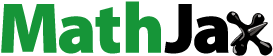
Abstract
With growing global concerns related to energy security and sustainability, interest in bio-fuels has increased significantly. Production of biofuel mostly begins with pyrolysis, a process that converts the biomass to liquid biooil, solid biochar and gases. A large amount of carbonaceous biochar is generated as a by-product during the pyrolysis. However, no major effort has been made in the past decade to utilize the biochar in pavement applications, especially as a bio-modifier to asphalt binders. In this study, an attempt was made to evaluate the carbonaceous biochar, obtained during bio-fuel production through pyrolysis of Mesua ferrea seed cover waste, as an asphalt modifier/extender to obtain bio-asphalts. Physical and chemical characterizations of biochar were carried out using scanning electron microscopy, Fourier transform infrared spectroscopy and energy dispersive X-ray analysis. This was followed by rheological characterization of asphalt binders (from two sources) modified with various concentrations of biochar (0, 5, 10, 15, and 20% by weight of binder). Flow behaviour, permanent deformation, and fatigue characteristics of bio-asphalts were evaluated and compared with control binders (no biochar). Aging susceptibility of biochar modified binders was also evaluated and compared for biochar contents and binder sources. Permanent deformation behaviour evaluated through multiple stress creep and recovery (MSCR) test indicated that the use of biochar improved the rutting resistance of binders. Improved binder performance against the effects of aging and deformation with the use of biochar makes it a promising asphalt modifier, especially for the warm tropical climatic conditions prevalent in India.
PUBLIC INTEREST STATEMENT
About 95% of all highways in the world are surfaced with asphalt mixtures. Asphalt binder is the key component in these mixtures as it binds the aggregates together to provide a smooth and comfortable riding surface. Considering the fact that asphalt binder is derived from petroleum crude—a non-renewable fossil fuel—there are concerns related to consistent supply of asphalt binder for future road infrastructure development. This research has focused on partial replacement of asphalt binder with the carbonaceous biochar, obtained during bio-fuel production through pyrolysis of Mesua ferrea seed cover waste. Biochar as well as the bio-asphalt binder are characterized using various physical, chemical and rheological tests. Biochar, a by-product of bio-fuel production during the pyrolysis, is derived from renewable biomass sources and hence can be a sustainable asphalt modifier. Findings of the study suggest that biochar-modified asphalt binders show an improved performance against the effects of aging and deformation, thus making the biochar as a promising asphalt modifier.
1. Introduction
In recent times, along with many other countries, India is also making continual efforts to transition from fossil-fuel-based resources to the renewable sources of energy (MNRE, Citation2009). In this regard, biofuels, which are derived from renewable biomass resources, have amassed substantial attention as alternative fuels to promote energy security and environmental stewardship. Production of biofuel mostly begins with pyrolysis (Mohan, Pittman, & Steele, Citation2006), a process that converts the biomass to the liquid biooil, solid biochar and gases. Pyrolysis is the thermal decomposition of organic matter in the absence of oxygen or when significantly less oxygen is available than required for combustion (Demirbas, Citation2009; Mohan et al., Citation2006). Liquid biooil is further upgraded to derive biofuels, and the solid biochar, rich in carbon, is generated as a by-product (Zhao, Huang, Ye, Shu, & Jia, Citation2014a).
Biochar obtained as pyrolysis by-product of switchgrass was used by Zhao et al. (Citation2014a). A commercially available activated carbon was used as the control modifier. Influence of method of pyrolysis, pyrolysis temperature, modifier size, and modifier content was evaluated using one source of neat PG 64–22 asphalt binder. Addition of biochar improved the rutting and aging resistance of asphalt binder, while showing little effect on fatigue and cracking properties. It was concluded that biochar is a more effective modifier than activated carbon within a dosage of 10% by weight of asphalt binder. In another study, Zhao, Huang, Shu, and Ye (Citation2014b) evaluated properties of asphalt binders and mixtures containing biochar obtained from pyrolysis of switchgrass. Two commercially available carbon-based additives carbon black and micro-sized carbon fibres were used as reference additives. Addition of biochar did not impact low-temperature cracking resistance of the asphalt binder, while the resistance decreased with carbon black and fibres. Biochar was found to improve the rutting resistance, cracking resistance, and resistance to moisture-induced damages of the mixtures. Celoglu, Yilmaz, Kok, and Yalcin (Citation2016) used biochars from pyrolysis of walnut crust and apricot seed shell as bitumen modifiers. Classical tests (penetration, softening point, viscosity) and dynamic analysis result showed that biochars increased the binder stiffness, and thus have promising potential to reduce permanent deformation of asphalt pavements.
