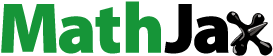
Abstract
Operators of Earthmoving Equipment (EME) are daily exposed to certain Whole-body vibration (WBV) dosage, exceeding the recommended values. In this study, the WBV experienced by operators of EME used in construction industries were measured using a tri-axial accelerometer (GCDC X16-4 3-Axis, G-Force Data logger, ±18 g acceleration range). These measurements were taken on the seats and floors of EME in x, y and z directions. Weighted root-mean-square accelerations (A RMS), Vibration dose values (VDVs), Crest Factors (CFs), time to reach health guidance caution zone (HGCZ) which are important evaluation parameters specified by the International Organiization for Standardization (ISO) were determined for each equipment used in the study. The values of the CF were mostly found to be greater than 9 indicating presence of multiple shocks during the operation of the equipment studied. The research revealed higher magnitude of acceleration in the vertical, z-axis, some very few are within the HGCZ (A RMS < 0.47 and >0.93 m/s2; VDV < 8.5 and >17 m/s1.75) specified by ISO, also accelerations on x- and y-axes fell within the HGCZ and very few below the lower limit 0.47 m/s2. The values of the A RMS and VDV were also compared with the European Parliament Directive 2002/44/EC (A(8) < 0.5 and >2.5 m/s2; VDV < 9 and >21 m/s1.75). Appropriate technology should be adopted to minimize the WBV reaching operators, and/or appropriate maintenance routine should be maintained in EMEs to combat the health risks associated with WBV.
PUBLIC INTEREST STATEMENT
Whole-body vibration (WBV) is often encountered during the operation of locomotive vehicle ranging from light cars to heavy earthmoving equipment. The International Organization for Standardization (ISO) and other international standards have stipulated certain limits of WBV dosage. They have also highlighted methods of WBV evaluation and the appropriate equipment (accelerometer and data acquisition devices) which are very expensive. Due to economy of evaluation, consumer device accelerometers compactible like android, apple and window devices were invented. This study investigated the inaccuracy of these consumer device accelerometers and suggests adequate and better technology to foster the attempt of WBV evaluation at an affordable cost. The accelerometers available on consumer devices are recommended for WBV monitoring rather than evaluation; they can however be used for evaluation upon great improvement.
1. Introduction
Whole-body vibration (WBV) is encountered in the operation of Earthmoving Equipment (EME) at different frequencies as a result of the undulated terrains where they are used, the engines’ vibration and the nature of the task they perform (Smith & Leggat, Citation2005; Zhao & Schindler, Citation2014). Typical terrains, where EME are used, are characterized by undulations, bumps, holes, pits and rocks. They can also be rough and sometimes slippery. WBV occurs when the human body is in contact with a surface which is vibrating e.g. in all forms of transport and when working near some industrial machinery. WBV arises from the usage of EME in a wide variety of operations performed in industries, such as mining and construction, forestry and agriculture and public utilities (Griffin, Citation1990).
EME as classified by Yamazaki Construction Company (Citation2003) includes scrapers, dozers, heavy haul trucks, backhoes, graders, pail loaders, etc. These equipment are used to perform tasks such as excavating, earthmoving and paving on the construction sites. Others are equipment such as plough attached to a tractor on the agricultural sites and equipment such as haulage trucks, scissors lift, tunnelling equipment, bucket chain excavator, bucket wheel excavator and gyratory equipment on the mining sites.
Human vibration can either be classified as Hand-arm Vibration (HAV) or WBV. HAV is a mechanical vibration or shock applied to the hand-arm system directly (usually through the hand or fingers) e.g. vibrations from the handles of power tools or work pieces shaken by vibrating or impacting tools (Griffin, Citation2008; Su, Hoe, Masilamani, & Awang-Mahmud, Citation2011). HAV can also be transmitted to the hands and arms system when operating vibrating tools (e.g. chain saws, hand grinders) and vibrating controls (e.g. steering wheels, levers). Exposure to HAV over a number of years can result in permanent physical damage commonly known as Raynaud’s disease (Paschold & Mayton, Citation2011) or white finger syndrome (Occupational Safety and Health Administration, Citation2000) or vibration can cause damage to the muscles and joints of the wrist and elbow (Paschold & Mayton, Citation2011).
