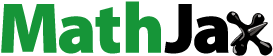
Abstract
Phosphor-converted white light-emitting diodes (pc-WLEDs) are energy efficient and environmentally friendly light sources with a long lifetime, applicable in both display backlights and general lighting. In this research, we investigated the influence of the red-emitting K2SiF6:Mn4+ conversion phosphor on the color quality of the 7000K conformal packaging multi-chip white LEDs (MCW-LEDs). The effect of the red-emitting Ba2Si5N8Eu2+ convention phosphor is demonstrated based on Mie Theory by Mat Lab and Light Tools software. The research results indicated that the color quality of MCW-LEDs was crucially affected by the red-emitting K2SiF6:Mn4+ conversion phosphor’s size. This research demonstrated a significant recommendation for selecting and developing the phosphor materials for MCW-LEDs manufacturing.
Public Interest Statement
Light-emitting diodes (LEDs) have found their way into many applications in daily life, from warm-white LEDs for energy-efficient lighting to display backlights for smartphones, tablets, and televisions. The current attention to energy saving and reduction of CO2 emission in the atmosphere should, therefore, give an additional boost to the development of LEDs for lighting. In this research, we investigated the influence of the red-emitting K2SiF6:Mn4+ conversion phosphor on the color quality of the 7000K conformal packaging multi-chip white LEDs (MCW-LEDs). The research results indicated that the color quality of MCW-LEDs was crucially affected by the red-emitting K2SiF6:Mn4+ conversion phosphor’s size. This research demonstrated a significant recommendation for selecting and developing the phosphor materials for MCW-LEDs manufacturing.
1. Introduction
Light-emitting diodes (LEDs) have found their way into many applications in daily life, from warm-white LEDs for energy-efficient lighting to display backlights for smartphones, tablets and televisions. The history of LEDs has been noticed more than a century. Already in 1907, H. J. Round published on light emission from a silicon carbide junction diode, the first light emitting diode (LED) ever. Independently, Losev observed emission from ZnO and SiC diodes, as published in 1927. At that time, the potential of the technology was not realized and the inventions remained largely unnoticed. It was not until 1962 that the first practical visible spectrum LEDs was developed, by Nick Holonyak at General Electric. In the decades that followed, LEDs were used extensively in numerical displays and signaling applications. However, only around 1995 high brightness and blue LEDs were developed, which made it possible to use LEDs for general lighting. Nowadays, LEDs use a mature technology which can compete with the traditional incandescent and (compact) fluorescent lamps. White light-emitting diodes (w-LED) are attracting tremendous attention in the recent years as a potential replacement for the conventional light sources because of their low electric consumption, high brightness, long lifetime, small size, robustness, fast switching, and environment friendly characters. Moreover, usinf LEDs can considered as a reduction of the worldwide electricity consumption. The current attention to energy saving and reduction of CO2 emission in the atmosphere should therefore give an additional boost to the development of LEDs for lighting. Remaining disadvantages of LEDs are the need for extensive cooling of high power devices (ultimately limiting the maximum power per LED chip), the need for current driving and the lack of high color quality white LEDs (Gibney, Citation2014; Liu & Luo, Citation2011; Luo, Run, Liu, & Wang, Citation2016; Sijbom, Verstraete, Joos, Poelman, & Smet, Citation2017; Smet, Parmentier, & Poelman, Citation2011; Wang, Li, Zhang, Zhan, & Zhang, Citation2015; Winkler, Trinh, Bodrogi, & Khanh, Citation2015). There has been considerable research in the lighting community in an effort to fabricate white light sources using InGaN based LEDs. One popular approach to produce white light is the combination of blue-emitting InGaN LED with a yellow-emitting Y3Al5O12:Ce3+(YAG:Ce3+) phosphor, but this type of white light suffers from poor color rendering index (CRI) due to the lack of a red light component. In the last few decades, there so many research focus on improving the optical performance of the pcLED in packaging direction. Thickness and concentration of phosphor are considered as the main factors in the white LEDs packaging because the luminous flux and color of LEDs are adjusted mainly through changing the phosphor thickness and concentration after the phosphor converters are chosen. Tran and Shi (Citation2008) and