Mesua ferrea (local name: “Nahor”) is a non-edible oil seed tree species abundant in north-eastern region of India (Bordoloi, Narzari, Chutia, Bhaskar, & Kataki, Citation2015). The seed cover of M. ferrea is generated as a waste during the process of oil extraction. Some studies (Bordoloi et al., Citation2015; Chutia, Kataki, & Bhaskar, Citation2013) have reported pyrolysis of M. ferrea seed cover to obtain biooil as a pyrolysis product, and a source for renewable fuel and value added chemicals. Biochar is generated as a by-product during the pyrolysis of the seed cover. The present study is the first attempt in India to utilize this by-product as a potential asphalt modifier.
Utilization of carbon-based materials for modification of asphalt binders has been attempted earlier also by asphalt researchers. Carbon black (Alliotti, Citation1962), carbon fibre (Huang, Chen, & Shu, Citation2009), coke dust (Taha, Ali, & Delwar, Citation1998), and carbon nano-tubes (Ziari, Farahani, Goli, & Sadeghpour Galooyak, Citation2014) are some of the carbon-based materials that have been successfully used as asphalt additives/modifiers for improved performance. Positive results reported with other carbon-based asphalt additives, together with the possibility of using a carbonaceous by-product of pyrolysis process, motivated the present study on the use of biochar as a modifier to asphalt binder.
The primary objective of this study was to investigate the possibility of incorporating carbonaceous biochar, obtained as a by-product during the pyrolysis of M. ferrea seed cover, in asphalt binders. Biochar-modified asphalt binders were produced with two sources of base asphalt binders at five different biochar contents. The biochar was characterized via scanning electron microscopy (SEM), Fourier transform infrared spectroscopy (FTIR), and energy dispersive X-ray spectroscopy (EDX). To assess the performance of bio-asphalt binders, they were tested for flow behaviour, permanent deformation, fatigue, and aging resistance. Flow behaviour of the binders was evaluated through measurement of viscosity of unaged and short-term aged bio-asphalt binders at varying shear rates. Resistance against the permanent deformation was evaluated through the Superpave rutting parameter (G*/sin δ) and multiple stress creep and recovery (MSCR) tests. Aging potential of the biochar-modified asphalt binders was also quantified through the measurement of rheological aging index (RAI). Properties of control (without biochar or neat binder) and biochar-modified binders were analysed and compared.
2. Materials description and methodology
2.1. Materials and their characterization
Two neat (unmodified) VG–30 asphalt binders (equivalent to 60/70 penetration grade) were selected for this study. The two binders were obtained from two different petroleum refineries in India. Two sources were selected to understand the effect of binder source on biochar modification. They are designated as source-1 and source-2 in this paper. Table presents the physical properties of these two binders.
Table 1. Properties of asphalt binders
The biochar used in the current study was a by-product obtained during the pyrolysis of M. ferrea seed cover at 450°C with heating rate 40°C/min and nitrogen flow rate of 100 mL/min. The schematic representation of pyrolysis experimental unit is given in Figure . Quartz reactor of length 480 mm and internal diameter 30 mm is equipped with inert gas (nitrogen) connection to create oxygen-less environment for pyrolysis (Figure ). The reactor was heated externally through an electric furnace, and the temperature of reactor was controlled by a Ni–Cr–Ni thermocouple attached inside the reactor. Further details on the pyrolysis process can be found elsewhere (Bordoloi et al., Citation2015; Kumar, Choudhary, Narzari, & Kataki, Citation2018). Figure shows the steps involved in the production of biochar. The resultant biochar sample was then allowed to cool to room temperature for further testing.
