treviglio, Italy developed an application software (INTRAC-Vibra) compatible with smartphones for the measurement and evaluation of WBV. The application regards filtering and frequency weighting and disregards the basicentric axes specified by ISO 2631-1 (Citation1997). It was however tested simultaneously with a standard vibrometer and revealed a mean error between 5.7 and 25% (Cutini & Bisaglia, Citation2014). The results obtained from the accelerometers showed no significant difference when tested with a two way analysis of variance (ANOVA). Other low cost means of evaluating WBV has lately been developed and no significant difference were found when compared with standard accelerometers (Koenig, Chiaramont, & Balbinot, Citation2008; Wolfgang & Burgess-Limerick, Citation2014b).
A recent study by Wolfgang and Burgess-Limerick (Citation2014a) developed a low-cost, yet effective consumer electronic accelerometer to evaluate WBV due to the high cost of evaluating WBV with the seat-pad accelerometer and data analysis systems. The consumer device developed uses apple iOS application, WBV (i-phone from OS 5 and above). The device was however tested with a standard tri-axial seat pad accelerometer, and there were no significant difference between the accelerations measured by the two devices.
This research made use of two accelerometers simultaneously (a standard accelerometer and a consumer device accelerometer available on apple device) with sufficient sensitivity for the required frequency bandwidth for WBV encountered during the operation of earthmoving equipment.
2. Methodology
The two accelerometers used to measure WBV magnitude are mounted on the seat of the earthmoving equipment following the basicentric axes of the human. The vibration values of EME were measured using a tri-axial accelerometer (GCDC X16-4 3-Axis, G-Force Data logger, ±18 g acceleration range, MEMS type) and consumer device accelerometer (LIS331DLH, 3-axis, MEMS type) available on iOS 5 apple device. The specifications of the accelerometers are compared on Table . The EME equipment selected for the study were bulldozers (B1 and B2), Excavators (E1and E2), Graders (G1 and G2), Pail loaders (P1 and P2), Vibrating Rollers (R1 and R2), Backhoes (H1 and H2). The specifications and makes of the equipment were consensually hidden due to the agreement between the researchers and the construction companies where the experiments were carried out.
Table 2. Specification of the accelerometers
The accelerometers were oriented to the basicentric axes of the operators’ body and were mounted on the seat of the EMEs used for the study. The accelerometers were put on just before commencement of earthmoving operation and stopped after the operation. The minimum time of measuring a WBV value was 25 min, maximum was 72 min and average was 37.25 min. The basicentric axis of the accelerometers are shown in Figure .
2.1. Equipment configuration and calibration (Tumble test)
The configuration and calibration instructions provided by the manufacturer of the GCDC X16–4 data logger were used to configure and calibrate the equipment respectively.
A 6-point tumble test was performed to calibrate the data logger, the tumble test used gravity as a reference acceleration. The equipment used for the test were;
(i) | marble tile; | ||||
(ii) | spirit level; and | ||||
(iii) | wooden cube. |
The procedures followed in the tumble test calibration were to:
(i) | use the configuration code; | ||||
(ii) | place the marble tile on a level table; | ||||
(iii) | ensure horizontal surface using the spirit level; | ||||
(iv) | place the GCDC tri-axial accelerometer in the wooden cube and on it; | ||||
(v) | place on surface 1 for about 10 s on the level tile; | ||||
(vi) | repeat step (v) for other surfaces; and | ||||
(vii) | download the data for analysis. |
The set-up of the calibration of the GCDC accelerometer is as shown in Plate .
The consumer device accelerometer available on the apple device doesn’t need any calibration and hence not calibrated.
The 6 points of the tumble test placed each axis in the line of positive and negative gravity, one axis registering gravity and the two other axes are perpendicular to gravity. The value of the calibration factor was found to be 2,048. This was used to divide the accelerations obtained from the GCDC tri-axial accelerometer.
2.2. Equipment and operators
The EME used for the study include bulldozer, excavator, pail loader, grader, vibrating roller and backhoe. The operating speeds of the equipment range from 0.22 to 9.92 m/s2. The conditions of the equipment as at when the study was done couldn’t have imposed inherent vibration on the equipment.
The operator selected were grouped into ages thus (less than 18; between 19 and 39; between 40 and 60; and above 60). Figure shows the number of operators that fall into each category of age range. The year of the operators operations’ experiences were adequate for them to be operating the equipment. The ages of the operator used in the study falls largely in the range 19–39 (68%) which is practically the active age of operators.
2.3. Determination of RMS average acceleration
ISO specified an RMS based method of evaluating WBV from the time-acceleration domain data obtained from the accelerometer. Equation (1) was used to evaluate the weighted acceleration, ARMS. Also, ISO evaluation frequency weighting factor of Wd (kx = ky = 1.4) and Wf (kz = 1) were used during the evaluation.(1)
(1)
where ARMS is the RMS average acceleration (m/s2); aw(t) is the acceleration at time t (m/s2); and T is the period of exposure (seconds).