On the other hand, WBV is the vibration of human body as a result of standing/lying on a vibrating floor or sitting on a vibrating seat, often encountered near heavy machinery and on construction equipment, trucks and buses. WBV is transmitted through the feet (standing or vibrating surface), buttocks, to the entire body (when lying on a vibrating surface or floor) or back (through the seat or seat backrest) (Griffin, Citation1990). Long-term exposure can result in serious physical damage to the lower portion of the spine or it can disturb the nervous system. Vibration whose frequency level is between 0.5 and 80 Hz are of most concern when measuring WBV (Paschold & Mayton, Citation2011).
Operation of EME involves long-hour, long-term exposure to WBV. A study by Hulshof et al. (Citation2009) showed that WBV in EME and forestry machines are of higher intensity when compared with taxis and other commuter vehicles, consequently operators of EME are exposed to higher dosage of WBV exceeding the recommended limits. Operators’ body parts have various frequencies they can tolerate before responding to the effect of the WBV. Figure shows the various frequencies at which human body responds to vibration.
Figure 1. Simplified mechanical system representing a body subjected to vibration (Rasmussen, Citation2009).
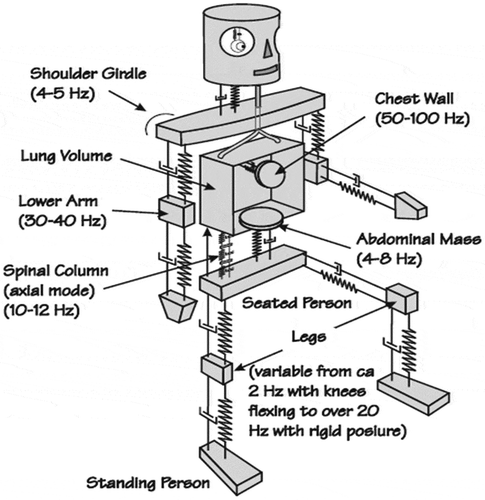
The human body is extremely complex in nature, when modelled as a mechanical system as shown in Figure , it can be considered to contain a number of linear as well as non-linear elements, and the mechanical properties are quite different from person to person (Cvetanovic & Zlatkovic, Citation2013; Rasmussen, Citation2009; VonGierke & Brammer, Citation2002). Exposure to vibration frequencies exceeding the stipulated threshold as shown in Figure results in unwanted medical conditions. Low back pain (LBP) is a type of Musculoskeletal disorder (MSD), that is common and often affects most people, linked with occupational exposure to WBV. MSDs are injuries and disorders of the soft tissues (muscles, tendons, ligaments, joints and cartilage) and nervous system. They can affect nearly all tissues, including the nerves and tendon sheaths, and most frequently involve the arms and back (OSHA, Citation2000). The symptoms of LBPs are often prevalent among the operators of EME (Zhao & Schindler, Citation2014), operators exposed to WBV, especially for prolong duration and long term, are prone to LBPs (Bovenzi, Citation2009; Bovenzi & Hulshof, Citation1998; Darby, Citation2008; Gallias and Griffin, Citation2009; Wolfgang & Burgess-Limerick, Citation2014a). WBV exposure in EME is associated with MSDs and specifically LBPs (Tiemessen, Hulsof, & Frings-Dresen, Citation2007). Other effects of WBV are spinal column, digestive, cardio-vascular and reproductive diseases (Joubert, Citation2009).
ISO stipulates Health Guidance Caution Zones (HGCZs) between upper bound of acceleration 0.93 m/s2 and lower bound 0.47 m/s2 (ISO 2631-1, Citation1997; McPhee, Foster, & Long, Citation2009).