Figure 1. Schematic diagram of pyrolysis set-up (after Choudhury, Chutia, Bhaskar, & Kataki, Citation2014).
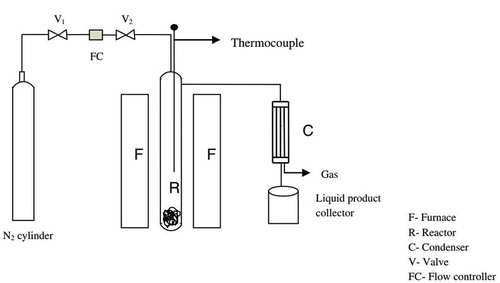
Figure 2. Steps involved in biochar production: (a) Mesua ferrea seed, (b) dried seed cover, (c) grinded seed cover prior to its pyrolysis and (d) biochar obtained from pyrolysis.
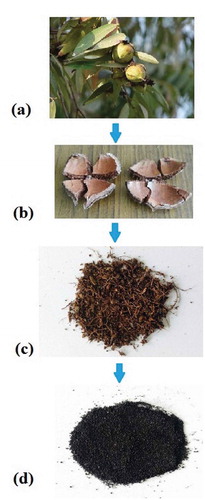
SEM image of biochar sample shown in Figure reveals highly irregular, porous and rough surface features. A rough and porous surface texture is expected to enhance the physicochemical interaction of biochar with asphalt binder bringing about more effective binder modification (Zhao et al., Citation2014b, Citation2014a).
Chemical functional groups present in the biochar were identified using the FTIR analysis. FTIR spectrum was recorded in a Nicolet IR spectrometer at ambient temperature (26 ± 2°C). A spectral range of 4000–400 cm–1 was used at a resolution of 4 cm–1 averaging 64 scans for each measurement. Results of FTIR analysis of biochar sample are shown in Figure . In the biochar spectrum, a strong peak at 3420 cm−1 corresponded to O–H stretching due to the presence of phenolic O–H group; a peak at 2910 cm−1 was attributed to C-H stretching of aliphatic CHx, a peak at 1639 cm−1 indicated conjugated C = C phenyl rings; a peak at 1438 cm−1 is attributed to C–H in-plane bends; and a peak at 632 cm−1 indicated the presence of C–Br stretch aliphatic bromo compounds. A weak peak between 1032 and 1192 cm−1 corresponded to C–O secondary alcohol stretch and a peak around 875–602 cm−1 is attributed to strong bend C–H phenyl rings. A weak peak between 1032 and 1192 cm−1 corresponded to C–O secondary alcohol stretch and a peak around 875–602 cm−1 is attributed to strong bend C–H phenyl rings. Broad peak at 632 cm−1 indicated the presence of C–Br stretch aliphatic bromo compounds.
EDX analysis was used for the elemental analysis of biochar. Results of EDX analysis shown in Figure indicate that carbon is the major element (87.5%) followed by oxygen (10.3%), potassium (1.3%), and other trace elements.
2.2. Sample preparation
Biochar modified asphalt binders were prepared by first drying the biochar for 2 h at 110°C and then introducing it in the asphalt binder at the contents of 5%, 10%, 15% and 20% by weight of binder. The biochar was first sieved through a 150-μm sieve before its addition to the asphalt binders. Blending was performed for 30 min at 160°C using a high shear mechanical blender. Biochar was added in small increments during blending to avoid its agglomeration in the binder.
