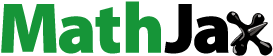
Abstract
Membrane bioreactor (MBR) is a progressive alternative to conventional waste-water treatment. However, membrane fouling is one of the most significant barriers to further development of the MBR technology since it has a detrimental effect on system performance and stability. Therefore, a number of studies have been developed to comprehend the fouling nature and distinguish the effective flux enhancers, so as to develop a highly effective fouling control strategy. Key findings of these studies are summarized in the current review. Polysaccharides, proteins and humic substances tend to play the prime role in inducing fouling. The main factors affecting fouling behaviour in MBRs are membrane characteristics, membrane operation conditions and activated sludge properties, and recent evidence points out that the modification of the biomass characteristics with the use of chemical agents and adsorbents is among the most efficient fouling-hindering techniques. The current review not only aims to provide a comprehensive overview of the up-to-date methods of fouling alleviation using membrane flux enhancers in MBR but also intends to shed light on the mechanisms of their action, which, together with the experience of previous findings, can be the basis for developing a new advanced fouling mitigation strategy.
Keywords:
PUBLIC INTEREST STATEMENT
Water crisis and hunger are among the most urgent global problems of the current century. Therefore, clean water and sanitation and zero hunger are among the prime goals of the 2030 Agenda for Sustainable Development adopted by the United Nations. This brings a strong need for resource recovery and water reuse. The MBR process is among the most sophisticated methods for future water reuse due to its exceptional capabilities. However, membrane fouling is the main barrier towards the increase of its economic efficiency. Based on a number of research works, this review intends to provide a holistic overview of the cutting-edge technologies of fouling mitigation, and, hence, an increase of system efficiency. The provided results can serve as a basis for developing a new advanced fouling mitigation strategy, which has the potential to make MBR systems a dominating technology in the cost-sensitive water-reuse markets.
Competing Interests
The authors declare no competing interests of a personal, professional or financial nature.
1. Introduction
Excellent nutrient removal efficiency, compactness, complete biomass retention without a secondary clarifier, together with low carbon footprint increase the competitiveness of membrane bioreactor (MBR) technology in municipal and industrial waste-water treatments. In addition, stringent environmental regulations and shift of the waste-water management paradigm towards the circular economy, resulting in high-quality effluent requirements, will drive the expansion of the global MBR market to 8.27 USD billion by 2025 (Research and Markets, Citation2014).
Risk of high operating costs, primarily attributed to membrane fouling, and the need for skilled technical support, able to deal with fouling events, are the main restraints to the further penetration of MBR into cost-sensitive markets, including small communities and developing countries. Straightforward decrease of membrane flux in order to alleviate fouling will increase the capital investments due to the rising demand in the membrane area and therefore is not a market-wise solution.
Membrane fouling in MBR has been a subject of numerous academic studies, focused on fouling mechanisms and prevention and control strategies, which also represents the practical interest of the waste-water treatment industry (Gkotsis, Banti, Peleka, Zouboulis, & Samaras, Citation2014). According to the Web of Science, the number of publications related to “MBR” and “fouling” is over 1700, with average citation rating 14.8, including major contributions from China—601 papers, USA—154 papers and Australia—157 papers. All these studies pursue one goal—to improve the understanding of fouling mechanisms: current challenges, research needs and to provide practical inputs on fouling mitigation for the industry.
Several comprehensive analytical reviews, published the since early 2000s, have summarized studies on membrane fouling in MBR (Table ). According to the Scopus, the works of Drews (Citation2010), Judd (Citation2008), Le-Clech, Chen, and Fane (Citation2006), Meng et al. (Citation2009) and Porcelli and Judd (Citation2010) are the most comprehensive and highly cited. These reviews perfectly cover the identification, investigation and characterization of membrane fouling as well as the optimization of MBR operating conditions for fouling mitigation. The latest one has been published by Meng et al. (Citation2017). It updates the progress in the fundamental understanding of MBR fouling and fouling control strategies, summarizes recent findings on the composition and characteristics of foulants and microbial ecology in bio-cake layers as well as novel fouling control strategies in lab-, pilot- and full-scale MBRs.
Table 1. Prior art reviews on membrane fouling in MBR
It appears from the available reviews that the chemical cleaning of membranes, including chemically enhanced backwash (CEB) and cleaning in place (CIP), is generally considered as the essential practical process of membrane permeability recovery while dealing with fouling (Meng et al., Citation2017). At the same time, all the above-mentioned authors associate chemical cleaning with numerous adverse effects to membrane integrity and sludge activity due to the frequent exposure of membranes and biomass to aggressive chemicals (D. Zhao & Yu, Citation2015). The remaining fouling mitigation strategies either are based on the mechanical scouring effects of limited efficiency and applicability (Hoffmann & Krause, Citation2013) or have not matured to the level of validation in operational or sometimes even in a relevant environment.
Notably, the existing reviews identify the prospective concept of membrane flux enhancement in MBR but provide very limited analysis of publications related to this research. Membrane flux enhancement in MBR is the concept of dosing various additives to the MBR mixed liquor to improve filterability. In this case, additives can act by various mechanisms: adsorption, coagulation, flocculation, oxidation, etc. Limited studies are also available on a combination of additives (Drews, Citation2010; Ji, Li, Qiu, & Li, Citation2014; J. C. Lee et al., Citation2001; Nguyen, Guo, Ngo, & Vigneswaran, Citation2010; Zarei, Moslemi, & Mirzaei, Citation2016). Therefore, membrane flux enhancement is, by nature, a combination of MBR and various physicochemical waste-water treatment processes at the membrane separation stage, developed to the level of successful commercial operation that provides a good opportunity for practical fouling mitigation solutions.
This paper focuses on MBR membrane fouling characterization, prevention and mitigation in relation to and by means of membrane flux enhancement, highlighting the types of research on adsorption, coagulation, flocculation, bio-flocculation and oxidation mechanisms.
2. Membrane foulants and flux enhancers
Application of various additives for membrane flux enhancement in MBR is a response to the current understanding of fouling mechanisms that can be influenced by such additives. Therefore, it is reasonable to mention that currently, membrane fouling is classified based on several criteria: nature and phase of foulants, stage and nature of fouling process and the ability to recover membrane permeability after the fouling occurred (Table ).
Table 2. Types of membrane pore blocking in terms of Hermia’s pore-blocking models
Membrane pore clogging occurs because of suspended solids agglomeration and should be distinguished from membrane surface fouling, even though both lead to the same result—a decrease of membrane permeability. The deposition of foulants in the membrane pores and on their surface follows a number of blocking filtration mechanisms. Hermia’s pore-blocking models (Table ) are the fundamental unified analytical description of the blocking mechanisms formulated in the common frame of power-law non-Newtonian (further extended to Newtonian) fluids (Chellam & Cogan, Citation2011; F. Wang & Tarabara, Citation2008; Jelemenský, Sharma, Paulen, & Fikar, Citation2016; Bowen, Calvo, & Hernández, Citation1995).
Although clogging is the most frequent problem of MBR plants, it is not of the prime interest to the authors of the current review.
The main practical interest, from the point of view of membrane flux enhancement, is the investigation of the interactions of additives with biopolymers and biofilms that can be present as particles, colloids or solutes and incorporate or promote inorganic binding, precipitation, adhesion, etc. (Table ).
Table 3. Membrane fouling classifications in MBR
2.1. Biopolymers
According to Hu, Wang, Tian, Ngo, and Chen (Citation2016), Juang, Lee, and Lai (Citation2010), Kunacheva and Stuckey (Citation2014) and Zhang, Yu, Zhang, and Song (Citation2015), polysaccharides and proteins are the major components of biopolymers causing membrane fouling in low-pressure membrane filtration processes. Polysaccharides are the primary membrane foulants in MBRs (Chu & Li, Citation2005; Fonseca, Summers, & Greenberg, Citation2007; Jin et al., Citation2013; Lesjean et al., Citation2005; Meng et al., Citation2017; Satyawali & Balakrishnan, Citation2009; Sweity et al., Citation2011; Tarnacki, Lyko, Wintgens, Melin, & Natau, Citation2005; Tu, Zhang, Xu, Zhang, & Zhu, Citation2010; J. Zhang, Chua, Zhou, & Fane, Citation2006). This is primarily attributed to the gelling properties of polysaccharides that can be enhanced in the presence of multivalent cations acting as bridges for the carboxyl groups (Xin, Bligh, Kinsela, Wang, & David Waite, Citation2015). Owing to the reversibility of gels, several studies have reasonably referred to a polysaccharide-caused fouling as reversible (Merle, Dramas, Gutierrez, Garcia-Molina, & Croué, Citation2016). On the one hand, the formation of a gel layer on the membrane surface decreases membrane flux, but on the other hand, it serves as a substrate for bacteria attachment and further biofilm development.
It is generally well accepted that proteins are the key organic constituents that contribute to the foulant layer, interacting with polysaccharides (Neemann, Rosenberger, Jefferson, & McAdam, Citation2013; X.-M. Wang & Waite, Citation2009). The protein–polysaccharide interaction is non-covalent and can be classified by electrostatics, steric exclusion, hydrophobic interactions and hydrogen bonding. It is well known that pH and ionic strength influence the physical state of proteins and polysaccharides when present in the mixture. For pH conditions close to but still above the pH of isoelectric point, positively charged micro-regions on proteins can bridge over to discrete regions of the long-chain polysaccharide molecules, increasing the solute size and subsequently the probability of non-covalent network formation that imparts high fouling rates, thus explaining the apparent role of protein in the irreversible fouling fraction previously reported in the literature (McClements, Citation2006). It is also clear that proteins provide an easy food for bacteria in further biofilm development.
Humic substance is another massive organic component of MBR mixed liquor (Aryal et al., Citation2009). They are introduced into the sewer network presumably through rainwater and storm-water runoff, along with other constituents of domestic sewage, due to insufficient drinking water preparation (Bratby, Citation2016) and through cell decay (S. C. Wu & Lee, Citation2011). In addition, 40–50% of soluble microbial products (SMPs) were observed to be humic, fulvic and hymathomelanic acids (W. Chen, Westerhoff, Leenheer, & Booksh, Citation2003). In the study by Chuang, Chang, Chang, and Sung (Citation2009), humic substances in the effluent were singled out as those having significant fouling potential. Being small but strongly hydrophobic, molecules of humic substances adsorb to membranes and bind with polysaccharides and proteins via hydrophobic and electrostatic interactions (Z. Wang, Cao, & Meng, Citation2015), forming large molecular assemblies on the membrane surface and inside pores, facilitating further deposition of hydrophobic fragments (Kimura, Ogyu, Miyoshi, & Watanabe, Citation2015). Such complex interaction makes humic substances responsible for the development of irreversible fouling.
All together, polysaccharides, proteins and humic substances constitute the large group of foulants determined in the literature as SMPs (Figure ).
SMPs are thought to form biomolecular assemblies, adsorb onto the membrane surface, block membrane pores and/or form a gel structure on the membrane surface where they provide not only hydraulic resistance to permeate flow resulting in membrane flux decrease, but also a substrate for biofilm formation and possible nutrient source for biofilm development.
2.2. Biofilms and bio-cakes
In the MBR systems, biomolecular assemblies tend to attach to the membrane surface or to the gel layer of other biopolymers, accumulating at the solid–liquid interface or being suspended in the bulk solution in the form of biofilms. Biofilms represent a stable highly competitive agglomeration, which causes severe fouling problems (Saeki, Karkhanechi, Matsuura, & Matsuyama, Citation2016; Flemming & Wingender, Citation2010).
Biofilms develop bacteria cohesion and the further production of extracellular polymeric substances (EPSs) that are determined as autochthonous macromolecules found at or outside the cell surface and in the intercellular space of microbial aggregates (Flemming & Wingender, Citation2001). In further steps, biofilm maturation and bacterial detachment take place (Vanysacker, Boerjan, Declerck, & Vankelecom, Citation2014), escalating biofouling development.
A complex system of biofilm and EPSs forms a bio-cake on the membrane surface, causing a steep decrease of membrane flux. SMPs play an important role in the initial fouling, whereas EPSs produced by the deposited microbial cells become a major foulant after the TMP increase (Z. Zhou et al., Citation2015). Meanwhile, the presence of SMPs and colloids in the cake layer highly increases cake resistance.
One of the most complex subjects involved is the influence of activated sludge constituents and floc characteristics on sludge fouling propensity, as several parameters interact and influence sludge filterability simultaneously (Jørgensen et al., Citation2017). The size of activated sludge flocs affects the membrane biofouling rate at all stages of initial biofilm formation and bio-cake development. Small flocs have high specific contact interaction energy and easily adhere to the membrane surface (Shen et al., Citation2015). In the complex process of biofilm formation and development, the surface hydrophobicity of sludge flocs is another important factor affecting the membrane fouling rate through the promotion of bacteria adhesion (Gutman, Walker, Freger, & Herzberg, Citation2013). In general, for all major foulants, higher MLSS concentration increases the membrane fouling rates and flux declines due to a higher probability of biopolymerization, fostering of biofilm growth and particle aggregation.