Several studies had been carried out to evaluate WBV exposure among operators of EME (Cann, Salmoni, Vi, & Eger, Citation2004; Contratto & Du, Citation2008; Costa & Arezes, Citation2009; Cvetanovic & Zlatkovic, Citation2013; Eger, Salmoni, Cann, & Jack, Citation2006; Kittusamy & Buchholz, Citation2004; Kumar, Citation2004; Mayton, Miller, & Jobe, Citation2008; Muzammil, Siddiqui, & Hasan, Citation2004; Pope et al., Citation2001; Viswanathan, Jorgensen, & Kittusamy, Citation2006; Wolfgang and Burgess-limerick, Citation2014a). However, no research was found to compensate for variation in nature of terrain in Nigeria and every other part of the world where related research had been carried out. Moreso, global synergy is required as to the standard dosage of human vibrations.
A recent study by Wolfgang and Burgess-Limerick (Citation2014b) developed a low-cost, yet effective consumer electronic accelerometer to evaluate WBV due to the high cost of evaluating WBV with the seat-pad accelerometer and data-analysis systems. The consumer devices developed uses apple iOS application, WBV (i-phone from OS 5 and above). The device was however tested with a standard tri-axial seat pad accelerometer, and there were no significant differences between the accelerations measured by the two devices.
The novelty of the research is to enumerate the problem of WBV in a different terrain and suggests possible recovery strategy. Section 1 highlights the research problem of WBV and sourced relevant literature; Section 2 gives the detailed methodology of the research; Section 3 shows the research findings, while Section 4 presents conclusions.
2. Materials and methods
2.1. Whole-body vibration measurement and evaluation
The magnitude of WBV experienced by the operators of the selected EME was measured using the calibrated tri-axial accelerometer X16-4 (GCDC X16-4 3-Axis, G-Force Data logger, ±18 g acceleration range) MEMS-type accelerometer and a consumer device accelerometer called WBV, LIS331DLH MEMS type as well as available on apple device OS5s. Comparison of these two accelerometers was carried out by Akinnuli and colleagues (Akinnuli, Dahunsi, Ayodeji & Bodunde, Citation2017). The measurement captures the acceleration in the x, y, and z directions by both the accelerometers, but methodological choice was given to the calibrated tri-axial accelerometer (X16-4 data logger) based on the recommendations made by Akinnuli and colleagues (Akinnuli et al., Citation2017).
2.2. Experimental design
EME were selected in the Federal University of Technology, Akure, Ondo State, along Ohanaze Road, behind the School of Earth and Mineral Sciences where construction works were being done in the University and also selected at Banks-Iyin Road Links, Ado Ekiti, Ekiti State, where constructions were ongoing as well. These selections were based on availability of EME performing earthmoving operation, which could allow for the WBV measurements.
The EMEs selected for the study are bulldozers, excavators, graders, pail loaders, vibrating rollers and backhoes. They were selected because they are the major EMEs used during construction projects in the selected study areas. Two of each equipment were chosen for the purpose of comparison. The bulldozers were coded B1 and B2, excavators were coded E1 and E2, graders were coded G1 and G2, pail loaders were coded P1 and P2, vibrating rollers were coded R1 and R2 and backhoes were coded H1 and H2, respectively.
The accelerometers were oriented to the basicentric axes of the operators’ body. The basicentric axis of the accelerometer is as shown in Figure .
2.3. Analysis of WBV data
The magnitude of WBV was measured using the tri-axial accelerometer GCDC, X16-4 MEMS-type accelerometer and consumer device accelerometer called WBV, LIS331DLH MEMS type, but choice was given to the GCDC, X16-4 MEMS-type accelerometer. A time-acceleration domain was collected and downloaded into a computer. They were analysed and the ISO evaluation indices were determined using the weighting factor of Wd (kx = ky = 1.4) and Wf (kz = 1), respectively.
