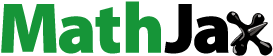
Abstract
Pakistan is primarily an agriculture country with the capability of producing wheat, cotton, sugarcane and rice, which together are more than 75% of the total crop output. Underground water is the main source of irrigation. Most of the water pumps use diesel generator while others run on electricity. The diesel is very expensive and electric supply situation is very unreliable in Pakistan. Photovoltaic (PV) powered water pumps are an attractive alternative solution, but due to the high cost of solar panels, they are not within the reach of a common farmer. In this paper, a novel architecture for an irrigation water pump, simultaneously powered by utility and PV panels, is proposed. No battery backup is required. The system employs maximum power point tracking. The pump controller receives a single control input to produce desired water flow rate and concurrently maximizing the utilization of PV resource. The proposed solution allows the farmer to incrementally add solar panels to an existing grid powered pumping system, thereby reducing the initial investment required for full solar deployment. The investment in the partial deployment of solar panels is paid back to the farmer in reduced electricity tariff.
Public Interest Statement
Water is a significant resource for the social, economic and sustainable development of developing countries like Pakistan. Agriculture is a backbone of Pakistan’s economy. The timely availability of water is critical for food crop production. The average availability of electricity from the grid is around 8–12 h per day in rural agriculture areas. Most of the water pumps use diesel generator while others run on electricity. The diesel is costly and electric supply situation is very unreliable. Therefore, food crop production is becoming a major concern due to insufficient rainfall and load shedding. The stand-alone solar powered water pumps are too expensive for most farmers. The objective of this research is to provide a reliable and economical solution for water pumping. A novel hybrid solar/utility powered irrigation water pumping system is investigated in this research. The solution works with any installed solar capacity with existing grid powered pump, thus reducing initial investment, while decreasing their long-term energy costs, thus balancing economic development and environmental sustainability.
1. Introduction
Water is a significant resource for the social, economic and sustainable development of Pakistan. From this perspective, the irrigated agriculture is of great importance in the socioeconomic life of the country. The agriculture is a major occupation in Pakistan. The agriculture in Pakistan largely depends upon irrigation. In Pakistan, the irrigated terrains supply more than 90% of the total agriculture production. It accounts for about 24.9% of GDP and employs around 49% of the labor force, according to Punjab Irrigation and Power Department (http://irrigation.punjab.gov.pk). The need of food grains is increasing day by day due to population growth. The role of the farmer is important in improving the food grain situation. So, farmers need to be supported in all aspects. The availability of water in time is crucial prerequisite for crop production to meet the agriculture needs. Due to insufficient rainfall and load shedding, food crop production is becoming a major problem in Pakistan.
The government of Pakistan has taken up many irrigation projects to provide barrage and canal irrigation, but due to geographical location, the coverage area is limited. According to the Alternative Energy Development Board, Government of Pakistan, (http://www.aedb.org) around one million agriculture tube wells are in operation at present and nearly 30% are operated by the national electric grid. It consumes approximately 15–20% of the total energy delivered by the electric grid. Due to power shortage in Pakistan, Government of Pakistan is increasing electricity price for agriculture tube wells. The average availability of electricity from the grid is around 8–12 h per day in rural remote areas. Due to the current energy crisis and high electricity tariff, the agriculture sector in Pakistan having groundwater as the source of irrigation is badly affected. Therefore, an efficient, reliable and cost-effective energy solution for the agriculture sector in Pakistan is needed for irrigation.
