Abstract
In view of the combustion characteristics of annular chamber of micro gas turbine, a planar laser-induced fluorescence (PLIF) technique was used to investigate the methane/air premixed turbulent multiple flames in the annular combustor and the effect of CO2 on the characteristic parameters of flame structure was further discussed. The results show that the distribution of the OH radicals exhibits a ring structure with flame lift and bifurcation phenomena. The instantaneous and average image of OH-PLIF reveals that the multiple flames have the common characteristics of turbulent and laminar premixed flame structure. The quantitative analysis of the characteristic parameters of flame structure shows that the increase of CO2 concentration, the flame premixed conical structure is stretched, the overall flame chemistry reaction area is reduced and the intensity of local combustion is weakened; The Coanda effect causes the flame to bend towards the inner wall, and the relative bending of the outer flame increases as well as the flame angle.
PUBLIC INTEREST STATEMENT
Micro gas turbine is one of the most efficient technology with great development potential. Flame reaction zone parameters is the most concerned area in the study of combustion mechanism and industrial application. The study on the structure of the reaction zone of flame can provide help for the design of nozzles and combustors with high stability, high efficiency and low emission in industry. PLIF technology can well present the combustion state in the combustor. In this paper, the changing process of multi-flame interaction structure is obtained by PLIF and reveals the characteristics of annular turbulent flame. Besides, research shows the relationship between flame parameters and CO2 dilution quantitatively rather than qualitatively by setting characteristic parameters of flame structure.
1. Introduction
It is known that fossil energy reserves are limited and will cause serious pollution problems, so substitute energy is needed urgently. Micro gas turbine has the characteristics of high fuel adaptability, low emission, high power density and low maintenance cost (Waitz, Gauba, & Al Gautam, Citation1998). As a key part, the internal flow field changes and combustion situation of combustion chamber directly affect combustion stability, energy efficiency and pollutant emissions. The flame reaction zone is the key to the combustion mechanism research and industrial application of micro gas turbine (Liu, Citation2016). The study on the structure of the flame reaction zone can provide guidance for the optimization design of the combustion chamber (Bidabadi, Ghashghaei Nejad, Rasam, Sadeghi, & Shabani, Citation2018; Bo, Costa, Li, Aldén, & Bai, Citation2017; Santis, Ingham, Ma, & Pourkashanian, Citation2016; Zornek, Mosbach, & Aigner, Citation2018).
The annular combustor has been widely used in the micro gas turbine because of its small pressure loss, strong adaptability to different working conditions and no need for the interconnector. The special design of the premix nozzle increases the interaction between the flames and makes it easier to meet the ignition requirements. Worth and Dawson (Citation2013) studied the global flame dynamics of a model annular gas turbine combustor undergoing strong self-excited circumferential instabilities is presented. Wang et al. (Chao, Liu, Liu, Jiang, & Lin, Citation2015) had experimental investigation on detonation combustion patterns of hydrogen/vitiated air within annular combustor. Li, Peng, and Liu (Citation2006) simulated the annular combustion chamber and studied the influence of combustion and cooling on the annular structure of the flame. It was found that any diluent injection would cause local temperature higher near the diluent hole. Philip et al. (Sheehan, Citation2016) simulated the ignition process in the annular burner and compared it with the experimental data.