Shuai, He, Tran, and Shi (Citation2011) experimentally studied the effects of phosphor thickness and concentration on LEDs luminous flux and correlated color temperature. From the results, higher luminous efficacy are taken with the lower phosphor concentration and higher phosphor thickness (lower trapping efficiency and fewer backs scattering of light). Sommer et al. firstly studied the effects of phosphor thickness, concentration, and size on the spatial color distribution of white LEDs (Sommer et al., Citation2008). The spatial color uniformity of white LEDs could be improved by changing the phosphor concentration or thickness. An increase of the phosphor thickness or concentration can alter the color from bluish to yellowish, and the growth in the central zone is faster than that in the border area. In Li et al. (Citation2012) and Liu, Liu, Wang, and Luo (Citation2008a, Citation2008b, Citation2008c), the effect of phosphor location on the spatial color distribution was investigated. The results showed that to obtain high color uniformity, packaging elements should make the blue light and yellow light have a similar radiation pattern. In the last year, some researchers were concentrated on enhancing the optical performance of multi-chip white LEDs (MCW-LEDs) by adding green or red phosphor into the phosphor compounding (Anh & Hsiao-Yi, Citation2016; Anh et al., Citation2017; Minh, Quang, Nhan, Anh, & Lee, Citation2017). It can be found that the optical properties of MCW-LEDs can be enhancing clearly. From this point of view, improving the color quality of MCW-LEDs by adding diffusers into the phosphor compounding is still needed to investigate. In this research, we try to fill the remaining gap.
As rare-earth-free alternatives, the manganese-based phosphors K2SiF6:Mn4+ has d-d transitions, which are partially spin-allowed for Mn4+. Upon incorporation in the host compound, Mn4+ is the preferred valence state for LED applications, due to better excitation properties and narrower emission bands. In K2SiF6:Mn4+, the Intra configurational 3d3 transitions of the Mn4+ ion are responsible for the luminescence, yielding narrow line emission. Furthermore, K2SiF6:Mn4+ has the relatively high values of the color quality scale (CQS), color purity and absorption strength. The specific red line emission seen in K2SiF6:Mn4+ around 630 nm is caused by 3d3–3d3 transitions in Mn4+. The 3d3 electron configuration of Mn4+ results in 120 possible distributions of the three electrons over the ten available single-particle states of the 3d shell. The electronic Coulomb repulsion gives rise to a splitting of the 120-fold degenerate configuration in eight LS terms that can be found for the free Mn4+ ion, the magnitude of this splitting is characterized by the Racah parameters B and C. Because of these advantages, the red-emitting K2SiF6:Mn4+ conversion phosphor is chosen for enhancing the color quality of the 7,000K Conformal Packaging MCW-LEDs (Sijbom et al., Citation2017; Smet et al., Citation2011; Wang et al., Citation2015).
In this research, the red-emitting K2SiF6:Mn4+ phosphor is proposed to enhancing the color quality of the 7000K conformal packaging MCW-LEDs. This paper can be divided into 3 section. The physical model of the 7000K conformal packaging MCW-LEDs were presented in the first section. In the second section, the mathematical optical description based on Mie theory of red-emitting K2SiF6:Mn4+ phosphor with Mat lab software and the simulation results with the commercial Light Tools software were collected. Finally, the research results were deeply discussed, analyzed, and investigated in the third sections and some conclusion was proposed. According to the results, we can conclude that the red-emitting K2SiF6:Mn4+ conversion phosphor’s size crucial influenced on the color quality of the 7000K conformal packaging MCW-LEDs. The red-emitting K2SiF6:Mn4+ conversion phosphor could be considered as a prospective solution for MCW-LEDs improvement.
2. Research method
The simulation comprises the setup of the 7000K conformal packaging MCW-LEDs. In this situation, we use the real-world model of MCW-LEDs (Figure (a)). In this simulation stage, we set the main parameters of the in-cup MCW-LEDs like below:
(1) | The depth, the inner and outer radius of the reflector to 2.07, 8 and 9.85 mm, respectively. | ||||
(2) | Four LED chips are covered with a fixed thickness of 0.08 mm and 2.07 mm. Each blue chip has a dimension of 1.14 mm by 0.15 mm, the radiant flux of 1.16 W, and the peak wavelength of 453 nm (Figure (b)). |
Figure 1. (a) The MCW-LED product of the Siliconware Precision Industries Co., Ltd., Taiwan, (b) Illustration of MCW-LEDs with the in-cup phosphor packaging.