The solar water pumping system is the promising solution for irrigation. It is a clean, environmentally friendly and reliable source of supplying water that requires low maintenance. It has no running cost and long life as compared to a diesel generator. The amount of power extracted directly from solar panels is not sufficient, in terms of voltage and current, for running water pumping motor. The power electronic converter plays important role in system configuration. Different power electronic converters are used for maximum energy harvesting. The maximum power point tracking (MPPT) is used for extracting maximum energy from photovoltaic (PV) panels. MPPT is a not a physical tracking mechanism but an electronic method to operate PV panel at the optimal point. The DC–DC converter is employed for MPPT. The single stage water pumping configurations require the only inverter connected directly with PV panel cutting the cost of DC–DC converter. However, the inverter is responsible for both motor speed control and MPPT. A low cost and high-efficiency converter is proposed by Caracas, De Carvalho Farias, Teixeira, and De Souza Ribeiro (Citation2014) for autonomous solar water pumping system. Another important factor is the use of batteries for storing energy in solar water pumping system. The system with batteries is not considered a promising solution as the batteries require regular maintenance and increase the system cost. There are currently three different pumping configurations that are mostly used:
1. DC drives with positive displacement pumps.
2. AC drive powering induction motor/centrifugal pump.
3. AC drive powering a three-phase permanent magnet synchronous motor.
The selection criteria of motors depend on various factors including cost, compatibility and efficiency. The first-generation PV pumps incorporated permanent-magnet DC motors, for low-to-medium head applications. However, the DC motor is not a suitable candidate for water pumping due to its commutator and brushes arrangement. Furthermore, the initial and maintenance costs of DC motor are higher. The induction motor is suitable for solar water pumping considering disadvantages of DC motor. Nowadays, induction motor driven by variable speed inverter is a standard motor for solar water pumping applications. This is because of its simplicity, low price and robustness compared to DC motor (Vitorino, De Rossiter Corrêa, Jacobina, & Lima, Citation2011). In the last 5 years, the electronically commutated brushless DC motors have also been used in solar pumping applications because it requires less maintenance (Kumar & Singh, Citation2017). Recently, a permanent magnet synchronous motor has been employed for PV water pumping system (Antonello, Carraro, Costabeber, Tinazzi, & Zigliotto, Citation2017). It has high torque density, high efficiency and small size. But it requires special magnetics design and is quite expensive. Switched reluctance motor has been reported in the literature for solar water pumping system (Singh, Mishra, & Kumar, Citation2016). However, it requires complex control and generates high noise. The centrifugal and positive displacement pumps are commonly used in solar water pumping systems. The centrifugal pumps are widely used due to their robustness, simplicity and low maintenance requirement (Elrefai, Hamdy, ElZawawi, & Hamad, Citation2016). In solar powered water pumping system, the sun irradiance can change due to climate conditions. The level of insolation for the clear, cloudy and overcast day is shown in Figure (Thomas, Citation1987). During the cloudy season when the insolation level is low, the motor stops pumping water due to low power. The literature survey indicates that AC motor with a variable frequency inverter is optimized at low speed during low irradiation (Eskander & Zaki, Citation1997; Santiago-Gonzalez, Cruz-Colon, Otero-De-Leon, Lopez-Santiago, & Ortiz-Rivera, Citation2011). The various control algorithms, different types of power electronic converters and motors are proposed by the researchers in order to improve system reliability, efficiency and performance, while decreasing cost and complexity (Al-Badi et al., Citation2018; Antonello et al., Citation2017; Ashhab, Citation2008; Asumadu-Sarkodie, Sevinç, & Jayaweera, Citation2016; El-Shimy, Citation2013; Franklin, Cerqueira, & De Santana, Citation2014; Khatib, Mohamed, Sopian, & Mahmoud, Citation2013; Narayana, Mishra, & Singh, Citation2017; Rehman & Sahin, Citation2016; Rezk, Citation2016; Sawle, Gupta, & Kumar Bohre, Citation2016; Sharma, Kumar, & Singh, Citation2016; Singh et al., Citation2016). However, these studies have not considered the performance and control of hybrid utility–solar PV water pumping system. In this regard, a novel hybrid water pumping system is presented and investigated in this research. The overall objective of the proposed system is to drive a water pump partially powered from PV while taking the remaining power from the grid. If utility power is not available, due to load shedding, which is a very common scenario in rural areas in developing countries, the pump will run from available solar power at a reduced flow rate. If the PV power is not available, due to cloudy weather, then the motor will take all the required power from the utility. The advantage of mixing power from the utility and solar panels is twofold; initial investment is lower and the electricity cost from the grid is reduced proportionally to the amount of installed PV capacity.