Planar Laser Induced Fluorescence (PLIF) combustion diagnostic technology represents the reaction region of the flame in the flow field by measuring the concentration of the hydroxyl radicals (it is also defined as OH radicals) (Häber, Gebretsadik, Bockhorn, & Zarzalis, Citation2015; Kutne, Meier, Boxx, Slabaugh, & Lucht, Citation2015; Zhu et al., Citation2016). Because of its high sensitivity, short response time and low disturbance of flow field, it has been widely used in the practice of combustion diagnosis. There have been a lot of studies using PLIF technology to study the combustion characteristics of wide range of syngas compustions, such as different ratios of CH4 and different dilutions (Yang, Shen, Hai, Wu, & Lu, Citation2015; Yong, Jeon, & Chang, Citation2006). Pu et al. (Citation2018) studied the premix combustion characteristics of methane syngas using OH-PLIF technology. The results showed that the increase of methane content and equivalent ratio weakened OH signal strength, and the increase of Vch4 also made the flame height higher, which inhibited the flame propagation speed. Liao and Hermanson (Citation2018) studied the structure of the flame reaction zone near the nozzle of the strong pulsed jet under the action of swirling flow based on PLIF technology. Data show that: In the early stage of combustion with swirl fuel injection, its tip discontinuity of the instantaneous image is not obvious. Jin, Tang, Wu, and Huang (Citation2014; Jin et al., Citation2013) studied the relationship between combustion velocity, structure and fuel composition of premixed flame of syngas, and found that preferential diffusion and flow field stretch acted on the change of flame structure. Wu et al. (Citation2018) extracted the conical flame contour from PLIF images of Bunsen burner flame and calculated the flame propagation rate.
The adiabatic temperature of premixed flame is high and the NOx emission is large. CO2 dilution can inhibit the generation of pollutants and reduce the flame temperature to affect the combustion process. Besides, biomass gas has high carbon dioxide content Therefore, it is extremely important to reveal the combustion characteristics and laws under the condition of CO2 dilution. Zhen et al. (Citation2017) studied the effects of varies diluents on temperature and concentration fields of OH radicals by using PLIF thermometry and bi-directional PLIF, and drew the conclusion that CO2 has excellent performance in reducing local high temperature of flame. Jourdaine, Mirat, Caudal, Lo, and Schuller (Citation2017) compared the stability of methane air and methane oxygen combustion under CO2 dilution premixed rotatory flow. The position of flame front and combustion gas was obtained by PLIF technology, and the shape of flame was deduced.
The structure of single flame, fuel composition and other influencing factors have been analyzed in detail in the above studies, however, the complex combustion chamber experiments with multiple flames interactions and the effect of CO2 on the size of flame structure are rarely involved. Previous experiment methods are difficult to capture the changing process of multi-flame interaction structure. In addition, numerical simulation is mostly used to study the combustion characteristics of multi-beam flame interaction in annular combustor compared to experiment.
In this paper, PLIF technique is used to measure the OH radical distribution of lean combustion premixed turbulent multiple flames under CO2 dilution in a annular combustion chamber. The changing process of multi-flame interaction structure inside the combustion chamber are studied and mainly analyzes the effects of CO2 dilution on multiple flames interaction structure. These work provide support for optimizing annular combustion chamber structure and achieving low emission combustion.
2. Experiment
2.1. Experimental system
The experimental system consists of OH-PLIF laser diagnostic system, air supply system and combustor, as shown in Figure . The air supply system consists of a high-pressure cylinder, a rotor flowmeter, a gas mixing chamber and a one-way valve. The gases of each component are fully premixed in the mixing chamber before entering the burner nozzle. In the process of the experiment, the Reynolds Numbers range from 800 to 2000, the equivalent ratio is 0.6 to 0.9 and the CO2 dilution is 4%, 8%, 12%, and 16%.
2.2. Combustor structure
The annular combustor is used according to the combustor structure of Capstone C65 micro gas turbine, and the size is determined by the principle of modeling. The material is made of quartz with good light transmittance and treated by dihydroxylation. The outer diameter of annular combustion chamber is 80 mm, the inner diameter is 40 mm, the ring width is 20 mm, and the overall height is 180 mm. The four nozzles are in the same plane, 30 mm away from the bottom of the chamber. The inner diameter d of the nozzle is 6 mm, and the wall thickness is 2 mm. The model is shown in Figure .
The combustion chamber is of annular structure, and four nozzles are evenly arranged along the circumference. Methane/air premixed gas enters the annular chamber from the nozzles for ignition and combustion.