As is often observed in real-life chemical and biological processes, in real MBR systems, all types of fouling discussed above take place continuously, changing from one form to another. In fact, each fouling process occurs temporarily and results not only in a membrane flux decline, but also in a release of products that initiate another fouling stage: dead cells release SMPs to the mixed liquor and SMPs form the depositions on the membrane surface and in the pores, feeding living microorganisms that produce further SMPs and EPSs. Because of the accumulation of dead cells and biopolymers in the bottom fouling layers, the system becomes clearly different from biofilms applied in waste-water treatment processes (Meng et al., Citation2017). The system of bio-cake is characterized by concentration gradients of oxygen and substrates from the surface to bottom layers, close to the membrane. In addition to the biological processes occurring in the bio-cake, hydrolysis and humification take place, converting polysaccharides and proteins into hardly degradable and heavily removable assemblies.
A number of studies have pointed out that the major foulants act according to the particular pore-blocking models, which helps distinguish the fouling nature and a suitable cleaning approach (Table ).
Table 4. Analysis of the characteristic pore-blocking patterns for the typical foulants
Concerning the fouling types, in terms of membrane permeability recovery, they can be categorized as reversible, irreversible and irrecoverable fouling (Table ). Reversible fouling is caused by deposition of the foulants on the membrane surface, leading to the formation of the cake layer. It can be removed by means of physical cleaning. However, irreversible and irrecoverable fouling are associated with internal pore blocking and pore constriction, caused by adsorption/deposition of the dissolved/colloidal matter inside the membrane pores and near their openings. Irreversible biofouling can be removed by a more aggressive cleaning approach—chemical cleaning: 1) CEB, applying NaOCl, NaOH, H2O2 and biocides (SBS) and 2) CIP or “cleaning in air” (CIA) via soaking the membranes in NaOCl and NaOH solutions. On the contrary, irrecoverable fouling, being the result of the gradual long-term accumulation of the foulants in membrane pores, is robust to all the cleaning strategies and cannot be removed by the existing means of cleaning (Geilvoet, Citation2010; Gkotsis, Mitrakas, Tolkou, & Zouboulis, Citation2017; Janus & Ulanicki, Citation2015; Judd, Citation2008; Wang et al., Citation2014).
Table 5. Characteristic fouling types for the blocking mechanisms and the best suitable cleaning methods
However, as admitted by Lee et al. (Citation2013), in some cases, the definition of each fouling type might not directly correspond to the fouling mechanisms defined in the filtration models.
2.3. Highlighted flux enhancers
Membrane flux enhancement is an emerging strategy, tested to a certain degree in the lab with promising first results, but with very limited experience from the field.
A range of chemicals have been evaluated as membrane flux enhancers in various studies (Table ). Their flux enhancement activity is strongly associated with the membrane fouling nature that they are able to affect. The most well-studied ones are adsorbents, coagulants and flocculants. Further studies have shown that membrane flux enhancers are additives that not only help with fouling but also solve other important tasks—a reduction of the carbon footprint of the MBR plant, improvement of related sludge properties, costs of sludge processing, etc.
Table 6. Membrane flux enhancement in MBR
This work reviews laboratory research and piloting experience with major flux enhancers, with specific regard to adsorption, coagulation, flocculation, bio-flocculation and oxidation mechanisms.
3. Mechanisms of membrane flux enhancement in MBR
Review of the recent studies shows five main points of focus in membrane fouling enhancement in terms of the mechanisms of action and types of applied chemicals: adsorption, coagulation, flocculation, bio-flocculation and oxidation.
3.1. Adsorption
According to Iorhemen, Hamza and Tay (Citation2016), the adsorbents in biological treatment systems adsorb dissolved organic matter, especially SMP and, consequently, mitigate membrane fouling. Different adsorbents can be used for this purpose, but the most studied ones are granular and powdered activated carbons.
Granular activated carbon (GAC) was successfully applied in several MBR fouling studies due to its well-predictable ability not only to adsorb organic constituents of mixed liquor but also to provide additional scouring on the membrane surface. Owing to the relatively low specific gravity, GAC is easy to fluidize and therefore it can be a perspective and energy-efficient flux enhancer (Kim et al., Citation2011). Being bifunctional, GAC mostly acts as a mechanical flux enhancer, rapidly increasing the membrane flux after dosing due to bio-cake detachment, but not affecting irreversible fouling at all (Wu et al., Citation2017). Various studies have reported 20–60% of flux enhancement with GAC (Johir, Aryal, Vigneswaran, Kandasamy, & Grasmick, Citation2011, Johir, Shanmuganathan, Vigneswaran, & Kandasamy, Citation2013).
At the same time, those studies took into account only a part of the GAC properties that can affect flux enhancement in MBR:
granule size strongly affects the mechanical scouring effectiveness of GAC (Johir, Citation2013; Wang et al., Citation2016);
a low mechanical strength of GAC granules negatively affects the overall flux enhancement due to membrane pore blocking with small particles; and
GAC porous type (related to carbon source and production technology) and adsorption activity were not studied at a proper level.
One of the most common additives is powdered activated carbon (PAC). When PAC is mixed with activated sludge, biologically activated carbon is formed, which improves the removal efficiency and mitigates membrane fouling (Aun Ng, Sun, & Fane, Citation2006; Seo, Suzuki, & Ohgaki, Citation1996). According to Kim and Lee (Citation2003), lower fouling propensity was detected in the MBR process when the PAC was directly inserted into the bulk solution and mixed with biomass.
A much lower fouling rate (TMP rise) for the MBR with PAC addition, compared to the system without this agent, was observed in the study by Aun Ng et al. (Citation2006). In the work by Ying and Ping (Citation2006), reduction of the EPS deposition on the membrane surface was noticed, which occurred after the addition of PAC to the sewage. On the contrary, at much higher PAC dosages, the effect of this additive was significantly weakened.
PAC is effective for treating highly toxic effluents, as shown by Lesage, Sperandio, and Cabassud (Citation2005). Additionally, according to Satyawali and Balakrishnan (Citation2009), it exhibited significant efficiency while treating high-strength waste water from the alcohol distillery, especially in terms of improving sludge de-waterability and affecting the SMP composition (protein/carbohydrate ratio). It is noteworthy that PAC dosing enhances system stability and promotes the maintenance of consistent permeate flux in terms of TOC removal, as shown by Guo, Vigneswaran, Ngo, Van Nguyen, and Ben Aim (Citation2006), Iversen, Koseoglu, et al. (Citation2009) and Munz, Gori, Mori, and Lubello (Citation2007).
Except for PAC, other adsorbents can be used to mitigate fouling in the MBR system. This was illustrated by J. C. Lee et al. (Citation2001), where natural zeolites in combination with alum were employed for such a purpose. Zeolite-added sludge possessed buffer capacity towards ammonium ion. Furthermore, the nitrification rate, as well as the organic removal efficiency, was also remarkably improved.
Nevertheless, weaknesses of applying the adsorbents, such as their high cost and the issue of exhausted material disposal, still remain the main limiting factors for their application as membrane flux enhancers in MBR.
3.2. Coagulation
One of the promising strategies to reduce fouling in MBR is to modify sludge filtration characteristics by the addition of coagulants. At the same time, practitioners should be careful with the use of some flux enhancers, such as ferric ions, which can potentially enhance the gelation of polysaccharides on the membranes.
While studying the effect of inorganic salts, such as ferric chloride, aluminium sulphate, polyaluminium chloride (PACl) and polymeric ferric sulphate (PFS), on fouling reduction in MBR, a significant improvement of filtration performance was observed. According to Wu et al. (Citation2006), PACl, PFS and their mixture had a better influence on mixed liquor filterability enhancement than monomeric additives. Polymeric coagulants exhibited a reduction of the initial TMP and TMP increase rates; in other words, membrane fouling was successfully alleviated, and among all of the additives, PFS was the most effective. Several further studies (Ji et al., Citation2010; Ji, Qiu, Wong, & Li, Citation2008; J. Wu & Huang, Citation2008) also demonstrated the high effectiveness of PFS use as a fouling mitigation agent. In the work by Wu and Huang (Citation2008), PFS was the focus of interest as a fouling retardation agent during long-term runs of MBR. According to the findings, PFS removed organic compounds of high molecular weight from supernatants via coagulation, entailing the mitigation of gel-layer formation on the membrane surface and suspended flocs enlarging by supplying positive charges to organic particles and enhancing charge neutralization. The positive influence of PACl on filtration performance was indicated by Guo et al. (Citation2010), Koseoglu, Yigit, Civelekoglu, Harman, and Kitis (Citation2012) and Nouri et al. (Citation2013).
However, according to other studies, monomeric metal salts also exhibited high efficiency in sewage treatment. Short-term experiments conducted by Guo et al. (Citation2010) revealed that FeCl3 along with PACl considerably reduced SMP concentration in the effluent stream, improved sludge settleability together with oxygen transfer and gained superior efficiency in terms of fouling control compared to their organic analogues, like starch. As specified by Song et al. (Citation2008), FeCl3 was efficient in the reduction of specific resistance, whereas the addition of alum, which was also examined, assured high phosphorus removal and the decrease in membrane filtration resistance without any deterioration in the nitrogen removal efficiency. Enhancement of the filterability in MBR applying either FeCl3 or aluminium sulphate was also discussed by Huyskens et al. (Citation2012), Iversen, Koseoglu, et al. (Citation2009), Koseoglu et al. (Citation2008), Ji et al. (Citation2008) and Nouri et al. (Citation2013).
Eventually, based on all the aforementioned studies and according to the recent findings, prime foulants such as effluent organic matter, whose elimination via coagulation and flocculation is of high priority, generally comprise two main forms: proteins in conjunction with polysaccharides and humic substances (Fang, Shi, & Zhang, Citation2006; Flemming & Wingender, Citation2001; V. Iversen, Mehrez, et al., Citation2009; Miyoshi, Tsuyuhara, Ogyu, Kimura, & Watanabe, Citation2009).
According to Geng and Hall (Citation2007), bound EPSs in activated sludge flocs are not directly associated with membrane fouling; however, EPSs are responsible for the release of SMPs into the effluent stream, and, as demonstrated before, SMPs in the mixed liquor cause the deterioration of filtration performance. This necessitates the defying of the mechanism by which chemical additives remove SMPs from the water. In the study by Bratby (Citation2016), the authors elucidated the origin of the surface charge of bacteria, which, as assumed, arises from chemical reactions at the surface. Many bacterial solid surfaces contain functional groups that are readily ionizable, such as –OH, –COOH and –OPO3H2 (Bratby, Citation2016):
Thus, the charge of such particles becomes dependent on the degree of ionization (proton transfer) and, hence, on the pH of the surrounding liquid. At low pH values, a positively charged surface prevails, whereas under alkaline conditions, the surface becomes mainly negatively charged.
Ipso facto, since typically investigations on the performance of filter aids are conducted at higher pH values, the surfaces of SMP carry net negative charges due to the broken edges of the hydroxyl groups (Ibeid, Elektorowicz, & Oleszkiewicz, Citation2017).
When Al/Fe-based coagulants are added to the water, the salts dissociate and metal ions react with water molecules, by breaking the bonds of the lowest energy between the protons and the hydroxyl groups and, subsequently, interacting with the latter ones. As a result, metal hydrolysis occurs (Gregory & Duan, Citation2001; Hem & Roberson, Citation1967), followed by the formation of colloidal particles, the so-called “micelles”, depicted below.
Positively charged granules of the micelles attract negatively charged SMPs by means of electrostatic forces, van der Waals attraction and, presumably, electric or magnetic forces, resulting in the formation of organic/inorganic solid complexes and the ruining of aggregative stability of the disperse system (Figure ).
Figure 2. The formation of organic/inorganic solid complexes with the aid of a) ferric (III) and b) aluminium coagulants.
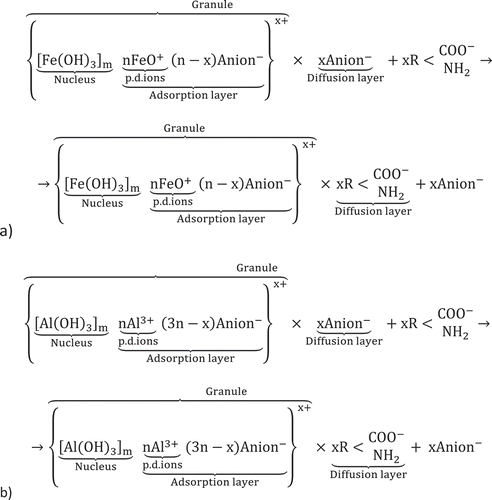
In addition, the newly formed particulate matter of the metal hydroxides possesses very high adsorption ability, thus accumulating SMP on its surface. Consequently, the aggregated flocs agglomerate with each other via adhesion forces, increase in size, being held together by weak physical interactions, and, finally, precipitate (Inan, Dimoglo, Şimşek, & Karpuzcu, Citation2004).
Concerning humic substances, as stated by Bolto (Citation1995), at pH 5–6, various aluminium humates may be formed through the polynuclear aluminium species, produced during the following stoichiometric reaction:
The more explicit mechanism for aluminium salt coagulation of humic substances is depicted in Figure .
Figure 3. Pathways for the coagulation of humic substances by aluminium ions at higher pH levels (Bolto, Citation1995; Bratby, Citation2016).