2. Description of proposed water pumping system
The proposed system is shown in Figure . In the proposed systems, each PV panel output is extracted through a MPPT DC/DC converter in order to get maximum power from PV panels. If the sun irradiation on one PV panel decreases, it does not affect other solar panels, because each PV panel has an independent MPPT controller, thus avoiding partial shading effects. Utility AC power is converted to DC by a three-phase rectifier. The outputs from DC/DC converter and three-phase rectifier are connected to common DC bus. This DC voltage is used to drive a three-phase voltage source inverter, which provides the power to the motor. The motor speed is regulated for a given flow rate using variable frequency inverter. This is an efficient way of driving motor according to desired flow rate. At daytime, solar power is utilized, and utility power is saved, thus saving electricity bill. So, an optimal way is developed and tested to drive motor, partially powered by solar panels, while taking remaining power from the utility.
The combined utility and solar powered pumping system is the best solution for varying insolation. When the insolation level decreases, the MPPT tracks the new maximum power point (MPP) and utility supplies the remaining power for required power demand. When the insolation increases, the MPPT tracks the new MPP, and in this case, power from utility decreases. If solar power is zero, then the motor will run on utility power.
2.1. Design of DC–DC converter
A flyback converter is used for implementing MPPT. The flyback converter is derived from the buck–boost converter and provides isolation between input and output voltage. The turn ratio of the transformer provides increased design flexibility in the transfer relationship. A 500-W PV panel is used as the input of the converter. The transformer equivalent circuit that includes the magnetizing inductance is shown in Figure (Hart, Citation2011).
The flyback converter is designed based on specifications shown in Table . The value of magnetizing inductance is selected to ensure that flyback converter always operates in continuous conduction mode (CCM). The minimum value of magnetizing inductance required for CCM operation is (Hart, Citation2011)
Table 1. Parameters of flyback converter
The flyback converter will operate in discontinuous conduction mode when power demand at the output is very low. The value of output capacitor is computed to ensure that output voltage ripple does not exceed 5% of the average output voltage.
2.2. Modeling of PV cell and maximum power point tracking
The equivalent electric circuit of PV cell is used to study and model the input–output characteristic of the solar cell. A typical equivalent circuit model of PV cell is shown in Figure (González-Longatt, Citation2005). The model comprises a current source, a diode and series resistance. This does not consist of parallel resistance because its effect is very small. The temperature effect on diode saturation current is included in this model. The effect of temperature on photo current
is also included in the model (González-Longatt, Citation2005).
The total current of the cell is the difference of photo current
and diode current
.
where = Cell voltage
= Cell temperature
The temperature-dependent photocurrent is
where is given in data sheet (measured under illumination of
)
is the reference temperature (25°C)
The diode saturation current at the reference temperature is
The diode saturation current at any other temperature is calculated by the following equation:
A typically I–V and P–V curve for a 500-W PV module, identifying and
, and
and
, is shown in Figure . Power delivered is zero at the end of curve. At any other point on the I–V curve, PV module deliver certain power and that point is called operating point. The unique point occurs near the knee of I–V curve, where power is maximum, and that point is called MPP. The module produces the maximum power at MPP, as shown in Figure .
Perturb & Observer (P&O) method is used for MPPT. P&O method is most common and widely used algorithm for tracking the MPP of PV module (Anurag, Bal, Sourav, & Nanda, Citation2016). The flowchart of P&O is shown in Figure .
2.3. Modeling of pump
The centrifugal pumps are normally used for low-to-middle head applications. The power required by the pump is given as
By applying the principle of motor-pump power balance equation (Khader & Daud, Citation2013), the above can be integrated with motor speed-torque performance as
The water flow rate can be written as
In this study, following pump parameters are selected:
The speed of pump for a given flow rate is determined by centrifugal pump equation which is
In this equation, a, b and c are constant which can be determined from pump geometry (Ghafouri, Khayatzadeh, & Khayatzadeh, Citation2012). For a given flow rate, the speed of the motor is determined, and the motor speed is regulated to desired speed to meet water demand.