2.3. Test methods
Rheological investigations on biochar modified asphalt binders were conducted through dynamic shear rheometer (DSR) and rotational viscometer. To study the effect of biochar on aging characteristics of asphalt binder, short-term and long-term aging of control and biochar modified binders were simulated in a rolling thin film oven (RTFO) and a pressure aging vessel (PAV), respectively. The short-term aging of control and biochar-modified binders was done at 163°C for 85 min as per ASTM D2872 (ASTM, Citation2017a). Long-term aging was performed as per ASTM D6521 (ASTM, Citation2017b) on short-term aged binders subjected to 2.1 MPa pressure and 100°C temperature for 20 h.
Shear rate dependence of biochar modified asphalt binders was found through viscosity measurements with Brookfield rotational viscometer (spindle 27) at varying shear rates. Anton Paar MCR 102 DSR was used for measurement of rheological characteristics of different biochar modified binders. For unaged and short-term aged binders, a parallel plate geometry (25 mm dia plate with 1 mm gap) was used, whereas long-term aged binders were tested using 8 mm dia plate with 2 mm gap.
MSCR test was conducted on short-term aged binders as per ASTM D7405 (ASTM, Citation2017c) specifications using DSR with a parallel plate (25 mm diameter) geometry and 1 mm test gap. The MSCR test is used to evaluate permanent deformation resistance of asphalt binders under varying stress levels. Use of low- and high-stress levels in the test allows complete mobilization of the modifiers and thus helps to assess stress dependency of the biochar modified asphalt binders. Specimens were tested at 60°C at two stress levels of 0.1 and 3.2 kPa. The test consisted of 10 creep-recovery cycles at each stress level, with each cycle comprising of 1 s creep loading time and 9 s recovery time. The accumulated strain in the asphalt binder was recorded with time.
The non-recovered strain (εnr ) at the end of each creep and recovery cycle when divided by the appropriate stress level yields non-recoverable compliance (Jnr, kPa−1) as follows:
where εnr is the non-recoverable strain at the end of recovery and σ is the stress level. Jnr indicates the rutting resistance of asphalt binder. Lower Jnr values are desirable for better performance against rutting. Furthermore, the per cent difference in Jnr calculated at 3.2 kPa and 0.1 kPa stress levels yields another parameter Jnr, diff that indicates the sensitivity of the binder to varying stress levels. Thus, it can be obtained as follows:
where Jnr , 3.2 and Jnr , 0.1 are the non-recoverable compliance at stress levels of 3.2 kPa and 0.1 kPa, respectively. Lower Jnr, diff are desirable as they correspond to binders with lower stress sensitivity. AASHTO TP 70 (American Association of State Highway and Transportation Officials TP 70 [AASHTO], Citation2013) specifies an upper limit of 75% for the per cent difference in Jnr values (Jnr, diff ). Figure shows the experimental flowchart for the present study.
3. Results and discussion
3.1. Flow behaviour
Apparent viscosity was measured for control (without biochar) binder and biochar modified binders at 135°C as function of shear rate, and the results are presented in Figure . It is observed that viscosity of biochar modified binders increases with an increase in biochar content, suggesting an improvement in the binder stiffness. With respect to binder source, relatively higher values of viscosity are observed for source-2 binder. Source-2 binder is stiffer compared to source-1 binder as also observed from the test results presented in Table . Furthermore, all the combinations are able to meet the Superpave maximum viscosity requirement of 3.0 Pa.s at 135°C, for an unaged binder. Viscosity is found to be nearly constant with the increase in shear rate, indicating that both control and biochar modified binders exhibit Newtonian behaviour (i.e. viscosity is independent of shear rate). The per cent increase in viscosity, calculated by considering viscosity of control binder as the base, observed with the addition of biochar, is nearly the same at a given biochar content for both binder sources. For example, 5% biochar elevated the viscosity by 22.5% in case of source-1, and by 21.9% for source-2. This indicates that biochar has similar effect on the viscosity for both binder sources.