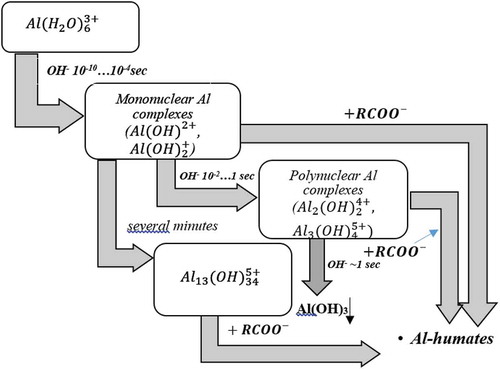
These and higher pH levels foster the adsorption of organic materials onto the flocs of aluminium hydroxide, occurring by the ligand-exchange reaction (Bolto, Citation1995):
Almost identical mechanisms of hydrolysis with the subsequent coagulation are involved when applying ferric salts (Gregory & Duan, Citation2001; Ødegaard, Fettig, & Ratnaweera, Citation1990).
The interaction of ferric salts with such a form of humic substances as fulvic acids has been described by Van Breemen, Nieuwstad, and Van Der Meent-Olieman (Citation1979), where the interaction between iron ions and organic groups depends on the content of fulvic acids. As assumed, in diluted solutions, the complex-like ions are formed, due to the combination of one Fe3+ ion with the COO− group. Such complexes may attract each other, resulting in the formation of polymeric iron hydroxides. The excess of ferric (III) coagulant promotes the yielding of hydroxide flocs and a subsequent co-precipitation of colloidal particles with their aid. Moreover, in the case of a high concentration of fulvic acids, they precipitate in the form of iron fulvinate, as three Fe3+ ions combine with the corresponding amount of COO− groups.
It is suggested that further investigations should be conducted to gain a better insight into the key mechanism controlling the removal of the high MW biopolymer molecules, with a view to further optimizing the coagulation process.
3.3. Flocculation
Another commonly used flocculant is homopolymer 2-propen-1-aminium, N,N-dimethyl-N-2-propenyl-chloride (PolyDADMAC or MPE50-the product of Nalco®), whose high effectiveness as a membrane fouling reducer was proven by various research teams (Huyskens et al., Citation2012; V. Iversen, Mehrez, et al., Citation2009; Jamal Khan, Visvanathan, & Jegatheesan, Citation2012; Koseoglu et al., Citation2008). In the study by Hwang, Lee, Park, Lee, and Chang (Citation2007), attempts to elucidate the mechanism of such significant flux enhancement through analysing the images acquired under a confocal laser scanning microscope (CLSM) were made. According to the attained results, the inhibited fouling rate could be attributed to an increase in cake porosity and uniform distributions of the boundary-layer components along its depth after adding PolyDADMAC to the bulk phase. The positive influence of this additive on MBR filtration performance was also confirmed by W. S. Guo, Vigneswaran, Ngo, Kandasamy, and Yoon (Citation2008). High levels of dissolved organic carbon (DOC) and chemical oxygen demand (COD) removal efficiency (over 95%) were attained with the aid of this chemical when running over a 7-day period. Besides NH4-N elimination being over 95–98%, PO4-P removal increased with time to over 99% after 7 days of operation. However, in terms of phosphorus elimination, the MBR with PolyDADMAC exhibited worse results than the sponge system. PolyDADMAC also enhanced the microbial activity of the biomass in the MBR. In the study by Yoon and Collins (Citation2006), the extent of short-term and long-term flux improvements by PolyDADMAC was tested at low temperatures (10–13°C) in order to overcome low permeate production under such conditions and, consequently, to reduce capital costs. As a result, at 10°C, the long-term flux increased from 17 LMH to 25 LMH with the extension of filtration ability from 22 days to more than 30 days. Additionally, a small municipal MBR plant succeeded in increasing the daily flux by 150% via dosing PolyDADMAC during the long-term run.
In the work by Nguyen et al. (Citation2010), the combination of PolyDADMAC and FeCl3 was applied with the evaluation of its impact on the treatment performance in a long-term submerged MBR. According to the results, this combined flocculant demonstrated that almost 100% of the total phosphates and DOC removal and 90% of the ammonia (NH4-N) compounds were eliminated during the 80-day operation. In addition, a good microbial activity with a stable specific oxygen uptake rate (SOUR), stable values of SVI and soluble carbohydrate concentration and a low rate of TMP rise were observed.
In the study by Ji et al. (Citation2010), among the various compounds, polyacrylamide (PAM) was investigated, and, as noticed, it significantly alleviated membrane fouling by affecting the zeta potential and viscosity. Additionally, according to the results obtained in the work by Ji et al. (Citation2014), where the fouling mitigation ability of PAM in combination with other modified starches was examined, the composites exhibited a strong positive effect on SMP, the fractal dimension of sludge flocs and floc size in sustainable filtration.
Meanwhile, it is quite difficult to figure out the exact mechanism for system destabilization, governed by the polyelectrolyte additives. The type of predominant phenomenon depends on whether the polymer is used as a primary coagulant or as a flocculant aid and the polymer characteristics (charge density and molecular weight). Three main pathways of their action have been distinguished (Bolto, Citation1995; Bratby, Citation2016; Ji et al., Citation2008): charge neutralization, bridging and the electrostatic charge patch mechanism.
According to McEwen (Citation1998) and Amjad (Citation2010), the charge neutralization model deals with the use of relatively low MW cationic polymers with a high charge density, such as PolyDADMAC, which causes the destabilization of negatively charged colloids through charge neutralization, accomplished by the adsorption of the polymer on the particle surface. The cationic nature of PolyDADMAC and PAM is caused by the presence of quaternary ammonium functional groups in the polymer skeleton. Relevant interactions are shown in Figures and .
Figure 5. The elimination of bacterium with the aid of cationic PAM via the charge neutralization mechanism.
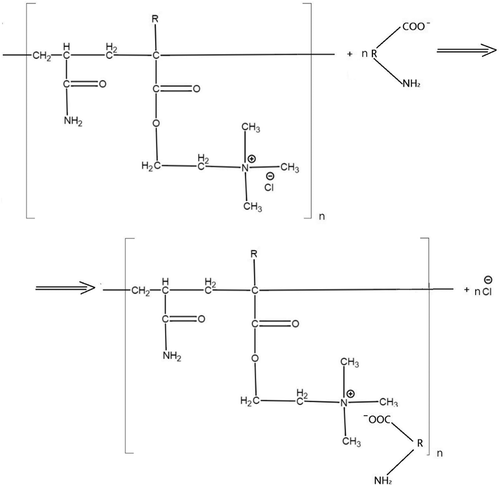
The superior role of the neutralization mechanism in flocculation employing PolyDADMAC was discussed in the studies by Hahn, Hoffmann, and Ødegaard (Citation1996) and Zahrim and Dexter (Citation2016). Meanwhile, as discussed in the latter work, flocculation occurs through the bridging mechanism as well.
The importance of the charge neutralization phenomenon, while applying PAM-based cationic polyelectrolytes for sludge conditioning, was noticed by Tiravanti, Lore, and Sonnante (Citation1985). However, this mechanism is not the only one governing the flocculation of negatively charged organic matter since cationic polyelectrolytes possessing high charge densities also act through the electrostatic charge patch mechanism (Bratby, Citation2016; Tiravanti et al., Citation1985). It is based on the complete adsorption of polyelectrolyte segments onto the surface of the impurity, followed by the generation of charge mosaics with positively and negatively charged regions and the final destabilization of the system, resulting from the alignment of the charge mosaics of adjacent particles, which provides strong electrostatic attraction (Figure ) (Bolto, Citation1995; Bratby, Citation2016).
Figure 6. Possible arrangement of the cations on the surface of the suspended solid and the charge patch agglomeration mechanism (Bolto, Citation1995).
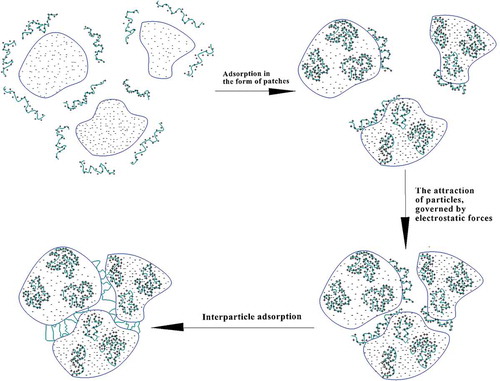
Thus, this mechanism is assumed to be predominant when applying PolyDADMAC (high MW) and low MW cationic PAM of high charge densities at relatively low particle concentrations (less than 1014 particles/L).
When discussing the flocculation mechanism of cationic polymers at high particle concentration, significant bridging effects are detected in the interim between the initial adsorption stages and the reaching of the equilibrium state, since higher particle concentrations intensify the collision frequency of suspended solids at the same time as the pendant polyelectrolyte loops are greatly extended into the solution (Bratby, Citation2016). Hence, presumably, the bridging mechanism plays an essential role in the case of applying PolyDADMAC and high MW cationic PAM of low charge density at high concentrations of suspended solids in the mixed liquor.
3.4. Bio-flocculation
Modified starches solely (in the form of starch-based cationic flocculants) were investigated by Deng et al. (Citation2015), W. Guo et al. (Citation2010), Huyskens et al. (Citation2012) and Koseoglu et al. (Citation2008, Citation2012), since they are inexpensive, offering inherent advantages over inorganic and synthetic polymers such as being derived from a renewable source of raw materials with simpler degradability after the use. However, the controversial effect of starches on membrane performance was discussed in the study by Drews (Citation2010). Presumably, this is caused by the fact that biopolymers like starch and chitosan do not tolerate wide dosing ranges, since over- or under-dosing might cause further fouling on the membrane (Vera Iversen, Citation2011). On this account, in the study by Koseoglu et al. (Citation2008), starch along with chitosan was reported to trigger the flocculation mechanism apparently better than the metal salts, whereas, according to Huyskens et al. (Citation2012), both polymers caused a considerable increase in irreversible fouling observed in the MBR. In a number of studies (Iversen, Mehrez, et al., Citation2009; Iversen, Mohaupt, Drews, Lesjean, & Kraume, Citation2008; Koseoglu et al., Citation2012), starch also had a detrimental effect on the system due to strong fouling phenomena. Additionally, in the work by Iversen, Koseoglu, et al. (Citation2009), a negative impact of starch-based flocculant on the oxygen uptake rate (OUR) was indicated, stressing that this polymer might therefore not be appropriate for application in MBR.
Concerning chitosan, Ji et al. (Citation2008) indicated the significant positive influence of this additive on the flocculation of sludge particles with the subsequent reduction of the fouling rates for both short- and long-term operations, which were about seven times lower than in the control MBR. In further research (Ji et al., Citation2010), chitosan was revealed to have a crucial effect on biomass morphological properties and successfully alleviated membrane fouling. Fouling mitigation ability of this agent was noticed by Shuyan (Citation2015) as well. Additionally, in the study by Iversen et al. (Citation2008), chitosan considerably reduced SMP levels in the supernatant. Furthermore, enhanced OURs were observed when using chitosan, according to Iversen et al. (Citation2008), Nouri et al. (Citation2013).
In marked contrast to all these findings, according to Guo et al. (Citation2010) and Koseoglu et al. (Citation2012), chitosan exhibited either the highest fouling rates among the tested additives or the lowest SMP removal because of the significant sludge viscosity increase.
In the study by Ngo and Guo (Citation2009), a modified green bio-flocculant (GBF) was introduced, which served as a membrane fouling reducer and an agent for improving microbial activity. The positive impact of this additive on treatment performance and fouling mitigation was also discussed in the work by Guo, Ngo, Wu, Hu, and Listowski (Citation2011).
Regarding effective natural bio-flocculants, particularly chitosan, its structure highly depends on pH (Figure ).
Figure 7. Structure of chitosan depending on pH conditions (Kumirska, Weinhold, Thöming, & Stepnowski, Citation2011; Sakkayawong, Thiravetyan, & Nakbanpote, Citation2005).
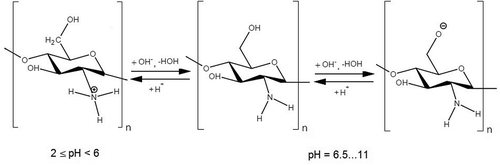
The mechanism for the removal of microbial substances using chitosan at acidic pH is as follows:
where is chitosan and
is the ionized carboxyl group of the bacterial surface;
—complex.
According to the findings, at acidic pH by its protonated amino groups, chitosan interacts electrostatically with negatively charged organic matter, resulting in their chemisorption. Therefore, in acidic solutions, chitosan exhibits strong antibacterial activity, as shown in the study by Kumirska et al. (Citation2011). Nevertheless, according to Guibal and Roussy (Citation2007), sometimes the amount of protonated amino groups is insignificant, far below the number of charges required for neutralization of the anions carried by suspended solids. In such cases, as assumed, the removal is assured by the combination of electrostatic patch and bridging mechanisms. At higher pH values between 6.0 and 6.5, amino groups of chitosan become less protonated and turn out to be fully deprotonated at pH 6.5. However, as shown in the work by Sakkayawong et al. (Citation2005), even in this case chitosan doesn’t lose its treatment efficiency, which is presumably entailed by the hydrophobic interactions of chitosan molecules with sludge aggregates of the same nature. Besides, since chitosan possesses a high molecular weight, the elimination of organic matter is expected to happen through the bridging mechanism. In the alkaline media, chitosan can adsorb organic contaminants through covalent bonding.