2.3. Method of measurement
OH-PLIF system is mainly composed of Nd:YAG laser (Quanta-Ray,Spectra), dye laser (Cobra Stretch Series, Sirah Lasertechnik, GmbH), CCD camera (Imager ProX) and receiver. The Nd:YAG laser generates an initial wavelength of 1064 nm, which is divided into 1064 nm and 532 nm by a 1/2 glass slide, then, becomes 355 nm after stacking. The dye laser uses coumarin153-ethanol diluent as dye medium. The laser from YAG laser is tuned by the grating in the dye laser, dyed and converted into a laser with a wavelength of 283.5 nm after passing through the octave crystal. Equipment make the laser stay above 8 mJ to excite the OH radicals base transition in order to produce the fluorescence signal.
The fluorescence signal is received by the CCD camera, which is equipped with OH filter (LaVision, VZ14-0353) and ultraviolet lens (UV-60 mm, F3.5 s) in front of the lens to filter the interference signal. The CCD camera has a gate width of 100 ns and a gain of 70 ns to minimize the impact of ambient light. In each working condition, 200 transient OH-PLIF photos are continuously recorded with a frequency of 200 Hz and a period of 1 s. The recorded images are processed by system software Davis8 (LaVision, GmbH), besides, the background and noise were eliminated.
The measurement error is mainly affected by temperature, laser parameters and the collection system. The corresponding values for the uncertainties in the temperature, collisional quenching coefficient, and fluorescence signal are 1%, 5%, and 2.2%, respectively. Therefore, it can be concluded that the mean OH concentration measurements have a maximum uncertainty of ~17%. Related settings and contents have been referred to the earlier works by Krishna and Ravikrishna (Citation2015).
3. Results and discussions
3.1. Premixed turbulent flame shape
Methane and carbon dioxide flow remain unchanged, while air flow increases. The CCD image of premixed flame in annular combustion chamber is shown in Figure . The total flow rate ranges from 800 L/h to 2000 L/h. In the case of low air flow, the flame near the nozzle forms a stable cone-shaped blue premixed flame, which can clearly distinguish the outline of the inner and outer flame and the flame at the four nozzles do not interfere with each other. With the increase of air flow, the flame began to grow longer and fluctuate, and the four airflows began to influence each other. The flame changed from a stable conical state to a ring state, but it was still in an unstable state. When the nozzle velocity reaches –4 m/s, the flame at the four nozzles ignites with each other to make the ring structure tend to be stable and the flame distribution is more uniform. As the air flow continues to increase, the turbulence of the flow field increases continuously and the overall color of the flame becomes dark.
3.2. Effects of the multi-flame interaction structure
The instantaneous images of OH-PLIF of premix turbulent flame under different working conditions are shown in Figure . “t” in is the time that the photo is taken in one period. The changing process of multi-flame interaction structure is captured in figures and the flame front represented by OH radical presents a state of folding and curling with the change of time, which mainly appeared in the upstream and downstream of fuel jet. In addition, the premixed conical flame structure also produces distortion phenomenon. With the increase of CO2 dilution, the flame front becomes more unstable. The distribution area of OH fluorescence signal in the whole annular combustion chamber increases, but the combustion intensity in the flame chemical reaction area at the nozzle decreases. The structure of the flame front is closely related to the turbulence intensity. In the experiment, the turbulence vortex disturb the outer layer of the reaction zone of the flame, but not the preheating zone of the inner layer. The turbulence scale is approximately the same as the laminar flame thickness, so the flame retains a certain laminar flame structure and is located in the flame sheet model region. OH radicals mainly concentrate on the front of premixed flame. Due to the influence of the annular structure of combustion chamber and air flow disturbance, OH radicals formed in premixed combustion zone gradually spread outward. Under the action of high temperature, OH radical is slowly consumed and gradually formed the external front of the flame. With the decrease of the equivalent ratio, the discontinuous pulsation of the flame front changes with time, suggesting the phenomenon of local extinction and reignition of the turbulent front. Moreover, the flame cone structure was stretched, the width of the flame root decreased, and the length increased. The overall OH radicals fluorescence intensity of the combustion chamber is reduced.