3.2. Rutting parameter
Superpave rutting parameter (G*/sin δ; G*: complex shear modulus, δ: phase angle) was measured using DSR at 10 rad/s for unaged and short-term aged binders to assess their resistance towards permanent deformation. The results of G*/sin δ measured from 46°C to 76°C for source-1 binders are illustrated in Figure . Similar trends are observed for source-2 binders. A consistent increase in G*/sin δ with increase in biochar content is seen at all temperatures for both unaged and short-term aged binders. Improvement in G*/sin δ values is attributed to the stiffening effect resulting from the addition of biochar, and is in agreement with the viscosity results. Source-2 binders exhibit comparatively higher G*/sin δ values than source-1 binders at all biochar contents and temperatures. This is also expected as the source-2 control binder is relatively stiffer than source-1 control (as seen from viscosity results for control binders).
Figure 8. Superpave rutting parameter (G*/sin δ) of source-1 asphalt binders: (a) unaged state and (b) short-term aged state.
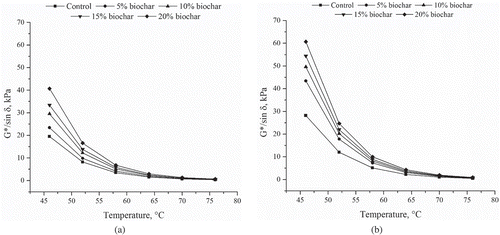
Using the results of G*/sin δ, failure temperatures and corresponding high performance grades (PG) of all binders are determined. Failure temperatures in unaged and short-term aged states correspond to the maximum temperature at which G*/sin δ attains a minimum value of 1.0 kPa and 2.2 kPa, respectively. Table presents the results of failure temperature and PG. It is seen that addition of biochar consistently increases the failure temperature of binders from both sources. This points towards better resistance against permanent deformation of the biochar modified binders (Fini, Hosseinnezhad, Oldham, Chailleux, & Gaudefroy, Citation2017; Julaganti, Choudhary, & Kumar, Citation2017; Oruç, Yılmaz, & Sancak, Citation2016). A bump in the high PG grade from PG 70 to PG 76 is observed for binder source-2.
Table 2. Failure temperature results
3.3. Aging susceptibility
The effect of aging on the properties of control and biochar modified binders was evaluated through RAI at 64°C based on the Superpave rutting parameter:
Lower RAI values indicate a low susceptibility towards aging and hence better aging resistance (Ali, Mashaan, & Karim, Citation2013; Ashish, Singh, & Bohm, Citation2017). RAI results shown in Figure indicate that there is a marginal but consistent decrease in RAI values with subsequent increase in the biochar content for binders of both sources. It is observed that with comparatively higher RAI values, source-2 binders are more aging susceptible than source-1 binders. The results further indicate that addition of biochar slightly reduces the aging susceptibility of the binders as the RAI of biochar modified binders is lower than the control binders. Hence, an increase in biochar content lowers the changes in G*/sin δ caused due to aging, and thus helps improve the resistance of the binder towards aging. The results of FTIR analysis demonstrated the presence of phenolic group in pyrolytic carbon, and the presence of phenolic compounds is known to impart anti-oxidant properties to asphalt binders. The ability of phenolic structures to act as asphalt anti-oxidant has been reported previously (Pan, Citation2012; Williams & McCready, Citation2008). A phenolic structure consists of one or more hydroxyl groups attached to benzene ring. The structure has the ability to neutralise oxygen containing free radicals, i.e. ketones and sulfoxides generated during asphalt binder oxidation (Pan, Citation2012). This likely explains the decrease in RAI values with an increase in biochar percentage.
3.4. Fatigue parameter
Superpave binder fatigue parameter (G*sin δ) was determined for long-term aged binders. Short-term aged binders were subjected to long-term aging in PAV, and were then tested through DSR at intermediate-service temperatures of 19°C to 34°C at 10 rad/s frequency. Failure temperature in this case is defined as the temperature corresponding to the Superpave specified maximum limit of 5000 kPa for the fatigue parameter (G*sin δ). The test results are presented in Figure . Comparatively higher stiffness of source-2 control binder than source-1 control binder is evident from Figure . An increase in biochar content from 0% to 20% slightly increases the failure temperature by 4.9°C for source-1 and by 4.6°C in case of binders from source-2. Nearly equal slopes of G*sin δ vs. temperature curves shown in Figure (for both binder sources) indicate that addition of biochar does not alter temperature dependency of binders for the intermediate service temperatures.