2.4. Determination of crest factor
The crest factor was determined to ascertain the presence of shock, multiple shocks, jolts or jars in the vibration exposure magnitude of the operators. Equation (2) was used to determine the value of the crest factor in each axis of the acceleration.(2)
(2)
where CF is the Crest Factor; Maximum aw is the highest value of acceleration in each axis (m/s2); and ARMS is the RMS average acceleration (m/s2).
2.5. Determination of VDV
To factor in the presence of multiple shock in vibration exposure, the fourth-power VDV, in m/s1.75 is evaluated using the relationship in Equation (3).(3)
(3)
where aw (t) is the acceleration at time t (m/s2); and T is the period of exposure (seconds).
3. Results and discussions
The results obtained from the two accelerometers gave accelerations in x, y and z axes. The axis with the highest value of WBV is compared with the limits stipulated by ISO. ISO stipulated an exposure action value (EAV) of 0.47 m/s2 and exposure limit value (ELV) of 0.93 m/s2 using the average RMS acceleration and VDV action value of 8.5 m/s1.75 and limit value of 21 m/s1.75. The highest RMS acceleration and VDV values exist mostly in z-axis due to the nature of terrain where EME are operating. Figures and show respectively the RMS acceleration and VDV obtained from the standard WBV acceleration data logger while Figures and show respectively those obtained from the consumer device accelerometer. Figures however show that operators of EME are exposed to extreme WBV. Highest values of acceleration found in z-axis is consistent with the findings of Wolfgang and Burgess-limerick (Citation2014b) and Smets, Eger and Grenier (Citation2010).
Comparing the results obtained from the standard and consumer device accelerometers. The standard accelerometer (data logger) detected accurately the zone of WBV exposure (i.e. falling below exposure action value; falling within HGCZ; or falling above limit value). However, the consumer device accelerometer also detected the zone of WBV exposure which agrees with the study of McGlothlin, Burgess-Limerick, and Lynas (Citation2015), a study that developed an iOS application for evaluating WBV. An error of ±0.077 m/s2 was reported. The error reported didn’t justify the accuracy of consumer device accelerometer for evaluating WBV.
Figures and show respectively the average RMS acceleration and VDV of results obtained from the two accelerometers along x-axis for the sake of comparison, the comparison however shows that there exist great differences in the values of accelerations on x-axis. The trend lines indicate the behaviour of the vibration from one equipment to another. Figures and show respectively the variation in the average RMS acceleration and VDV along y-axis. Similarly Figures and show those on z-axis.
4. Conclusions and recommendations
Apart from measuring and evaluating the extreme WBV encountered by operators of EME during operations, a standard and consumer device vibration measuring devices were compared during this study. The comparison was done simultaneously by mounting a GCDC data logger and an apple device accelerometer on the seats of EME. The results however revealed a great difference in vibration values gotten from the two accelerometers in all the equipment under study. Even though the consumer device accelerometer revealed high and extreme value of WBV encountered during the operation of EME, it didn’t measure accurately the WBV values.
The consumer device accelerometer can only be used for WBV monitoring rather than measurement. This study recommends the usage of standard WBV measuring devices e.g. seat pad accelerometers, data loggers and other devices recommended by the International Organisation of Standard through their ISO 2631-1 (Citation1997) standard and European Union Parliaments through their 2002/44/EC for WBV measurement and evaluation. The consumer device accelerometers may just be used for monitoring WBV.
The accuracy of the standard accelerometers do not erase the fact that measuring and evaluating WBV with them are rather too expensive, hence continuous improvements, further studies and inventions are needed to increase the precision and accuracy of accelerometers built-in with consumer device for economy of WBV evaluation. Other economic methods of evaluating WBV may as well be invented and validated with standard WBV measuring device to salvage the high cost of measuring WBV with expensive accelerometers.
The novel contribution of this investigation is an awareness of the inaccuracy of WBV values gotten from measurements using consumer device accelerometers and the intimation of a better and appropriate technology to improve its accuracy to salvage the problem of the high cost of WBV evaluation.
Funding
The authors received no direct funding for this research.
Acknowledgement
The authors wish to acknowledge the management of KOPEK, Smace and DROMO construction Industries, Nigeria for allowing the usage of their equipment and their operators to take part in this research.
Additional information
Notes on contributors
B.O. Akinnuli
B.O. Akinnuli is an associate professor in the Department of Industrial and Production Engineering of the Federal University of Technology, Akure, Nigeria.
O.A. Dahunsi
O.A. Dahunsi is an associate professor in the Department of Mechanical Engineering of the Federal University of Technology, Akure, Nigeria.
S.P. Ayodeji
S.P. Ayodeji is an associate professor in the Department of Industrial and Production Engineering of the Federal University of Technology, Akure, Nigeria. He is currently the Head of Department.
O.P. Bodunde
O.P. Bodunde is a lecturer in the Department of Mechanical and Mechatronics Engineering of the Afe Babalola University, Ado-Ekiti, Nigeria and a PhD Student in the Department of Mechanical Engineering of the Federal University of Technology, Akure, Nigeria.
The current interest of this group of researchers is basically but not limited to Occupational Safety and Ergonomics.