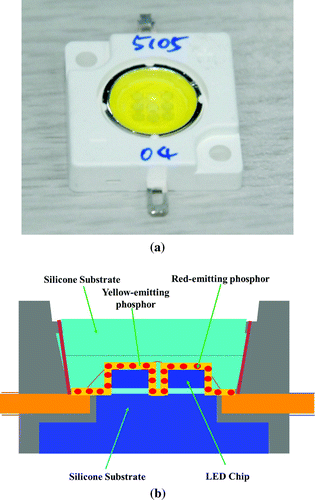
In this simulation, the phosphor layer consist of the yellow-emitting YAG:Ce and the red-emitting K2SiF6:Mn4+ conversion phosphors particles and the silicone glue, which respectively have the refractive indices of 1.85, 1.95 and 1.50. Also, the average radius of the yellow-emitting YAG:Ce phosphor particles are set to 7.25 μm like a value of real particle size. To make the color of MCW-LEDs produce the same when the concentration of the red-emitting K2SiF6:Mn4+ phosphor varies, the yellow-emitting YAG:Ce phosphor concentration should be inversely changed to provide same correlated color temperature (CCT) value. The weight percentage of the MCW-LEDs phosphor layers can be expressed as:
Here the Wsilicone, WYellow phosphor and WRed phosphor are in turn the weight percentage of the silicone glue, the yellow-emitting YAG: Ce phosphor and the red-emitting CeTb phosphors (Anh & Hsiao-Yi, Citation2016; Anh et al., Citation2017; Minh et al., Citation2017).
The influence of the concentration of the red-emitting K2SiF6:Mn4+ phosphor on the optical performance of the MCW-LEDs can be demonstrated using Mie theory (Liu & Luo, Citation2011; Winkler et al., Citation2015). Here, we can apply Mie-scattering theory (Frisvad, Christensen, & Jensen, Citation2012; Jonasz & Fournier, Citation2007; Luo & Hu, Citation2014; Mackowski, Citation2012; Mishchenko, Travis, & Lacis, Citation2002; Quinten, Citation2011; Sommer et al., Citation2011; Wriedt, Citation2012; Zhong et al., Citation2011). The scattering coefficient μsca(λ) (mm−1), the absorption coefficient μabs(λ) (mm−1), anisotropy factor g(λ) (mm−1), and reduced scattering coefficient δsca(λ) (mm−1) can be computed by the below expressions (1), (2), (3), and (4):(1)
(1)
(2)
(2)
(3)
(3)
(4)
(4)
In these equations, N(r) indicates the distribution density of diffusional particles (mm3). Cabs and Csca is the absorption and scattering cross sections (mm2), p(θ,λ,r) is the phase function, λ is the light wavelength (nm), r is the radius of diffusional particles (μm), and θ is the scattering angle (°), and f(r)) is the size distribution function of the diffuser in the phosphorous layer. Moreover, f(r) and N(r) can be calculated by:(4)
(4)
(5)
(5)
N(r) is composed of the diffusive particle number density Ndif(r) and the phosphor particle number density Nphos(r). In these equations, fdif(r) and fphos(r) are the size distribution function data of the diffusor and phosphor particle. Here KN is the number of the unit diffusor for one diffuser concentration and can be calculated by the following equation:(6)
(6)
where M(r) is the mass distribution of the unit diffuser and can be proposed by the below equation:(7)
(7)
Here ρdiff(r) and ρphos(r) are the density of diffuser and phosphor crystal.
3. Results and discussion
As displayed in Figure , the scattering coefficients grew with increasing red phosphor concentration. It means that the white-light quality can be enhanced by controlling red phosphor concentration. The reduced scattering coefficient of red phosphor with wavelengths 453, 555 and 680 nm are the same each other’s (Figure ). It indicated that the scattering stability of red phosphor is used for controlling the color quality of MCW-LEDs. The results indicated that red phosphor particles had a significant advantage in producing blue light. The more the blue light emitted, the more the yellow ring phenomenon reduced. Figure show that the anisotropy coefficient is the same of the red and the blue light. However, the anisotropy coefficient of the yellow light is higher than others. Moreover, the scattering amplitude of the blue light is highest, and the scattering amplitude of the red light is lowest in comparison with each other (Figure ).