2.3.1. Determination of RMS average acceleration
ISO specified an RMS-based method of evaluating WBV from the time–acceleration domain data obtained from the accelerometer. The Equation 1 is used to evaluate the weighted root-mean-square acceleration, A RMS. The weighted acceleration method of WBV evaluation is the recommended technique by the ISO through the ISO 2631-1 (Citation1997) and the European Parliament Directive 2002/44/EC.
where
A RMS = RMS average acceleration (m/s2);
aw (t) = acceleration at time t (m/s2); and
T = period of exposure (seconds).
2.3.2. Determination of crest factor
The crest factor (CF) was determined to ascertain the presence of shock, multiple shocks, jolts or jars in the vibration exposure magnitude of the operators. Equation 2 is used to determine the value of the CF in each axis of the acceleration.
where
CF = crest factor;
Maximum aw = highest value of acceleration in each axis (m/s2); and
A RMS = RMS average acceleration (m/s2).
2.3.3. Determination of vibration dose value (VDV)
To factor in the presence of multiple shock in vibration exposure, the fourth-power VDV, in m/s1.75, is evaluated using the relationship in Equation 3.
where aw (t) = acceleration at time t (m/s2) and
T = period of exposure (seconds).
2.3.4. Determination of seat-effective amplitude transmissibility (SEAT)
Having determined the RMS average accelerations and the VDVs on the floor and on the seat, the SEAT for the RMS average acceleration and the VDV were determined using the relationship in Equations 4 and 5. SEAT value for convenient operation of any equipment that exposes its operator to WBV is 1. The SEAT was determined to know if the values of SEATs will exceed 1.
where
= RMS average acceleration on the seat (m/s2)
= RMS average acceleration on the seat (m/s2)
VDVseat = Vibration dose value on the seat (m/s1.75)
VDVfloor = Vibration dose value on the floor (m/s1.75)
3. Results and discussions
The specifications and the maintenance histories of the vehicle gotten from the maintenance log of the consulted companies are shown in Table and it could be deduced that the equipment used for the research were not too old such that inherent vibration of the machine could exceed the recommended action and limit values 0.47 and 0.93 m/s2, respectively, by ISO standards. The values of the average speed were gotten from a digital velocity measurement available on the consumer device accelerometer (WBV, LIS331DLH MEMS type) mentioned in 2.1 above.
Table 1. Selected earthmoving equipment specification, speed and maintenance history
3.1. WBV evaluation
The evaluation parameters specified by ISO 2631-1 (Citation1997) RMS average acceleration, VDVs, CFs, SEAT determined were thus analysed as shown in various illustrations.
The values of the A RMS on the seat and the floor are shown in Figures and . B1 and B2 represent bulldozer, E1 and E2 represent excavator, G1 and G2 represent grader, P1 and P2 represent pail loader, R1 and R2 represent vibrating roller and H1 and H2 represent backhoe loader in the Figures. Comparing the values of the accelerations with the recommended Exposure Action Value (EAV) and Exposure Limit Value (ELV) 0.47 and 0.93 m/s2, respectively, more values of the acceleration were found above the EAV. The acceleration on the vertical axis (z-axis) is greater than the accelerations on the horizontal and lateral axes (x- and y-axes), respectively.
All the values of vertical acceleration fell in the HGCZ i.e. between the EAV and ELV. This implies that the vertical acceleration during the operation of EME was severe and needed action. ISO recommends action when any of the three axes accelerations are found above the EAV and most importantly quick action when they are found above the ELV.
WBV exposure exceeding and falling within the HGCZ is in agreement with the inferences of Eger et al. (Citation2006) and Mayton et al. (Citation2008). Also comparing results with those of Wolfgang and Burgess-limerick’s (Citation2014b); Eger et al.’s (Citation2006); Kumar’s (Citation2004); and Smets et al.’s (Citation2010), there is consistency in the finding that the highest value of acceleration is predominantly found on the vertical axis (z-axis).