3.5. MSCR test results
MSCR test was performed on control and biochar-modified binders at a typical high-service temperature of 60°C. Three parameters were obtained from the test: accumulated strain, non-recoverable compliance (Jnr ), and difference in non-recoverable compliance (Jnr, diff ).
Figure shows the plot of accumulated strain recorded as a function of time. Results up to a time of 100 s correspond to the first 10 load cycles at the stress level of 0.1 kPa, and the results from 100 s to 200 s represent the 10 load cycles at 3.2 kPa. The addition of biochar reduces the final accumulated strain values by 25%, 30%, 45%, and 63%, respectively, at the contents of 5%, 10%, 15%, and 20% for source-1 binders. Corresponding reductions were 15%, 40%, 42%, and 62% for source-2 binders. This indicates a favourable effect of addition of biochar on permanent deformation resistance of asphalt binders (Mirzababaei, Nejad, & Vanaei, Citation2017; Singh, Ashish, Kataware, & Habal, Citation2017).
Figure indicates the results of Jnr at 3.2 kPa stress level. Lesser Jnr values are desirable for better performance against permanent deformation (DuBois, Mehta, & Nolan, Citation2014; Singh et al., Citation2017; Wasage, Stastna, & Zanzotto, Citation2011), because a lesser Jnr indicates that higher strains are recovered during the recovery phase of the MSCR test. As seen from Figure , Jnr decreases with an increase in biochar content at both stress levels and for binders of both sources. Jnr values of source-2 binders are significantly lower than those from source-1.
The parameter Jnr, diff is used as a measure of stress-sensitivity of an asphalt binder. It is preferable to use binders with less sensitivity towards stresses arising from vehicle axle loads. As per AASHTO TP 70, a maximum limit of 75% is specified for Jnr, diff . Figure shows the results of Jnr, diff . All binders are found to meet the specified limit of 75%. Furthermore, it is noted that with an increase in biochar content, there is consistent decrease in Jnr, diff , and thus the stress-sensitivity. Considering the effect of the two binder sources, source-2 binders exhibit lower Jnr, diff than the binders from source-1. The results indicate that addition of biochar reduces the stress-sensitivity of asphalt binders, and the extent of reduction is dependent on the binder source.
4. Conclusions
The present study investigated the feasibility of using carbonaceous biochar, a by-product of pyrolysis of Mesua ferrea seed cover, as modifier/extender to asphalt binders. Bio-asphalt binders were prepared with five contents of biochar (0, 5, 10, 15, and 20% by weight of binder) along with two sources of base asphalt binders. Flow behaviour, permanent deformation, fatigue and aging characteristics of biochar modified binders were evaluated and compared with control binders (without biochar). On the basis of results and discussion presented earlier, the following conclusions can be drawn:
Addition of biochar increased the viscosity of asphalt binders and showed a Newtonian behaviour up to 20% biochar content used in the study.
Addition of biochar improved the permanent deformation resistance in terms of the Superpave rutting parameter (G*/sin δ) at high-service temperatures. Addition of biochar decreased the susceptibility towards aging of the bio-asphalt binders estimated using the RAI.
MSCR results showed that addition of biochar reduced accumulated strain and non-recoverable compliance (J nr), thereby improving rutting resistance of the binders. Based on J nr,diff results, the stress-sensitivity of all the binders was found to be within the specified limit and it decreased with an increase in the biochar content.
The findings of the study indicate that biochar obtained as a by-product during pyrolysis of M. ferrea seed cover waste can be a viable additive to enhance performance of asphalt binders. Use of biochar—a by-product derived from renewable biomass resource during bio-fuel production—will also be a step forward in the direction of energy security and sustainability.