Figure 4. Instantaneous image of premixed flame OH-PLIF in annular combustor. (a) φ = 0.7, VCO2 = 4%; (b) φ = 0.7, VCO2 = 12%; (c) φ = 0.5, VCO2 = 4%
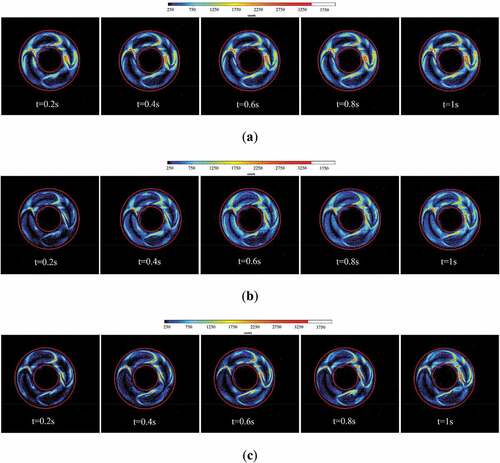
Under three working conditions, the distribution area of the OH group in the annular combustion chamber is the same, besides, the central symmetric premixed turbulent flame structure is formed outside the four nozzles, and the flame bifurcation phenomenon appears. Compared with the CCD image forming ring flame in Figure , it is observed that the premix flame in the instantaneous image of OH-PLIF is not formed at the nozzle, but is held up.
The increase in CO2 dilution, premixed flow cannot stabilize fire at the nozzle. Flame at the top of the upstream to the downstream flame roots extend, upstream of flame combustion products by diffusion and combustion instability changed the movement of downstream airflow, undermined its originally can satisfy the cone-shaped flame temperature and speed conditions. Furthermore, the lack of a fixed ignition source also promotes the diffusion of premixed gas to the outside in the annular space where flame lift occurs.
Under the same experimental conditions as in Figure , the average OH-PLIF image of methane premix turbulent flame obtained from the superposition of 200 instantaneous images is shown in Figure . Because the flame is in a turbulent state, the front of the instantaneous turbulent flame appears as a ruffled brush with a certain randomness. By superimposing and averaging several instantaneous images, the range of the fold area on the flame front can be obtained. Through a large number of data and image analysis, the fluorescence intensity of the fold area in this experiment ranges from 150 to 500 counts. The concentration of OH radicals in the reaction zone is significantly higher than that in the chemical equilibrium, which represents the main reaction zone of the flame.
Figure 5. Average OH-PLIF image of premixed flame in annular combustor. (a) φ = 0.7, VCO2 = 4%; (b) φ = 0.7, VCO2=12%; (c) φ = 0.5, VCO2 = 4%
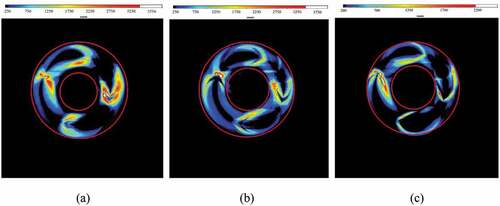
It can be seen from Figure (a) that the distribution of OH radicals in the combustion chamber forms a continuous ring structure, Besides, the OH radical distribution areas of each nozzle are connected with each other and maintain an obvious premixed conical flame structure. Figure (b) shows that the CO2 dilution increases, the thickness of the flame cone reaction zone decreases as well as the maximum OH group fluorescence intensity, but the overall distribution of OH radical have slightly change from Figure (a). With the increase of dilution, the occurrence of local high temperature is reduced, and the gradient of temperature field in the flame is slowed down, but it has little influence on the overall combustion intensity of the annular combustion chamber.
The results in Figure (c) show that the distribution of OH radicals in the combustion chamber cannot maintain a complete annular flame structure when the dilution is the same and the equivalent ratio is reduced. The mixed conical flame structure at the nozzle was stretched, the OH group distribution area and fluorescence intensity were reduced, and the fold degree of flame front was relatively reduced. The effect of methane concentration diffusion and flow field makes the flame length longer, but the effective area of combustion reaction zone decreases as a whole.