The magnitude of acceleration was found to be more severe on the floor. This indicates that there is acceleration attenuation from the floor to the seat. Attenuation is the reduction of the vibration from the floor to the seat; obviously, the floor will experience more vibration dose than the seat. The vertical acceleration in the excavators and the backhoes are all above ELV, this is due to the fact that the excavator and the backhoe mostly work on a single station and vibrate while doing the earthmoving task. The high values of vertical acceleration experienced in the excavators and backhoes are a result of poor maintenance history (major overhauling done, as shown in Table ) of them.
CFs are used to determine the presence of shock in vibration accelerations. If CF > 9, it indicates that there is presence of multiple shock in the acceleration magnitude. This limits the usage of average RMS acceleration for the evaluation of WBV. If any of the axes’ CF is greater than 9 in a particular evaluation, there is shock in such vibration. CFs were found mostly to be greater than 9 indicating presence of multiple shocks during WBV exposure of the operator of EME, Appendix A shows details of the evaluation data.
3.2. Evaluation of vibration dose values (VDV)
Since most CF > 9, the average RMS acceleration is not sufficient to evaluate the WBV exposure. The VDV uses the fourth-power value of the acceleration which is used to evaluate vibration that includes multiple shocks. Just like the average RMS acceleration, the VDV exposure action and limit values are 8.5 and 17 m/s1.75, respectively. Figures – show the vibration dosage behaviour of the evaluation. Figure ’s VDV indicates that all the vertical accelerations are above the ELV stipulated by standard. Some of the x- and y-axes accelerations are also found above the ELV and some are between the HGCZ recommendations. This connotes that all the equipment used for the study needs appropriate maintenance.
3.3. Seat-effective amplitude transmissibility (SEAT)
The difference in the average RMS accelerations and VDVs on the floor and seat of the EME is a topic of the SEAT. SEAT is used to determine if the vibration is attenuated from the floor to the seat. If SEAT is greater 1, then the conditions of the vibration on the seat include the behaviour of the operator during operation or poor seat design. As seen in Figure , the SEAT value was set as 1, it is shown with the straight line between the dots perpendicular to the acceleration axis. Figure shows more attenuation of the vibration by the seats of the EME. For the bulldozer and the grader DN8 and C14G, respectively, the SEAT values were found to be greater than 1, indicating poor isolation from the vibration by the seat of the EME; this is as a result of the maintenance history of the equipment.
3.4. Comparison of ISO 2631-1(Citation1997) with 2002/44/EC
Figure shows the comparison between the two standards used in the study, both standards use 8-hour energy equivalent of RMS average acceleration in the axis where the highest acceleration exists. For equipment G2 and P2, the highest values of acceleration were found in the y-axis. This could be attributed to even/level terrain where the equipment are used during the WBV measurement.
Figure 8. ISO 2631-(Citation1997) and 2002/44/EC standard comparison.
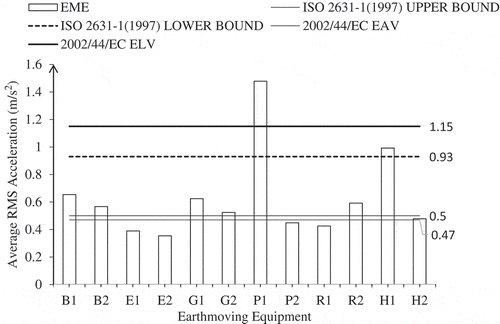
It could then be inferred that high x-axis or y-axis accelerations arose as a result of operating speed. This inference is consistent with the finding of Wolfgang and Burgess-limerick’s (Citation2014b) which attributed high values of vertical vibration acceleration to the road-way condition. This is as well consistent with the findings of Eger, Contratto, and Dickey (Citation2011) and McPhee et al. (Citation2009).
Contratto and Du’s (Citation2008) findings inferred that speed and height of bumps (a typical undulated terrain where EME are used) have significant effect on the magnitude of the acceleration.