Additional information
Funding
Notes on contributors
Rajan Choudhary
Rajan Choudhary The group’s key research areas are asphalt technology, pavement materials characterization, thermo-chemical conversion of biomass and wastes to bio-fuels, carbon-sequestration, geosynthetic and fibre-reinforced soil engineering, and pavement geotechnics. The research group is involved in inter-disciplinary research on innovative and novel technologies that contribute towards sustainable road construction. The group is currently exploring novel technological and material advancements for achieving sustainable road construction along with improved pavement performance.
References
- Ali, A. H. , Mashaan, N. S. , & Karim, M. R. (2013). Investigations of physical and rheological properties of aged rubberised bitumen. Advances in Materials Science and Engineering , 239036, 1–7. doi:10.1155/2013/239036
- Alliotti, A. G. (1962). Carbon black– Its nature and possible effects on the characteristics of bituminous road binders. Proceedings of the 1st Australian Road Research Board (ARRB) conference, Canberra.
- AASHTO. (2013). Standard method of test for Multiple Stress Creep Recovery (MSCR) test of asphalt binder using a Dynamic Shear Rheometer (DSR). American Association of State and Highway Transportation Officials, AASHTO TP 70-13.
- Ashish, P. K. , Singh, D. , & Bohm, S. (2017). Investigation on influence of nanoclay addition on rheological performance of asphalt binder. Road Materials and Pavement Design , 18(5), 1007–1026. doi:10.1080/14680629.2016.1201522
- ASTM D2872 – 12e1 . (2017a). Standard test method for effect of heat and air on a moving film of asphalt (Rolling thin-film oven test) . West Conshohocken, PA: ASTM International. doi:10.1520/D2872-12E01
- ASTM D6521 – 13 . (2017b). Standard practice for accelerated aging of asphalt binder using a Pressurized Aging Vessel (PAV) . West Conshohocken, PA: ASTM International. doi:10.1520/D6521-13
- ASTM D7405 – 15 . (2017c). Standard test method for Multiple Stress Creep and Recovery (MSCR) of asphalt binder using a dynamic shear rheometer . West Conshohocken, PA: ASTM International. doi:10.1520/D7405-15
- Bordoloi, N. , Narzari, R. , Chutia, R. S. , Bhaskar, T. , & Kataki, R. (2015). Pyrolysis of Mesua ferrea and Pongamia glabra seed cover: Characterization of bio-oil and its sub-fractions. Bioresource Technology , 178, 83–89. doi:10.1016/j.biortech.2014.10.079
- Celoglu, M. E. , Yilmaz, M. , Kok, B. V. , & Yalcin, E. (2016, June 1–3). Effects of various biochars on the high temperature performance of bituminous binder. 6th Euraphalt & Eurobitume Congress . Prague, Czech Republic: European Asphalt Pavement Association (EAPA) and Eurobitume. doi: 10.14211/EE.2016.232
- Choudhury, N. D. , Chutia, R. S. , Bhaskar, T. , & Kataki, R. (2014). Pyrolysis of jute dust: Effect of reaction parameters and analysis of products. Journal of Material Cycles and Waste Management , 16(3), 449–459. doi:10.1007/s10163-014-0268-4
- Chutia, R. S. , Kataki, R. , & Bhaskar, T. (2013). Thermogravimetric and decomposition kinetic studies of Mesua ferrea L. deoiled cake. Bioresource Technology , 139, 66–72. doi:10.1016/j.biortech.2013.03.191
- Demirbas, A. (2009). Biofuels – Securing the planet’s future energy needs . London: Springer Publications. doi:10.1007/978-1-84882-011-1
- DuBois, E. , Mehta, Y. , & Nolan, A. (2014). Correlation between multiple stress creep recovery (MSCR) results and polymer modification of binder. Construction and Building Materials , 65, 184–190. doi:10.1016/j.conbuildmat.2014.04.111
- Fini, E. H. , Hosseinnezhad, S. , Oldham, D. J. , Chailleux, E. , & Gaudefroy, V. (2017). Source dependency of rheological and surface characteristics of bio-modified asphalts. Road Materials and Pavement Design , 18(2), 408–424. doi:10.1080/14680629.2016.1163281
- Huang, B. , Chen, X. , & Shu, X. (2009). Effects of electrically conductive additives on laboratory-measured properties of asphalt mixtures. Journal of Materials in Civil Engineering , 21(10), 612–617. doi:10.1061/(ASCE)0899-1561(2009)21:10(612)
- Julaganti, A. , Choudhary, R. , & Kumar, A. (2017). Rheology of modified binders under varying doses of WMA additive–Sasobit. Petroleum Science and Technology , 35(10), 975–982. doi:10.1080/10916466.2017.1297827
- Kumar, A. , Choudhary, R. , Narzari, R. , & Kataki, R. (2018, February 21–22). Rheological evaluation of asphalt binders containing pyrolytic biochar. 17th Annual International Conference on Pavement Engineering, Asphalt Technology and Infrastructure . Liverpool, United Kingdom: Liverpool John Moores University.