3.3. Characteristic parameters of flame structure in annular combustor
As shown in Figure , the bifurcated flame at the nozzle is divided into inner flame and outer flame, and the characteristic parameters of flame structure are defined to characterize the law of interaction between turbulence and flame. Where Li and Lo are the chord length of the inner flame and the outer flame respectively, and are the straight length between the root of the flame and the top of the flame; b represents the maximum thickness of the flame; f is the maximum distance from the middle camber line in the flame to its chord line; relative bending F is the percentage value of f and L; θ is the angle between the inner and outer flame chord lines.
Figure shows the variation of flame structure characteristic parameters with carbon dioxide concentration. As can be seen from Figure (a,b), the length of both the inner flame and the outer flame decrease with the increase of CO2 dilution; Figure (c,d) shows the variation of the thickness of inner and outer flame. The variation rules of them are basically the same and both show a downward trend. In addition, when equivalence ratio is relatively low, the influence degree is greater. The results of the experiment show that the VCO2 is improved, the temperature of the adiabatic flame decreases, besides, it reduces the speed and intensity of the fire, and the overall reaction zone of premixed flame shrinks. Furthermore, the chemical reaction retention time of premixed flame is very small under different CO2 dilution degree, and the difference becomes larger with the decrease of equivalent ratio in lean combustion zone.
Figure (e,f) shows the rule that the opening angle of flame and the relative bending of the outer flame change with the dilution of CO2. When the dilution increased, the opening angle between the inner and outer flame also increased; The higher the equivalence ratio, the greater the degree of the angle. The relative bending trend of the outer flame is the same as that of angle. These two characteristic parameters are closely related to the tensile action of flame. With the increase of CO2 dilution, the local tensile rate of the flame increases. A large amount of unburned gas in the flow field under the extension of the flow and contact with the outside air can continue to burn. So that the flame root area becomes narrower and the opening angle increases.
The increase of CO2 dilution increases the Reynolds number to some extent and promotes the Coanda effect. The Coanda effect is the tendency for a fluid to move away from its original direction of flow and instead follow the surface of the bulge (Bardia, Saraf, Maslow, Khabbaz, & Mahmood, Citation2016). If the D/r ratio (ratio of jet diameter to inner wall radius of curvature) is less than 0.5, the Coanda effect is observed. The D/r ratio of the annular combustion chamber model is 0.3. When the jet flows through the inner annular wall, the Coanda effect induces its inclination and stretches the frontal structure of the flame. Therefore, the width of the inner flame becomes narrower. Besides, under the combined action of the Coanda effect and the extrusion of the outer wall, the relative bending of the flame increases and the flame angle expands.
4. Conclusions
The OH radical distribution of methane premixed flame in annular combustor under different dilution conditions of carbon dioxide is experimentally studied by using PLIF technique. The effect of carbon dioxide dilution on combustion state was obtained by observing the instantaneous and average images of OH radical. The variation trend of characteristic parameters of flame structure was quantitatively analyzed, and the following conclusions were drawn:
Methane premixed flame presents typical turbulent combustion characteristics such as fold and crimp in the annular combustion chamber, and laminar premixed flame structure is also retained at the nozzle, which is located in the folded flame sheet pattern area. The geometrical structure of the annular combustion chamber keeps the OH radical in annular distribution, and the phenomenon of lift and flame bifurcation occurs.
The length and width of inner flame and outer flame decreased with the increase of VCO2,besides, the change trend of inner flame width was the largest, the overall flame reaction region was reduced, the relative bending of the outer flame is increased.as well as flame angle. The flame premixed conical structure is stretched and the flame combustion speed and intensity are reduced.
The Coanda effect causes the flame to bend toward the inner wall, thus affecting the relative bending of the outer flame and the angle.