The type of equipment, age and the maintenance history of the equipment sourced for this study tell on the levels of WBV exposure of the operators. The vibrating roller which ordinarily, one will expect a high magnitude of WBV; the case is reverse. This arguably is as a result of the nature of terrain where the roller is used. This agrees with the report of Scarlett et al. (Citation2007) that the nature of the terrain may increase the magnitude of vertical accelerations while the speed influences the magnitude of the transverse and the longitudinal accelerations. Vibrating roller does finishing work of a grader to compact the soil just before the asphalt is laid in construction projects. Other machines show high magnitude of z-axis acceleration.
Scarlett et al. (2007) also predicted variability in the acceleration values on the floor and seat of an equipment. This study measured the acceleration on the floor simultaneously with that of the seat to evaluate the seat absorbability of the WBV.
4. Conclusions
The study was carried out to examine ergonomic exposure of the operators of EME in the construction sites to WBV. The problem is not the operation of EME, but exposure to extreme WBV magnitudes. The study revealed that operators of EME are exposed to WBV values exceeding the EAV and ELV recommended by ISO and those by European Union through their 2002/44/EC European Parliament and the Council of the European Union, (2002/44/EC), (Citation2002).
The values of vertical acceleration were predominantly found to be the highest values due to the nature of terrains. Since the nature of the site terrain cannot be manipulated, seats of the EME can be improved to attenuate the vibration reaching the seated operator.
More studies are required in the area of establishing proportional division to all the factors contributing to WBV, since it is not only WBV that contributes to MSDs. There should be a dosage–exposure relationship to depict the time an operator should be exposed to WBV.
More study times are required to establish appropriate dose–response relationship and regulation/standard of WBV exposure should be made from this particularly for Nigerian Operators. As pointed out in the study, it is impossible to manipulate terrains before carrying out earthmoving operations. Hence, newer/brand new equipment and/or appropriately maintained equipment should be used for similar research in the future to infer variability of inferences i.e. to compare the results of WBV gotten from newer equipment with those gotten from the present research and to justify the need to establish a dose–response relationship for comfortable operations of EME.
Acknowledgements
The authors wish to acknowledge the management of KOPEK, Smace and DROMO construction Industries, Nigeria, for allowing their operators to take part in this research.
Additional information
Funding
Notes on contributors
B.O. Akinnuli
Dr. B.O. Akinnuli is an Associate Professor in the Department of Industrial and Production Engineering of the Federal University of Technology, Akure, Nigeria.
O.A. Dahunsi
Dr. O.A Dahunsi is an Associate Professor in the Department of Mechanical Engineering of the Federal University of Technology, Akure, Nigeria.
S.P. Ayodeji
Dr. S.P. Ayodeji is an Associate Professor in the Department of Industrial and Production Engineering of the Federal University of Technology, Akure, Nigeria. He is currently the Head of Department.
O.P. Bodunde
Mr. O.P. Bodunde is a Lecturer in the Department of Mechanical and Mechatronics Engineering of the Afe Babalola University, Ado-Ekiti, Nigeria, and a PhD Student in the Department of Mechanical Engineering of the Federal University of Technology, Akure, Nigeria.
The current interest of this group of researchers is basically, but not limited to, Occupational Safety and Ergonomics.
References
- Akinnuli, B. O. , Dahunsi, O. A. , Ayodeji, S. P. , & Bodunde, O. P. , (2017). Investigation and validation of consumer device accelerometer for the assessment of whole-body vibration. Cogent Engineering , 4(1398703), 1–11. doi:10.1080/23311916.2017.1398703
- Bovenzi, M. (2009). A longitudinal study of low back pain and daily exposure in professional drivers. In Proceedings of the 4th international conference on whole-body vibration injuries (pp. 3–4). June 2–4th Montreal, Canada.
- Bovenzi, M. , & Hulshof, C. T. J. (1998). An updated review of epidemiologic studies of the relationship between exposure to whole-body vibration and low back pain. Journal of Sound and Vibration , 215(4), 595. doi:10.1006/jsvi.1998.1598
- Cann, A. P. , Salmoni, A. W. , Vi, P. , & Eger, T. R. (2004). An exploratory study of whole-body vibration exposure and dose while operating heavy equipment in the construction industry. Applied Occupational and Environmental Hygiene , 18(12), 999–1005. doi:10.1080/715717338
- Contratto, M. S. , & Du, J. C. (2008). Evaluation of vibration exposure of operators in wheel tractor-scrapper. In Proceedings of the 2nd American conference on human vibration, Chicago, IL. June 4–6: 28–29.