Starch grafts cationic moieties during carboxylation with the aid of the quaternary ammonium compound. According to Ji et al. (Citation2014), cationic starches act through the charge neutralization mechanism since they get attracted to the surface of negatively charged organic particles and result in the decrease of the absolute electrokinetic potential value, resulting in system destabilization and subsequent agglomeration of the particles. The starches are characterized by the ability to generate larger flocs, although their binding to colloid particles is incomplete (Koseoglu et al., Citation2012). On the contrary, according to Pal, Mal, and Singh (Citation2005), the main mechanism of action for cationic starches is the bridging phenomenon; moreover, longer chains of the polymer backbone are preferable since they foster the extension of the tail from one particle surface to another, thus assuring high flocculation efficiency.
3.5. Oxidation
Ozonation can be applied as an alternative method to modify the mixed liquor properties in order to alleviate membrane fouling. The positive effect of ozone on long-term MBR performance was confirmed by X. Huang and Wu (Citation2008) and J. Wu and Huang (Citation2010), who determined the optimum ozone concentration, which could assure slow formation of the gel layer on the membrane surface as well as the enhancement of suspended particles’ flocculation ability in the ozone–MBR system.
According to the investigation carried out by K. R. Lee and Yeom (Citation2007), ozone played a crucial role in membrane fouling control since it promoted keeping the dynamic viscosity of the activated sludge at a relatively low level, thus permitting stable membrane filtration for more than 150 days without chemical cleaning of the membrane. In the study by Yeom et al. (Citation2005), ozone was applied in combination with alkaline for biomass pretreatment in a pilot-scale MBR-type aerobic digestion process. As discussed, the experiment ran smoothly without significant membrane fouling, even at the relatively high levels of MLSS concentration (11,000–25,000 mg/L).
4. Research needs
The current literature review provides background and identifies the most prospective additives as membrane fouling enhancers in MBR (Table ). However, the use of additives is not usually practised in full-scale MBRs since it is uncertain whether the cost of chemical use is justified by membrane fouling decrease (Krzeminski, Leverette, Malamis, & Katsou, Citation2017). Furthermore, the long-term implications of using or stopping to use the additives have not been researched in detail, and there is a gap in process control approaches for the application of flux enhancers in MBR.
Table 7. Intrinsic mechanisms of chemical action in the reduction of a system’s fouling propensity under membrane flux enhancement in MBR
Since adsorption was proven to be an efficient fouling alleviation tool, it is worth mentioning that its application in the MBR process still has a high potential. However, in order to make it more feasible, cost-effective adsorbents should be used. A number of cheap non-conventional materials can serve this purpose. For example, nano-magnetic polymers, cyclodextrin and covalent organic polymers were found to have great binding/degrading capacities for organic compounds (Alaba et al., Citation2018). In addition, agricultural and industrial waste materials like wood char, rice husk, fruit peels, fly/zeolite incinerator ash and polymer resins are gaining more interest as potential adsorbents (Mallampati, Xuanjun, Adin, & Valiyaveettil, Citation2015; Mateen et al., Citation2016; Rene & Lewis, Citation2017). However, these materials need to be thoroughly tested for their effectiveness to mitigate fouling in the MBR system.
Concerning ozonation, additional trials are needed to make its application more economical when dealing with the mixed liquor, characterized by high levels of suspended solids. Moreover, the possibility of the generation of highly toxic non-biodegradable/inseparable ozonation by-products should be checked.
5. Conclusions
In conclusion, membrane flux enhancement applying coagulants, flocculants and bio-flocculants is a prospective direction of membrane fouling mitigation in MBR that has the potential to facilitate further penetration of MBR into cost-sensitive markets, including small communities and developing countries.
Further lab research in this direction should be focused on combining various agents as membrane flux enhancers, considering the identified mechanisms of their action: retention of SMP, reduction of specific cake resistance, an increase of sludge floc size and hydrophobicity as well as the neutralization of their charge. The possibility of applying them all together with non-conventional cost-effective adsorbents or oxidizing agents should also be taken into consideration.
In general, applied future research should be focused on developing pilot case studies with various membrane flux enhancers as well as bridging the gap in MBR process control, including the flux enhancement part.
Additional information
Funding
Notes on contributors
O. Kulesha
The authors belong to the Water, Environment, Sanitation and Health (WESH) research group at the Norwegian University of Life Sciences, Faculty of Science and Technology. The group focuses on new engineering solutions and concepts in waste-water treatment, combining membrane separation processes and chemical treatment methods. This paper opens a series of publications related to the research on chemical enhancement and fouling prevention in membrane biological reactors.
Notes
1. PFS, polymeric ferric sulphate.
2. PAC, powdered activated carbon.
References
- Ábel, M. , Szabó, G. , Poser, O. , László, Z. , & Hodúr, C. (2013). Enzyme recovery and fouling mitigation by ultrasound-enhanced ultrafiltration. Desalination and Water Treatment , 51(25–27), 4921–4926. doi:10.1080/19443994.2013.795325
- Alaba, P. A. , Oladoja, N. A. , Sani, Y. M. , Ayodele, O. B. , Mohammed, I. Y. , Olupinla, S. F. , & Daud, W. M. W. (2018). Insight into wastewater decontamination using polymeric adsorbents. Journal of Environmental Chemical Engineering , 6(2), 1651–1672. doi:10.1016/j.jece.2018.02.019
- Amiraftabi, M. S. , Mostoufi, N. , Hosseinzadeh, M. , & Mehrnia, M. R. (2014). Reduction of membrane fouling by innovative method (injection of air jet). Journal of Environmental Health Science and Engineering , 12(1), 1–8. doi:10.1186/s40201-014-0128-0
- Amjad, Z. (2010). The science and technology of industrial water treatment . London, New York: IWA Publishing.
- Aryal, R. K. , Lebegue, J. , Shon, H. K. , Vigneswaran, S. , Kandasamy, J. , & Grasmick, A. (2009). Temporal variation of foulant characteristics in membrane bioreactor. Desalination and Water Treatment , 6(1–3), 69–73. doi:10.5004/dwt.2009.647
- Aslam, M. , Lee, P. H. , & Kim, J. (2015). Analysis of membrane fouling with porous membrane filters by microbial suspensions for autotrophic nitrogen transformations. Separation and Purification Technology , 146, 284–293. doi:10.1016/j.seppur.2015.03.042
- Aun Ng, C. , Sun, D. , & Fane, A. G. (2006). Operation of membrane bioreactor with powdered activated carbon addition. Separation Science and Technology , 41(7), 1447–1466. doi:10.1080/01496390600634632
- Berg, C. H. , Kalfas, S. , Malmsten, M. , & Arnebrant, T. (2001). Proteolytic degradation of oral biofilms in vitro and in vivo: Potential of proteases originating from Euphausia superba for plaque control. European Journal of Oral Sciences , 109(5), 316–324. doi:10.1034/j.1600-0722.2001.00099.x
- Böhm, L. , Drews, A. , Prieske, H. , Bérubé, P. R. , & Kraume, M. (2012). The importance of fluid dynamics for MBR fouling mitigation. Bioresource Technology , 122, 50–61. doi:10.1016/j.biortech.2012.05.069
- Bolto, B. A. (1995). Soluble polymers in water purification. Progress in Polymer Science , 20(6), 987–1041. doi:10.1016/0079-6700(95)00010-D
- Bowen, W. R. , Calvo, J. I. , & Hernández, A. (1995). Steps of membrane blocking in flux decline during protein microfiltration. Journal of Membrane Science , 101(1–2), 153–165. doi:10.1016/0376-7388(94)00295-A
- Bratby, J. (2016). Coagulation and flocculation in water and wastewater treatment (3d ed.). London: IWA Publishing. doi:10.2166/9781780407500
- Brookes, A. , Jefferson, B. , Guglielmi, G. , & Judd, S. J. (2006). Sustainable flux fouling in a membrane bioreactor: Impact of flux and MLSS. Separation Science and Technology , 41(7), 1279–1291. doi:10.1080/01496390600634509
- Cao, T. A. , Van De Staey, G. , & Smets, I. Y. (2015). Integrating activated sludge floc size information in MBR fouling modeling. Water Science and Technology , 71(7), 1073–1080. doi:10.2166/wst.2015.070
- Chang, I. S. , & Judd, S. J. (2002). Air sparging of a submerged MBR for municipal wastewater treatment. Process Biochemistry , 37(8), 915–920. doi:10.1016/S0032-9592(01)00291-6
- Chellam, S. , & Cogan, N. G. (2011). Colloidal and bacterial fouling during constant flux microfiltration: Comparison of classical blocking laws with a unified model combining pore blocking and EPS secretion. Journal of Membrane Science , 382(1–2), 148–157. doi:10.1016/j.memsci.2011.08.001
- Chen, F. , Bi, X. , & Ng, H. Y. (2016). Effects of bio-carriers on membrane fouling mitigation in moving bed membrane bioreactor. Journal of Membrane Science . 499, 134–142. Retrieved from http://www.sciencedirect.com/science/article/pii/S0376738815302829
- Chen, J. , Zhang, M. , Li, F. , Qian, L. , Lin, H. , Yang, L. , … Liao, B. Q. (2016). Membrane fouling in a membrane bioreactor: High filtration resistance of gel layer and its underlying mechanism. Water Research , 102, 82–89. doi:10.1016/j.watres.2016.06.028
- Chen, W. , Westerhoff, P. , Leenheer, J. A. , & Booksh, K. (2003). Fluorescence excitation-emission matrix regional integration to quantify spectra for dissolved organic matter. Environmental Science and Technology , 37(24), 5701–5710. doi:10.1021/es034354c
- Christensen, M. L. , Bugge, T. V. , Hede, B. H. , Nierychlo, M. , Larsen, P. , & Jørgensen, M. K. (2016). Effects of relaxation time on fouling propensity in membrane bioreactors. Journal of Membrane Science . doi:10.1016/j.memsci.2016.01.006
- Chu, H. P. , & Li, X. Y. (2005). Membrane fouling in a membrane bioreactor (MBR): Sludge cake formation and fouling characteristics. Biotechnology and Bioengineering , 90(3), 323–331. doi:10.1002/bit.20409
- Chuang, S. H. , Chang, W. C. , Chang, M. C. , & Sung, M. A. (2009). The effects of soluble organic matters on membrane fouling index. Bioresource Technology , 100(5), 1875–1877. doi:10.1016/j.biortech.2008.09.054
- Collins, J. H. , Yoon, S. H. , Musale, D. , Kong, J. F. , Koppes, J. , Sundararajan, S. , … Kronoveter, K. (2006). Membrane performance enhancer evaluations on pilot- and full-scale membrane bioreactors. Water and Environment Journal , 20(1), 43–47. doi:10.1111/j.1747-6593.2006.00030.x
- Dalmau, M. , Atanasova, N. , Gabarrón, S. , Rodriguez-Roda, I. , & Comas, J. (2015). Comparison of a deterministic and a data driven model to describe MBR fouling. Chemical Engineering Journal , 260, 300–308. doi:10.1016/j.cej.2014.09.003
- Deng, L. , Guo, W. , Hao, H. , Farzana, M. , Zuthi, R. , Zhang, J. , … Zhang, X. (2015). Membrane fouling reduction and improvement of sludge characteristics by bioflocculant addition in submerged membrane bioreactor. Separation and Purification Technology , 156, 450–458. doi:10.1016/j.seppur.2015.10.034
- Deowan, S. A. , Galiano, F. , Hoinkis, J. , Johnson, D. , Altinkaya, S. A. , Gabriele, B. , … Figoli, A. (2016). Novel low-fouling membrane bioreactor (MBR) for industrial wastewater treatment. Journal of Membrane Science , 510, 524–532. doi:10.1016/j.memsci.2016.03.002
- Dizge, N. , Koseoglu-Imer, D. Y. , Karagunduz, A. , & Keskinler, B. (2011). Effects of cationic polyelectrolyte on filterability and fouling reduction of submerged membrane bioreactor (MBR). Journal of Membrane Science , 377(1–2), 175–181. doi:10.1016/j.memsci.2011.04.048
- Drews, A. (2010). Membrane fouling in membrane bioreactors-Characterisation, contradictions, cause and cures. Journal of Membrane Science , 363(1–2), 1–28. doi:10.1016/j.memsci.2010.06.046
- El Rayess, Y. , Albasi, C. , Bacchin, P. , Taillandier, P. , Mietton-Peuchot, M. , & Devatine, A. (2012). Analysis of membrane fouling during cross-flow microfiltration of wine. Innovative Food Science & Emerging Technologies , 16, 398–408. doi:10.1016/j.ifset.2012.09.002
- Ergön-Can, T. , Köse-Mutlu, B. , Koyuncu, İ. , & Lee, C. H. (2017). Biofouling control based on bacterial quorum quenching with a new application: Rotary microbial carrier frame. Journal of Membrane Science , 525(December 2015), 116–124. doi:10.1016/j.memsci.2016.10.036
- Etemadi, H. , Yegani, R. , & Seyfollahi, M. (2017). The effect of amino functionalized and polyethylene glycol grafted nanodiamond on anti-biofouling properties of cellulose acetate membrane in membrane bioreactor systems. Separation and Purification Technology , 177, 350–362. doi:10.1016/j.seppur.2017.01.013
- Evenblij, H. , Verrecht, B. , Van Der Graaf, J. H. J. M. , & Van Der Bruggen, B. (2005). Manipulating filterability of MBR activated sludge by pulsed substrate addition. Desalination , 178(1–3 SPEC. ISS.), 193–201. doi:10.1016/j.desal.2005.02.006
- Fang, H. H. P. , Shi, X. , & Zhang, T. (2006). Effect of activated carbon on fouling of activated sludge filtration. Desalination , 189(1–3 SPEC. ISS.), 193–199. doi:10.1016/j.desal.2005.02.087
- Field, R. W. , Wu, D. , Howell, J. A. , & Gupta, B. B. (1995). Critical flux concept for microfiltration fouling. Journal of Membrane Science , 100(3), 259–272. doi:10.1016/0376-7388(94)00265-Z
- Flemming, H. , & Wingender, J . (2010). Nature reviews, The Biofilm Matrix , 8(9), 623–33. doi:10.1038/nrmicro2415
- Flemming, H.-C.-C. , & Wingender, J. (2001). Relevance of microbial extracellular polymeric substances (EPSs) – Part I: Structural and ecological aspects. Water Science and Technology : A Journal of the International Association on Water Pollution Research , 43(6), 9–16. Retrieved from http://www.ncbi.nlm.nih.gov/pubmed/11381977%5Cnhttp://www.ncbi.nlm.nih.gov/pubmed/11381954
- Fonseca, A. C. , Summers, R. S. , & Greenberg, A. R. (2007). Extra-cellular polysaccharides, sol- uble microbial products, and natural organic matter impact on nanofiltration membranes flux decline. Environmental Science & Technology , 41(7), 2491–2497. doi:10.1021/es060792i
- Frechen, F. B. , Schier, W. , & Linden, C. (2008). Pre-treatment of municipal MBR applications. Desalination , 231(1–3), 108–114. doi:10.1016/j.desal.2007.10.025
- Gabarrón, S. , Gómez, M. , Dvořák, L. , Růžičková, I. , Rodriguez-Roda, I. , & Comas, J. (2014). Ragging in MBR: Effects of operational conditions, chemical cleaning, and pre-treatment improvements. Separation Science and Technology (Philadelphia) , 49(14), 2115–2123. doi:10.1080/01496395.2014.927489
- Geilvoet . (2010). The Delft Filtration Characterisation method. Assessing membrane bioreactor activated sludge filterability (Doctoral Dissertation). Delft University of Technology, The Netherlands. Retrieved from: uuid:5fbf0f3b-146b-4a67-9239-333482ad62bd.