- Mirzababaei, P. , Nejad, F. M. , & Vanaei, V. (2017). Investigation of rutting performance of asphalt binders containing warm additive. Petroleum Science and Technology , 35(1), 79–85. 2016.1247173. doi:10.1080/10916466
- MNRE . (2009). National biofuels policy . Ministry of new and renewable energy, Govt. of India. Retrieved May 15, 2017, from http://mnre.gov.in/file-manager/UserFiles/biofuel_policy.pdf
- Mohan, D. , Pittman, C. U. , & Steele, P. H. (2006). Pyrolysis of wood/biomass for bio-oil: A critical review. Energy and Fuels , 20(3), 848–889. doi:10.1021/ef0502397
- Oruç, Ş. , Yılmaz, B. , & Sancak, K. (2016). Effect of boron-containing additives on rheological properties of asphalt binder. Road Materials and Pavement Design , 17(4), 810–824. doi:10.1080/14680629.2015.1120228
- Pan, T. (2012). A first-principles based chemophysical environment for studying lignins as an asphalt antioxidant. Construction and Building Materials , 36, 654–664. doi:10.1016/j.conbuildmat.2012.06.012
- Singh, D. , Ashish, P. K. , Kataware, A. , & Habal, A. (2017). Evaluating performance of PPA-and-elvaloy-modified binder containing WMA additives and lime using MSCR and LAS tests. Journal of Materials in Civil Engineering , 29(8), 04017064:1–11. 1943-5533.0001934. doi:10.1061/(ASCE)MT
- Taha, R. , Ali, G. , & Delwar, M. (1998). Evaluation of coke dust-modified asphalt using Superpave. Journal of Materials in Civil Engineering , 10(3), 174–179. doi:10.1061/(ASCE)0899-1561(1998)10:3(174)
- Wasage, T. L. J. , Stastna, J. , & Zanzotto, L. (2011). Rheological analysis of multi-stress creep recovery (MSCR) test. International Journal of Pavement Engineering , 12(6), 561–568. doi:10.1080/10298436.2011.573557
- Williams, R. C. , & McCready, N. S. (2008). The utilization of agriculturally derived lignin as an antioxidant in asphalt binder. InTrans Project Reports , 14. Retrieved from http://lib.dr.iastate.edu/intrans_reports/14
- Zhao, S. , Huang, B. , Shu, X. , & Ye, P. (2014b). Laboratory investigation of biochar-modified asphalt mixture. Transportation Research Record , 2445, 56–63. doi:10.3141/2445-07
- Zhao, S. , Huang, B. , Ye, X. P. , Shu, X. , & Jia, X. (2014a). Utilizing bio-char as a bio-modifier for asphalt cement: A sustainable application of bio-fuel by-product. Fuel , 133, 52–62. doi:10.1016/j.fuel.2014.05.002
- Ziari, H. , Farahani, H. , Goli, A. , & Sadeghpour Galooyak, S. (2014). The investigation of the impact of carbon nano tube on bitumen and HMA performance. Petroleum Science and Technology , 32(17), 2102–2108. doi:10.1080/10916466.2013.763827