- Costa, N. , & Arezes, P. M. (2009). The influence of operator driving characteristics in whole-body vibration exposure from electrical fork-lift trucks. International Journal of Industrial Ergonomics , 39, 34–38. doi:10.1016/j.ergon.2008.06.004
- Cvetanovic, B. , & Zlatkovic, D. (2013). Evaluation of whole-body vibration risk in agricultural tractor drivers. Bulgarian Journal of Agricultural Science , 19(5), 1155–1160.
- Darby, A. M. (2008). Whole-body vibration and ergonomics toolkits. Health and Safety Laboratory . Retrieved February 16, 2015, from, Health and Safety Executives www.hse.gov.uk/research/rrpdf/rr612.pdf
- Eger, T. , Contratto, M. , & Dickey, J. (2011). Influence of driving speed, terrain, seat performance and ride control on predicted health risk based on ISO 2631-1 and EU directive 2002/44/EC. Journal of Low Frequency, Noise and Vibration Active Control , 30(4), 291–312. doi:10.1260/0263-0923.30.4.291
- Eger, T. , Salmoni, A. , Cann, A. , & Jack, R. (2006). Whole-body vibration exposure experienced by mining equipment operators. Occupational Ergonomics , 6(3–4), 121–127.
- European Parliament and the Council of the European Union, (2002/44/EC) . (2002). Minimum health and safety requirements regarding the exposure of workers to the risks arising from physical agents (Vibration). Official Journal of the European Communities Directive 2002/44/EC on the OJ L177 , 6(7), 13.
- Gallais, L. , & Griffin, M. J. (2009). Low back pain and risk factors for low back pain in taxi drivers. In Proceedings of the 4th international conference on whole body vibration injuries, Montreal, Canada . June 2–4th: 5–6
- Griffin, M. J. (1990). Handbook of human vibration . London: Academic Press.
- Griffin, M. J. (2008). Negligent exposures to hand-transmitted vibration. International Archives of Occupational and Environmental Health , 81(5), 645–659. doi:10.1007/s00420-007-0251-7
- Hulshof, C. , Tiemessen, I. , Bovenzi, M. , Hagberg, M. , Lundstrom, R. , Nilsson, T. , … Palmer, K. (2009). Low back pain in drivers exposed to whole-body vibration: The vibrisks multicenter study. In Proceedings of the 4th International Conference on Whole-body Vibration Injuries , Montreal, Canada . June 2–4th: 1–2
- International Standards Organization, (ISO) ISO 2631–1 . (1997). Mechanical vibration and shock. Guide for the Evaluation of Human Exposure to Whole-body Vibration, Part 1 . ISO 2631/1-1997.
- Joubert, D. M. (2009). Professional driving and adverse reproductive outcomes: The evidence to date and research challenges. The Open Occupational Health & Safety Journal , 1(1–6), 1–6.
- Kittusamy, N. K. , & Buchholz, B. (2004). Whole-body vibration and postural stress among operators of construction equipment: A literature review. Journal of Safety Research , 35(3), 255–261. doi:10.1016/j.jsr.2004.03.014
- Kumar, S. (2004). Vibration in operating heavy haul trucks in overburden mining. Applied Ergonomics , 35(6), 509–520. doi:10.1016/j.apergo.2004.06.009
- Mayton, A. G. , Miller, R. E. , & Jobe, C. C. (2008). Assessment of whole-body vibrtaion exposure on haulage trucks and front-end loaders at US aggregate stone operations. In Proceedings of the 2nd American conference on human vibration, Chicago, IL . June 4–6: 26–27.
- McPhee, B. , Foster, G. , & Long, A. (2009). Bad vibrations (2nd ed.). Sydney: Coal Services Health & Safety Trust.