- Geng, Z. , & Hall, E. R. (2007). A comparative study of fouling-related properties of sludge from conventional and membrane enhanced biological phosphorus removal processes. Water Research , 41(19), 4329–4338. doi:10.1016/j.watres.2007.07.007
- Gkotsis, P. , Banti, D. , Peleka, E. , Zouboulis, A. , & Samaras, P. (2014). Fouling issues in Membrane Bioreactors (MBRs) for wastewater treatment: Major mechanisms, prevention and control strategies. Processes , 2(4), 795–866. doi:10.3390/pr2040795
- Gkotsis, P. , Peleka, E. , Zamboulis, D. , Mitrakas, M. , Tolkou, A. , & Zouboulis, A. (2016). Wastewater treatment in membrane bioreactors: The use of polyelectrolytes to control membrane fouling. Environmental Processes . doi:10.1007/s40710-016-0168-9
- Gkotsis, P. K. , Mitrakas, M. M. , Tolkou, A. K. , & Zouboulis, A. I. (2016). Batch and continuous dosing of conventional and composite coagulation agents for fouling control in a pilot-scale MBR. Chemical Engineering Journal , 311, 255–264. doi:10.1016/j.cej.2016.11.099
- Gkotsis, P. K. , Mitrakas, M. M. , Tolkou, A. K. , & Zouboulis, A. I. (2017). Batch and continuous dosing of conventional and composite coagulation agents for fouling control in a pilot-scale MBR. Chemical Engineering Journal , 311, 255–264. doi:10.1016/j.cej.2016.11.099
- González, E. , Díaz, O. , Vera, L. , Rodríguez-Gómez, L. E. , & Rodríguez-Sevilla, J. (2018). Feedback control system for filtration optimisation based on a simple fouling model dynamically applied to membrane bioreactors. Journal of Membrane Science , 552(December 2017), 243–252. doi:10.1016/j.memsci.2018.02.007
- Gregory, J. , & Duan, J. (2001). Hydrolyzing metal salts as coagulants. Pure and Applied Chemistry , 73(12), 2017–2026. doi:10.1351/pac200173122017
- Guibal, E. , & Roussy, J. (2007). Coagulation and flocculation of dye-containing solutions using a biopolymer (Chitosan). Reactive and Functional Polymers , 67(1), 33–42. doi:10.1016/j.reactfunctpolym.2006.08.008
- Guo, W. , Ngo, H. , Wu, Z. , Hu, A. Y. , & Listowski, A. (2011). Application of bioflocculant and nonwoven supporting media for better biological nutrient removal and fouling control in a submerged MBR. Sustainable Environment Research , 21(1), 53–58.
- Guo, W. , Ngo, H. H. , Vigneswaran, S. , Dharmawan, F. , Nguyen, T. T. , & Aryal, R. (2010). Effect of different flocculants on short-term performance of submerged membrane bioreactor. Separation and Purification Technology , 70(3), 274–279. doi:10.1016/j.seppur.2009.10.003
- Guo, W. S. , Vigneswaran, S. , Ngo, H. H. , Kandasamy, J. , & Yoon, S. (2008). The role of a membrane performance enhancer in a membrane bioreactor : A comparison with other submerged membrane hybrid systems. Desalination, 231, 305–313. doi:10.1016/j.desal.2007.10.034
- Guo, W. S. , Vigneswaran, S. , Ngo, H. H. , Van Nguyen, T. B. , & Ben Aim, R. (2006). Influence of bioreaction on a long-term operation of a submerged membrane adsorption hybrid system. Desalination , 191(1–3), 92–99. doi:10.1016/j.desal.2005.06.034
- Gutman, J. , Walker, S. L. , Freger, V. , & Herzberg, M. (2013). Bacterial attachment and viscoelasticity: Physicochemical and motility effects analyzed using quartz crystal microbalance with dissipation (QCM-D). Environmental Science and Technology , 47(1), 398–404. doi:10.1021/es303394w
- Hahn H.H. , Hoffmann E. , Ødegaard H. (Eds.). (1996, September). Chemical water and wastewater treatment IV. Proceedings of the 7th Gothenburg Symposium 1996, Berlin: Springer.
- Hameed, S. (2017). Effect of the pre-treatment on the performance of MBR, Al-Kut WWTP, wasit governorate. Journal of Chemical Engineering & Process Technology , 8(1), 1–6. doi:10.4172/2157-7048.1000320
- Hem, J. D. , & Roberson, C. E. (1967). Form and stability of aluminum hydroxide complexes in dilute solution. USGS Water Supply Paper , 1827–A, 55.
- Hocaoglu, S. M. , & Orhon, D. (2010). Fate of proteins and carbohydrates in membrane bioreactor operated at high sludge age. Journal of Environmental Science and Health - Part A Toxic/Hazardous Substances and Environmental Engineering , 45(9), 1101–1108. doi:10.1080/10934529.2010.486342
- Hoffmann, C. , & Krause, S. (2013). An innovative approach to improve energy efficiency of Membrane Bioreactors (MBR). Filtrieren und Separieren, 13, 49–52.
- Holbrook, R. D. , Higgins, M. J. , Murthy, S. N. , Fonseca, A. D. , Fleischer, E. J. , Daigger, G. T. , … Novak, J. T. (2003). Effect of alum addition on the performance of submerged membranes for wastewater treatment. Water Environment Research : A Research Publication of the Water Environment Federation , 76(7), 2699–2702. Retrieved from http://www.ncbi.nlm.nih.gov/pubmed/16042118
- Hong, H. , Lin, H. , Mei, R. , Zhou, X. , Liao, B. Q. , & Zhao, L. (2016). Membrane fouling in a membrane bioreactor: A novel method for membrane surface morphology construction and its application in interaction energy assessment. Journal of Membrane Science , 516, 135–143. doi:10.1016/j.memsci.2016.06.006
- Hong, H. , Zhang, M. , He, Y. , Chen, J. , & Lin, H. (2014). Fouling mechanisms of gel layer in a submerged membrane bioreactor. Bioresource Technology , 166, 295–302. doi:10.1016/j.biortech.2014.05.063
- Hu, Y. , Wang, X. C. , Tian, W. , Ngo, H. H. , & Chen, R. (2016). Towards stable operation of a dynamic membrane bioreactor (DMBR): Operational process, behavior and retention effect of dynamic membrane. Journal of Membrane Science , 498, 20–29. doi:10.1016/j.memsci.2015.10.009
- Hua, L.-C. , Huang, C. , Su, Y.-C. , Nguyen, T.-N.-P. , & Chen, P.-C. (2015). Effects of electro-coagulation on fouling mitigation and sludge characteristics in a coagulation-assisted membrane bioreactor. Journal of Membrane Science . 495, 29–36. Retrieved from http://www.sciencedirect.com/science/article/pii/S0376738815300909
- Huang, J. , Wang, Z. , Zhang, J. , Zhang, X. , Ma, J. , & Wu, Z. (2015). A novel composite conductive microfiltration membrane and its anti-fouling performance with an external electric field in membrane bioreactors. Scientific Reports , 5, 1–8. doi:10.1038/srep09268
- Huang, X. , & Wu, J. (2008). Improvement of membrane filterability of the mixed liquor in a membrane bioreactor by ozonation. Journal of Membrane Science , 318(1–2), 210–216. doi:10.1016/j.memsci.2008.02.031
- Huyskens, C. , De Wever, H. , Fovet, Y. , Wegmann, U. , Diels, L. , & Lenaerts, S. (2012). Screening of novel MBR fouling reducers: Benchmarking with known fouling reducers and evaluation of their mechanism of action. Separation and Purification Technology , 95, 49–57. doi:10.1016/j.seppur.2012.04.024
- Hwang, B.-K. , Lee, W.-N. , Park, P.-K. , Lee, C.-H. , & Chang, I.-S. (2007). Effect of membrane fouling reducer on cake structure and membrane permeability in membrane bioreactor. Journal of Membrane Science , 288(1–2), 149–156. doi:10.1016/j.memsci.2006.11.032
- Ibeid, S. , Elektorowicz, M. , & Oleszkiewicz, J. A. (2015). Electro-conditioning of activated sludge in a membrane electro-bioreactor for improved dewatering and reduced membrane fouling. Journal of Membrane Science , 494, 136–142. doi:10.1016/j.memsci.2015.07.051
- Ibeid, S. , Elektorowicz, M. , & Oleszkiewicz, J. A. (2017). Impact of electrocoagulation of soluble microbial products on membrane fouling at different volatile suspended solids’ concentrations. Environmental Technology (United Kingdom) , 38(4), 385–393. doi:10.1080/09593330.2016.1195879
- Inan, H. , Dimoglo, A. , Şimşek, H. , & Karpuzcu, M. (2004). Olive oil mill wastewater treatment by means of electro-coagulation. Separation and Purification Technology , 36(1), 23–31. doi:10.1016/S1383-5866(03)00148-5
- Iorhemen, O. T., Hamza, R. A., & Tay, J. H. (2016). Membrane bioreactor (MBR) technology for wastewater treatment and reclamation: Membrane fouling. Membranes , 6(2), 13–16. Retrieved from http://doi.org/10.3390/membranes6020033
- Ivanovic, I. , & Leiknes, T. (2011). Effect of addition of different additives on overall performance of biofilm-MBR (BF-MBR). Desalination and Water Treatment, 34, 129–135.
- Iversen, V. (2010). Comprehensive assessment of flux enhancers in membrane bioreactors for wastewater treatment (Doctoral Dissertation). Technical University of Berlin, Germany. .