3. Economic analysis
The economic analysis is very important to compare quantitative cost and benefit information. The goal of the economic analysis is to compare the proposed hybrid water pumping system with standalone PV powered water pumping systems. In economic analysis, life cycle cost (LCC), net present value (NPV) and the payback period are calculated. LCC is a key factor to be considered with the investment cost. It includes capital cost, maintenance cost, replacement costs and operational cost.
where CC is the capital cost, MC is the maintenance cost, FC is the fuel cost, RC is the replacement cost, SV is the salvage value.
NPV shows the value of the entire system at the end of life cycle. It is the difference between the sum of discounted cash flows and initial invested amount.
Payback period is the core part of the project. Payback period measures the time required to recover the investment cost. Shorter payback period is preferred and indicates the feasibility of the project.
A 10 hp (7.46 kW) motor is considered for pumping water from underground. The grid running cost is calculated according to current electricity tariff for agriculture in Pakistan. The operating hours are taken according to sun hours (8 h/day). In case 1, grid running system is replaced with standalone solar water pumping. The cost analysis is performed according to current market prices of all equipment including solar panels, pump, drilled bore, mounting structure, inverter, controller, installation, cabling, grounding etc. The solar panel operating life is taken 25 years. The capital cost of a 10-hp standalone solar powered system is $19,937, and the payback period is 3.1 years. In case 2, only a few solar panels are installed, instead of full solar deployment. The farmer can install more solar panels in future with the existing system thus decreasing initial investment. In this case, only 2 kW solar power capacity is installed and integrated with the existing grid powered system. In this case, the capital cost is $4380, and the payback period is 0.8 year. The capital cost and payback period are shown in Figure . The proposed system has much lower capital cost and the farmer can incrementally add more solar panels to the existing system in future, thus decreasing initial investment.
4. Design of controller for proposed system
In the proposed system, the input variable is flow rate Q [m3/h]. The speed controller regulates the speed of the motor to the exact desired flow rate. If the utility is connected, the DC bus on the drive inverter remains stiff, the voltage is controlled by the utility and an increase in the flow rate demand is catered by increasing input power from the utility. In absence of utility power, the motor speed is limited by available input power from PV which depends on the size of panel and amount of insolation. In a typical scenario, without utility, the speed of the motor cannot be always regulated to meet the desired flow rate. In some case, where the load power is lower than PV potential, the PV panel is not operated at MPPT; instead, the control algorithm regulates DC bus voltage to 540 V by pulling down the duty cycle. If the load power is increased, the flyback converter output voltage starts to fall down below the set point, and MPPT control acts to regulate the voltage. The control structure for flyback converter is depicted in Figure .
The motor speed controller is shown in Figure . The speed controller calculates the motor slip. The value of slip computed by the controller is added to the actual motor speed to produce the required inverter frequency. This frequency is also used to produce the required inverter voltage to maintain V/f ratio constant. Space vector pulse width modulation scheme is used to control the speed of the motor (Gajdusek, Citation2012).
5. Results and discussion
The proposed system is studied and analyzed in two cases under different flow rates.
5.1. Case I
In this case, a single PV panel with a nominal power of 500 W is used. The result of the complete system is displayed for various water flow rate and corresponding reference and actual speed. The speed of the motor is regulated according to water demand, which significantly reduces the power consumption. The flow rates are set [0.8 1.2 1.4 1 m3/h] against time [0 1 2 3 s] respectively.
To meet desired flow rates, the power required from utility and solar power profile is shown in Figure . The motor mechanical speed, electromagnetic torque and DC bus voltage are shown in Figure .
The result shows that solar panel is operating at MPP and output power is 500 W. From the results, the motor is running from available solar power, and while taking remaining power from the utility. The 500-W power is coming from PV panel and remaining required power is added from the utility. The power demand depends on water flow rate. The system is mixing, both solar and utility power, to meet load power demand. The DC bus voltage increases, when the speed of the motor is decreased. This is because the mechanical power converted back into electrical, which charges the DC bus capacitor.