Figure 3. Reduced scattering coefficient of the red-emitting phosphor Ba2Si5N8Eu2+ with wavelengths of 453, 555, and 680 nm.
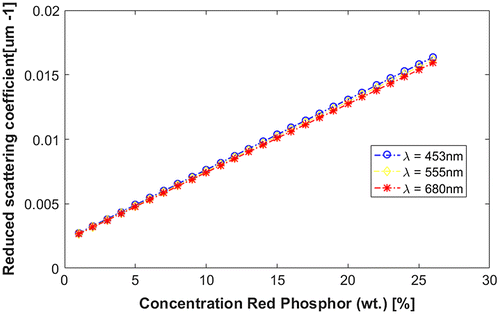
Figure 4. Anisotropy coefficient of the red-emitting phosphor Ba2Si5N8Eu2+ with wavelengths of 453, 555, and 680 nm.
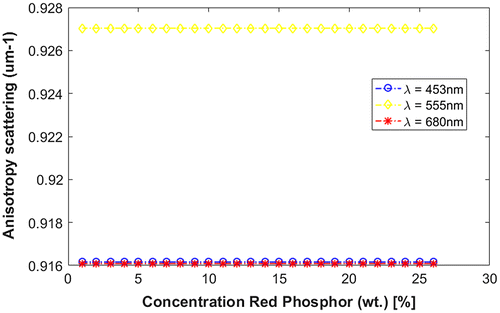
Figure 5. The scattering amplitude of the red-emitting phosphor Ba2Si5N8Eu2+ with wavelengths of 453, 555, and 680 nm.
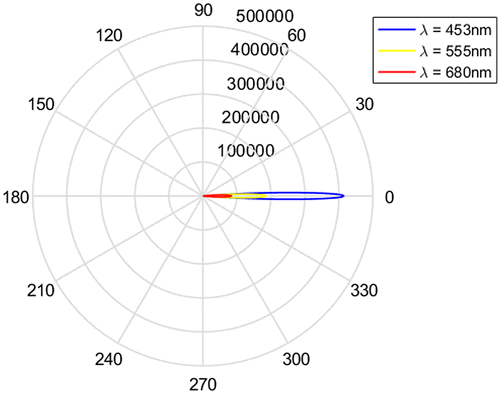
In this segment, the CRI, and CQS of the 7000K MCW-LEDs are calculated and obtained by using the commercial software Light Tools. In this simulation, the size of the red-emitting K2SiF6:Mn4+ phosphor varied continuously from 1 to 10 μm. From the simulation results, Figures and show the CQS and CRI of the 7000K conformal packaging MCW-LEDs while the size of the red phosphor changed from 1 to 10 μm. It can be indicated that the CQS and CRI grow crucially with the size of red phosphor in the range from 1 to 6 μm The highest CQS and CRI is obtained with the red weight vary from 4 to 6 μm As shown in Figures and , the CQS and CRI could be obtained highest value near 67 and 69, respectively. From that point of view, the CQS, and CRI, of high CCT MCW-LEDs can be controlled by adding red-emitting K2SiF6:Mn4+ phosphor in phosphor compound and varying its size.
4. Conclusion
The purpose of the current paper was to determine the influence of the red-emitting K2SiF6:Mn4+ conversion phosphor’s size on the color quality of the 7000K conformal packaging MCW-LEDs. The results of this investigation show that the size of the red K2SiF6:Mn4+ phosphor particles crucial influenced on the color quality of the MCW-LEDs. The both CRI, and CQS can obtain the best value at the 4–6 μm size of the red-emitting K2SiF6:Mn4+ conversion phosphor. Further studies need to be carried out to validate the influence of the red-emitting K2SiF6:Mn4+ phosphor’s concentration on the optical performance of the conformal packaging MCW-LEDs.
Acknowledgements
The authors appreciate the support of Professor Hsiao-Yi Lee, Department of Electrical Engineering, National Kaohsiung University of Applied Sciences, Kaohsiung City, Taiwan.