- Iversen, V. , Koseoglu, H. , Yigit, N. O. , Drews, A. , Kitis, M. , Lesjean, B. , & Kraume, M. (2009). Impacts of membrane flux enhancers on activated sludge respiration and nutrient removal in MBRs. Water Research , 43(3), 822–830. doi:10.1016/j.watres.2008.11.022
- Iversen, V. , Mehrez, R. , Horng, R. Y. , Chen, C. H. , Meng, F. , Drews, A. , … Kraume, M. (2009). Fouling mitigation through flocculants and adsorbents addition in membrane bioreactors: Comparing lab and pilot studies. Journal of Membrane Science . doi:10.1016/j.memsci.2009.08.014
- Iversen, V. , Mohaupt, J. , Drews, A. , Lesjean, B. , & Kraume, M. (2008). Side effects of flux enhancing chemicals in membrane bioreactors (MBRs): Study on their biological toxicity and their residual fouling propensity. Water Science and Technology , 57(1), 117–123. doi:10.2166/wst.2008.660
- Jamal Khan, S. , Visvanathan, C. , & Jegatheesan, V. (2012). Effect of powdered activated carbon (PAC) and cationic polymer on biofouling mitigation in hybrid MBRs. Bioresource Technology , 113, 165–168. doi:10.1016/j.biortech.2011.12.107
- Janus, T. , & Ulanicki, B. (2015). A behavioural membrane fouling model for integrated simulation of membrane bioreactors for wastewater treatment. Procedia Engineering , 119(1), 1328–1337. doi:10.1016/j.proeng.2015.08.964
- Jelemenský, M. , Sharma, A. , Paulen, R. , & Fikar, M. (2016). Time-optimal control of diafiltration processes in the presence of membrane fouling. Computers and Chemical Engineering , 91, 343–351. doi:10.1016/j.compchemeng.2016.04.018
- Jhaveri, J. H. , & Murthy, Z. V. P. (2016). A comprehensive review on anti-fouling nanocomposite membranes for pressure driven membrane separation processes. Desalination . 379, 137–154. Retrieved from http://www.sciencedirect.com/science/article/pii/S0011916415301119
- Ji, J. , Li, J. , Qiu, J. , & Li, X. (2014). Polyacrylamide-starch composite flocculant as a membrane fouling reducer: Key factors of fouling reduction. Separation and Purification Technology , 131, 1–7. doi:10.1016/j.seppur.2014.04.013
- Ji, J. , Qiu, J. , Wai, N. , Wong, F. S. , & Li, Y. (2010). Influence of organic and inorganic flocculants on physical-chemical properties of biomass and membrane-fouling rate. Water Research , 44(5), 1627–1635. doi:10.1016/j.watres.2009.11.013
- Ji, J. , Qiu, J. , Wong, F., sin , & Li, Y. (2008). Enhancement of filterability in MBR achieved by improvement of supernatant and floc characteristics via filter aids addition. Water Research , 42(14), 3611–3622. doi:10.1016/j.watres.2008.05.022
- Jiang, T. , Kennedy, M. D. , Guinzbourg, B. F. , Vanrolleghem, P. A. , & Schippers, J. C. (2005). Optimising the operation of a MBR pilot plant by quantitative analysis of the membrane fouling mechanism. Water Science and Technology , 51(6–7), 19–25.
- Jiang, W. , Xia, S. , Liang, J. , Zhang, Z. , & Hermanowicz, S. W. (2013). Effect of quorum quenching on the reactor performance, biofouling and biomass characteristics in membrane bioreactors. Water Research , 47(1), 187–196. doi:10.1016/j.watres.2012.09.050
- Jin, L. , Ong, S. L. , & Ng, H. Y. (2013). Fouling control mechanism by suspended biofilm carriers addition in submerged ceramic membrane bioreactors. Journal of Membrane Science , 427, 250–258. doi:10.1016/j.memsci.2012.09.016
- Johir, M. A. , Shanmuganathan, S. , Vigneswaran, S. , & Kandasamy, J . (2013). Performance of submerged membrane bioreactor (smbr) with and without the addition of the different particle sizes of gac as suspended medium. Bioresource Technology , 141, 13–18. doi:10.1016/j.biortech.2013.03.032
- Johir, M. A. H. , Aryal, R. , Vigneswaran, S. , Kandasamy, J. , & Grasmick, A . (2011). Influence of supporting media in suspension on membrane fouling reduction in submerged membrane bioreactor (smbr). Journal of Membrane Science , 374(1–2), 121–128. Retrieved from doi:10.1016/j.memsci.2011.03.023
- Jørgensen, M. K. , Nierychlo, M. , Nielsen, A. H. , Larsen, P. , Christensen, M. L. , & Nielsen, P. H. (2017). Unified understanding of physico-chemical properties of activated sludge and fouling propensity. Water Research , 120, 117–132. doi:10.1016/j.watres.2017.04.056
- Joss, A. , Böhler, M. , Wedi, D. , & Siegrist, H. (2009). Proposing a method for online permeability monitoring in membrane bioreactors. Water Science and Technology , 60(2), 497–506. doi:10.2166/wst.2009.370
- Juang, Y.-C. , Lee, D.-J. , & Lai, J.-Y. (2010). Visualizing fouling layer in membrane bioreactor. Separation Science and Technology , 45(7), 962–966. doi:10.1080/01496391003666882
- Judd, S. (2008). The status of membrane bioreactor technology. Trends in Biotechnology , 26(2), 109–116. doi:10.1016/j.tibtech.2007.11.005
- Kim, I. , Choi, D. C. , Lee, J. , Chae, H. R. , Hee Jang, J. , Lee, C. H. , … Won, Y. J. (2015). Preparation and application of patterned hollow-fiber membranes to membrane bioreactor for wastewater treatment. Journal of Membrane Science , 490, 190–196. doi:10.1016/j.memsci.2015.04.026
- Kim, J. , Kim, K. , Ye, H. , Lee, E. , Shin, C. , McCarty, P. L. , & Bae, J. (2011). Anaerobic fluidized bed membrane bioreactor for wastewater treatment. Environmental Science & Technology , 45(2),576–581. doi:10.1021/es1027103
- Kim, J. S. , & Lee, C. H. (2003). Effect of powdered activated carbon on the performance of an aerobic membrane bioreactor: Comparison between cross-flow and submerged membrane systems. Water Environment Research , 75(4), 300–307. doi:10.2175/106143003X141105
- Kim, M. J. , Sankararao, B. , & Yoo, C. K. (2011). Determination of MBR fouling and chemical cleaning interval using statistical methods applied on dynamic index data. Journal of Membrane Science , 375(1–2), 345–353. doi:10.1016/j.memsci.2011.04.001
- Kimura, K. , Ogyu, R. , Miyoshi, T. , & Watanabe, Y. (2015). Transition of major components in irreversible fouling of MBRs treating municipal wastewater. Separation and Purification Technology , 142, 326–331. doi:10.1016/j.seppur.2014.12.030
- Kimura, K. , Tanaka, K. , & Watanabe, Y. (2014). Microfiltration of different surface waters with/without coagulation: Clear correlations between membrane fouling and hydrophilic biopolymers. Water Research , 49, 434–443. doi:10.1016/j.watres.2013.10.030
- Kola, A. , Ye, Y. , Ho, A. , Le-Clech, P. , & Chen, V. (2012). Application of low frequency transverse vibration on fouling limitation in submerged hollow fibre membranes. Journal of Membrane Science , 409–410, 54–65. doi:10.1016/j.memsci.2012.03.017
- Koseoglu, H. , Yigit, N. O. , Civelekoglu, G. , Harman, B. I. , & Kitis, M. (2012). Effects of chemical additives on filtration and rheological characteristics of MBR sludge. Bioresource Technology , 117, 48–54. doi:10.1016/j.biortech.2012.04.067
- Koseoglu, H. , Yigit, N. O. , Iversen, V. , Drews, A. , Kitis, M. , Lesjean, B. , & Kraume, M. (2008). Effects of several different flux enhancing chemicals on filterability and fouling reduction of membrane bioreactor (MBR) mixed liquors. Journal of Membrane Science , 320(1–2), 57–64. doi:10.1016/j.memsci.2008.03.053
- Kraume, M. , Wedi, D. , Schaller, J. , Iversen, V. , & Drews, A. (2009). Fouling in MBR: What use are lab investigations for full scale operation? Desalination , 236(1–3), 94–103. doi:10.1016/j.desal.2007.10.055
- Krzeminski, P. , Leverette, L. , Malamis, S. , & Katsou, E. (2017). Membrane bioreactors – A review on recent developments in energy reduction, fouling control, novel configurations, LCA and market prospects. Journal of Membrane Science , 527(December 2016), 207–227. doi:10.1016/j.memsci.2016.12.010
- Kumar, R. V. , Goswami, L. , Pakshirajan, K. , & Pugazhenthi, G. (2016). Dairy wastewater treatment using a novel low cost tubular ceramic membrane and membrane fouling mechanism using pore blocking models. Journal of Water Process Engineering , 13, 168–175. doi:10.1016/j.jwpe.2016.08.012
- Kumirska, J. , Weinhold, M. X. , Thöming, J. , & Stepnowski, P. (2011). Biomedical activity of chitin/chitosan based materials- influence of physicochemical properties apart from molecular weight and degree of N-Acetylation. Polymers , 3(4), 1875–1901. doi:10.3390/polym3041875
- Kunacheva, C. , & Stuckey, D. C. (2014). Analytical methods for soluble microbial products (SMP) and extracellular polymers (ECP) in wastewater treatment systems: A review. Water Research , 61(September 2016), 1–18. doi:10.1016/j.watres.2014.04.044
- Le Clech, P. , Jefferson, B. , Chang, I. S. , & Judd, S. J. (2003). Critical flux determination by the flux-step method in a submerged membrane bioreactor. Journal of Membrane Science , 227(1–2), 81–93. doi:10.1016/j.memsci.2003.07.021
- Le-Clech, P. , Chen, V. , & Fane, T. A. G. (2006). Fouling in membrane bioreactors used in wastewater treatment. Journal of Membrane Science , 284(1–2), 17–53. doi:10.1016/j.memsci.2006.08.019
- Lee, J. C. , Kim, J. S. , Kang, I. J. , Cho, M. H. , Park, P. K. , & Lee, C. H. (2001). Potential and limitations of alum or zeolite addition to improve the performance of a submerged membrane bioreactor. Water Science and Technology , 43(11), 59–66.
- Lee, K. R. , & Yeom, I. T. (2007). Evaluation of a membrane bioreactor system coupled with sludge pretreatment for aerobic sludge digestion. Environmental Technology , 28(7), 723–730. doi:10.1080/09593332808618837
- Lee, S. , Park, S.-K. , Kwon, H. , Lee, S. H. , Lee, K. , Nam, C. H. , … Yi, T. (2016). Crossing the border between laboratory and field: Bacterial quorum quenching for anti-biofouling strategy in an MBR. Environmental Science & Technology . doi:10.1021/acs.est.5b04795
- Lee, S. J. , Dilaver, M. , Park, P. K. , & Kim, J. H. (2013). Comparative analysis of fouling characteristics of ceramic and polymeric microfiltration membranes using filtration models. Journal of Membrane Science , 432, 97–105. doi:10.1016/j.memsci.2013.01.013
- Lee, W.-N. , Chang, I.-S. , Hwang, B.-K. , Park, P.-K. , Lee, C.-H. , & Huang, X. (2007). Changes in biofilm architecture with addition of membrane fouling reducer in a membrane bioreactor. Process Biochemistry , 42(4), 655–661. doi:10.1016/j.procbio.2006.12.003
- Lesage, N. , Sperandio, M. , & Cabassud, C. (2005). Performances of hybrid adsorption/submerged membrane biological process for toxic waste removal.Water Science & Technology, 51(6-7), 173 - 180.
- Lesjean, B. , Rosenberger, S. , Laabs, C. , Jekel, M. , Gnirss, R. , & Amy, G. (2005). Correlation between membrane fouling and soluble/colloidal organic substances in membrane bioreactors for municipal wastewater treatment. Water Science and Technology , 51(6–7), 1–8.
- Lin, J. C. T. , Lee, D. J. , & Huang, C. (2010). Membrane fouling mitigation: Membrane cleaning. Separation Science and Technology , 45(7), 858–872. doi:10.1080/01496391003666940
- Mallampati, R. , Xuanjun, L. , Adin, A. , & Valiyaveettil, S. (2015). Fruit peels as efficient renewable adsorbents for removal of dissolved heavy metals and dyes from water. ACS Sustainable Chemistry and Engineering , 3(6), 1117–1124. doi:10.1021/acssuschemeng.5b00207
- Mannina, G. , & Di Bella, G. (2012). Comparing two start-up strategies for MBRs: Experimental study and mathematical modelling. Biochemical Engineering Journal , 68, 91–103. doi:10.1016/j.bej.2012.07.011
- Maqbool, T. , Khan, S. J. , Waheed, H. , Lee, C. H. , Hashmi, I. , & Iqbal, H. (2015). Membrane biofouling retardation and improved sludge characteristics using quorum quenching bacteria in submerged membrane bioreactor. Journal of Membrane Science , 483, 75–83. doi:10.1016/j.memsci.2015.02.011
- Marbelia, L. , Bilad, M. R. , Bertels, N. , Laine, C. , & Vankelecom, I. F. J. (2016). Ribbed PVC-silica mixed matrix membranes for membrane bioreactors. Journal of Membrane Science , 498, 315–323. doi:10.1016/j.memsci.2015.10.017
- Mateen, F. , Javed, I. , Rafique, U. , Tabassum, N. , Sarfraz, M. , Safi, S. Z. , … Ashraf, M. A. (2016). New method for the adsorption of organic pollutants using natural zeolite incinerator ash (ZIA) and its application as an environmentally friendly and cost-effective adsorbent. Desalination and Water Treatment , 57(14), 6230–6238. doi:10.1080/19443994.2015.1005146
- McClements, D. J. (2006). Non-covalent interactions between proteins and polysaccharides. Biotechnology Advances , 24(6), 621–625. doi:10.1016/J.BIOTECHADV.2006.07.003
- McEwen, J. B. (Ed.). (1998). Treatment process selection for particle removal . Denver: American Water Works Association.