5.2. Case II
In this case, utility power is not available, which is common in rural areas. Two PV panels are used in this study in order to analyze the behavior of the proposed system. If utility power is not available, then for a given flow rate, the speed of the motor is regulated, while maintaining DC bus voltage at 540 V. If for a given flow rate the required output power is higher than available solar power, then DC bus voltage collapses. In this case, the motor speed is decreased to maintain DC bus voltage at set point. If solar power is more than the desired flow rate power requirement, then DC bus voltage increases. So, in such case, pump controller pulls down the duty cycle of MPPT, while maintaining DC bus voltage at set point.
The flow rates are set [0.6 0.8 1 0.8 m3/h] against time [0 1 2 3.5 s] respectively. The power profile of solar panels is shown in Figure . The motor mechanical speed, electromagnetic torque and DC bus voltage are shown in Figure .
The results demonstrate that the controller is operating PV panels according to the desired flow rate. The PV panels are not operated at MPP when load power demand is low. When the load power demand is high, then pump controller operates PV panels at MPP, thus meeting the desired flow rate, while regulating DC bus voltage.
6. Conclusions
This research has presented a novel arrangement for an irrigation pump, powered by both utility and solar panels. The MPPT is implemented using flyback converter to maximize solar energy harvesting. The power obtained from solar and utility are added at DC bus, which is used to drive three-phase AC induction motor. The results are presented to validate the operation of the proposed system. The advantage of this system is that the solution works with any number of installed panels, thus reducing initial investment, which is very high due to the cost of panels. The purpose of this research is to provide a reliable and cost-effective solution to the irrigation needs of the farmer in Pakistan. The farmer can incrementally add solar panels in future to the existing system, thereby reducing dependency on the utility power and electricity bills. This solution can lift farmers out of poverty by enabling them to better irrigate their crop more affordable, while decreasing their long-term energy costs, thus balancing economic development and environmental sustainability.
Nomenclature
PV: | = | Photovoltaic |
DC: | = | Direct current |
| = | Open circuit voltage |
| = | Short circuit current |
I–V: | = | Current–voltage |
P–V: | = | Power–voltage |
V: | = | Cell voltage |
| = | Ripple in the voltage |
C: | = | Capacitance |
f: | = | Frequency |
Hz: | = | Hertz |
A: | = | Ampere |
W: | = | Watt |
rpm: | = | Revolution per minute |
| = | Diode reverse saturation current |
| = | Series resistance |
| = | Magnetizing inductance of flyback converter |
G: | = | Irradiance ( |
n: | = | Diode quality factor |
| = | Photocurrent |
T: | = | Temperature (℃) |
K: | = | Boltzmann’s constant ( |
MPPT: | = | Maximum power point tracking |
MPP: | = | Maximum power point |
| = | PV module voltage at maximum power point |
| = | PV module current at maximum power point |
P&O: | = | Perturb & Observer |
VSI: | = | Voltage source inverter |
Q: | = | Flow rate |
| = | Fluid density |
g: | = | Gravity force |
H: | = | Head of pump |
| = | Efficiency |
CC: | = | Capital cost |
MC: | = | Maintenance cost |
FC: | = | Fuel cost |
RC: | = | Replacement cost |
SV: | = | Salvage value |
LCC: | = | Life cycle cost |
NPV: | = | Net present value |
i: | = | Interest rate |
D: | = | Duty cycle |
Additional information
Funding
Notes on contributors
Waqas Hassan
Mr. Waqas Hassan has worked as a Lecturer at CASE from September 2011 to March 2017. He earned Bachelor (B.Sc.) and Master (MS) degrees in electrical engineering from CASE. He uniquely specializes in the field of electrical energy and control system. His areas of interest are power electronics, renewable energy, nanogrid and smart grid. He is pursuing Ph.D. degree in power electronic interfaces for DC nanogrid from the University of Sydney, NSW, Australia.