References
- Azizan, A., Fard, M., Azari, M. F., & Jazar, R. (2017). Effects of vibration on occupant driving performance under simulated driving conditions. Applied Ergonomics, 60, 348–355.10.1016/j.apergo.2016.12.020
- Bodunde, O. P. (2016). Ergonomic evaluation of dynamic and post effects of vibration on earthmoving equipment operators (Unpublished MEng Thesis, pp. 10–20). Mechanical Engineering Department, the Federal University of Technology, Akure.
- Bovenzi, M. (2009). A longitudinal study of low back pain and daily exposure in professional drivers. In Proceedings of the 4th International Conference on Whole-body Vibration Injuries, Montreal, Canada. June 2–4th, 3–4.
- Burgess-Limerick, R. (2014). Developing and validating an affordable whole-body vibration measurement device (Final Report, pp. 1–7). Minerals Industry Safety and Health Centre, Sustainable Minerals Institute, the University of Queensland, Australia.
- Cutini, M., & Bisaglia, C. (2014). Whole-body vibration monitoring using a smartphone. In Proceedings of the International Conference of Agricultural Engineering (AgEng), Zurich, Switzerland. July 6–10th, 1–8.
- Darby, A. M. (2008). Whole-body vibration and ergonomics toolkits. health and safety laboratory. Health and Safety Executives. Retrieved February 16, 2015 from www.hse.gov.uk/research/rrpdf/rr612.pdf
- Deboli, R., Calvo, A., & Preti, C. (2017). Whole-body vibration: Measurement of horizontal and vertical transmissibility of an agricultural tractor seat. International Journal of Industrial Ergonomics, 58, 69–78.10.1016/j.ergon.2017.02.002
- European Parliament and the Council of the European Union (2002/44/EC). (2002). Minimum health and safety requirements regarding the exposure of workers to the risks arising from physical agents (vibration). Official Journal of the European Communities Directive 2002/44/EC on the OJ L177, 6(7), 13.
- Gallais, L., & Griffin, M. J. (2009). Low back pain and risk factors for low back pain in taxi drivers. In Proceedings of the 4th International Conference on Whole body Vibration Injuries, Montreal, Canada. June 2–4th, 5–6.
- International Standards Organization (ISO 2631-1). (1997). Mechanical vibration and shock. Guide for the evaluation of human exposure to whole-body vibration, part 1. ISO 2631/1-1997.
- Joubert, D. M. (2009). Professional driving and adverse reproductive outcomes: The evidence to date and research challenges. The Open Occupational Health & Safety Journal, 1, 1–6.
- Koenig, D., Chiaramont, M. S., & Balbinot, A. (2008). Wireless network for measurement of whole-body vibration. Sensors, 8, 3067–3081.10.3390/s8053067
- Liu, C., Qiu, Y., & Griffin, M. J. (2017). Dynamic forces over the interface between a seated human body and a rigid seat during vertical whole-body vibration. Journal of Biomechanics, 61, 176–182.10.1016/j.jbiomech.2017.07.015
- Mayton, A. G., Porter, W. L., Xu, X. S., & Weston, E. B. (2017). Investigation of human body vibration exposures on haul trucks operating at U.S. surface mines/quarries relative to haul truck activity. International Journal of Industrial Ergonomics, In press, 1–11.
- McGlothlin, J., Burgess-Limerick, R., & Lynas, D. (2015). An iOS application for evaluating whole-body vibration within a workplace risk management process. Journal of Occupation and Environmental Hygiene, 12(7), 137–142.10.1080/15459624.2015.1009986
- McPhee, B., Foster, G., & Long, A. (2009). Bad vibrations (2nd ed.). Sydney: Coal Services Health & Safety Trust.
- Smets, M. P. H., Eger, T. R., & Grenier, S. G. (2010). Whole-body vibration experienced by haulage truck operators in surface mining operations: A comparison of various analysis methods utilized in the prediction of health risks. Applied Ergonomics, 41(6), 763–770. doi:10.1016/j.apergo.2010.01.002.
- Smith, D. R., & Leggat, P. A. (2005). Whole-body vibration: Health effects. Measurement and Minimization. Professional Safety, 50(7), 35–40.
- Stein, G. J., Chmurny, R., & Rosik, V. (2011). Compact vibration measuring system for in-vehicle applications. Measurement Science Review, 11(5), 154–159.
- Wolfgang, R., & Burgess-Limerick, R. (2014a). Using consumer electronic devices to estimate whole-body vibration exposure. Journal of Occupational and Environmental Hygiene, 11(6), D77–D81.10.1080/15459624.2014.888073
- Wolfgang, R., & Burgess-Limerick, R. (2014b). Whole-body vibration exposure of haul truck drivers at a surface coal mine. Applied Ergonomics, 45(6), 1700–1704.10.1016/j.apergo.2014.05.020
- Zhao, X., & Schindler, C. (2014). Evaluation of whole-body vibration exposure experienced by operators of a compact wheel loader according to ISO 2631-1:1997 and ISO 2631-5:2004. International Journal of Industrial Ergonomics, 44(6), 840–850.10.1016/j.ergon.2014.09.006