Nomenclature
VCO2 | = | Volume fraction of CO2 in the syngas fuel(%) |
VCH4 | = | Volume fraction of CH4 in the syngas fuel(%) |
PLIF | = | Planar laser-induced fluorescence |
φ | = | Equivalence ratio (–) |
Li | = | Inner flame chord length (mm) |
Lo | = | Outer flame chord length (mm) |
bi | = | Maximum thickness of inner flame (mm) |
bo | = | Maximum thickness of outer flame (mm) |
f | = | Maximum distance from the arc in the flame to its string (mm) |
F | = | The percentage value of f and L (%) |
θ | = | Angle between the inner and outer flame strings (°) |
Acknowledgements
The authors gratefully acknowledge financial support from Chongqing Science & Technology Commission [grant number CSTC2015shmszx90010].
Additional information
Funding
Notes on contributors
Zhonghua Wang
Zhonghua Wang is a postgraduate and Tang Qiang is an associate professor in the College of Energy and Power Engineering at Chongqing University. Zhongqing Yang, associate professor, is mainly engaged in scientific research of clean energy conversion and environmental protection. Pengao He is Qiang Tang’s student. They are mainly engaged in energy utilization and conversion, system energy saving, Combustion Pollutant Control and so on. Qiang Tang has authored and co-authored over 50 peer-reviewed scholarly articles within his research interest.
References
- Bardia, A., Saraf, R., Maslow, A., Khabbaz, K., & Mahmood, F. (2016). The coanda effect. Anesthesia & Analgesia, 123(3), 582. doi:10.1213/ANE.0000000000001474
- Bidabadi, M., Ghashghaei Nejad, P., Rasam, H., Sadeghi, S., & Shabani, B. (2018). Mathematical modeling of non-premixed laminar flow flames fed with biofuel in counter-flow arrangement considering porosity and thermophoresis effects: An asymptotic approach. Energies, 11(11), 2945.
- Chao, W., Liu, W., Liu, S., Jiang, L., & Lin, Z. (2015). Experimental investigation on detonation combustion patterns of hydrogen/vitiated air within annular combustor. Experimental Thermal & Fluid Science, 66(1), 269–11. doi:10.1016/j.expthermflusci.2015.02.024
- Häber, T., Gebretsadik, M., Bockhorn, H., & Zarzalis, N. (2015). The effect of total reflection in PLIF imaging of annular thin films. International Journal of Multiphase Flow, 76, 64–72. doi:10.1016/j.ijmultiphaseflow.2015.06.009
- Jin, F., Tang, C., Wu, J., & Huang, Z. (2014). Effect of preferential diffusion and flame stretch on flame structure and laminar burning velocity of syngas Bunsen flame using OH-PLIF. International Journal of Hydrogen Energy, 39(23), 12187–12193. doi:10.1016/j.ijhydene.2014.06.043
- Jin, F. U., Tang, C., Jin, W. U., Thi, L. D., Huang, Z., & Yang, Z. (2013). Study on laminar flame speed and flame structure of syngas with varied compositions using OH-PLIF and spectrograph. International Journal of Hydrogen Energy, 38(3), 1636–1643. doi:10.1016/j.ijhydene.2012.11.023
- Jourdaine, P., Mirat, C., Caudal, J., Lo, A., & Schuller, T. (2017). A comparison between the stabilization of premixed swirling CO 2 -diluted methane oxy-flames and methane/air flames. Fuel, 201, 156-164. doi: 10.1016/j.fuel.2016.11.017.
- Krishna, & Ravikrishna. (2015). Quantitative OH planar laser induced fluorescence diagnostics of syngas and methane combustion in a cavity combustor. Combustion Science & Technology, 187(11), 1661–1682. doi:10.1080/00102202.2015.1047015
- Kutne, P., Meier, W., Boxx, I., Slabaugh, C., & Lucht, R. P. (2015). 3 kHz PIV/OH-PLIF measurements in a gas turbine combustor at elevated pressure. Proceedings of the Combustion Institute, 35(3), 3793–3802. doi:10.1016/j.proci.2014.06.090
- Li, L., Peng, X. F., & Liu, T. (2006). Combustion and cooling performance in an aero-engine annular combustor. Applied Thermal Engineering, 26(16), 1771–1779. doi:10.1016/j.applthermaleng.2005.11.023
- Liao, Y. H., & Hermanson, J. C. (2018). OH-PLIF imaging of the reaction zone in Swirled, strongly-pulsed jet diffusion flames with a low Reynolds number. Combustion Science & Technology, 190(4), 615-631. doi: 10.1080/00102202.2017.1403909.