- Mehrnia, M. R. , & Homayoonfal, M. (2016). Fouling mitigation behavior of magnetic responsive nanocomposite membranes in a magnetic membrane bioreactor. Journal of Membrane Science , 520, 881–894. doi:10.1016/j.memsci.2016.08.046
- Meng, F. , Chae, S. R. , Drews, A. , Kraume, M. , Shin, H. S. , & Yang, F. (2009). Recent advances in membrane bioreactors (MBRs): Membrane fouling and membrane material. Water Research , 43(6), 1489–1512. doi:10.1016/j.watres.2008.12.044
- Meng, F. , Zhang, S. , Oh, Y. , Zhou, Z. , Shin, H. S. , & Chae, S. R. (2017). Fouling in membrane bioreactors: An updated review. Water Research , 114, 151–180. doi:10.1016/j.watres.2017.02.006
- Merle, T. , Dramas, L. , Gutierrez, L. , Garcia-Molina, V. , & Croué, J.-P. (2016). Investigation of severe UF membrane fouling induced by three marine algal species. Water Research , 93, 10–19. doi:10.1016/J.WATRES.2016.02.001
- Miller, D. J. , Kasemset, S. , Paul, D. R. , & Freeman, B. D. (2014). Comparison of membrane fouling at constant flux and constant transmembrane pressure conditions. Journal of Membrane Science , 454, 505–515. doi:10.1016/j.memsci.2013.12.027
- Miura, Y. , & Okabe, S. (2008). Quantification of cell specific uptake activity of microbial products by uncultured chloroflexi by microautoradiography combined with fluorescence in situ hybridization quantification of cell specific uptake activity of microbial products by uncultured Ch. Environmental Science & Technology , 42(19), 7380–7386. doi:10.1021/es800566e
- Miyoshi, T. , Tsuyuhara, T. , Ogyu, R. , Kimura, K. , & Watanabe, Y. (2009). Seasonal variation in membrane fouling in membrane bioreactors (MBRs) treating municipal wastewater. Water Research , 43. doi:10.1016/j.watres.2009.08.035
- Molobela, I. P. , Cloete, T. E. , & Beukes, M. (2010). Protease and amylase enzymes for biofilm removal and degradation of extracellular polymeric substances (EPS) produced by Pseudomonas fluorescens bacteria. African Journal of Microbiology Research , 4(14), 1515–1524. Retrieved from http://www.academicjournals.org/ajmr
- Moustafa, M. A. E. (2011). Effect of the pre-treatment on the performance of MBR, Berghausen WWTP. Germany. Alexandria Engineering Journal , 50(2), 197–202. doi:10.1016/j.aej.2011.01.019
- Munz, G. , Gori, R. , Mori, G. , & Lubello, C. (2007). Powdered activated carbon and membrane bioreactors (MBRPAC) for tannery wastewater treatment: Long term effect on biological and filtration process performances. Desalination , 207(1–3), 349–360. doi:10.1016/j.desal.2006.08.010
- Neemann, F. , Rosenberger, S. , Jefferson, B. , & McAdam, E. J. (2013). Non-covalent protein-polysaccharide interactions and their influence on membrane fouling. Journal of Membrane Science , 446, 310–317. doi:10.1016/j.memsci.2013.06.054
- Ngo, H. H. , & Guo, W. (2009). Membrane fouling control and enhanced phosphorus removal in an aerated submerged membrane bioreactor using modified green bioflocculant. Bioresource Technology , 100(18), 4289–4291. doi:10.1016/j.biortech.2009.03.057
- Nguyen, T. T. , Guo, W. , Ngo, H. H. , & Vigneswaran, S. (2010). A new combined inorganic-organic flocculant (CIOF) as a performance enhancer for aerated submerged membrane bioreactor. Separation and Purification Technology , 75(2), 204–209. doi:10.1016/j.seppur.2010.07.010
- Nouri, N. , Mehrnia, M. R. , Sarrafzadeh, M. H. , & Nabizadeh, R. (2013). Performance of membrane bioreactor in presence of flocculants. Desalination and Water Treatment , 52(16–18), 2933–2938. doi:10.1080/19443994.2013.833880
- Ødegaard, H. , Fettig, J. , & Ratnaweera, H. (1990). Coagulation with prepolymerized metal salts. Chemical Water and Wastewater Treatment. Proceedings of the 4th Gothenburg Symposium 1990 October 1 –3,1990 Madrid, Spain . 304, 189–220. Retrieved from http://link.springer.com/chapter/10.1007%252F978-3-642-76093-8_14
- Pal, S. , Mal, D. , & Singh, R. P. (2005). Cationic starch: An effective flocculating agent. Carbohydrate Polymers , 59(4), 417–423. doi:10.1016/j.carbpol.2004.06.047
- Pendashteh, A. R. , Fakhru’l-Razi, A. , Madaeni, S. S. , Abdullah, L. C. , Abidin, Z. Z. , & Biak, D. R. A. (2011). Membrane foulants characterization in a membrane bioreactor (MBR) treating hypersaline oily wastewater. Chemical Engineering Journal , 168(1), 140–150. doi:10.1016/j.cej.2010.12.053
- Poorasgari, E. , Bugge, T. V. , Christensen, M. L. , & Jørgensen, M. K. (2015). Compressibility of fouling layers in membrane bioreactors. Journal of Membrane Science , 475, 65–70. doi:10.1016/j.memsci.2014.09.056
- Porcelli, N. , & Judd, S. (2010). Chemical cleaning of potable water membranes: A review. Separation and Purification Technology , 71(2), 137–143. doi:10.1016/j.seppur.2009.12.007
- Psoch, C. , & Schiewer, S. (2006). Anti-fouling application of air sparging and backflushing for MBR. Journal of Membrane Science . doi:10.1016/j.memsci.2006.06.042
- Qin, L. , Zhang, G. , Meng, Q. , Zhang, H. , Xu, L. , & Lv, B. (2012). Enhanced submerged membrane bioreactor combined with biosurfactant rhamnolipids: Performance for frying oil degradation and membrane fouling reduction. Bioresource Technology , 126, 314–320. doi:10.1016/j.biortech.2012.08.103
- Rahimi, Z. , Zinatizadeh, A. A. L. , & Zinadini, S. (2015). Preparation of high antibiofouling amino functionalized MWCNTs/PES nanocomposite ultrafiltration membrane for application in membrane bioreactor. Journal of Industrial and Engineering Chemistry , 29, 366–374. doi:10.1016/j.jiec.2015.04.017
- Rene, E. R. , & Lewis, A. (2017). Sustainable heavy metal remediation (Vol. 2). Switzerland: Springer.
- Research and Markets . (2014). Membrane Bioreactor (MBR) Market Analysis by Product (Hollow Fiber, Flat Sheet, Multi- Tubular), by Configuration (Submerged, Side stream), by Application and Segment, 120.
- Rosenberger, S. , Evenblij, H. , Te Poele, S. , Wintgens, T. , & Laabs, C. (2005). The importance of liquid phase analyses to understand fouling in membrane assisted activated sludge processes - Six case studies of different European research groups. Journal of Membrane Science , 263(1–2), 113–126. doi:10.1016/j.memsci.2005.04.010
- Ruohomaki, K. , & Nyström, M. (2000). Fouling of ceramic capillary filters in vacuum filtration of humic acid. Filtration and Separation , 37(1), 51–57. doi:10.1016/S0015-1882(00)87633-8
- Saeki, D. , Karkhanechi, H. , Matsuura, H. , & Matsuyama, H. (2016). Effect of operating conditions on biofouling in reverse osmosis membrane processes: Bacterial adhesion, biofilm formation, and permeate flux decrease. Desalination , 378, 74–79. doi:10.1016/j.desal.2015.09.020
- Sakkayawong, N. , Thiravetyan, P. , & Nakbanpote, W. (2005). Adsorption mechanism of synthetic reactive dye wastewater by chitosan. Journal of Colloid and Interface Science , 286(1), 36–42. doi:10.1016/j.jcis.2005.01.020
- Sarkar, B. (2013). A combined complete pore blocking and cake filtration model during ultrafiltration of polysaccharide in a batch cell. Journal of Food Engineering , 116(2), 333–343. doi:10.1016/j.jfoodeng.2012.12.013
- Satyawali, Y. , & Balakrishnan, M. (2009). Effect of PAC addition on sludge properties in an MBR treating high strength wastewater. Water Research , 43(6), 1577–1588. doi:10.1016/j.watres.2009.01.003
- Seo, G. T. , Suzuki, Y. , & Ohgaki, S. (1996). Biological powdered activated carbon (BPAC) microfiltration for wastewater reclamation and reuse. Desalination , 106(1–3), 39–45. doi:10.1016/0011-9164(96)00090-2
- Shen, L. G. , Lei, Q. , Chen, J. R. , Hong, H. C. , He, Y. M. , & Lin, H. J. (2015). Membrane fouling in a submerged membrane bioreactor: Impacts of floc size. Chemical Engineering Journal , 269, 328–334. doi:10.1016/j.cej.2015.02.002
- Shuyan, Q. (2015). Nanofiber as Flocculant or modifier in membrane bioreactors. PhD Proposal , 1. doi:10.1017/CBO9781107415324.004
- Sofia, A. , Ng, W. J. , & Ong, S. L. (2004). Engineering design approaches for minimum fouling in submerged MBR. Desalination , 160(1), 67–74. doi:10.1016/S0011-9164(04)90018-5
- Soler-Cabezas, J. L. , Torà-Grau, M. , Vincent-Vela, M. C. , Mendoza-Roca, J. A. , & Martínez-Francisco, F. J. (2015). Ultrafiltration of municipal wastewater: Study on fouling models and fouling mechanisms. Desalination and Water Treatment , 56(13), 3427–3437. doi:10.1080/19443994.2014.969320
- Song, K. G. , Kim, Y. , & Ahn, K. H. (2008). Effect of coagulant addition on membrane fouling and nutrient removal in a submerged membrane bioreactor. Desalination , 221(1–3), 467–474. doi:10.1016/j.desal.2007.01.107
- Song, W. , Li, Z. , Li, Y. , You, H. , Qi, P. , Liu, F. , & Loy, D. A. (2018). Facile sol-gel coating process for anti-biofouling modification of poly (vinylidene fluoride) microfiltration membrane based on novel zwitterionic organosilica. Journal of Membrane Science , 550(December 2017), 266–277. doi:10.1016/j.memsci.2017.12.076
- Sun, Y. , Fang, Y. , Liang, P. , & Huang, X. (2016). Effects of online chemical cleaning on removing biofouling and resilient microbes in a pilot membrane bioreactor. International Biodeterioration and Biodegradation , 112, 119–127. doi:10.1016/j.ibiod.2016.05.010
- Sweity, A. , Ying, W. , Ali-Shtayeh, M. S. , Yang, F. , Bick, A. , Oron, G. , & Herzberg, M. (2011). Relation between EPS adherence, viscoelastic properties, and MBR operation: Biofouling study with QCM-D. Water Research , 45(19), 6430–6440. doi:10.1016/j.watres.2011.09.038
- Tarnacki, K. , Lyko, S. , Wintgens, T. , Melin, T. , & Natau, F. (2005). Impact of extra-cellular polymeric substances on the filterability of activated sludge in membrane bioreactors for landfill leachate treatment. Desalination , 179(1–3 SPEC. ISS.), 181–190. doi:10.1016/j.desal.2004.11.066
- Tiravanti, G. , Lore, F. , & Sonnante, G. (1985). Influence of the charge density of cationic polyelectrolytes on sludge conditioning. Water Research , 19(1), 93–97. doi:10.1016/0043-1354(85)90329-X
- Tu, X. , Zhang, S. , Xu, L. , Zhang, M. , & Zhu, J. (2010). Performance and fouling characteristics in a membrane sequence batch reactor (MSBR) system coupled with aerobic granular sludge. Desalination , 261(1–2), 191–196. doi:10.1016/j.desal.2010.03.034
- Tzoupanos, N. D. , & Zouboulis, A. I. (2010). Novel inorganic-organic composite coagulants based on aluminium. Desalination and Water Treatment , 13(1–3), 340–347. doi:10.5004/dwt.2010.1042
- Van Breemen, A. N. , Nieuwstad, T. J. , & Van Der Meent-Olieman, G. C. (1979). The fate of fulvic acids during water treatment. Water Research , 13(8), 771–779. doi:10.1016/0043-1354(79)90241-0
- Vanysacker, L. , Boerjan, B. , Declerck, P. , & Vankelecom, I. F. J. (2014). Biofouling ecology as a means to better understand membrane biofouling. Applied Microbiology and Biotechnology , 98(19), 8047–8072. doi:10.1007/s00253-014-5921-2
- Viero, A. F. , Sant’Anna, G. L. , & Nobrega, R. (2007). The use of polyetherimide hollow fibres in a submerged membrane bioreactor operating with air backwashing. Journal of Membrane Science , 302(1–2), 127–135. doi:10.1016/j.memsci.2007.06.036
- Waheed, H. , Xiao, Y. , Hashmi, I. , Stuckey, D. , & Zhou, Y. (2017). Insights into quorum quenching mechanisms to control membrane biofouling under changing organic loading rates. Chemosphere , 182, 40–47. doi:10.1016/j.chemosphere.2017.04.151
- Wang, C. , Yang, F. , Meng, F. , Zhang, H. , Xue, Y. , & Fu, G. (2010). High flux and antifouling filtration membrane based on non-woven fabric with chitosan coating for membrane bioreactors. Bioresource Technology , 101(14), 5469–5474. doi:10.1016/j.biortech.2010.01.126