Additional information
Funding
Notes on contributors
Phu Tran Tin
Phu Tran Tin received the Bachelor and Master degree from Ho Chi Minh City University of Science. Now, he was a lecturer at the Faculty of Electronics Technology (FET), Industrial University of Ho Chi Minh City. His major research interests are wireless communication in 5G, energy harvesting, the performance of cognitive radio, physical layer security, and optoelectronics.
Nguyen Huu Khanh Nhan
Nguyen Huu Khanh Nhan obtained his PhD from the Institute of Research and Experiments for Electrical and Electronic Equipment, Moscow. He serves as lecturer in Faculty of Electrical and Electronics Engineering, Ton Duc Thang University, Ho Chi Minh City, Vietnam. His research interests include VLSI, MEMS and LED driver chips.
Tran Hoang Quang Minh
Tran Hoang Quang Minh received his PhD from Tomsk Polytechnic University, Tomsk, Russian Federation. His research interests include high-voltage power systems, relay protections, wireless energy transfer, and optoelectronics. He serves as Lecturer in the Faculty of Electrical and Electronics Engineering, Ton Duc Thang University, Ho Chi Minh City, Vietnam.
References
- Anh, N. D. Q., & Hsiao-Yi, L. (2016). Improving the angular color uniformity and the lumen output for multi-chip white led lamps by green Ce0.67Tb0.33MgAl11O19: Ce, Tb Phosphor. Journal of the Chinese Institute of Engineers, 39(7), 871–875. doi:10.1080/02533839.2016.1208062
- Anh, N. D., Quoc, H-Y. L., Phuong, T. T., Nhan, N. H. K., Minh, T. H. Q., & Ly, T. H. (2017). Y2O3:Eu3 phosphor: A novel solution for an increase in color rendering index of multi-chip white LED packages. Journal of the Chinese Institute of Engineers, 40(3), 228–234. doi:10.1080/02533839.2017.1299592
- Frisvad, J. R., Christensen, N. J., & Jensen, H. W. (2012). Predicting the appearance of materials using Lorenz-Mie theory. In W. Hergert & T. Wriedt (Eds.), The Mie theory (Springer Series in Optical Sciences, pp. 101–133). Springer. doi:10.1007/978-3-642-28738-1_4
- Gibney, E. (2014). Nobel for blue LED that revolutionized lighting. Nature, 514(7521), 152–153. doi:10.1038/514152a
- Jonasz, M., & Fournier, G. R. (2007). General features of scattering of light by particles in water. In Light scattering by particles in water (pp. 87–143). Amsterdam: Elsevier. doi:10.1016/b978-012388751-1/50003-x
- Liu, S., & Luo, X. (2011). Design of LED packaging applications. In LED packaging for lighting applications (pp. 215–315). Wiley. doi:10.1002/9780470827857.ch6
- Li, S., Wang, K., Chen, F., Zhao, S., Zhao, Z., & Liu, S. (2012). Angular color uniformity enhancement of phosphor converted white LEDs integrated with compact modified freeform TIR components. 2012 13th international conference on electronic packaging technology & high density packaging, IEEE. doi:10.1109/icept-hdp.2012.6474888
- Liu, Z., Liu, S., Wang, K., & Luo, X. (2008a). Analysis of factors affecting color distribution of white LEDs. 2008 International Conference on Electronic Packaging Technology & High Density Packaging, IEEE. doi:10.1109/icept.2008.4607013
- Liu, Z., Liu, S., Wang, K., & Luo, X. (2008b). Effects of phosphor’s location on LED packaging performance. 2008 International Conference on Electronic Packaging Technology & High Density Packaging, IEEE. doi:10.1109/icept.2008.4606982
- Liu, Z., Liu, S., Wang, K., & Luo, X. (2008c). Optical analysis of color distribution in white LEDs with various packaging methods. IEEE Photonics Technology Letters, 20(24), 2027–2029. doi:10.1109/lpt.2008.2005998
- Luo, X., & Hu, R. (2014). Chip packaging: Encapsulation of nitride LEDs. In J. Huang, H-C. Kuo, & S-C. Shen (Eds.), Nitride semiconductor light-emitting diodes (LEDs) (pp. 441–481). Oxford: Woodhead publishing. doi:10.1533/9780857099303.2.441
- Luo, X., Run, H., Liu, S., & Wang, K. (2016). Heat and fluid flow in high-power led packaging and applications. Progress in Energy and Combustion Science, 56, 1–32. doi:10.1016/j.pecs.2016.05.003
- Mackowski, D. (2012). The extension of Mie theory to multiple spheres. In W. Hergert & T. Wriedt (Eds.), The Mie theory (Springer Series in Optical Sciences, pp. 223–256). Springer. doi:10.1007/978-3-642-28738-1_8
- Minh, T. H., Quang, N. H., Nhan, K., Anh, N. D. Q., & Lee, Hsiao-Yi (2017). Red-emitting α-SrO·3B2O3:Sm2 phosphor: An innovative application for increasing color quality and luminous flux of remote phosphor white LEDs. Journal of the Chinese Institute of Engineers, 40(4), 313–317. doi:10.1080/02533839.2017.1318720
- Mishchenko, M. I., Travis, L. D., & Lacis, A. A. (2002). Scattering, absorption, and emission of light by small particles. Cambridge: Cambridge University Press.