- Muzammil, M. , Siddiqui, S. S. , & Hasan, F. (2004). Physiological effects of vibrations on tractors drivers under variable ploughing conditions. Journal of Occupational Health , 46(5), 403–409. doi:10.1539/joh.46.403
- Occupational Safety and Health Administration (OSHA) . (2000). Ergonomics: The study of work . US, Department of Labor. Retrieved February 16, 2015, from. OSHA 3125 (2000) Revised www.osha.gov/Publications/osha3125.pdf
- Paschold, H. W. , & Mayton, A. G. (2011). Whole-body vibration: Building awareness in safety. American Society of Safety Engineers , 56(4): 30-35.
- Pope, M. , Magnusson, M. , Lundström, R. , Hulshof, C. , Bovenzi, M. , & Verbeek, J. (2001). Guidelines for whole-body vibration health surveillance. In Proceedings of the 2nd international conference on whole-body vibration injuries , Siena, Italy. November 7–9th: 49–50.
- Rasmussen, G. (2009). Human body vibration and its measurement. Journal of the Acoustic Society of America , 73(6). doi:10.1121/1.389513.
- Scarlett, A. J. , Price, J. S. , & Stayner, R. M. (2007). Whole-body vibration: Evaluation of emission and exposure levels arising from agricultural tractors. Journal of Terramechanics , 44(1), 65-73. doi:10.1016/j.jterra.2006.01.006
- Smets, M. P. H. , Eger, T. R. , & Grenier, S. G. (2010). Whole-body vibration experienced by haulage truck operators in surface mining operations: A comparison of various analysis methods utilized in the prediction of health risks. Applied Ergonomics , 41(6), 763–770. doi:10.1016/j.apergo.2010.01.002
- Smith, D. R. , & Leggat, P. A. (2005). Whole-body vibration: Health effects, measurement and minimization. Professional Safety , 50(7), 35–40.
- Su, T. A. , Hoe, V. C. W. , Masilamani, R. , & Awang-Mahmud, A. B. (2011). Hand-arm vibration syndrome among a group of construction workers in Malaysia. Occupational Environment Medicine , 68(1), 58–63. doi:10.1136/oem.2009.052373
- Tiemessen, I. , Hulsof, C. , & Frings-Dresen, M. (2007). An overview of strategies to reduce whole-body vibration exposure on drivers: A systematic review. Industrial Ergonomics , 37(3), 245–256. doi:10.1016/j.ergon.2006.10.021
- Viswanathan, M. , Jorgensen, M. J. , & Kittusamy, N. K. (2006). Field evaluation of a continuous passive lumbar motion system among operators of earthmoving equipment. International Journal of Industrial Ergonomics , 36(7), 651–659. doi:10.1016/j.ergon.2006.04.006
- VonGierke, H. E. , & Brammer, A. J. (2002). The effect of shock and vibration on human. In Chapter 42. Shock and vibration handbook (pp. 1–62). New York, NY: The McGraw-Hill Inc.
- Wolfgang, R. , & Burgess-Limerick, R. (2014a). Using consumer electronic devices to estimate whole-body vibration exposure. Journal of Occupational and Environmental Hygiene , 11(6), 77–81. doi:10.1080/15459624.2014.888073
- Wolfgang, R. , & Burgess-Limerick, R. (2014b). Whole-body vibration exposure of haul truck drivers at a surface coal mine. Applied Ergonomics , 45(6), 1700–1704. doi:10.1016/j.apergo.2014.05.020
- Yamazaki Construction Company (2003). Earth moving plan. Retrieved February 16, 2015, from yamazaki.co.jp/data/school/pdf/earthm.pdf
- Zhao, X. , & Schindler, C. (2014). Evaluation of whole-body vibration exposure experienced by operators of a compact wheel loader according to ISO 2631-1:1997 and ISO 2631-5:2004. International Journal of Industrial Ergonomics , 44(6), 840–850. doi:10.1016/j.ergon.2014.09.006