- Wang, F. , & Tarabara, V. V. (2008). Pore blocking mechanisms during early stages of membrane fouling by colloids. Journal of Colloid and Interface Science , 328(2), 464–469. doi:10.1016/j.jcis.2008.09.028
- Wang, J. , Wu, B. , Yang, S. , Liu, Y. , Fane, A. G. , & Chew, J. W . (2016). Characterizing the scouring efficiency of granular activated carbon (gac) particles in membrane fouling mitigation via wavelet decomposition of accelerometer signals. Journal of Membrane Science , 498, 105–115. doi:10.1016/j.memsci.2015.09.061
- Wang, X.-M. , & Waite, T. D. (2009). Role of gelling soluble and colloidal microbial products in membrane fouling. Environmental Science & Technology , 43(24), 9341–9347. doi:10.1021/es9013129
- Wang, Y. K. , Li, W. W. , Sheng, G. P. , Shi, B. J. , & Yu, H. Q. (2013). In-situ utilization of generated electricity in an electrochemical membrane bioreactor to mitigate membrane fouling. Water Research , 47(15), 5794–5800. doi:10.1016/j.watres.2013.06.058
- Wang, Z. , Cao, J. , & Meng, F. (2015). Interactions between protein-like and humic-like components in dissolved organic matter revealed by fluorescence quenching. Water Research , 68, 404–413. doi:10.1016/J.WATRES.2014.10.024
- Wang, Z. , Ma, J. , Tang, C. Y. , Kimura, K. , Wang, Q. , & Han, X. (2014). Membrane cleaning in membrane bioreactors: A review. Journal of Membrane Science , 468, 276–307. doi:10.1016/j.memsci.2014.05.060
- Wang, Z. , & Wu, Z. (2009). A review of membrane fouling in MBRs: Characteristics and role of sludge cake formed on membrane surfaces. Separation Science and Technology , 44(15), 3571–3596. doi:10.1080/01496390903182578
- Weerasekara, N. A. , Choo, K. H. , & Lee, C. H. (2014). Hybridization of physical cleaning and quorum quenching to minimize membrane biofouling and energy consumption in a membrane bioreactor. Water Research , 67, 1–10. doi:10.1016/j.watres.2014.08.049
- Won, Y. J. , Lee, J. , Choi, D. C. , Chae, H. R. , Kim, I. , Lee, C. H. , & Kim, I. C. (2012). Preparation and application of patterned membranes for wastewater treatment. Environmental Science and Technology , 46(20), 11021–11027. doi:10.1021/es3020309
- Wu, B. , Zamani, F. , Lim, W. , Liao, D. , Wang, Y. , Liu, Y. , Chew, J. W. , & Fane, A. G . (2017). Effect of Mechanical Scouring by Granular Activated Carbon (Gac) on Membrane Fouling Mitigation. Desalination, 403, 80–87. doi:10.1016/j.desal.2015.12.003
- Wu, J. , Chen, F. , Huang, X. , Geng, W. , & Wen, X. (2006). Using inorganic coagulants to control membrane fouling in a submerged membrane bioreactor. Desalination , 197(1–3), 124–136. doi:10.1016/j.desal.2005.11.026
- Wu, J. , & Huang, X. (2008). Effect of dosing polymeric ferric sulfate on fouling characteristics, mixed liquor properties and performance in a long-term running membrane bioreactor. Separation and Purification Technology , 63(1), 45–52. doi:10.1016/j.seppur.2008.03.033
- Wu, J. , & Huang, X. (2010). Use of ozonation to mitigate fouling in a long-term membrane bioreactor. Bioresource Technology , 101(15), 6019–6027. doi:10.1016/j.biortech.2010.02.081
- Wu, S. C. , & Lee, C. M. (2011). Correlation between fouling propensity of soluble extracellular polymeric substances and sludge metabolic activity altered by different starvation conditions. Bioresource Technology , 102(9), 5375–5380. doi:10.1016/j.biortech.2010.11.093
- Xia, L. , Law, A. W. K. , & Fane, A. G. (2013). Hydrodynamic effects of air sparging on hollow fiber membranes in a bubble column reactor. Water Research , 47(11), 3762–3772. doi:10.1016/j.watres.2013.04.042
- Xiao, Y. , Waheed, H. , Xiao, K. , Hashmi, I. , & Zhou, Y. (2018). In tandem effects of activated carbon and quorum quenching on fouling control and simultaneous removal of pharmaceutical compounds in membrane bioreactors. Chemical Engineering Journal . doi:10.1016/j.cej.2018.02.073
- Xin, Y. , Bligh, M. W. , Kinsela, A. S. , Wang, Y. , & David Waite, T. (2015). Calcium-mediated polysaccharide gel formation and breakage: Impact on membrane foulant hydraulic properties. Journal of Membrane Science , 475, 395–405. doi:10.1016/J.MEMSCI.2014.10.033
- Xu, M. , Wen, X. , Huang, X. , Yu, Z. , & Zhu, M. (2013). Mechanisms of membrane fouling controlled by online ultrasound in an anaerobic membrane bioreactor for digestion of waste activated sludge. Journal of Membrane Science , 445, 119–126. doi:10.1016/j.memsci.2013.06.006
- Xu, W. , & Chellam, S. (2005). Initial stages of bacterial fouling during dead-end microfiltration. Environmental Science and Technology , 39(17), 6470–6476. doi:10.1021/es0500862
- Yavuztürk Gül, B. , & Koyuncu, I. (2017). Assessment of new environmental quorum quenching bacteria as a solution for membrane biofouling. Process Biochemistry , 61(December 2016), 137–146. doi:10.1016/j.procbio.2017.05.030
- Yeom, I. T. , Lee, K. R. , Choi, Y. G. , Kim, H. S. , Kwon, J. H. , Lee, U. J. , & Lee, Y. H. (2005). A pilot study on accelerated sludge degradation by a high-concentration membrane bioreactor coupled with sludge pretreatment. Water Science and Technology , 52(10–11), 201–210.
- Yigit, N. , Civelekoglu, G. , Harman, I. , Köseoǧlu, H. , & Kitis, M. (2011). Effects of various backwash scenarios on membrane fouling in a membrane bioreactor. Environmental Earth Sciences , 237(1–3), 917–929. doi:10.1007/978-3-540-95991-5-87
- Yin, Z. , Tarabara, V. V. , & Xagoraraki, I. (2016). Effect of pressure relaxation and membrane backwash on adenovirus removal in a membrane bioreactor. Water Research , 88, 750–757. doi:10.1016/j.watres.2015.10.066
- Ying, Z. , & Ping, G. (2006). Effect of powdered activated carbon dosage on retarding membrane fouling in MBR. Separation and Purification Technology , 52(1), 154–160. doi:10.1016/j.seppur.2006.04.010
- Yonekawa, H. , Tomita, Y. , & Watanabe, Y. (2004). Behavior of micro-particles in monolith ceramic membrane filtration with pre-coagulation. Water Science and Technology , 50(12), 317–325.
- Yoon, S.-H.-H. , & Collins, J. H. (2006). A novel flux enhancing method for membrane bioreactor (MBR) process using polymer. Desalination , 191(1–3), 52–61. doi:10.1016/j.desal.2005.04.124
- Yu, H. Y. , Hu, M. X. , Xu, Z. K. , Wang, J. L. , & Wang, S. Y. (2005). Surface modification of polypropylene microporous membrane to improve its antifouling property in MBR: CO2 plasma treatment. Separation and Purification Technology , 45(1), 8–15. doi:10.1016/j.seppur.2005.01.012
- Yu, H. Y. , Xu, Z. K. , Lei, H. , Hu, M. X. , & Yang, Q. (2007). Photoinduced graft polymerization of acrylamide on polypropylene microporous membranes for the improvement of antifouling characteristics in a submerged membrane-bioreactor. Separation and Purification Technology , 53(1), 119–125. doi:10.1016/j.seppur.2006.07.002
- Yuan, W. , Kocic, A. , & Zydney, A. L. (2002). Analysis of humic acid fouling during microfiltration using a pore blockage-cake filtration model. Journal of Membrane Science , 198(1), 51–62. doi:10.1016/S0376-7388(01)00622-6
- Yusuf, Z. , Wahab, N. A. , & Abusam, A. (2017). Neural network-based model predictive control with CPSOGSA for SMBR filtration. International Journal of Electrical and Computer Engineering (IJECE) , 7(3), 1538. doi:10.11591/ijece.v7i3.pp1538-1545
- Zahrim, A. Y. , & Dexter, Z. D. (2016). Decolourisation of palm oil mill biogas plant wastewater using Poly-Diallyldimethyl Ammonium Chloride (polyDADMAC) and other chemical coagulants. IOP Conference Series: Earth and Environmental Science , 36, 1. doi:10.1088/1755-1315/36/1/012025
- Zarei, A. , Moslemi, M. , & Mirzaei, H. (2016). The combination of KMnO4 oxidation and polymeric flocculation for the mitigation of membrane fouling in a membrane bioreactor. Separation and Purification Technology , 159, 124–134. doi:10.1016/j.seppur.2016.01.003
- Zarragoitia-González, A. , Schetrite, S. , Alliet, M. , Jáuregui-Haza, U. , & Albasi, C. (2008). Modelling of submerged membrane bioreactor: Conceptual study about link between activated slugde biokinetics, aeration and fouling process. Journal of Membrane Science , 325(2), 612–624. doi:10.1016/j.memsci.2008.08.037
- Zhang, H. , Gao, Z. , Zhang, L. , & Song, L. (2014). Performance enhancement and fouling mitigation by organic flocculant addition in membrane bioreactor at high salt shock. Bioresource Technology , 164, 34–40. doi:10.1016/j.biortech.2014.04.053
- Zhang, H., Sun, B., Zhao, X., & Gao, Z. (2008). Effect of ferric chloride on fouling in membrane bioreactor. Separation and Purification Technology , 63(2), 341–347. doi:10.1016/j.seppur.2008.05.024
- Zhang, H. , Yu, H. , Zhang, L. , & Song, L. (2015). Bioresource technology stratification structure of polysaccharides and proteins in activated sludge with different aeration in membrane bioreactor. Bioresource Technology , 192, 361–366. doi:10.1016/j.biortech.2015.05.025
- Zhang, J. , Chua, H. C. , Zhou, J. , & Fane, A. G. (2006). Factors affecting the membrane performance in submerged membrane bioreactors. Journal of Membrane Science , 284(1–2), 54–66. doi:10.1016/j.memsci.2006.06.022
- Zhang, J. , Satti, A. , Chen, X. , Xiao, K. , Sun, J. , Yan, X. , … Huang, X. (2015). Low-voltage electric field applied into MBR for fouling suppression : Performance and mechanisms. Chemical Engineering Journal , 273, 223–230. doi:10.1016/j.cej.2015.03.044
- Zhang, Z. , Wang, Y. , Leslie, G. L. , & Waite, T. D. (2015). Effect of ferric and ferrous iron addition on phosphorus removal and fouling in submerged membrane bioreactors. Water Research , 69, 210–222. doi:10.1016/j.watres.2014.11.011
- Zhao, C. , Xu, X. , Chen, J. , Wang, G. , & Yang, F. (2014). Highly effective antifouling performance of PVDF/graphene oxide composite membrane in membrane bioreactor (MBR) system. Desalination , 340(1), 59–66. doi:10.1016/j.desal.2014.02.022
- Zhao, D. , & Yu, S. (2015). A review of recent advance in fouling mitigation of NF/RO membranes in water treatment: Pretreatment, membrane modification, and chemical cleaning. Desalination and Water Treatment , 55(4), 870–891. doi:10.1080/19443994.2014.928804
- Zhao, R. J. , Wang, W. S. , Zhu, F. F. , Liu, T. , Li, Y. H. , & Bian, Y. L. (2015). Surface modification of PVDF membrane by simultaneously using low temperature plasma and ammonium carbonate solution. Desalination and Water Treatment , 56(9), 2276–2283. doi:10.1080/19443994.2014.961172
- Zheng, Y. , Zhang, W. , Tang, B. , Ding, J. , Zheng, Y. , & Zhang, Z. (2018). Membrane fouling mechanism of biofilm-membrane bioreactor (BF-MBR): Pore blocking model and membrane cleaning. Bioresource Technology , 250(August 2017), 398–405. doi:10.1016/j.biortech.2017.11.036
- Zhou, S. , Xue, A. , Zhao, Y. , Li, M. , Wang, H. , & Xing, W. (2013). Grafting polyacrylic acid brushes onto zirconia membranes: Fouling reduction and easy-cleaning properties. Separation and Purification Technology , 114, 53–63. doi:10.1016/j.seppur.2013.04.023
- Zhou, Z. , Meng, F. , He, X. , Chae, S.-R. , An, Y. , & Jia, X. (2015). Metaproteomic analysis of biocake proteins to understand membrane fouling in a submerged membrane bioreactor. Environmental Science & Technology , 49(2), 1068–1077. doi:10.1021/es504489r
- Zuthi, M. F. R. , Guo, W. , Ngo, H. H. , Nghiem, D. L. , Hai, F. I. , Xia, S. , … Liu, Y. (2017). New and practical mathematical model of membrane fouling in an aerobic submerged membrane bioreactor. Bioresource Technology , 238, 86–94. doi:10.1016/j.biortech.2017.04.006