- Liu, W. (2016). A theory for steady and self-sustained premixed combustion waves | Cogent OA. Cogent Engineering, 3(1), 1205308. doi:10.1080/23311916.2016.1205308
- Pu, G., Huang, B., Zhang, X., Du, J., Zhu, T., & Chen, B. (2018). Investigation of flame structure and burning intensity of partially premixed methane enrichment of syngas using OH-PLIF and kinetic simulation. Combustion Theory and Modelling, 22(3), 432–445. doi:10.1080/13647830.2017.1402128
- Santis, A. D., Ingham, D. B., Ma, L., & Pourkashanian, M. (2016). CFD analysis of exhaust gas recirculation in a micro gas turbine combustor for CO 2 capture. Fuel, 173, 146–154. doi:10.1016/j.fuel.2016.01.063
- Sheehan, A. (2016). Simulation of the ignition process in an annular multiple-injector combustor and comparison with experiments. Journal of Engineering for Gas Turbines & Power, 137(3), 031501.
- Waitz, I., Gauba, A., & Al Gautam, E. (1998). Combustors for micro-gas turbine engines. Transactions of the Asme Journal of Fluids Engineering, 12(1), 109. doi:10.1115/1.2819633
- Worth, N. A., & Dawson, J. R. (2013). Self-excited circumferential instabilities in a model annular gas turbine combustor: Global flame dynamics. Proceedings of the Combustion Institute, 34(2), 3127–3134. doi:10.1016/j.proci.2012.05.061
- Wu, B., Pu, G., Zhang, X., Huang, B., Du, J., & Cameselle, C. (2018). Study on the laminar burning velocity of Medium-Btu syngas flame with N2 dilution based on OH-PLIF technology. Cogent Engineering, 5(1), 1–14. doi:10.1080/23311916.2018.1536306
- Yang, Z., Shen, W., Hai, Z., Wu, Y., & Lu, J. (2015). Effects of inert dilution on the propagation and extinction of lean premixed syngas/air flames. Fuel, 157, 115–121. doi:10.1016/j.fuel.2015.05.007
- Yong, K. J., Jeon, C. H., & Chang, Y. J. (2006). Evaluation of the equivalence ratio of the reacting mixture using intensity ratio of chemiluminescence in laminar partially premixed CH-air flames. Experimental Thermal & Fluid Science, 30(7), 663–673. doi:10.1016/j.expthermflusci.2006.01.005
- Zhen, Y., Xin, Y., Peng, J., Wang, L., Dong, Z., Li, X., … Xu, H. (2017). Effects of N 2, CO 2 and H 2 O dilutions on temperature and concentration fields of OH in methane Bunsen flames by using PLIF thermometry and bi-directional PLIF. Experimental Thermal & Fluid Science, 81, 209–222. doi:10.1016/j.expthermflusci.2016.10.017
- Zhou, B., Costa, M., Li, Z., Aldén, M., & Bai, X. S. (2017). Characterization of the reaction zone structures in a laboratory combustor using optical diagnostics: From flame to flameless combustion. Proceedings of the Combustion Institute, 36(3), 4305-4312. doi: 10.1016/j.proci.2016.06.182.
- Zhu, J., Zhao, G., Long, T., Sun, M., Li, Q., & Liang, J. (2016). Simultaneous OH and CH_2O PLIF imaging of flame structures. Journal of Experiments in Fluid Mechanics, 30(5), 55-60. doi: 10.11729/syltlx20160026.
- Zornek, T., Mosbach, T., & Aigner, M. (2018). Optical measurements of a lcv-combustor operated in a micro gas turbine with various fuel compositions. Journal of Engineering for Gas Turbines and Power. doi:10.1115/1.4040908