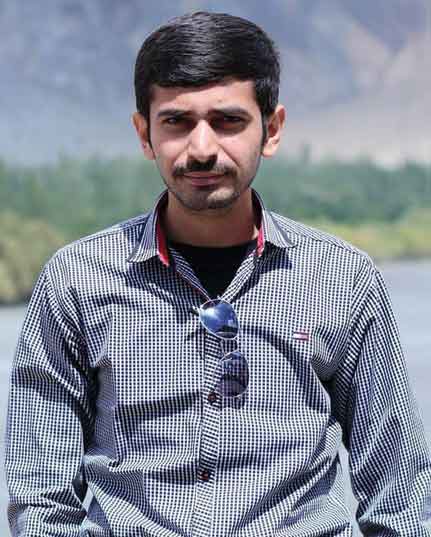
Farrukh Kamran
Dr. Farrukh Kamran is Professor of Electrical Engineering at CASE, Chief Technology Officer at Sky Electric (Pvt.) Ltd. (www.skyelectric.com) and Vice President (Engineering) at CARE (Pvt.) Ltd. (www.carepvtltd.com). He holds MS and Ph.D. degrees in electrical engineering from Georgia Institute of Technology, Atlanta, GA, USA. He specializes in electronic/digital system design including power electronics and communication systems. He has 17 years of teaching and industry experience. He has authored or coauthored more than 30 technical papers, 6 of these have been published in IEEE Transactions on Power Electronics and Industry Applications.
References
- Al-Badi, A. , Yousef, H. , Al Mahmoudi, T. , Al-Shammaki, M. , Al-Abri, A. , & Al-Hinai, A. (2018). Sizing and modelling of photovoltaic water pumping system. International Journal of Sustainable Energy , 37(5), 415-427.
- Alternative Energy Development Board (AEDB) . (2017). Retrieved from http://www.aedb.org
- Antonello, R. , Carraro, M. , Costabeber, A. , Tinazzi, F. , & Zigliotto, M. (2017). Energy-efficient autonomous solar water-pumping system for permanent-magnet synchronous motors. IEEE Transactions on Industrial Electronics , 64(1), 43–51. doi:10.1109/TIE.2016.2595480
- Anurag, A. , Bal, S. , Sourav, S. , & Nanda, M. (2016). A review of maximum power-point tracking techniques for photovoltaic systems. International Journal of Sustainable Energy , 35(5), 478–501. doi:10.1080/14786451.2014.918979
- Ashhab, M. D. S. S. (2008). Optimization and modeling of a photovoltaic solar integrated system by neural networks. Energy Conversion and Management , 49(11), 3349–3355. doi:10.1016/j.enconman.2007.10.036
- Asumadu-Sarkodie, S. , Sevinç, Ҫ. , & Jayaweera, H. M. (2016). A hybrid solar photovoltaic-wind turbine-rankine cycle for electricity generation in Turkish Republic of Northern Cyprus. Cogent Engineering , 3(1), 1180740. doi:10.1080/23311916.2016.1180740
- Caracas, J. V. M. , De Carvalho Farias, G. , Teixeira, L. F. M. , & De Souza Ribeiro, L. A. (2014). Implementation of a high-efficiency, high-lifetime, and low-cost converter for an autonomous photovoltaic water pumping system. IEEE Transactions on Industry Applications , 50(1), 631–641. doi:10.1109/TIA.2013.2271214
- Elrefai, M. , Hamdy, R. A. , ElZawawi, A. , & Hamad, M. S. (2016, December). Design and performance evaluation of a solar water pumping system: A case study. In Power Systems Conference (MEPCON), 2016 Eighteenth International Middle East (pp. 914–920). Cairo, Egypt: IEEE.