- Quinten, M. (2011). Beyond Mie’s Theory II – The generalized Mie theory. Optical Properties of Nanoparticle Systems (pp. 317–339). Weinheim: Wiley. doi:10.1002/9783527633135.ch10
- Shuai, Y., He, Y., Tran, N. T., & Shi, F. G. (2011). Angular CCT uniformity of phosphor-converted white LEDs: Effects of phosphor materials and packaging structures. IEEE Photonics Technology Letters, 23(3), 137–139. doi:10.1109/LPT.2010.2092759
- Sijbom, H. F., Verstraete, R., Joos, J. J., Poelman, D., & Smet, P. F. (2017, August). K2SiF6:Mn4+ as a red phosphor for displays and warm-white LEDs: A review of properties and perspectives. Optical Materials Express, 7(9), 3332. doi:10.1364/ome.7.003332
- Smet, P. F., Parmentier, A. B., & Poelman, D. (2011). Selecting conversion phosphors for white light-emitting diodes. Journal of the Electrochemical Society, 158(6), R37–R54. doi:10.1149/1.3568524
- Sommer, C., Reil, F., Krenn, J. R., Hartmann, P., Pachler, P., Hoschopf, H., & Wenzl, F. P. (2011). The impact of light scattering on the radiant flux of phosphor-converted high power white light-emitting diodes. Journal of Lightwave Technology, 29(15), 2285–2291. doi:10.1109/jlt.2011.2158987
- Sommer, C., Wenzl, F-P., Hartmann, P., Pachler, P., Schweighart, M., Tasch, S., & Leising, G. (2008). Tailoring of the color conversion elements in phosphor-converted high-power leds by optical simulations. IEEE Photonics Technology Letters, 20(9), 739–741. doi:10.1109/lpt.2008.921063
- Tran, N. T., & Shi, F. G. (2008). Studies of phosphor concentration and thickness for phosphor-based white light-emitting-diodes. Journal of Lightwave Technology, 26(21), 3556–3559. doi:10.1109/jlt.2008.917087
- Wang, Y., Li, H., Zhang, R., Zhan, M., & Zhang, J. (2015). Investigations on the luminescence of emission-tunable Ca10K(PO4)7:Eu2, Sr2, Mg2 phosphors for white LEDs. RSC Advances, 5(4), 2689–2693. doi:10.1039/c4ra11198b
- Winkler, H., Trinh, Q., Bodrogi, P., & Khanh, T. Q. (2015). LED lighting: Technology and perception. Weinheim: Wiley-VCH.
- Wriedt, T. (2012). Mie theory: A review. In W. Hergert & T. Wriedt (Eds.), The Mie theory (Springer Series in Optical Sciences, pp. 53–71). Springer. doi:10.1007/978-3-642-28738-1_2
- Zhong, J., Xie, M., Zhigui, Q., Zhang, R., Huang, M., & Zhao, F. (2011). Mie theory simulation of the effect on light extraction by 2-D nanostructure fabrication. 2011 symposium on photonics and optoelectronics (SOPO), IEEE. doi:10.1109/sopo.2011.5780566