- El-Shimy, M. (2013). Sizing optimisation of stand-alone photovoltaic generators for irrigation water pumping systems. International Journal of Sustainable Energy , 32(5), 333–350. doi:10.1080/14786451.2012.697463
- Eskander, M. N. , & Zaki, A. M. (1997). A maximum efficiency-photovoltaic-induction motor pump system. Renewable Energy , 10(1), 53–60. doi:10.1016/0960-1481(96)00004-3
- Franklin, T. , Cerqueira, J. J. F. , & De Santana, E. (2014). Fuzzy and PI controllers in pumping water system using photovoltaic electric generation. IEEE Latin America Transactions , 12(6), 1049–1054. doi:10.1109/TLA.2014.6893999
- Gajdusek, P. (2012). Programmable laboratory invertor and space vector PWM . Department of Electrical Power Engineering, FEEC, VUT.
- Ghafouri, J. , Khayatzadeh, H. , & Khayatzadeh, A. (2012). Dynamic modeling of variable speed centrifugal pump utilizing MATLAB/SIMULINK. International Journal of Science and Engineering Investigations , 1(5), 1–7.
- González-Longatt, F. M. (2005). Model of photovoltaic module in Matlab. Ii Cibelec , 2005, 1–5.
- Hart, D. W. (2011). Power electronics . Tata McGraw-Hill Education.
- Khader, S. , & Daud, A.-K. (2013). PV-grid tie system energizing water pump. Smart Grid and Renewable Energy , 4(5), 409–418. doi:10.4236/sgre.2013.45047
- Khatib, T. , Mohamed, A. , Sopian, K. , & Mahmoud, M. (2013). Optimal sizing of the energy sources in hybrid PV/diesel systems: A case study for Malaysia. International Journal of Green Energy , 10(1), 41–52. doi:10.1080/15435075.2011.647173
- Kumar, R. , & Singh, B. (2017). Single stage solar PV fed brushless DC motor driven water pump. IEEE Journal of Emerging and Selected Topics in Power Electronics , 5(3), 1377–1385. doi:10.1109/JESTPE.2017.2699918
- Narayana, V. , Mishra, A. K. , & Singh, B. (2017). Development of low-cost PV array-fed SRM drive-based water pumping system utilising CSC converter. IET Power Electronics , 10(2), 156–168. doi:10.1049/iet-pel.2016.0112
- Rehman, S. , & Sahin, A. Z. (2016). A wind-solar PV hybrid power system with battery backup for water pumping in remote localities. International Journal of Green Energy , 13(11), 1075–1083. doi:10.1080/15435075.2012.729169
- Rezk, H. (2016). A comprehensive sizing methodology for stand-alone battery-less photovoltaic water pumping system under the Egyptian climate. Cogent Engineering , 3(1), 1242110. doi:10.1080/23311916.2016.1242110
- Santiago-Gonzalez, J. , Cruz-Colon, J. , Otero-De-Leon, R. , Lopez-Santiago, V. , & Ortiz-Rivera, E. (2011). Three phase induction motor drive using flyback converter and PWM inverter fed from a single photovoltaic panel. In Power and Energy Society General Meeting, 2011 (pp. 1-6). Michigan: IEEE.
- Sawle, Y. , Gupta, S. , & Kumar Bohre, A. (2016). PV-wind hybrid system: A review with case study. Cogent Engineering , 3(1), 1189305. doi:10.1080/23311916.2016.1189305
- Sharma, U. , Kumar, S. , & Singh, B. (2016). Solar array fed water pumping system using induction motor drive. In Power Electronics, Intelligent Control and Energy Systems (ICPEICES) , IEEE International Conference (pp. 1-6). Delhi: IEEE.
- Singh, B. , Mishra, A. K. , & Kumar, R. (2016). Solar powered water pumping system employing switched reluctance motor drive. IEEE Transactions on Industry Applications , 52(5), 3949–3957. doi:10.1109/TIA.2016.2564945
- Thomas, M. G. (1987). Water pumping: The solar alternative (No. SAND87-0804; ALSNL199600000193). Sandia National Laboratories (SNL-NM), Albuquerque, NM (United States).
- Vitorino, M. A. , De Rossiter Corrêa, M. B. , Jacobina, C. B. , & Lima, A. M. N. (2011). An effective induction motor control for photovoltaic pumping. IEEE Transactions on Industrial Electronics , 58(4), 1162–1170. doi:10.1109/TIE.2010.2054053